Estimation of double-differential cross-sections of 9Be(p,xn) reaction for new nuclear data library JENDL-5
Abstract
Double-differential cross-sections of the 9Be(p,xn) reaction are newly evaluated based on the Wakabayashi’s function and neutronics analysis up to 12 MeV for a new nuclear data library, JENDL-5. We devoted our efforts especially to the re-optimization of the absolute cross-sections and the interpolation of the neutron energy spectra. Through the comparisons between the thick target yield measurements and Monte-Carlo simulations at different proton energies and neutron emission angles, we conclude that JENDL-5 gives the best evaluation in the world.
1.Introduction
The cross-section of the 9Be(p,xn) reaction is highly important for the design of neutron sources with small accelerators. However, its evaluated values in the world’s nuclear data libraries have large uncertainties since available experimental data-sets of the cross-sections are very scarce, and some data does not match each other. It is also because the study of the nuclear theories/modelings, which is often applied to the prediction of cross-sections or interpolation of experimental values, is still on a scientific challenge for the few-body systems, viz., light nuclei such as 9Be.
Looking at the status of absolute cross-section measurements which are available in the experimental database EXFOR [15], several data-sets are certainly found below ∼15 MeV. However, most of them were measured before the 70s, in which the data are given in a narrow energy region and discrepant each other by more than
In the nuclear data evaluation, the statistical theory [8] which is combined with nuclear structure models is often used for the interpolation of available experimental data. It is also applied to the prediction/estimation of differential and double-differential cross-sections (DDX). Indeed, our nuclear reaction model code CCONE [12] is known as one of the powerful tools for the nuclear data evaluation, with a minimal optimization of the model parameters therein, as it has been applied to the development of our nuclear data libraries, e.g., JENDL-4.0 [17]. However, it is difficult to apply such a kind of code to the light nuclei (
One of our special purpose nuclear data libraries, JENDL-4.0/HE [13], provides the evaluated cross-sections data up to 200 MeV to be used for the design of accelerator-based applications such as the neutron sources, accelerator-driven system for the transmutation of radioactive wastes, and so forth. Indeed, this library is implemented in the Particle and Heavy Ion Transport Code System (PHITS) code [16] for an optional use. This library certainly includes data of 9Be for incident protons, but we managed to apply the CCONE code to the data evaluation for 9Be despite the inaccuracy of the measurements and nuclear models mentioned above, which means the data for p+9Be in JENDL-4.0/HE could have a large uncertainty. Quite recently, one of the authors, Wakabayashi et al. [21], proposed a function which describes the cross section and DDX of 9Be(p,xn) where a number of parameters are optimized with selected experimental data-sets. The function is expected to be more suitable to the practical nuclear data evaluation since it is more flexible than the nuclear models.
The objectives of the present study are:
evaluation of the cross section for the 9Be(p,xn) reaction based on the Wakabayashi’s function,
generation of DDX with a physically reasonable interpolation scheme for the 9Be(p,xn) reaction based on the function
2.Data evaluation for 9Be(p,xn)
2.1.The Wakabayashi’s function
The energy dependence of the absolute cross-section is rather smooth except for the lower energy region (
2.2.Incorporation of the function to nuclear data file
In the present study, we incorporated the Wakabayashi’s function to a nuclear data file, which finally enables us to perform neutronics analyses with both MCNP and PHITS. In a first step, the energy dependent cross-section of the 9Be(p,xn) reaction was calculated with the function, taking care of the selection of the energy grids to fully describe the resonant shape in the lower energy region. In the next step, we produced data table of the neutron energy spectra from the function at different proton energies and emission angles. In this case, much attention was paid also to the determination of neutron energy nodes especially around the peak positions to minimize the data size. Such point-wise data-sets were compiled in the ENDF-6 format [20] file.11 Since a normalized angle-energy distribution was required to be compiled for each incident energy in the ENDF-6 format, we normalized DDX with respect to the neutron energy
Fig. 1.
Evaluated and measured cross-sections of the 9Be(p,xn) reaction.
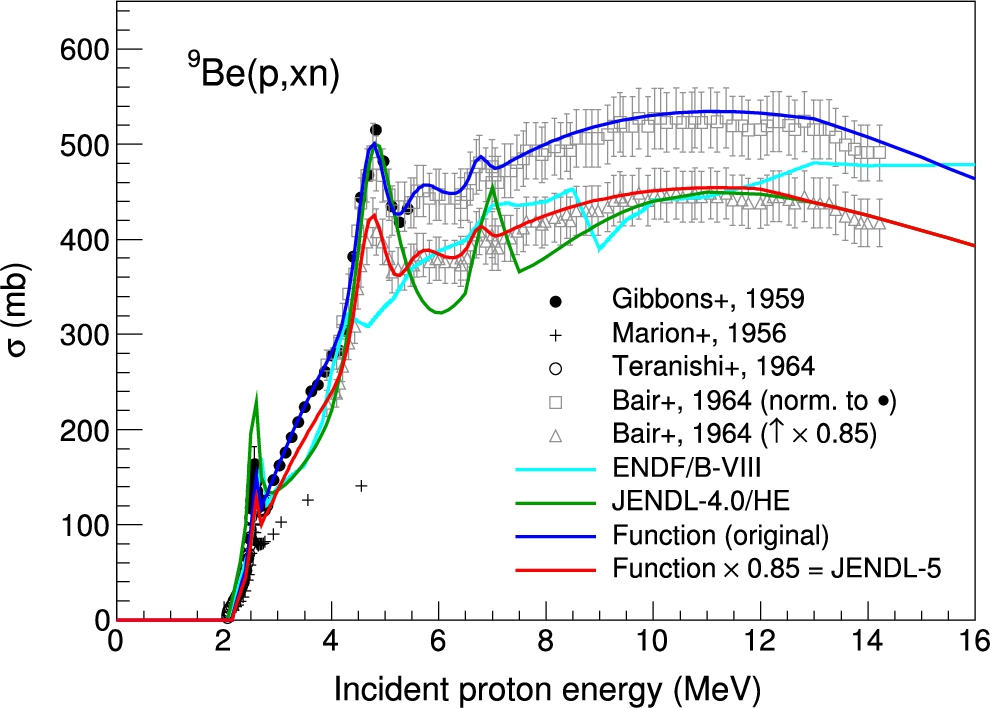
In compilation process, an interpolation scheme must be given to the data-tables because the data we generated from the function were forced to be discretized in principle. In the present study, the linear-linear interpolation was assumed for excitation function and also for each neutron energy spectrum at an emission angle. On the other hand, the two-dimensional unit-base [20] was assumed for the interpolation between the neutron spectra of two adjacent incident proton energies to minimize pile-ups of peaks which appear if the normal one-dimensional interpolation is used. Finally, we replaced the 9Be(p,xn) data in JENDL-4.0/HE with the present data tables from the threshold energy of the reaction to 12 MeV. Such a data merging is necessary because a complete data set of cross-sections (which includes those for the other types of reactions) is expected in the neutronics simulation.
Figure 1 shows the cross-section obtained from the function together with available experimental data [2,7,19] and evaluations. It is clear that the original function is designed to reproduce the measured data by Gibbons et al. [7] and the normalized data by Bair et al. [2]. Figure 2 illustrates an example of DDX extracted from the present data file with experimental data [3]. The two peaks observed in this spectrum correspond to the ground and excited states of 9B, with a full width at half maximum of 0.07 MeV [21]. As shown by one of the curves (INT = 2), it is difficult to produce sharp peaks correctly if we use the normal linear-linear interpolation. This issue encountered with the numerical data-table is solved in the present study with the unit-base interpolation, where we kept the ratio of the peak energy to maximum energy constant in the unit base, for the neutron spectrum interpolation as illustrated by the other curve (INT = 22). It is concluded that the function gives a reasonable estimation of the cross-section and DDX, and the applied interpolation schemes are reasonable.
Fig. 2.
Example of DDXs which are extracted from the present data file with the interpolation. The red curve (INT = 22) shows data with the unit-base interpolation while the blue curve (INT = 2) shows data with the normal linear-linear interpolation. Note that those curves are taken from the final data file (JENDL-5) where the absolute cross section is reduced by
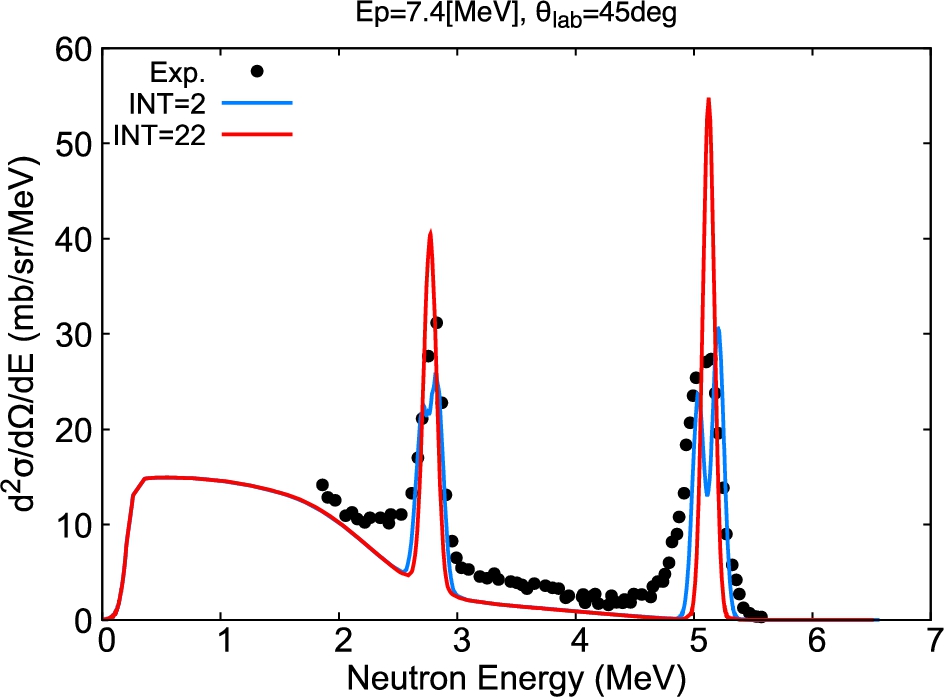
2.3.Neutronics analysis
Although the measured cross-sections including DDX are very scarce, there exists experimental data of TTY which were published in the current century, at different incident energies and emission angles, fortunately. Therefore, those measurements are quite useful to validate the present nuclear data file generated as described above. For example, the measured data by Howard et al. [9] are quite useful as they give neutron energy spectra at
Neutronics simulations were performed by the MCNP code [22] with the ACE files of the present data file (original function), JENDL-4.0/HE [13] and ENDF/B-VIII.0 [5]. Figure 3 illustrates an example of the TTY simulation results together with the experimental data by Howard et. al. [9]. The result obtained with ENDF/B-VIII.0 is not consistent with the shape of the measured data. That with JENDL-4.0/HE seems to give a better estimation, but it largely underestimates the experimental data in the lower energy region. It is obvious that the result (original function) with the present file based on the original Wakabayashi’s function simulates the measured neutron spectrum better than the existing libraries. However, the result with the present data overestimates the measured spectrum by
Fig. 3.
Example of simulation results for the thick target yield for
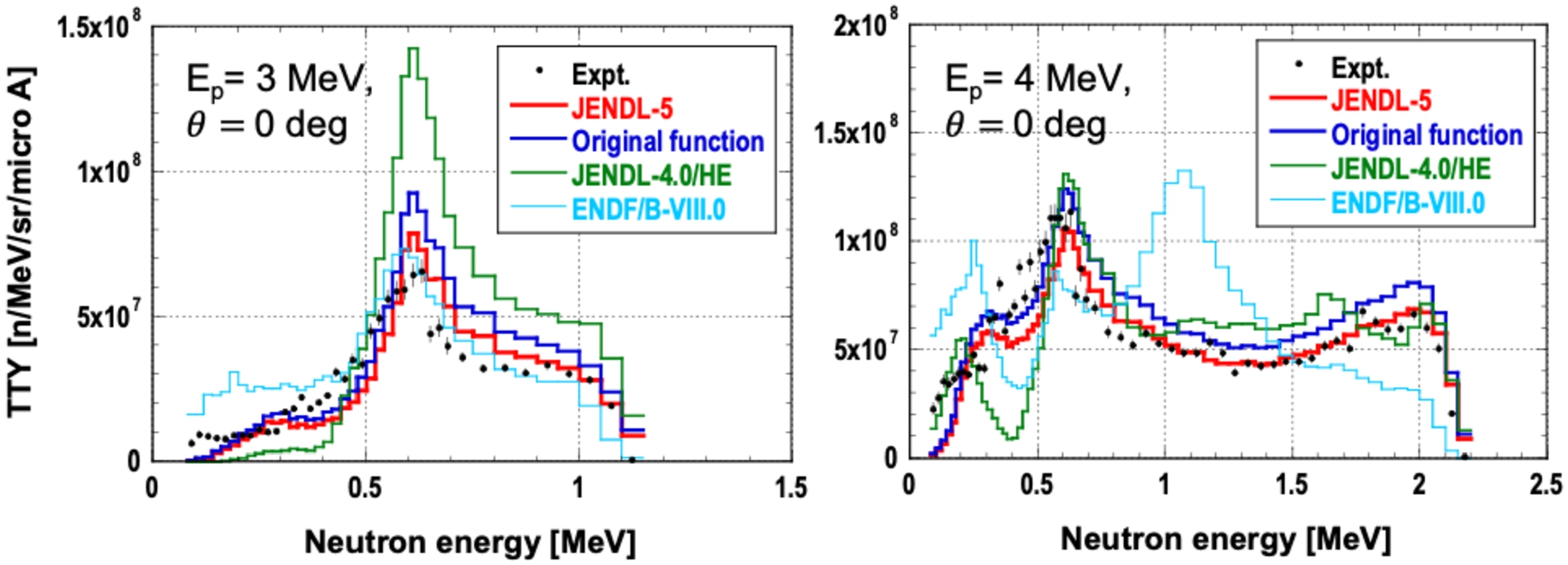
2.4.Estimation of cross-sections for JENDL-5
Even though we have confirmed that the function already gave the best estimation of TTY among the data libraries in the world, we noticed that the present data overestimated measured TTY systematically by
For the new nuclear data library JENDL-5, we finally decided to reduce the absolute cross-section by
2.5.JENDL-5 and remarks on unsolved issue of p + 9 Be evaluation
The new nuclear data library JENDL-5, which was compiled in the ENDF-6 format, was released in December 2021.22 This is the first major update of the previous version JENDL-4.0 which dates from 2010. The features of JENDL-5 relevant to the small accelerator might be summarised as follows:
revision of a large part of JENDL-4.0 such as neutron reaction data, thermal scattering law data
neutron activation cross section data
the first originally evaluated thermal scattering law data for 16 materials such as light and heavy water, benzene, and ethanol
reaction data induced by light charged particles (proton, deuteron and alpha-particle) and photon.
JENDL-5 application libraries including ACE format files will also be soon available. Touching upon the PHITS code, the current version of code package includes JENDL-4.0 in the ACE format. Similarly, JENDL-4.0/HE is also included for a number of nuclei relevant with accelerator applications. In the future, instead of JENDL-4 and JENDL-4/HE, JENDL-5 for neutrons below 20 MeV and for neutrons and protons up to 200 MeV for specific nuclides will be bundled to the PHITS package.
Although we have obtained the nuclear data evaluation for
Fig. 4.
Example of simulation results for the thick target yield at the backward angle.
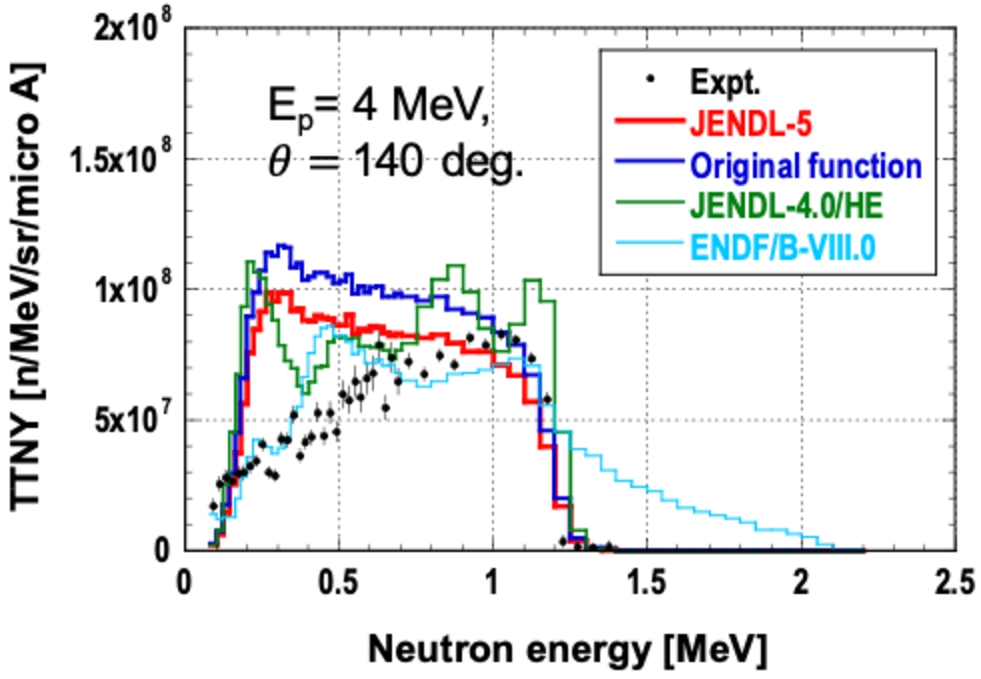
3.Conclusion
We have evaluated the cross section and DDX of the 9Be(p,xn) reaction based on the function proposed by Wakabayashi et. al. up to 12 MeV. Through the neutronics analysis, we finally decided to reduce the absolute cross-section by
Notes
1 We adopted the laboratory angle-energy law (MF = 6, LAW = 7) in the ENDF-6 format to compile the normalized spectra.
Acknowledgements
The authors would like to thank members of RIKEN/RANS for a useful discussion on this study.
References
[1] | J. Allison, K. Amako, J. Apostolakis, P. Arce, M. Asai, T. Aso, E. Bagli, A. Bagulya, S. Banerjee, G. Barrand, B.R. Beck, A.G. Bogdanov, D. Brandt, J.M.C. Brown, H. Burkhardt, P. Canal, D. Cano-Ott, S. Chauvie, K. Cho, G.A.P. Cirrone, G. Cooperman, M.A. Cortés-Giraldo, G. Cosmo, G. Cuttone, G. Depaola, L. Desorgher, X. Dong, A. Dotti, V.D. Elvira, G. Folger, Z. Francis, A. Galoyan, L. Garnier, M. Gayer, K.L. Genser, V.M. Grichine, S. Guatelli, P. Guèye, P. Gumplinger, A.S. Howard, I. Hřivnáčová, S. Hwang, S. Incerti, A. Ivanchenko, V.N. Ivanchenko, F.W. Jones, S.Y. Jun, P. Kaitaniemi, N. Karakatsanis, M. Karamitros, M. Kelsey, A. Kimura, T. Koi, H. Kurashige, A. Lechner, S.B. Lee, F. Longo, M. Maire, D. Mancusi, A. Mantero, E. Mendoza, B. Morgan, K. Murakami, T. Nikitina, L. Pandola, P. Paprocki, J. Perl, I. Petrović, M.G. Pia, W. Pokorski, J.M. Quesada, M. Raine, M.A. Reis, A. Ribon, A. Ristić Fira, F. Romano, G. Russo, G. Santin, T. Sasaki, D. Sawkey, J.I. Shin, I.I. Strakovsky, A. Taborda, S. Tanaka, B. Tomé, T. Toshito, H.N. Tran, P.R. Truscott, L. Urban, V. Uzhinsky, J.M. Verbeke, M. Verderi, B.L. Wendt, H. Wenzel, D.H. Wright, D.M. Wright, T. Yamashita, J. Yarba and H. Yoshida, Recent developments in Geant4, Nuclear Instruments and Methods in Physics Research Section A: Accelerators, Spectrometers, Detectors and Associated Equipment 835: ((2016) ), 186–225, https://www.sciencedirect.com/science/article/pii/S0168900216306957. doi:10.1016/j.nima.2016.06.125. |
[2] | J.K. Bair, C.M. Jones and H.B. Willard, Neutrons from the proton bombardment of 6Li, 7Li, 9Be, 11B and 18O, Nuclear Physics 53: ((1964) ), 209. doi:10.1016/0029-5582(64)90598-X. |
[3] | R.W. Bauer, J.D. Anderson and C. Wong, A search for an excited state of 9B near 1.7 MeV, Nuclear Physics 56: ((1964) ), 117. doi:10.1016/0029-5582(64)90458-4. |
[4] | A. Boudard, J. Cugnon, J.-C. David, S. Leray and D. Mancusi, New potentialities of the Liege intranuclear cascade model for reactions induced by nucleons and light charged particles, Phys. Rev. C 87: ((2013) ), 014606. doi:10.1103/PhysRevC.87.014606. |
[5] | D.A. Brown, M.B. Chadwick, R. Capote, A.C. Kahler, A. Trkov, M.W. Herman, A.A. Sonzogni, Y. Danon, A.D. Carlson, M. Dunn, D.L. Smith, G.M. Hale, G. Arbanas, R. Arcilla, C.R. Bates, B. Beck, B. Becker, F. Brown, R.J. Casperson, J. Conlin, D.E. Cullen, M.-A. Descalle, R. Firestone, T. Gaines, K.H. Guber, A.I. Hawari, J. Holmes, T.D. Johnson, T. Kawano, B.C. Kiedrowski, A.J. Koning, S. Kopecky, L. Leal, J.P. Lestone, C. Lubitz, J.I.M. Damián, C.M. Mattoon, E.A. McCutchan, S. Mughabghab, P. Navratil, D. Neudecker, G.P.A. Nobre, G. Noguere, M. Paris, M.T. Pigni, A.J. Plompen, B. Pritychenko, V.G. Pronyaev, D. Roubtsov, D. Rochman, P. Romano, P. Schillebeeckx, S. Simakov, M. Sin, I. Sirakov, B. Sleaford, V. Sobes, E.S. Soukhovitskii, I. Stetcu, P. Talou, I. Thompson, S. van der Marck, L. Welser-Sherrill, D. Wiarda, M. White, J.L. Wormald, R.Q. Wright, M. Zerkle, G. Žerovnik and Y. Zhu, ENDF/B-VIII.0: The 8th major release of the nuclear reaction data library with CIELO-project cross sections, new standards and thermal scattering data, Nuclear Data Sheets 148: ((2018) ), 1–142. Special Issue on Nuclear Reaction Data. |
[6] | J.L. Conlin and P. Romano, A Compact ENDF (ACE) Format Specification, Technical Report, LA-UR-19-29016, U.S. Department of Energy, Office of Scientific and Technical Information, 2019. doi:10.2172/1561065. |
[7] | J.H. Gibbons and R.L. Macklin, Total neutron yields from light elements under proton and alpha bombardment, Physical Review 114: ((1959) ), 571. doi:10.1103/PhysRev.114.571. |
[8] | W. Hauser and H. Feshbach, The inelastic scattering of neutrons, Phys. Rev. 87: ((1952) ), 366. doi:10.1103/PhysRev.87.366. |
[9] | W.B. Howard, S.M. Grimes, T.N. Massey, S.I. Al-Quraishi, D.K. Jacobs, C.E. Brient and J.C. Yanch, Measurement of the thick-target 9Be(p,n) neutron energy spectra, Nuclear Science and Engineering 138: ((2001) ), 145. doi:10.13182/NSE01-A2206. |
[10] | H. Iwamoto, O. Iwamoto and S. Kunieda, G-HyND: A hybrid nuclear data estimator with Gaussian processes, Journal of Nuclear Science and Technology 59: (3) ((2022) ), 334–344. doi:10.1080/00223131.2021.1971120. |
[11] | O. Iwamoto et al., Japanese Evaluated Nuclear Data Library version 5: JENDL-5, 2023, J. Nucl. Sci. Technol., to be published (accepted). |
[12] | O. Iwamoto, N. Iwamoto, S. Kunieda, F. Minato and K. Shibata, The CCONE code system and its application to nuclear data evaluation for fission and other reactions, Nucl. Data Sheets 131: ((2016) ), 259. doi:10.1016/j.nds.2015.12.004. |
[13] | S. Kunieda, O. Iwamoto, N. Iwamoto, F. Minato, T. Okamoto et al., Overview of JENDL-4.0/HE and benchmark calculations, in: Proceedings of the 2016 Symposium on Nuclear Data: JAEA-Conf 2016-004, (2017) , pp. 41–46. |
[14] | R.E. MacFarlane and A.C. Kahler, Methods for processing ENDF/B-VII with NJOY, Nuclear Data Sheets 111: (12) ((2010) ), 2739–2890. doi:10.1016/j.nds.2010.11.001. |
[15] | N. Otuka, E. Dupont, V. Semkova, B. Pritychenko, A.I. Blokhin, M. Aikawa, S. Babykina, M. Bossant, G. Chen, S. Dunaeva, R.A. Forrest, T. Fukahori, N. Furutachi, S. Ganesan, Z. Ge, O.O. Gritzay, M. Herman, S. Hlavač, K. Katō, B. Lalremruata, Y.O. Lee, A. Makinaga, K. Matsumoto, M. Mikhaylyukova, G. Pikulina, V.G. Pronyaev, A. Saxena, O. Schwerer, S.P. Simakov, N. Soppera, R. Suzuki, S. Takács, X. Tao, S. Taova, F. Tárkányi, V.V. Varlamov, J. Wang, S.C. Yang, V. Zerkin and Y. Zhuang, Towards a more complete and accurate experimental nuclear reaction data library (EXFOR): International collaboration between nuclear reaction data centres (NRDC), Nuclear Data Sheets 120: ((2014) ), 272–276. doi:10.1016/j.nds.2014.07.065. |
[16] | T. Sato, Y. Iwamoto, S. Hashimoto, T. Ogawa, T. Furuta, S-i. Abe, T. Kai, P.-E. Tsai, N. Matsuda, H. Iwase, N. Shigyo, L. Sihver and K. Niita, Features of Particle and Heavy Ion Transport code System (PHITS) version 3.02, Journal of Nuclear Science and Technology 55: (6) ((2018) ), 684–690. doi:10.1080/00223131.2017.1419890. |
[17] | K. Shibata, O. Iwamoto, T. Nakagawa, N. Iwamoto, A. Ichihara, S. Kunieda, S. Chiba, K. Furutaka, N. Otuka, T. Ohsawa, T. Murata, H. Matsunobu, A. Zukeran, S. Kamada and J. Katakura, A new library for nuclear science and engineering, J. Nucl. Sci. Technol. (Tokyo) 48: ((2011) ), 1–30, JENDL-4.0. doi:10.1080/18811248.2011.9711675. |
[18] | E.Sh. Soukhovitskiĩ, S. Chiba, R. Capote, J.M. Quesada, S. Kunieda and G.B. Morogovskiĩ, Supplement to OPTMAN code, Manual Version 10 (2008), JAEA-Data/Code, 2008-025, Japan Atomic Energy Agency, 2008. |
[19] | E. Teranishi and B. Furubayashi, Level width of the ground state of 9B, Physics Letters 9: ((1964) ), 157. doi:10.1016/0031-9163(64)90124-6. |
[20] | A. Trkov, M. Herman and D.A. Brown (eds), ENDF-6 Formats Mannual: Data Formats and Procedures for the Evaluated Nuclear Data Files ENDF/B-VI, ENDF/B-VII and ENDF/B-VIII, Written by the Members of the Cross Sections Evaluation Working Group, National Nuclear Data Center Brookhaven National Laboratory Upton, NY 11973-5000, USA, 2018. |
[21] | Y. Wakabayashi, A. Taketani, T. Hashiguchi, Y. Ikeda, T. Kobayashi, S. Wang, M. Yan, M. Harada, Y. Ikeda and Y. Otake, A function to provide neutron spectrum produced from the 9Be + p reaction with protons of energy below 12 MeV, Journal of Nuclear Science and Technology 55: (8) ((2018) ), 859–867. doi:10.1080/00223131.2018.1445566. |
[22] | C.J. Werner, J.S. Bull, C.J. Solomon, F.B. Brown, G.W. McKinney, M.E. Rising, D.A. Dixon, R.L. Martz, H.G. Hughes, L.J. Cox, A.J. Zukaitis, J.C. Armstrong, R.A. Forster and L. Casswell, MCNP Version 6.2 Release Notes, Technical Report, 2018-02-05, U.S. Department of Energy, Office of Scientific and Technical Information, 2018. doi:10.2172/1419730. |