Factors Associated with Respiratory Health and Function in Duchenne Muscular Dystrophy: A Systematic Review and Evidence Grading
Abstract
Background:
Despite advances in the medical management of the disease, respiratory involvement remains a significant source of morbidity and mortality in children and adults with Duchenne muscular dystrophy (DMD).
Objective:
The objective of this systematic literature review was to synthesize and grade published evidence of factors associated with respiratory health and function in DMD.
Methods:
We searched MEDLINE, Embase, and the Cochrane Library for records of studies published from January 1, 2000 (to ensure relevance to current care practices), up until and including December 31, 2022, reporting evidence of prognostic indicators and predictors of disease progression in DMD. The quality of evidence (i.e., very low to high) was assessed using the Grading of Recommendations, Assessment, Development and Evaluations (GRADE) framework.
Results:
The bibliographic search strategy resulted in the inclusion of 29 articles. In total, evidence of 10 factors associated with respiratory health and function in patients with DMD was identified: glucocorticoid exposure (high- to very low-quality evidence), DMD mutations (low-quality evidence), DMD genetic modifiers (low-quality evidence), other pharmacological interventions (i.e., ataluren, eteplirsen, idebenone, and tamoxifen) (moderate- to very low-quality evidence), body mass index and weight (low-quality evidence), and functional ability (low-quality evidence).
Conclusions:
In conclusion, we identified a total of 10 factors associated with respiratory health in function in DMD, encompassing both pharmacological therapies, genetic mutations and modifiers, and patient clinical characteristics. Yet, more research is needed to further delineate sources of respiratory heterogeneity, in particular the genotype-phenotype association and the impact of novel DMD therapies in a real-world setting. Our synthesis and grading should be helpful to inform clinical practice and future research of this heavily burdened patient population.
INTRODUCTION
In recent decades, improvements to the standard of care of Duchenne muscular dystrophy (DMD), a rare, X-linked neuromuscular disease characterized by progressive muscle degeneration, has significantly transformed prognosis of affected patients [1]. In addition to glucocorticoid therapy and proactive cardiac intervention, one key contributor to this development is improved respiratory management, in particular the routine use of mechanical ventilatory support in adults. Yet, despite these significant advances in care, respiratory involvement remains a significant source of morbidity and mortality in children and adults with DMD. Common complications include respiratory muscle fatigue, mucus plugging, atelectasis, pneumonia, and respiratory failure. In the absence of intervention (e.g., lung volume recruitment, assisted coughing, and assisted ventilation), patients are at risk of severe dyspnoea, atelectasis or pneumonia, and death due to respiratory arrest or respiratory-induced cardiac arrhythmias [2]. Moreover, in part due to limitations with current measures of functional ability (e.g., the six-minute walk test) due to non-trivial inter- and intra-patient variability, endpoints in randomized controlled trials (RCTs) are increasingly also defined in terms of respiratory outcomes [3, 4].
In spite of their importance for clinical practice and research, there is currently no up-to-date synthesis of factors significantly impacting respiratory health and function in children and adults with DMD. Indeed, in addition to informing respiratory care, counselling, and prognosis, such data would be expected to also help facilitate selection of patients to research (e.g., at different levels of risks of respiratory outcomes), as well as comparative analysis and contextualization of treatment efficacy as observed in single-arm trials [5]. To bridge this evidence gap, the objective of this study was to review, synthesize, and grade published evidence of factors associated with respiratory health and function in DMD.
MATERIALS AND METHODS
We conducted this systematic literature review according to the guidance provided in the Preferred Reporting Items for Systematic Reviews and Meta-Analyses (PRISMA) statement [6].
Search strategy
We implemented the bibliographic searches analogously to the strategy employed in our previously published scoping review of prognostic indicators of disease progression in DMD [7], revised for the review topic and updated to present date. Specifically, we searched MEDLINE (via Ovid), Embase (via Ovid), and the Cochrane Library (via the Wiley online platform) for records of studies published from January 1, 2000 (to ensure relevance to current care practices), up until and including December 31, 2022, reporting evidence of prognostic indicators and predictors of disease progression in DMD. The search string contained “Duchenne muscular dystrophy” as a Medical Subject Heading term or free text term, in combination with variations of the term “predictor” and “prognostic indicator” (full search strings are provided in the supplemental material online).
Table 1
PICOS eligibility criteria for study inclusion
Inclusion | Exclusion | |
Population | Patients diagnosed with DMD | None |
Intervention | Any | None |
Comparators | Any | None |
Outcome | Prognostic indicator/predictor of respiratory health and function | None |
Study design | Any | Systematic literature reviews were not formally included, but screened for relevant references |
Note: Population, Intervention, Comparison, Outcomes and Study design (PICOS). Duchenne muscular dystrophy (DMD).
Inclusion criteria
We employed eligibility criteria for study inclusion based on the Population, Intervention, Comparison, Outcomes and Study design (PICOS) framework identical to those specified in our previous scoping review [7] amended for the review topic (Table 1). We did not consider editorial letters or conference abstracts (as they lack details essential for meaningful synthesis and grading) and did not formally include identified systematic reviews (but screened their reference lists for potential publications).
Screening and data extraction
One investigator (EL) initially screened article titles and abstracts for eligibility, and subsequently reviewed full-text versions of selected records. The reason for exclusion was recorded and confirmed by a second investigator (SA). For all articles that met the inclusion criteria upon full-text review, the following information was extracted into a pre-specified data extraction form: Author; title; study year; geographical setting(s); study design; site(s)/data source(s); study period; sample population characteristics; case ascertainment; pharmacological interventions (incl. number of exposed, dose, and duration of exposure); outcome measures(s); method of analysis; and outcome results. For the purpose of this review, we only considered statistically significant factors of respiratory health and function (as reported in the included studies). Per previous research, we considered commonly used respiratory interventions, such as lung volume recruitment, cough assists, and assisted ventilation, to be milestones or outcomes indicative of increasing deterioration in respiratory function [2, 8–10] and did therefore not collate evidence pertaining to the effects of such devices and procedures. Finally, due to the progressive nature of DMD, we did not consider age a predictor of interest.
Upon identification of the relevant literature, two investigators (EL and SA) systematically screened reference lists of all included publications with the aim to identify additional records of interest not captured by the employed search strategy.
Level of evidence
The quality of the identified evidence of factors associated with respiratory health and function in DMD was assessed using the Grading of Recommendations, Assessment, Development and Evaluations (GRADE) framework [11]. GRADE rates the overall quality of evidence based on design limitations, risk of bias, consistency of the results across available studies, the precision of the results, directness, and likelihood of publication. The tool comprises of four levels of evidence, also known as certainty in evidence or quality of evidence: (1) very low (i.e., the true effect is probably markedly different from the estimated effect), (2) low (i.e., the true effect might be markedly different from the estimated effect), (3) moderate (i.e., the authors believe that the true effect is probably close to the estimated effect), and (4) high (i.e., the authors have a lot of confidence that the true effect is similar to the estimated effect). Per the GRADE manual, two investigators (EL and AA) independently provided an initial rating of all included records. Next, the quality of evidence at the outcome level was rated down for issues or limitations pertaining to study limitations, inconsistency of results, imprecision, indirectness of evidence, and publication bias, and/or rated up in case of a large magnitude of effect, a dose response, or if confounders are likely to minimize the effect. Finally, each investigator independently provided an overall GRADE quality rating of each outcome and study [11]. All GRADE ratings were subsequently reviewed and confirmed by HL and RMQ.
Table 2
Characteristics of included studies
Author (year) [country] | Study design | Data source(s)/site(s) | Study period | Sample, n (age)† | Case ascertainment | Pharmacological intervention(s) | n (%) exposed† | Dose, mean | Duration of exposure, mean (SD) |
Angliss et al. (2020) [AU] [12] | Retrospective cohort study | Children’s Health Queensland (Brisbane, AU) | 2008–2018 | 29 patients with DMD (median age: 15 years, IQR: 10–18 years) | NR | Glucocorticoids (DFZ and/or PRED) | 21 (72%) | • DFZ: NR • PRED: 0.25 mg/kg/day | 1–5 years |
ACE inhibitors (agents NR) | 21 (72%) | NR | NR | ||||||
Spinal surgery | 7 (24%) | NR | NR | ||||||
Balaban et al. (2005) [NR] [13] | Retrospective cohort study | NR | NR | 49 patients with DMD (mean age: 14 years, range: 12–15 years) | Muscle biopsy, dystrophin genotyping studies, and EMG | Glucocorticoids (DFZ and/or PDN) | 30 (61%) | • DFZ: 0.9 mg/kg/day | • DFZ: 5.85 (1.5) years |
• PDN: 0.75 mg/kg/day | • PDN: 5.49 (1.98) years | ||||||||
Bello et al. (2020) [IT] [14] | Retrospective cohort study | Italian DMD Network (IT, multi-centre) | 1990–2018 | 327 patients with DMD (mean age: 12 years, range: 4–45 years) | Genetic testing; or dystrophin levels and/or overt muscle weakness by 5 years of age; or loss of ambulation by 13 years of age without glucocorticoid treatment or by 16 years of age with glucocorticoid treatment | Glucocorticoids (agents NR) | 134 (41%) | NR | 4.5 (3.9) years |
NIV | 87 (27%) | NA | NR | ||||||
Biggar et al. (2001) [CA] [15] | Retrospective cohort study | The Bloorview MacMillan Children’s Center (Toronto, CA) | 1993–1999 | 54 patients with DMD (mean age: 12 years, range: 7–15 years) | Age at onset of symptoms (<5 years of age), male sex, proximal muscle weakness, increased serum creatine kinase levels, and muscle biopsy and/or genetic testing | Glucocorticoids (DFZ) | 30 (56%) | Initial dose • 0.9 mg/kg/day At 10 years of age • 0.76 (0.19) mg/kg/day At 15 years of age • 0.61 (0.20) mg/kg/day | 3.2 (1.3) years |
Biggar et al. (2006) [CA] [16] | Retrospective cohort study | The Bloorview MacMillan Children’s Center (Toronto, CA) | 1990–2004 | 74 patients with DMD (mean age: 15 years, range: 10–18 years) | Age at onset of symptoms (<5 years of age), male sex, proximal muscle weakness, increased serum creatine kinase levels, and muscle biopsy and/or genetic testing | Glucocorticoids (DFZ) | 40 (54%) | Initial dose • 0.9 mg/kg/day At 10 years of age • 0.8 (0.18) mg/kg/day At 15 years of age • 0.55 (0.09) mg/kg/day At 18 years of age • 0.5 (0.2) mg/kg/day | 5.5 years |
Buyse et al. (2011) [BE] [17] | RCT | NR | 2005–2007 | 21 patients with DMD (mean age: 12 years, range: 8–16 years) | NR | Idebenone | 13 (62%) | 450 mg/day | 52 weeks |
Daftary et al. (2007) [US] [18] | Case-control study | Cincinnati Children’s Hospital Medical Center (Ohio, US) | NR | 35 patients with DMD (mean age: 12 years, range: 8–17 years) | Genetic testing or muscle biopsy | Glucocorticoids (DFZ and/or PDN) | 10 (29%) | Initial dose • DFZ: 0.9 mg/kg/day • PDN: 0.75 mg/kg/day | 8.2 (range: 1–14) years |
Escolar et al. (2011) [US] [19] | RCT | 12 institutions in the CINRG network (names and locations NR) | NR | 64 patients with DMD (mean age: 7 years, range: 4–10 years) | NR | Glucocorticoids (PDN) | 64 (100%) | Daily dose • 0.75 mg/kg/day Weekend dose • 10 mg/kg/week | 12 months |
Fayssoil et al. (2021) [FR] [20] | Retrospective cohort study | Neuromuscular clinic (name and location NR) | 2015–2018 | 74 patients with DMD (median age: 22 years, range: 19–25 years) | NR | Glucocorticoids (DFZ or PDN) | 14 (19%) | • DFZ: NR • PDN: 0.75 mg/kg/day | NR |
Henricson et al. (2013) [*] [21] | Prospective cohort study | Multi-country, multi-centre (CINRG DNHS) | 2005–2009 | 340 patients with DMD (mean age: NR, range: 2–28 years) | Clinical presentation, family history of DMD, and molecular diagnostic characterization of DMD-associated dystrophinopathy | Glucocorticoids (agents NR) | 210 (62%) | NR | NR |
Houde et al. (2008) [CA] [22] | Retrospective cohort study | The multidisciplinary Neuromuscular Clinic of the Marie-Enfant Rehabilitation Centre (Montreal, CA) | NR | 29 patients with DMD (mean age: NR, range: NR) | Muscle biopsy and/or genetic testing | Glucocorticoids (DFZ) | 8 (28%) | Initial dose • 0.9 mg/kg per day (adjusted according to evolution or side effects to a maximum of 1 mg/kg) | NR |
ACE inhibitors (agents NR) | NR | NR | NR | ||||||
Humbertclaude et al. (2012) [FR] [8] | Retrospective cohort study | The French dystrophinopathy database (UMD–DMD France) (FR, multi-centre) | NR | 262 patients with DMD (mean age: 11 years; range: NR) | Genetic testing and loss of ambulation before 13 years of age | Glucocorticoids (agents NA) | 0 (0%) | NA | NA |
Iff et al. (2022) [*] [23] | Indirect treatment comparison study | • Study 204 (open-label study; US, multi-centre [NCT02286947]) • Study 301 (open-label study; US, multi-centre [NCT02255552]) • CINRG DNHS (prospective cohort study; multi-country, multi-centre [NCT00468832]) | • Study 204 : 2014–2018 • Study 301 : 2014–2019 • CINRG-DNHS: 2006–2016 | Study 204 • 20 patients with DMD (mean age: 13 years; range: 10–17 years) Study 301 • 52 patients with DMD (mean age: 11 years; range: 10–16 years) CINRG DNHS • 20 patients with DMD (mean age: 12 years; range: 10–18 years) | • Study 204: NRa • Study 301: NRa • CINRG DNHS: NRa | Eteplirsen | • Study 204 : 20 (100%) • Study 301 : 52 (100%) • CINRG DNHS: 0 (0%) | NR | Specific to each study (see article for details) |
Glucocorticoids (agents NR) | • Study 204 : 20 (100%) • Study 301 : 52 (100%) • CINRG DNHS: 20 (100%) • DEMAND III: 11 (100%) | NR | NR | ||||||
Kelley et al. (2019) [*] [25] | Retrospective cohort study | CINRG DNHS (multi-country, multi-centre) | NR | 175 patients with DMD (mean age: 12 years, range: 3–25 years) | NRa | Glucocorticoids (agents NR) | NR | NR | 3.40 (SE: 0.31) years |
Kelley et al. (2022) [*] [24] | Retrospective cohort study | CINRG DNHS (multi-country, multi-centre) | NR | 169 patients with DMD (mean age: 12 years, range: 5–25 years) | NRa | Glucocorticoids (agents NR) | NR | NR | 3.40 (SE: 0.31) years |
Khan et al. (2019) [*] [26] | Indirect treatment comparison study | • Study 201 (RCT; US; single-centre [NCT01396239]) • Study 202 (open label, multiple dose extension study; US, multi-centre [NCT01540409]) • Study 204 (open-label study; US, multi-centre [NCT02286947]) • Study 301 (open-label study; US, multi-centre [NCT02255552]) • CINRG DNHS (prospective cohort study; multi-country, multi-centre [NCT00468832]) | • Study 201/202: NR • Study 204: NR • Study 301: NR • CINRG DNHS: NR | Study 201/202 • 12 patients with DMD (mean age: 10 years; range: 10–11 years) Study 204 • 20 patients with DMD (mean age: 13 years; range: 10–17 years) Study 301 • 42 patients with DMD (mean age: 11 years; range: 10–16 years) CINRG DNHS • 172 patients with DMD (mean age: 12 years; range: 10–18 years) | • Study 201/202: NRa • Study 204: NRa • Study 301: NRa • CINRG DNHS: NRa | Eteplirsen | • Study 201/202 : 12 (100%) • Study 204 : 20 (100%) • Study 301 : 42 (100%) • CINRG DNHS: 0 (0%) | • Study 201/202 : 30 or 50 mg/kg/week • Study 204 : 30 mg/kg/week • Study 301 : 30 mg/kg/week | Specific to each study (see article for details) |
Glucocorticoids (agents NR) | • Study 201/202 : 12 (100%) • Study 204: NR • Study 301 : 42 (100%) • CINRG DNHS: 0 (0%) | • Study 201/202: NR • Study 204: NR • Study 301: NR CINRG DNHS: NA | • Study 201/202:>24 weeks (mean NR) • Study 204: NR • Study 301:>24 weeks (mean NR) • CINRG DNHS: NA | ||||||
Kim et al. (2017) [US] [27] | Retrospective cohort study | MD STARnet (US, multi-centre) | NR | 660 patients with DMD (mean age: NR, range: NR) | Clinical presentation, genetic testing, muscle biopsy, and/or elevated creatine kinase levels, X-linked pedigree, and family history of DMD (based on genetic testing or muscle biopsy) | Glucocorticoids (DFZ, PDN, or PRED) | • Early treatment (mean age: 4 years): 59 (9%) • Late treatment (mean age: 8 years): 259 (39%) | NR | 5.9–6.4 years |
Koeks et al. (2017) [*] [28] | Retrospective cohort study | The TREAT-NMD global DMD database (multi-national, multi-centre) | 2007–2013 | 5,345 patients with DMD (mean age: NR, range: NR) | Genetic testing | Glucocorticoids (DFZ, PDN, or PRED) | Current use • 2,658 (50%) Past use • 522 (10%) | NR | NR |
Lee et al. (2016) [US] [29] | Cross-sectional | The Comprehensive Neuromuscular Center at Cincinnati Children’s Hospital Medical Center (Cincinnati, US) | 2013 | 43 patients with DMD (mean age: 16 years, range: 10–30 years) | Clinical presentation, elevated creatine kinase levels, and positive family history of DMD, or muscle biopsy and/or genetic testing | Glucocorticoids (DFZ or PDN) | 43 (100%) | NR | 8.9 (SD: 3.1) years |
LoMauro et al. (2018) [IT] [30] | Retrospective cohort study | Istituto di Ricovero e Cura a Carattere Scientifico (San Giovanni Rotondo, IT) | NR | 115 patients with DMD (mean age: NR, range: 6–24 years) | NR | Glucocorticoids (agents NR) | NR | NR | NR |
Mayer et al. (2017) [*] [31] | Post-hoc RCT analysis study | Multi-country, multi-centre | 2009–2012 | 64 patients with DMD (mean age: NR, range: 10–19 years) | NR | Idebenone | 31 (48%) | 900 mg/day | 52 weeks |
Glucocorticoids (agents NA) | 0 (0%) | NA | NA | ||||||
McDonald et al. (2018) [*] [9] | Prospective cohort study | CINRG DNHS (multi-country, multi-centre) | 2006–2009; 2012–2016 | 397 patients with DMD (mean: 11 years, range: 2–28 years) | Clinical and molecular diagnostic picture consistent with typical DMD (see article for details) | Glucocorticoids (DFZ, PDN, and/or PRED) | 335 (81%) | NR | 4.7–8.9 years |
McDonald et al. (2022) [*] [32] | Indirect treatment comparison study | • Study 019 (open-label study; multi-country, multi-centre [NCT01557400]) • CINRG DNHS (prospective cohort study; multi-country, multi-centre [NCT00468832]) | • Study 019: NR • CINRG DNHS: 2006–2016 | Study 019 • 45 patients with DMD (mean age: NR, range: NR) CINRG DNHS • 45 patients with DMD (mean age: NR, range: NR) | • Study 019: NRa • CINRG DNHS: NRa | Ataluren | 45 (50%) | 40 mg/kg/day | NRb |
Glucocorticoids (DFZ, PDN, and/or PRED) | NR | NR | NR | ||||||
Mitelman et al. (2022) [*] [33] | Indirect treatment comparison study | • Study 201 (RCT; US; single-centre [NCT01396239]) • Study 202 (open label, multiple dose extension study; US, multi-centre [NCT01540409]) • Study 405 (retrospective cohort study; US, multi-centre) • CINRG DNHS (prospective cohort study; multi-country, multi-centre [NCT00468832]) | NRa | Study 201/202/405 • 12 patients with DMD (mean age: 9 years; range: 7–11 years) CINRG DNHS • 20 patients with DMD (mean age: NR; range: NR) | • Study 201/202: NRa • Study 405: NRa • CINRG DNHS: NRa | Eteplirsen | 12 (14%) | 30 or 40 mg/kg/week | 5.72 (SD: 0.90) (range: 4.13–6.88) |
Glucocorticoids (DFZ or PDN) | 27 (100%) | NR | NR | ||||||
Pradhan et al. (2006) [IN] [34] | Retrospective cohort study | NR | NR | 34 patients with DMD (mean age: 9 years, range: NR) | Clinical presentation (e.g., difficulty rising from the floor and walking, calf hypertrophy, and positive Gower’s sign), increased creatine kinase levels, myogenic pattern on EMG, and deletion of the dystrophin gene | Glucocorticoids (PRED) | 15 (44%) | 0.75 mg/kg/day | NR |
Schara et al. (2001) [DE] [35] | Retrospective cohort study | NR | NR | 26 patients with DMD (mean: NR, range: NR) | NR | Glucocorticoids (DFZ) | 13 (50%) | 0.9 mg/kg/day | NR |
Silversides et al. (2003) [CA] [36] | Retrospective cohort study | The Bloorview MacMillan Children’s Center (Toronto, CA) | 1998–2002 | 33 patents with DMD (mean age: 15 years, range: 10–18 years) | Age at onset of symptoms (<5 years of age), male sex, proximal muscle weakness, increased serum creatine kinase levels, and muscle biopsy and/or genetic testing | Glucocorticoids (DFZ) | 21 (64%) | Initial dose • 0.9 mg/kg/day At 10 years of age • 0.76 (0.19) mg/kg/day At 15 years of age • 0.61 (0.20) mg/kg/day At 18 years of age • 0.59 (0.15) mg/kg/day | 5.1 (SD: 2.4) years |
ACE inhibitors (agents NR) | 6 (18%) | NR | NR | ||||||
Cardiotonic agents (digoxin) | 3 (9%) | NR | NR | ||||||
Trucco et al. (2022) [NL/FR/UK] [37] | Retrospective cohort study | The UK NorthStar Network (multi-site, UK) and the Association Française contre les Myopathies (AFM) Network (multi-site, FR) | 2003–2020 | 142 patents with DMD (mean age: 9 years, range: 5–NR) | NR | Glucocorticoids (agents NR) | 142 (100%) | NR | NR |
Tsabari et al. (2021) [IL] [38] | Prospective cohort study | Hadassah University Hospital (Jerusalem, IL) and the Duchenne Muscular Dystrophy Regulatory Science Consortium (D-RSC) Data Platform (country NR) | 2016–2019 | 97 patents with DMDd (mean age: 9 years, range: 6–14 years) | NR | Tamoxifen | 9 (9%)d | 20 mg/day | ≤3 years |
Glucocorticoids (agents NR) | 88 (100%)d | NR | NR |
Note: Australia (AUS). Belgium (BE). Canada (CAN). Confidence interval (CI). Cooperative International Neuromuscular Research Group Duchenne Natural History Study (CINRG DNHS). Deflazacort (DFZ). Duchenne muscular dystrophy (DMD). Electromyography (EMG). France (FR). India (IN). Inter-quartile range (IQR). Israel (IL). Italy (IT). Muscular Dystrophy Surveillance, Tracking, and Research Network (MD STARnet). Neuromuscular Reference Center (NMRC). Not applicable (NA). Not reported (NR). Prednisolone (PRED). Prednisone (PDN). Standard deviation (SD). Standard error (SE). The Netherlands (NL). United Kingdom (UK). United States of America (US). †Details for the sample analysed with respect to outcomes of respiratory health and function. *Multi-national (see article for details). aCase ascertainment details not reported but provided in referenced publications. bDistribution across categories of duration of treatment reported in the article. cMean age in treated patients: 9 years (range: 7–11 years). d13–88 untreated patients (depending on the assay).
RESULTS
The bibliographic search strategy resulted in the identification of 29 articles [8, 9, 12–38] (Table 2). Identified studies included patients with DMD from 10 countries (i.e., Australia, Belgium, Canada, France, Germany, India, Italy, the Netherlands, the United Kingdom [UK], and the United States of America [USA]). However, 38% (11 of 29) were based on multi-national cohorts and/or did not disclose patients’ country of residence. Additionally, several sets of studies were based on identical, or very similar, samples. In total, 10% (3 of 29) of articles described results from randomized research, 10% (3 of 29) from prospective cohort studies, 59% (17 of 29) from retrospective cohort studies, 14% (4 of 29) from indirect treatment comparison studies, 3% (1 of 29) from case-control studies, and 3% (1 of 29) from cross-sectional studies.
Factors associated with respiratory health and function in DMD
We identified evidence of 10 factors associated with respiratory health and function in patients with DMD, namely glucocorticoid exposure, DMD mutations, DMD genetic modifiers, other pharmacological interventions (i.e., ataluren, eteplirsen, idebenone, and tamoxifen), body mass index (BMI) and weight, and functional ability.
Glucocorticoid exposure
We identified one RCT reporting evidence of benefits of glucocorticoids on respiratory outcomes (Table 3). Specifically, Escolar et al. [19] compared the efficacy and safety of daily versus weekend prednisone among 64 US patients with DMD recruited via the Cooperative International Neuromuscular Research Group (CINRG). After 12 months of follow-up, although equivalence was met for the primary outcomes (i.e., muscle testing), patients receiving a weekend regimen had significantly higher predicted forced vital capacity (FVC) than those treated with a daily regimen (mean difference in change: 4.6%, 95% CI: –9.8 to 19.1). A weekend regimen was also associated with higher predicted forced expiratory volume in one second (FEV1) (mean difference in change vs. daily regimen: 6.1%, 95% CI: –9.1% to 20.4%).
Table 3
GRADE assessment of studies of factors associated with respiratory outcomes in DMD
Author (year) [country] | Predictor(s)/indicator(s) | Outcome measure(s) | Method of analysis | Outcome results | Initial GRADE | Outcome GRADE modification | Overall GRADE |
Angliss et al. (2020) [AU] [12] | Glucocorticoids (DFZ and/or PRED) | Maximum TcCO2 (mm Hg) | Descriptive (Mann-Whitney U test) | Mean (SD) across follow-up • 49.0 (5.0) mm Hg (treated) vs. 56.0 (3.7) mm Hg (untreated), p = 0.03. | Low | – | Low |
Maximum REM TcCO2 (mm Hg) | Mean (SD) across follow-up • 47.7 (6.3) mm Hg (treated) vs. 56.0 (3.7) mm Hg (untreated), p = 0.02. | – | |||||
FEV1 (litres) | Regression analysis (random-effects model) | Mean (95% CI) annual rate of decline • –0.005 (–0.32 to –0.02) litres (treated) vs. –0.07 (–0.11 to –0.04) litres (untreated), p < 0.01. | – | ||||
FEV1 (Z-score) | Mean (95% CI) annual rate of decline • –0.52 (–0.60 to 0.43) (treated) vs. –0.57 (–0.66 to –0.49) (untreated), p < 0.001. | – | |||||
FEV1 (% predicted) | Mean (95% CI) annual rate of decline • –6.55% (–7.57% to –5.54%) (treated) vs. –7.67% (–8.73% to –6.62%) (untreated), p < 0.001. | – | |||||
FVC (litres) | Mean (95% CI) annual rate of decline • 0.003 (–0.03 to 0.30) litres (treated) vs. –0.09 (–0.12 to –0.06) litres (untreated), p < 0.001. | – | |||||
FVC (Z-score) | Mean (95% CI) annual rate of decline • –0.58 (–0.67 to –0.50) (treated) vs. 0.68 (–0.75 to –0.60) (untreated), p < 0.001. | – | |||||
FVC (% predicted) | Mean (95% CI) annual rate of decline • –6.60% (–7.55% to –5.65%) (treated) vs. –7.39% (–8.23% to –6.54%) (untreated), p < 0.001. | – | |||||
Balaban et al. (2005) [NR] [13] | Glucocorticoids (DFZ and/or PDN) | FVC (litres) | Descriptive (Mann-Whitney U test) | Mean (SD) FVC change between the ages of 7 and 15 years • 0.75 (0.62) litres (DFZ) vs. 0.13 (0.35) litres (no treatment), p = 0.020. • 0.46 (0.50) litres (PDN) vs. 0.13 (0.35) litres (no treatment), p = 0.046. | Low | – | Low |
Bello et al. (2020) [IT] [14] | Glucocorticoids (agents NR) | FVC (% predicted) | Regression analysis (GEE model) | • β: 14.5, SE: 2.1, p < 0.0001. | Low | – | Low |
FEV1 (% predicted) | • β: 15.1, SE: 2.0, p < 0.0001. | – | |||||
PEF (% predicted) | • β: 14.2, SE: 1.9, p < 0.0001. | – | |||||
Mutation 3’ of exon 44 | FVC (% predicted) | • β: –6.1, SE: 2.3, p = 0.008. | – | ||||
Mutation type: skip 8 | FVC (% predicted) | • β: 13.8, SE: 8.3, p = 0.049.a | – | ||||
Mutation type: skip 44 | FVC (% predicted) | • β: 7.1, SE: 3.3, p = 0.016.a | – | ||||
Mutation type: skip 51 | FVC (% predicted) | • β: –5.7, SE: 2.3, p = 0.007.a | – | ||||
Mutation type: skip 53 | FVC (% predicted) | • β: –10.3, SE: 2.7, p < 0.0001.a | – | ||||
Splice site | FVC (% predicted) | • β: 13.9, SE: 7.0, p < 0.023. | – | ||||
Mutation 3’ of exon 44 | FEV1 (% predicted) | • β: 6.3, SE: 2.5, p = 0.011. | – | ||||
Mutation type: skip 8 | FEV1 (% predicted) | • β: 15.3, SE: 7.9, p = 0.027.a | – | ||||
Mutation type: skip 51 | FEV1 (% predicted) | • β: –7.5, SE: 4.3, p = 0.042. | – | ||||
Mutation type: skip 53 | FEV1 (% predicted) | • β: –6.6, SE: 3.2, p = 0.021.a | – | ||||
Nonsense | FEV1 (% predicted) | • β: –7.1, SE: 4.3, p = 0.048.b | – | ||||
Splice site | FEV1 (% predicted) | • β: 11.6, SE: 5.1, p = 0.011.a | – | ||||
Mutation 3’ of exon 44 | PEF (% predicted) | • β: –5.8, SE: 2.3, p = 0.010. | – | ||||
Mutation type: skip 8 | PEF (% predicted) | • β: 23.0, SE: 4.2, p < 0.0001. | – | ||||
Mutation type: skip 51 | PEF (% predicted) | • β: –5.7, SE: 3.4, p = 0.048. | – | ||||
Mutation type: skip 53 | PEF (% predicted) | • β: –5.9, SE: 2.7, p = 0.014.a | – | ||||
SNP modifier rs28357094 (dominant) | FVC (% predicted) | • β: –4.5, SE: 2.5, p = 0.020.a | – | ||||
SNP modifier rs1883832 (additive) | FVC (% predicted) | • β: –6.1, SE: 2.2, p = 0.005.b | – | ||||
SNP modifier rs1883832 (additive) | FEV1 (% predicted) | • β: –4.8, SE: 2.2, p = 0.030.b | – | ||||
SNP modifier rs28357094 (dominant) | PEF (% predicted) | • β: –8.7, SE: 3.1, p = 0.005.b | – | ||||
SNP modifier rs1883832 (additive) | PEF (% predicted) | • β: –4.1, SE: 1.8, p = 0.024.a | – | ||||
CD40 rs1883832 (additive) | NIV | Regression analysis (Cox proportional hazards model) | • HR: 1.71, 95% CI: 1.01 to 2.88, p = 0.044.b | – | |||
Biggar et al. (2001) [CA] [15] | Glucocorticoids (DFZ) | FVC (% predicted) | Descriptive (Student’s t-test) | Mean (SD) at 13 years of age • 82.5% (14%) (treated) vs. 43.2% (9%) (untreated), p < 0.002. Mean (SD) at 15 years of age • 88% (18%) (treated) vs. 39% (20%) (untreated), p < 0.001. | Low | – | Low |
Biggar et al. (2006) [CA] [16] | Glucocorticoid (DFZ) | FVC (% predicted) | Descriptive (Student’s t-test) | Mean (SD) at 10 years of age • 95% (14%) (treated) vs. 65% (13%) (untreated), p < 0.05. Mean (SD) at 15 years of age • 88% (12%) (treated) vs. 47% (19%) (untreated), p < 0.05. Mean (SD) at 18 years of age • 81% (13%) (treated) vs. 34% (10%) (untreated), p < 0.05. | Low | – | Low |
NIV | Descriptive (Fisher’s exact test) | Prevalence at 18 years of age • 0% (treated) vs. 46% (untreated), p < 0.05. | – | ||||
Buyse et al. (2011) [BE] [17] | Idebenone | PEF (litre/min) | Descriptive (Student’s t-test) | Mean (SD) change across follow-up (from baseline to 52 weeks) • 30.8 (54.4) litre/min (treated) vs. –13.8 (51.0) litre/min (untreated), p = 0.039. | High | Moderate (small sample size) | Moderate |
PEF (% predicated) | Mean (SD) change across follow-up (from baseline to 52 weeks) • 2.8% (13.8%) (treated) vs. –8.5% (13.8%) (untreated), p = 0.042. | ||||||
Daftary et al. (2007) [US] [18] | Glucocorticoids (DFZ and/or PDN) | PCF | Regression analysis (linear model) | • β: 27.3, SE: 12.1, t: 2.26, p < 0.0328. | Low | Very low (small sample size) | Very low |
Escolar et al. (2011) [US] [19] | Glucocorticoid (PDN) regimen (weekend and daily) | FVC (% predicted) | Descriptive (Student’s t-test) | Mean (95% CI) difference in change (weekend vs. daily regimen) across follow-up (from baseline to 12 months) • 4.6% (–9.8% to 19.1%), p-value (lower) = 0.03, p-value (upper) = 0.23. | High | – | High |
FEV1 (% predicted) | Mean (95% CI) difference in change (weekend vs. daily regimen) across follow-up (from baseline to 12 months) • 6.1% (–9.1% to 20.4%), p-value (lower) = 0.02, p-value (upper) = 0.31. | – | |||||
Fayssoil et al. (2021) [FR] [20] | Functional ability (the GMW Scale) | Right diaphragm TF (%) | Correlation analysis (Spearman’s Rho [r]) | • r = –0.45, p = 0.012. | Low | – | Low |
Left diaphragm TF (%) | • r = –0.75, p = 0.001. | – | |||||
Right diaphragm motion (mm) | • r = –0.53, p < 0.0001. | – | |||||
Left diaphragm motion (mm) | • r = –0.59, p < 0.0001. | – | |||||
BMI | Right diaphragm motion (mm) | • r = 0.38, p = 0.002. | – | ||||
Left diaphragm motion (mm) | • r = 0.41, p = 0.016 | – | |||||
Henricson et al. (2013) [*] [21] | Glucocorticoids (agents NR) | Invasive ventilation | Descriptive (χ2 test)c | Prevalence in patient > 18 years of age • 0% (treated) vs. 13% (untreated), p < 0.001. | Low | – | Low |
NIV | Prevalence in patient > 18 years of age • 32% (treated) vs. 56% (untreated), p < 0.001. | – | |||||
FVC (% predicted) | Descriptive (Kruskal-Wallis H test) | Mean for patients 10–12 years of age • 78% (treated [current user]) vs. 63% (untreated [naive]), p < 0.05. Mean for patients 13–15 years of age • 70% (treated [current user]) vs. 40% (untreated [naive]), p < 0.05. Mean for patients 16–18 years of age • 44% (treated [current user]) vs. 35% (untreated [naive]), p < 0.05. Mean for patients > 18 years of age • 32% (treated [current user]) vs. 22% (untreated [naive]), p < 0.05. | – | ||||
Houde et al. (2008) [CA] [22] | Glucocorticoids (DFZ) | FVC (% predicted) | Descriptive (Student’s t-test) | Mean (SD) at 16 years of age • 66% (14%) (treated) vs. 48% (22%) (untreated), p < 0.007. | Low | Very low (small sample size) | Very low |
Humbertclaude et al. (2012) [FR] [8] | Age at loss of ambulation: < 8 years (Group A),≥8 and < 11 years (Group B), and≥11 years (Group C) | FVC (% predicted) | Descriptive (Student’s t-test or Mann-Whitney U test) | Maximum (SD) value • 74.67% (13.71) (Group A) vs. 83.66% (14.15) years (Group B) vs. 85.01% (14.82) years (Group C), p = 0.005. Mean (SD) age at maximum value • 8.55 (1.83) years (Group A) vs. 9.81 (2.65) years (Group B) vs. 11.67 (2.63) years (Group C), p < 0.001. Mean (SD) change per year (descending phase) • 8.83% (6.37) (Group A) vs. 7.52% (4.22) (Group B) vs. 6.03% (2.94) (Group C), p = 0.003. Mean (SD) age at minimum value (descending phase) • 12.96 (4.06) years (Group A) vs. 16.19 (4.76) years (Group B) vs. 19.90 (6.68) years (Group C), p < 0.001. | Low | – | Low |
FVC (litres) | Maximum (SD) value • 1.596 (0.395) litres (Group A) vs. 1.971 (0.536) litres (Group B) vs. 2.569 (0.776) litres (Group C), p < 0.001. Mean (SD) age at maximum value • 10.26 (1.71) years (Group A) vs. 12.45 (2.04) years (Group B) vs. 14.58 (2.25) years (Group C), p < 0.001. Minimum (SD) value (descending phase) • 0.680 (0.401) litres (Group A) vs. 1.119 (0.599) litres (Group B) vs. 1.644 (0.943) litres (Group C), p < 0.001. Mean (SD) age at minimum value (descending phase) • 15.35 (3.37) years (Group A) vs. 17.89 (4.26) years (Group B) vs. 21.05 (6.76) years (Group C), p < 0.001. | – | |||||
Moderate respiratory insufficiency (FVC < 50%) | Mean (SD) age at first occurrence • 12.79 (2.32) years (Group A) vs. 15.12 (3.30) years (Group B) vs. 18.91 (4.61) years (Group C), p < 0.0001. | – | |||||
Correlation analysis (Spearman’s Rho [r]) | r = 0.54, p < 0.0001. | – | |||||
Severe respiratory insufficiency (FVC < 30%) | Descriptive (Student’s t-test or Mann-Whitney U test) | Mean (SD) age at first occurrence • 14.72 (1.89) years (Group A) vs. 18.11 (2.62) years (Group B) vs. 22.12 (5.72) years (Group C), p < 0.0001. | – | ||||
Correlation analysis (Spearman’s Rho [r]) | r = 0.67, p < 0.0001. | – | |||||
Death due to respiratory failure | Survival analysis (Gray’s test) | Cumulative incidence proportion at 20 years of age • 19% (Group A) vs. 4.3% (Group B) vs. 1.7% (Group C), p = 0.03. | – | ||||
Iff et al. (2022) [*] [23] | Eteplirsen | FVC (% predicted) | Regression analysis (mixed-effects model) | Mean annual change across follow-up • –3.47% (treated) vs. -5.95% (untreated), p = 0.0001. | Low | – | Low |
Kelley et al. (2019) [*] [25] | ADRB2 genotype variant (Gly16 and ARG16) | Nocturnal ventilation | Kaplan-Meier (log-rank test) | Mean (SE) (95% CI) age at first initiation • 21.80 (0.59) (20.86 to 23.42) years (Gly16) vs. 25.91 (1.31) (23.35 to 28.49) years (Arg16), p < 0.05. Median (SE) (95% CI) age at first initiation • 22.17 (0.40) (21.38 to 22.96) years (Gly16) vs. 28.30 (4.14) (20.19 to 36.41) years (Arg16), p < 0.05. | Low | – | Low |
Nocturnal ventilation | Regression analysis (Cox proportional hazards model) | HR (Gly16 vs. Arg16): 6.52, SE: 0.71, 95% CI: 1.64 to 25.99, p < 0.05. | – | ||||
Ambulatory | Nocturnal ventilation | HR (ambulatory vs. non-ambulatory): 0.05, SE: 0.79, 95% CI: 0.01–0.23, p < 0.05. | – | ||||
Weight | Nocturnal ventilation | HR (per kg): 1.02, SE: 0.01, 95% CI: 1.00–1.04, p < 0.05. | – | ||||
Kelley et al. (2022) [*] [24] | ADRB2 genotype variant (Gly16 vs. Arg16) | FVC (% predicted) | Regression analysis (GAMM) | Coefficient (Gly16 vs. Arg16): –5.80, SE: 1.32, T-value: –4.41, p < 0.001. | Low | – | Low |
FVC (litre) | Patients expressing the Gly16 polymorphism demonstrated higher absolute FVC between the ages of 5.0 and 12.8 years, and lower FVC between the ages of 15.9 and 25.0 years, compared with patients expressing the Arg16 polymorphism (p < 0.001) (GAMM coefficients NR). | – | |||||
Ambulatory | FVC (% predicted) | Coefficient (ambulatory vs. non-ambulatory): –15.11, SE: 1.68, T-value: –9.01, p < 0.001. | – | ||||
Glucocorticoids (agents NR) | FVC (% predicted) | Coefficient (duration in years; first-order smooths): 2.29, SE: 9.00, T-value: 39.28, p < 0.001. | – | ||||
Khan et al. (2019) [*] [26] | Eteplirsen | FVC (% predicted) | Regression analysis (linear mixed-effects model) | Mean (SE) (95% CI) difference in annual change across follow-up treated vs. untreated (10-18 years of age) (exon 51 CINRG DNHS natural history cohort) • Study 201/202 : 3.80% (0.819%) (2.19% to 5.42%), p < 0.001. • Study 204 : 2.34% (0.793%) (0.77% to 3.90%), p = 0.004. • Study 301 : 2.21% (0.919%) (0.40% to 4.02%), p < 0.017. Mean (SE) (95% CI) difference in annual change across follow-up treated vs. untreated (10-18 years of age) (genotyped CINRG DNHS natural history cohort) • Study 201/202 : 3.48% (0.735%) (2.03% to 4.92%), p < 0.001. • Study 204 : 2.01% (0.706%) (0.62% to 3.40%), p = 0.005. • Study 301 : 1.89% (0.845%) (0.23% to 3.54%), p = 0.026. Mean (SE) (95% CI) difference in annual change across follow-up treated vs. untreated (10–18 years of age) (total CINRG DNHS natural history cohort) • Study 201/202 : 3.37% (0.733%) (1.93% to 4.80%), p < 0.001. • Study 204 : 1.90% (0.703%) (0.52% to 3.28%), p = 0.007. • Study 301 : 1.77% (0.843%) (0.12% to 3.43%), p = 0.036. | Low | Very low (inconsistency of results) | Very low |
Kim et al. (2017) [US] [27] | Timing of initiation of glucocorticoids (DFZ, PDN, or PRED) (early treatment [age≤5 years] vs. late treatment [> 5 years]) | FVC (litre) | Regression analysis (GEE model) | β (early treatment [age≤5 years] vs. late treatment [> 5 years]): –0.39, SE: 0.12, p < 0.01. | Low | – | Low |
Late initiation (i.e., age > 5 years) of glucocorticoids (DFZ, PDN, or PRED) | β (late treatment [> 5 years] vs. no treatment): –0.21, SE: 0.10, p = 0.04. | – | |||||
Koeks et al. (2017) [*] [28] | Glucocorticoids (DFZ, PDN, or PRED) | Ventilation support | Descriptive (χ2 test) | Prevalence of ventilation support at end of follow-up in patients≥20 years of age • 28% (treated), 58% (previously treated), and 70% (never treated), p = 0.0001. | Low | – | Low |
Lee et al. (2016) [US] [29] | The Performance of the Upper Limb (PUL) score | FVC (% predicted) | Correlation analysis (Pearson’s correlation coefficient [r]) | r = 0.70, p≤0.0001. | Low | – | Low |
MIP (% predicted) | r = 0.52, p = 0.0042. | – | |||||
MIP (cmH2O) | r = 0.72, p = 0.0052. | – | |||||
MEP (% predicted) | r = 0.73, p < 0.0001. | – | |||||
MEP (cmH2O) | r = 0.81, p = 0.0008. | – | |||||
PCF (L/min) | r = 0.46, p = 0.0020. | – | |||||
LoMauro et al. (2018) [IT] [30] | Glucocorticoids (agents NR) | FVC (% predicted) | Regression analysis (see article for details) | Predicted FVC was higher among treated patients compared with untreated and previously treated patients, respectively, in the age range 15.1–21.3 years (p < 0.05). | Low | – | Low |
Mayer et al. (2017) [*] [31] | Idebenone | FVC (ml) | Regression analysis (random coefficient model) | Mean (95% CI) difference in change (treated vs. placebo) across follow-up (from week 13 to week 52) • 136 (50 to 223) ml, p = 0.003. | Low | – | Low |
FVC (litre) | Regression analysis (Cox proportional hazards model) | Patients with persistent decline by any margin across follow-up (from baseline to week 52) • HR (idebenone vs. placebo): 0.36 (95% CI: 0.19 to 0.67), p = 0.002. Patients with any relative decline of≥10% across follow-up (from baseline to week 52) • HR (idebenone vs. placebo): 0.35 (0.16 to 0.77), p = 0.009. Patients with a persistent relative decline of≥10% across follow-up (from baseline to week 52) • HR (idebenone vs. placebo): 0.39 (0.16 to 0.95), p = 0.039. | – | ||||
FVC (% predicted) | Regression analysis (random coefficient model) | Mean (95% CI) difference in change (treated vs. placebo) across follow-up (from week 13 to week 52) • 4.06% (1.28% to 6.84%), p = 0.005. | – | ||||
Regression analysis (Cox proportional hazards model) | Patients with persistent decline by any margin across follow-up (from baseline to week 52) • HR (idebenone vs. placebo): 0.43 (95% CI: 0.24 to 0.75), p = 0.003. Patients with any relative decline of≥10% across follow-up (from baseline to week 52) • HR (idebenone vs. placebo): 0.46 (0.26 to 0.81), p = 0.008. Patients with a persistent relative decline of≥10% across follow-up (from baseline to week 52) • HR (idebenone vs. placebo): 0.54 (0.30 to 1.00), p = 0.048. | – | |||||
McDonald et al. (2018) [*] [9] | Brooke Scale | FVC (% predicted) | Descriptive (test NR) | Mean (SD) at Brooke Scale scores • 85.2% (19.1%) at Brooke score 1, 73.3% (18.5%) at Brooke score 2, 57.3% (16.7%) at Brooke score 3, 50.3% (17.7%) at Brooke score 4, 30.9% (18.0%) at Brooke score 5, and 19.1% (13.5%) at Brooke score 6, p < 0.0001. | Low | – | Low |
Glucocorticoids (DFZ, PDN, and/or PRED) | Regression analysis (mixed-effects model) | Mean annual change in patients 7 to 18 years of age • β: –6.06, p < 0.001. | – | ||||
McDonald et al. (2022) [*] [32] | Ataluren | FVC (% predicted) | Kaplan-Meier (log-rank test) | Median age at predicted FVC < 60% • 18.1 years (treated) vs. 15.1 years (untreated), p = 0.0004. | Low | – | Low |
FVC < 1 litre | Survival functions (treated vs. untreated): p = 0.0039. | – | |||||
Mitelman et al. (2022) [*] [33] | Eteplirsen | FVC (% predicted) | Regression analysis (mixed-effects model) | Mean annual change across follow-up • –3.3% (treated) vs. –6.0% (untreated), p < 0.0001. | Low | Very low (small sample size) | Very low |
Pradhan et al. (2006) [IN] [34] | Glucocorticoids (PRED) | PEF (litre/sec) | Descriptive (Student’s t-test) | Changes between groups were significant between months 0–2 (p = 0.002) and months 2–4 (p = 0.023) (data NR). | Low | Very low (small sample size) | Very low |
Schara et al. (2001) [DE] [35] | Glucocorticoids (DFZ) | FVC (% predicted) | Descriptive (Mann-Whitney U test) | Median (range) across follow-up • 86.8% (62% to 122%) (treated) and 55% (10% to 105%) (untreated), p = 0.0173. | Low | Very low (small sample size) | Very low |
Silversides et al. (2003) [CA] [36] | Glucocorticoids (DFZ) | FVC (litres) | Descriptive (Student’s t-test) | Mean (SD) at end of follow-up • 2.0 (0.4) litres (treated) and 1.4 (0.5) litres (untreated), p = 0.001. | Low | – | Low |
FVC (% predicted) | Mean (SD) at end of follow-up • 83% (12%) (treated) and 41% (19%) (untreated), p < 0.001. | – | |||||
Trucco et al. (2022) [NL/FR/UK] [37] | DMD mutations amenable to skipping of exons 44, 45, 51, and 53 | FVC (% predicted) | Regression analysis (mixed-effects model) | Mean annual change across follow-up (after 9 years of age) • Exon 44: –2.7%, 95% CI: –4.5 to –1.0, p < 0.01. • Exon 45: –3.4%, 95% CI: –5.1 to –1.8, p < 0.01. • Exon 51: –5.9%, 95% CI: –7.1 to –4.8, p < 0.01. • Exon 53: –4.5%, 95% CI: –6.1 to –3.0, p < 0.01. | Low | – | Low |
Tsabari et al. (2021) [IL] [38] | Tamoxifen | FVC (% predicted) | Descriptive | Mean change after 12 months • 5.7% (p = NS) (treated) and –8.6% (p < 0.05) (untreated). | Low | Very low (small sample size) | Very low |
Note: Australia (AUS). Belgium (BE). Body mass index (BMI). Canada (CAN). Confidence interval (CI). Cooperative International Neuromuscular Research Group Duchenne Natural History Study (CINRG DNHS). Deflazacort (DFZ). Duchenne muscular dystrophy (DMD). Forced expiratory volume in one second (FEV1). Forced vital capacity (FVC). France (FR). Generalized additive mixed model (GAMM). Gardner Medwin Walton (GMW). Generalized estimating equation (GEE). Hazard ratio (HR). India (IN). Israel (IL). Italy (IT). Maximal voluntary ventilation (MVV). Maximum inspiratory pressure (MIP). Non-invasive ventilation (NIV). Not applicable (NA). Not reported (NR). Not significant (NS). Peak cough flow (PCF). Peak expiratory flow (PEF). Prednisolone (PRED). Prednisone (PDN). Rapid eye movement (REM). Standard deviation (SD). Standard error (SE). The Netherlands (NL). Thickening fraction (TF). Transcutaneous carbon dioxide (TcCO2). United Kingdom (UK). United States of America (US). aIn treated and untreated patients (i.e., meta-analysis cohort). bIn untreated patients (i.e., the CINRG DNHS cohort). cDerived p-value (based on data reported in the article).
We identified 13 observational studies reporting evidence of benefits of glucocorticoids on FVC in patients with DMD (Table 3). Compared with no treatment, significant improvements in predicted FVC were reported by Angliss et al. [12] in their study of 21 Australian patients receiving deflazacort and/or prednisolone (total n = 29) (mean annual rate of decline: –6.60% vs. –7.39%, p < 0.001 [random-effects model]); Bello et al. [14] in 134 Italian patients treated with glucocorticoids (agents not reported [NR]) (total n = 327) (estimated coefficient [β]: 14.5, SE: 2.1, p < 0.0001 [generalized estimating equation [GEE] model]); Biggar et al. [15] in 30 Canadian patients receiving deflazacort (total n = 54) (mean at 15 years of age: 88% vs. 39%, p < 0.001); Biggar et al. [16] in 40 Canadian patients treated with deflazacort (total n = 74) (mean at 18 years of age: 81% vs. 34%, p < 0.05); Henricson et al. [21] in a multi-national prospective cohort study (CINRG Duchenne Natural History Study [CINRG DNHS]) comprising of 210 patients treated with glucocorticoids (agents NR) (total n = 340) (mean for patients > 18 years of age: 32% vs. 22%, p < 0.05); Houde et al. [22] in 8 Canadian patients treated with deflazacort (total n = 29) (mean at 16 years of age: 66% vs. 48%, p < 0.007); Kelley et al. [24] in a multi-national sample comprising of 169 patients from the CINRG DNHS (based on regression analysis of duration of exposure; p < 0.001); LoMauro et al. [30] in Italian patients with DMD treated with glucocorticoids (n = NR, agents NR) (total n = 115) (predicted FVC was significantly higher among treated patients compared with untreated and previously treated patients, respectively, in the age range 15.1–21.3 years (p-value NR); McDonald et al. [9] in a multi-national cohort comprising of 335 patients from CINRG DNHS receiving either deflazacort, prednisone, and/or prednisolone (total n = 397) (mean annual change in patients 7 to 18 years of age: β: –6.06, p < 0.001 [mixed-effects model]); Schara et al. [35] in 13 German patients treated with deflazacort (total n = 26) (median across follow-up: 86.8% vs. and 55%, p = 0.0173); and Silversides et al. [36] in 21 Canadian patients treated with deflazacort (total n = 33) (mean at end of follow-up: 83% vs. 41%, p < 0.001). Moreover, in the retrospective cohort study by Balaban et al. [13], involving 49 patients with DMD (country NR), the mean change in FVC was significantly higher in those treated with deflazacort or prednisone compared with no treatment (0.75 litres [deflazacort] vs. 0.13 litres [no treatment], p = 0.02; and 0.46 litres [prednisone] vs. 0.13 litres [no treatment], p = 0.046). Kim et al. [27] found that late treatment with deflazacort, prednisone, or prednisolone was associated with improved FVC compared with early treatment (β: –0.39, p < 0.01 [generalized estimating equation model]), as well as no treatment (β: –0.21, p = 0.04 [generalized estimating equation model]), among 660 US patients with DMD. Angliss et al. [12] reported of a significantly reduced decline in FVC in patients treated with deflazacort and/or prednisolone compared with untreated patients (0.003 litres [95% CI: –0.03 to 0.30] vs. –0.09 litres [95% CI: –0.12 to –0.06], p < 0.001). Finally, Silversides et al. [36] also found that the mean FVC was higher in patients treated with deflazacort compared with no treatment (2.0 litres [treated] and 1.4 litres [untreated], p = 0.001).
Further benefits of glucocorticoids in DMD include improved FEV1 [12, 14]; maximum transcutaneous carbon dioxide (TcCO2) and rapid eye movement (REM) TcCO2 [12]; peak cough flow (PCF) [18]; predicted peak expiratory flow (PEF) [14, 34]; and lower prevalence of ventilation support [16, 21, 28].
DMD mutations and genetic modifiers
We identified four observational studies reporting evidence of effects of DMD mutations or genetic modifiers on respiratory health and function in DMD (Table 3). Specifically, in a retrospective cohort study encompassing 327 patients with DMD, Bello et al. [14] investigated the association between mutations and genetic modifiers and predicted FVC, predicted FEV1, predicted PEF, and use of non-invasive ventilation (NIV), respectively. Mutations situated 3’ of DMD intron 44 were associated with lower FVC, FEV1, and PEF, deletions amenable to skipping of exons 51 and 53 with lower FVC and PEF, and deletions amenable to skipping of exon 8 and exon 44 were associated with higher FVC, FEV1, and PEF. The SPP1 rs28357094 and CD40 rs1883832 minor alleles were found to be associated with lower FVC, FEV1, and PEF, and CD40 rs1883832 was associated with an increased risk of use of NIV (HR: 1.71 [95% CI: 1.01 to 2.88], p = 0.044). Moreover, based on data collected as part of the CINRG DNHS, Kelley et al. [25] found that patients with the ADRB2 genotype variant Gly16 required nocturnal ventilation at an earlier mean age than those with the Arg16 variant (21.80 years [95% CI: 20.86 to 23.42] vs. 25.91 years [95% CI: 23.35 to 28.49], p < 0.05). This corresponded to a higher average risk of nocturnal ventilation across follow-up (HR: 6.52 [95% CI: 1.64 to 25.99], p < 0.05). In a subsequent study based on the same data source [24], patients expressing the Gly16 polymorphism were reported to demonstrate systematically lower predicted FVC at any given age compared with patients expressing the Arg16 polymorphism (p < 0.01). Finally, in their retrospective cohort study comprising of 142 UK and French patients, Trucco et al. [37] estimated the mean annual change in predicted FVC across follow-up (after 9 years of age) at –2.7% for exon 44, –3.4% for exon 45, –5.9% for exon 51, and –4.5% for exon 53 (all p < 0.01).
Other pharmacological interventions
We identified three indirect treatment comparison studies that investigated the effect of eteplirsen on respiratory outcomes, all based on similar sample populations (Table 3). Specifically, Iff et al. [23] included 20 US patients from Study 204 (phase II; open-label), 52 US patients from Study 301 (phase III; open-label), as well as 20 untreated patients from the multi-country CINRG DNHS prospective cohort. Khan et al. [26] included 12, 20, and 42 patients from Study 201/202 (phase II RCT and open-label extension), Study 204, and Study 301, respectively, as well as 172 patients from CINRG DNHS. Finally, Mitelman et al. [33] included 12 patients from Study 201/202 and Study 405 (retrospective cohort study), as well as 20 patients from CINRG DNHS. Iff et al. [23] reported the mean annual change in predicted FVC across the (study-specific) follow-up at –3.47% for patients treated with eteplirsen and –5.95% for those not treated (p = 0.0001). Corresponding results by Mitelman et al. [33] were reported at –3.3% and –6.0%, respectively (p < 0.0001). Finally, Khan et al. [26] reported mean differences (treated vs. untreated) in annual changes in predicted FVC across follow-up by study (i.e., 201/202, 204, and 301) in relation to three comparator samples: (1) glucocorticoid-treated patients amenable to exon 51 skipping (exon 51 CINRG DNHS), (2) glucocorticoid-treated and genotyped patients (genotyped CINRG DNHS), and (3) all glucocorticoid-treated patients (total CINRG DNHS). Across the three studies (i.e., 201/202, 204, and 301), the mean difference in annual change (treated vs. untreated) was reported at between 2.21% and 3.80% in relation to the exon 51 CINRG DNHS cohort, 1.89% and 3.48% in relation to the genotyped CINRG DNHS cohort, and 1.77% and 3.37% in relation to the total CINRG DNHS cohort.
We identified two RCTs investigating the effect of idebenone on respiratory outcomes in patients with DMD. Specifically, in the RCT conducted by Buyse et al. [17], involving 21 Belgian patients followed for 12 months, there was a significant difference in the change in mean PEF (litre/min) from baseline to end of follow-up between patients treated with idebenone and untreated patients (30.8 vs. –13.8, p = 0.039). Additionally, Buyse et al. [17] estimated the mean change in predicted PEF across the 12-month follow-up at 2.8 and –8.5 for treated and untreated patients, respectively (p = 0.042). The RCT by Mayer et al. [31] included a multi-national sample of 64 patients with DMD followed for 52 weeks and found that patients treated with idebenone had significantly higher FVC at end of follow-up compared with placebo (mean difference in change: 136 ml [95% CI: 50 to 223], p = 0.003). Idebenone was also associated with lower risk of decline in FVC (decline by any margin, any relative decline of≥10%, and persistent relative decline of≥10%, all p≤0.039). Additionally, Mayer et al. [31] estimated the mean difference in change (idebenone vs. placebo) in predicted FVC across follow-up at 4.06 (95% CI: 1.28 to 6.84, p = 0.005). Idebenone was also associated with lower risk of decline in predicted FVC.
We identified one indirect treatment comparison study, McDonald et al. [32], examining benefits of ataluren in terms of predicted FVC. Specifically, in this study, encompassing 45 patients with DMD from a phase III open-label study (Study 019) and 45 from the CINRG DNHS prospective cohort, the median age at predicted FVC<60% (indicative of increasing deterioration in respiratory function and an elevated risk of hypoventilation [32]) was estimated at 18.1 years among patients treated with ataluren and 15.1 years in the natural history cohort (p = 0.0004). Additionally, there was a significant difference between ataluren and controls in the cumulative incidence proportion of patients reaching FVC < 1 litre (predictive of mortality within three years [32]) (p = 0.0039).
We identified one prospective observational study reporting of effects of tamoxifen on predicted FVC. Among 97 Israeli DMD patients, Tsabari et al. [38] reported that the mean change in predicted FVC after 12 months was not significantly different from zero in those treated with tamoxifen and –8.6% in untreated patients (p < 0.05).
Body mass index (BMI) and weight
We identified two retrospective cohort studies describing effects of BMI/weight on respiratory outcomes in DMD. Specifically, Fayssoil et al. [20] reported of a significant relationship between BMI and right diaphragm motion (r = 0.38, p = 0.002) and left diaphragm motion (r = 0.41, p = 0.016) among 74 French DMD patients. Additionally, Kelley et al. [25] found an increased risk of nocturnal ventilation in heavier patients (HR [per kg]: 1.02 [95% CI: 1.00–1.04], p < 0.05).
Functional ability
We identified four observational studies describing effects of functional ability on respiratory outcomes in DMD. Specifically, Fayssoil et al. [20] found the Gardner Medwin Walton scale (a single-item, generic measure of functional disability, with levels ranging from normal [0] to bedridden [10]) to be significantly associated with right diaphragm thickening fraction (TF) (r = –0.45, p = 0.012), left diaphragm TF (r = –0.75, p = 0.001), right diaphragm motion (r = –0.53, p < 0.0001), and left diaphragm motion (r = –0.59, p < 0.0001). Additionally, McDonald et al. [9] estimated mean predicted FVC across categories of Brooke scale scores among 397 patients from CINRG DNHS. Estimates ranged from 82.5% among patients with a Brooke score of 1 (“Starting with arms at the sides, the patient can abduct the arms in a full circle until they touch above the head” to 19.1% in those with a score of 6 (“Cannot raise hands to the mouth and has no useful function of hands”). Lee et al. [29] investigated the association between the Performance of the Upper Limb (PUL) score and respiratory outcomes in 43 US patients with DMD. Pearson’s correlation coefficient for predicted FVC was estimated at 0.70 (p≤0.0001). Finally, in two retrospective cohort studies based on data from CINRG DNHS, Kelley et al. [24, 25] found that ambulatory patients had a lower risk of requiring nocturnal ventilation compared with their non-ambulatory counterparts (HR: 0.05 [95% CI: 0.01 to 0.23], p < 0.05), as well as better FVC (p < 0.001).
Rating of the quality of the evidence
Per the manual of GRADE, we initially attributed included RCTs a high rating and observational studies a low rating. Next, we downgraded the rating for Buyse et al. [17], Daftary et al. [18], Houde et al. [22], Mitelman et al. [33], Pradhan et al. [34], and Schara et al. [35], and Tsabari et al. [38] due to a small sample size; and Khan et al. [26] due to inconsistency of results. Finally, we provided an overall rating of the quality of the evidence of each study (Table 3).
DISCUSSION
As a result of the pathophysiology of the disease, children with DMD typically experience the first signs and symptoms of compromised respiratory health and function –including difficulties with airway clearance and ventilation due to respiratory muscle degeneration and loss of chest wall –around the time at which they start becoming more fully dependent on wheelchairs for mobility. Yet, the trajectory of respiratory impairment over time varies greatly between, as well as within, patients [2]. Indeed, further insights into the sources of this heterogeneity would be expected to not only help improve respiratory disease management in general, including the specification of patient-centric therapies, but also inform counselling initiatives and discussions of prognosis with affected children and their families, as well as planning of future studies. To that end, in this systematic review, encompassing a total of 29 studies involving children and adults with DMD from 10 countries, we synthesized and graded the body of evidence of factors associated with respiratory health and function in DMD. In summary, our analysis identified 10 sets of predictors:
Glucocorticoid exposure (high- to very low-quality evidence);
• DMD mutations (low-quality evidence);
• DMD genetic modifiers (low-quality evidence);
• Other pharmacological interventions (i.e., ataluren, eteplirsen, idebenone, and tamoxifen) (moderate- to very low-quality evidence);
• BMI and weight (low-quality evidence); and
• Functional ability (low-quality evidence).
There is a considerable body of evidence demonstrating significant effects of glucocorticoids on respiratory health and function in DMD. In particular, compared with no treatment, patients receiving glucocorticoids have been found to have markedly greater FVC as measured at a range of different ages, including 15 years of age (88% vs. 39% [15]), 16 years of age (66% vs. 48% [22]), 18 years of age (81% vs. 34% [16]), and > 18 years of age (32% vs. 22% [21]). However, it is important to note that these estimates were derived from samples with varying demographic and clinical characteristics, as well as different glucocorticoid exposure profiles (e.g., criteria for exposure initiation, duration of exposure, dose, and regimen), and are therefore not directly comparable. Although we found few estimates pertaining to specific glucocorticoid agents or regimens, we did identify one retrospective cohort study [27] comparing late treatment initiation (i.e., at an age > 5 years) with deflazacort, prednisone, or prednisolone with early treatment (i.e., at an age≤5 years) and no treatment, respectively. Interestingly, late treatment was found to be associated with improved FVC compared with the other strata (and detrimental effects of early treatment were also reported for cardiomyopathy fracture risks). The authors note that the lower FVC observed in patients treated early could potentially reflect greater total body growth suppression from longer and/or early glucocorticoid treatment, but more research is needed to understand the mechanisms behind these results. Additionally, we identified one RCT [19] assessing the equivalence of daily versus weekend prednisone. Although the authors conclude that both regimens are equally safe and effective in preserving muscle strength and preventing BMI increases, a significantly higher predicted FVC was reported for children receiving the weekend regimen (mean difference in annual change: 4.6%).
We found evidence that mutations situated 3’ of DMD intron 44, deletions amenable to skipping of exons 51 and 53, the SPP1 rs28357094 and CD40 rs1883832 minor alleles, and the ADRB2 genotype variant Gly16 are associated with weaker muscle leading to worse respiratory outcomes [14, 24, 25] in DMD. In contrast, deletions amenable to skipping of exon 8 and exon 44 have been reported to be associated with better respiratory health and function [14]. That being said, in many studies, the causal effect of specific DMD mutations and/or genetic modifiers are not easily quantified due to concurrent exposure to pharmacological interventions, predominantly glucocorticoids. More research is thus needed to further establish the genotype-phenotype association in terms of respiratory health and function in DMD to inform treatment strategies, as well as the selection of patients to research.
Fig. 1
PRISMA diagram of the selection process of the included publications.
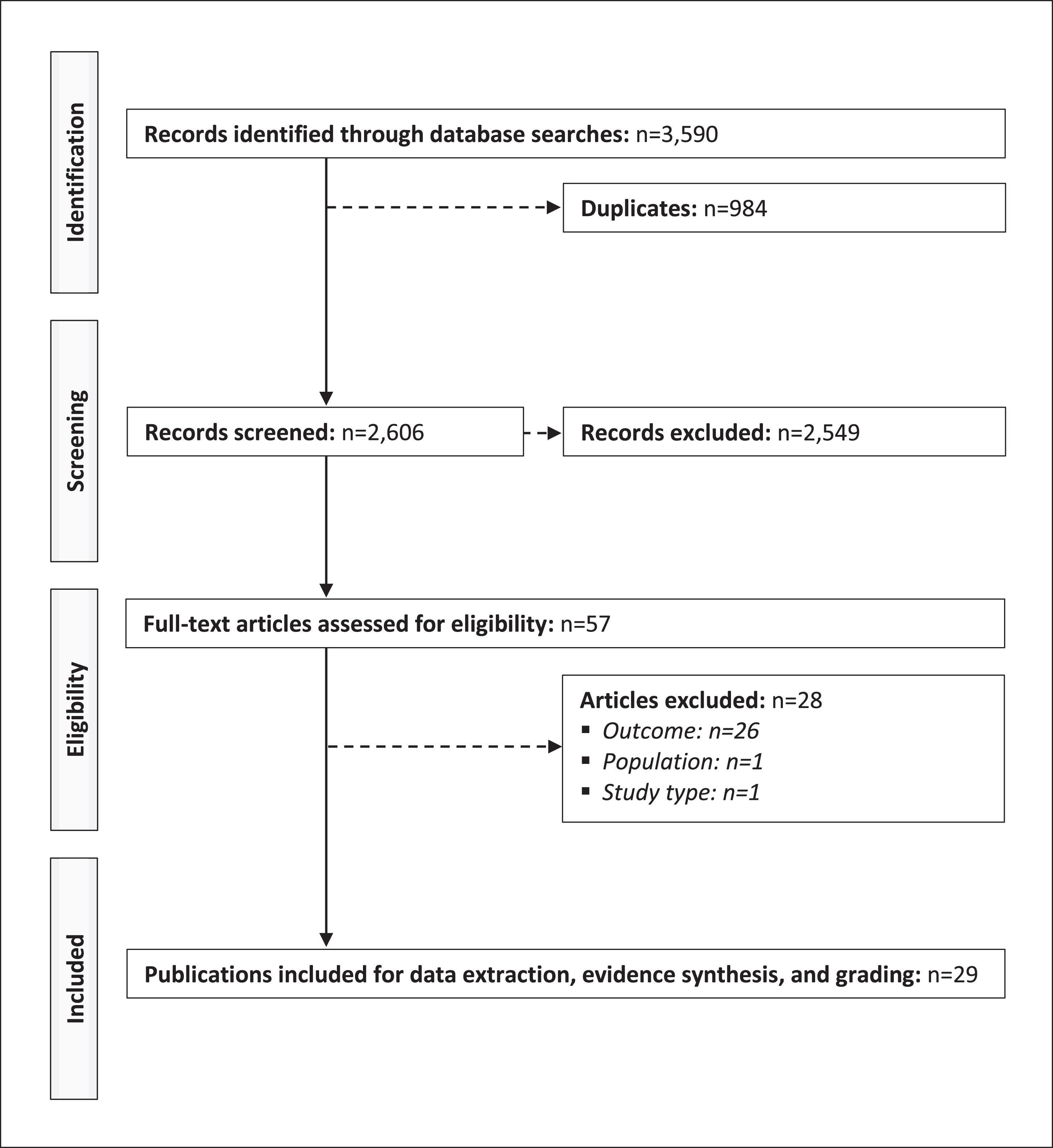
Ataluren is an orally administered, small-molecule compound for nonsense mutation DMD promoting readthrough of an in-frame premature stop codon to enable the production of full-length dystrophin. We found one indirect treatment comparison study [32] quantifying the benefits of this therapy in terms of respiratory outcomes in relation to natural history controls from CINRG DNHS. Specifically, based on propensity score matching, ataluren was shown to be associated with a three-year delay in predicted FVC decreasing to < 60%, as well as significant improvements in the cumulative incidence proportion of reaching an absolute FVC < 1 litre [9, 10].
We found three indirect treatment comparison studies [23, 26, 33] quantifying the effects of eteplirsen, a phosphorodiamidate morpholino oligomer promoting dystrophin production by restoring the translational reading frame of the DMD gene in patients with gene mutations amenable to exon 51 skipping. Specifically, by pooling treated patients from phase II and III clinical trials, as well as a retrospective cohort study, with natural history controls from CINRG DNHS, eteplirsen was shown to result in a significantly reduced annual decline in predicted FVC compared with standard of care (e.g., –3.47% vs. –5.95% [23] and –3.3% vs. –6.0% [33]).
We found two RCTs [17, 31] investigating the effect of idebenone –a short-chain benzoquinone with anti-oxidant properties –on FVC or PEF in children and adults with DMD, both of which demonstrated significant treatment effects versus no treatment. However, it is important to mention that subsequent clinical trials [39, 40] were terminated due to lack of efficacy and that idebenone currently is not approved nor recommended for use in patients with DMD [2].
We found one prospective observational study, Tsabari et al. [38], examining the effects of tamoxifen –a selective estrogen receptor modulator –on respiratory health and function in DMD. Although the mean annual change in predicted FVC was not statistically significant from zero in the exposed cohort, untreated patients experienced a significant decline of 8.6% (p < 0.05). Despite these data, analogous to idebenone, tamoxifen is currently not approved nor recommended for the treatment of DMD [2, 41].
We found two observational studies reporting of significant effects of BMI or weight on respiratory outcomes in DMD. Interestingly, Fayssoil et al. [20] described a positive association between BMI and right and left diaphragm motion, whereas Kelley et al. [25] found a negative association between weight and risk of requiring nocturnal ventilation support. More research is needed to further establish the impact of these factors, in particular considering the typical distribution of weight over time in DMD, where younger children commonly struggle with obesity due to glucocorticoid exposure and reduced physical activity and many adolescents and adults often become underweight due to functional impairment and feeding problems.
Functional ability, quantified by the Gardner Medwin Walton scale [20], the Brooke scale [9], and current ambulatory status [24, 25], was found to be significantly associated with several respiratory outcomes in DMD, including right and left diaphragm thickening fraction and motion, predicted FVC, and nocturnal ventilation. Similarly, Lee et al. [29] reported upper extremity functioning measured using the PUL to be significantly associated with predicted FVC (among other measures). Yet, little is known of the comparative ability of the above-mentioned scales, or other measures of functional ability, to accurately predict respiratory health and function in DMD and more research is needed to further establish the relationship between degeneration and wasting of different muscle groups and respiratory health and function in DMD.
Strengths of our review include the unrestrictive search strategy, as well as the comprehensive evidence grading. Concerning limitations, we were unable to perform a formal meta-analysis, including assessment of, for example, heterogeneity and publications bias, due to the wide range of respiratory outcomes identified. Additionally, we limited the search strategy to include only studies published after calendar year 2000, and we did not pursue grey literature, which means that data pertaining to the impact of some interventions might not have been fully identified. Finally, due to the observational nature of most of the synthesized evidence, conclusions regarding causality should be made with some caution.
In conclusion, we identified a total of 10 factors associated with respiratory health in function in DMD, encompassing both pharmacological therapies, genetic mutations and modifiers, and patient clinical characteristics. Yet, more research is needed to further delineate sources of respiratory heterogeneity, in particular the genotype-phenotype association and the impact of novel DMD therapies in a real-world setting. Our synthesis and grading should be helpful to inform clinical practice and future research of this heavily burdened patient population.
DECLARATIONS
FUNDING
This study was funded by PTC Therapeutics.
CONFLICTS OF INTEREST
Dr Alemán reports being sub-investigator of clinical trials in DMD sponsored by Pfizer and Reveragen, and receiving a research grant from PTC. Ms Zhang, Dr Werner, and Dr Tomazos are employees of PTC Therapeutics and may own stock/options in the company. Professor Lochmüller reports being principal investigator of clinical trials in DMD sponsored by Pfizer, PTC Therapeutics, Santhera, Sarepta, and Reveragen. Professor Quinlivan reports having received honoraria for teaching, consultancy, and iDMC membership from PTC therapeutics, Sanofi-Genzyme, Santhera, Sarepta, TRiNDS, and Astellas, as well as research and trial funding from PTC therapeutics, Santhera, and MDUK. The remaining authors have no conflicts of interest.
DATASETS/DATA AVAILABILITY STATEMENT
The data supporting the findings of this study are available within the article and/or its supplementary material.
SUPPLEMENTARY MATERIAL
[1] The supplementary material is available in the electronic version of this article: https://dx.doi.org/10.3233/JND-230094.
REFERENCES
[1] | Emery AEH . The muscular dystrophies. Lancet. (2002) ;359: (9307):687–95. |
[2] | Birnkrant DJ , Bushby K , Bann CM , Alman BA , Apkon SD , Blackwell A et al. Diagnosis and management of Duchenne muscular dystrophy, part 2: Respiratory, cardiac, bone health, and orthopaedic management. Lancet Neurol. (2018) ;17: (4):347–61. |
[3] | Finder J , Mayer OH , Sheehan D , Sawnani H , Abresch RT , Benditt J , et al. Pulmonary Endpoints in Duchenne Muscular Dystrophy. A Workshop Summary. Am J Respir Crit Care Med. (2017) ;196: (4):512–9. |
[4] | Mercuri E , Signorovitch JE , Swallow E , Song J , Ward SJ . Categorizing natural history trajectories of ambulatory function measured by the 6-minute walk distance in patients with Duchenne muscular dystrophy. Neuromuscul Disord. (2016) ;26: (9):576–83. |
[5] | Goemans N , Signorovitch J , Sajeev G , Yao Z , Gordish-Dressman H , McDonald CM , et al. Suitability of external controls for drug evaluation in Duchenne muscular dystrophy. Neurology. (2020) ;95: (10):e1381–e91. |
[6] | Page MJ , McKenzie JE , Bossuyt PM , Boutron I , Hoffmann TC , Mulrow CD , et al. The PRISMA 2020 statement: An updated guideline for reporting systematic reviews. Bmj. (2021) ;372: , n71. |
[7] | Ferizovic N , Summers J , de Zárate IBO , Werner C , Jiang J , Landfeldt E , et al. Prognostic indicators of disease progression in Duchenne muscular dystrophy: A literature review and evidence synthesis. PLoS One. (2022) ;17: (3):e0265879. |
[8] | Humbertclaude V , Hamroun D , Bezzou K , Berard C , Boespflug-Tanguy O , Bommelaer C , et al. Motor and respiratory heterogeneity in Duchenne patients: Implication for clinical trials. European journal of paediatric neurology: EJPN: Official journal of the European Paediatric Neurology Society. (2012) ;16: (2):149–60. |
[9] | McDonald CM , Gordish-Dressman H , Henricson EK , Duong T , Joyce NC , Jhawar S , et al. Longitudinal pulmonary function testing outcome measures in Duchenne muscular dystrophy: Long-term natural history with and without glucocorticoids. Neuromuscular Disorders. (2018) ;28: (11):897–909. |
[10] | Phillips MF , Quinlivan RCM , Edwards RHT , Calverley PMA . Changes in spirometry over time as a prognostic marker in patients with duchenne muscular dystrophy. American Journal of Respiratory and Critical Care Medicine. (2001) ;164: (12):2191–4. |
[11] | Guyatt GH , Oxman AD , Schünemann HJ , Tugwell P , Knottnerus A . GRADE guidelines: A new series of articles in the Journal of Clinical Epidemiology. J Clin Epidemiol. (2011) ;64: (4):380–2. |
[12] | Angliss ME , Sclip KD , Gauld L . Early NIV is associated with accelerated lung function decline in Duchenne muscular dystrophy treated with glucocorticosteroids. BMJ Open Respir Res. (2020) ;7: (1). |
[13] | Balaban B , Matthews DJ , Clayton GH , Carry T . Corticosteroid treatment and functional improvement in Duchenne muscular dystrophy long-term effect. American Journal of Physical Medicine and Rehabilitation. (2005) ;84: (11):843–50. |
[14] | Bello L , D’Angelo G , Villa M , Fusto A , Vianello S , Merlo B , et al. Genetic modifiers of respiratory function in Duchenne muscular dystrophy. Annals of Clinical and Translational Neurology. (2020) ;7: (5):786–98. |
[15] | Biggar WD , Gingras M , Fehlings DL , Harris VA , Steele CA . Deflazacort treatment of Duchenne muscular dystrophy. J Pediatr. (2001) ;138: (1):45–50. |
[16] | Biggar WD , Harris VA , Eliasoph L , Alman B . Long-term benefits of deflazacort treatment for boys with Duchenne muscular dystrophy in their second decade. Neuromuscular Disorders. (2006) ;16: (4):249–55. |
[17] | Buyse GM , Goemans N , van den Hauwe M , Thijs D , de Groot IJM , Schara U , et al. Idebenone as a novel, therapeutic approach for Duchenne muscular dystrophy: Results from a 12 month, double-blind, randomized placebo-controlled trial. Neuromuscular disorders: NMD. (2011) ;21: (6):396–405. |
[18] | Daftary AS , Crisanti M , Kalra M , Wong B , Amin R . Effect of long-term steroids on cough efficiency and respiratory muscle strength in patients with Duchenne muscular dystrophy. Pediatrics. (2007) ;119: (2):e320–4. |
[19] | Escolar DM , Hache LP , Clemens PR , Cnaan A , McDonald CM , Viswanathan V , et al. Randomized, blinded trial of weekend vs daily prednisone in Duchenne muscular dystrophy. Neurology. (2011) ;77: (5):444–52. |
[20] | Fayssoil A , Nguyen LS , Stojkovic T , Prigent H , Carlier R , Amthor H , et al. Determinants of diaphragm inspiratory motion, diaphragm thickening, and its performance for predicting respiratory restrictive pattern in Duchenne muscular dystrophy. Muscle and Nerve. (2022) ;65: (1):89–95. |
[21] | Henricson EK , Abresch RT , Cnaan A , Hu F , Duong T , Arrieta A , et al. The cooperative international neuromuscular research group Duchenne natural history study: Glucocorticoid treatment preserves clinically meaningful functional milestones and reduces rate of disease progression as measured by manual muscle testing and other commonly used clinical trial outcome measures. Muscle and Nerve. (2013) ;48: (1):55–67. |
[22] | Houde S , Filiatrault M , Fournier A , Dubé J , D’Arcy S , Bérubé D , et al. Deflazacort use in Duchenne muscular dystrophy: An 8-year follow-up. Pediatr Neurol. (2008) ;38: (3):200–6. |
[23] | Iff J , Gerrits C , Zhong Y , Tuttle E , Birk E , Zheng Y , et al. Delays in pulmonary decline in eteplirsen-treated patients with Duchenne muscular dystrophy. Muscle and Nerve. (2022) ;66: (3):262–9. |
[24] | Kelley EF , Cross TJ , McDonald CM , Hoffman EP , Bello L . Influence of beta2 adrenergic receptor genotype on longitudinal measures of forced vital capacity in patients with Duchenne muscular dystrophy. Neuromuscular Disorders. (2022) ;32: (2):150–8. |
[25] | Kelley EF , Cross TJ , Snyder EM , McDonald CM , Hoffman EP , Bello L . Influence of beta2 adrenergic receptor genotype on risk of nocturnal ventilation in patients with Duchenne muscular dystrophy. Respiratory Research. (2019) ;20: (1):221. |
[26] | Khan N , Eliopoulos H , Han L , Kinane TB , Lowes LP , Mendell JR , et al. Eteplirsen treatment attenuates respiratory decline in ambulatory and non-ambulatory patients with duchenne muscular dystrophy. Journal of Neuromuscular Diseases. (2019) ;6: (2):213–25. |
[27] | Kim S , Zhu Y , Romitti PA , Fox DJ , Sheehan DW , Valdez R , et al. Associations between timing of corticosteroid treatment initiation and clinical outcomes in Duchenne muscular dystrophy. Neuromuscular Disorders. (2017) ;27: (8):730–7. |
[28] | Koeks Z , Bladen CL , Salgado D , van Zwet E , Pogoryelova O , McMacken G , et al. Clinical Outcomes in Duchenne Muscular Dystrophy: A Study of 5345 Patients from the TREAT-NMD DMD Global Database. J Neuromuscul Dis. (2017) ;4: (4):293–306. |
[29] | Lee HN , Sawnani H , Horn PS , Rybalsky I , Relucio L , Wong BL . The Performance of the Upper Limb scores correlate with pulmonary function test measures and Egen Klassifikation scores in Duchenne muscular dystrophy. Neuromuscul Disord. (2016) ;26: (4-5):264–71. |
[30] | LoMauro A , Romei M , Gandossini S , Pascuzzo R , Vantini S , D’Angelo MG , et al. Evolution of respiratory function in Duchenne muscular dystrophy from childhood to adulthood. European Respiratory Journal. (2018) ;51: (2):1701418. |
[31] | Mayer OH , Leinonen M , Rummey C , Meier T , Buyse GM . Efficacy of Idebenone to Preserve Respiratory Function above Clinically Meaningful Thresholds for Forced Vital Capacity (FVC) in Patients with Duchenne Muscular Dystrophy. Journal of Neuromuscular Diseases. (2017) ;4: (3):189–98. |
[32] | McDonald CM , Muntoni F , Penematsa V , Jiang J , Kristensen A , Bibbiani F , et al. Ataluren delays loss of ambulation and respiratory decline in nonsense mutation Duchenne muscular dystrophy patients. Journal of Comparative Effectiveness Research. (2022) ;11: (3):139–55. |
[33] | Mitelman O , Abdel-Hamid HZ , Byrne BJ , Connolly AM , Heydemann P , Proud C , et al. A Combined Prospective and Retrospective Comparison of Long-Term Functional Outcomes Suggests Delayed Loss of Ambulation and Pulmonary Decline with Long-Term Eteplirsen Treatment. Journal of Neuromuscular Diseases. (2022) ;9: (1):39–52. |
[34] | Pradhan S , Ghosh D , Srivastava NK , Kumar A , Mittal B , Pandey CM , et al. Prednisolone in Duchenne muscular dystrophy with imminent loss of ambulation. J Neurol. (2006) ;253: (10):1309–16. |
[35] | Schara U , Mortier , Mortier W . Long-Term Steroid Therapy in Duchenne Muscular Dystrophy-Positive Results versus Side Effects. J Clin Neuromuscul Dis. (2001) ;2: (4):179–83. |
[36] | Silversides CK , Webb GD , Harris VA , Biggar DW . Effects of deflazacort on left ventricular function in patients with Duchenne muscular dystrophy. Am J Cardiol. (2003) ;91: (6):769–72. |
[37] | Trucco F , Ridout D , Domingos J , Maresh K , Chesshyre M , Munot P , et al. Genotype-related respiratory progression in Duchenne muscular dystrophy-A multicenter international study. Muscle and Nerve. (2022) ;65: (1):67–74. |
[38] | Tsabari R , Simchovitz E , Lavi E , Eliav O , Avrahami R , Ben-Sasson S , et al. Safety and clinical outcome of tamoxifen in Duchenne muscular dystrophy. Neuromuscular Disorders. (2021) ;31: (9):803–13. |
[39] | ClinicalTrials.gov. A Phase III Double-blind Study With Idebenone in Patients With Duchenne Muscular Dystrophy (DMD) Taking Glucocorticoid Steroids (SIDEROS). Available from: https://www.clinicaltrials.gov/ct2/show/NCT02814019 (accessed February 12, 2023). |
[40] | ClinicalTrials.gov. A Phase III Double-blind Study With Idebenone in Patients With Duchenne Muscular Dystrophy (DMD) Taking Glucocorticoid Steroids (SIDEROS). Available from: https://www.clinicaltrials.gov/ct2/show/NCT03603288 (accessed February 12, 2023). |
[41] | Birnkrant DJ , Bushby K , Bann CM , Apkon SD , Blackwell A , Brumbaugh D , et al. Diagnosis and management of Duchenne muscular dystrophy, part 1: Diagnosis, and neuromuscular, rehabilitation, endocrine, and gastrointestinal and nutritional management. Lancet Neurol. (2018) ;17: (3):251–67. |