Diagnostic Challenges of Neuromuscular Disorders after Whole Exome Sequencing
Abstract
Background:
Whole-exome sequencing (WES) facilitates the diagnosis of hereditary neuromuscular disorders. To achieve an accurate diagnosis, physicians should interpret the genetic report carefully along with clinical information and examinations. We described our experience with (1) clinical validation in patients with variants found using WES and (2) a diagnostic approach for those with negative findings from WES.
Methods:
WES was performed on patients with the clinical impression of hereditary neuromuscular disorders. Information on clinical manifestations, neurological examination, electrodiagnostic studies, histopathology of muscle and nerve, and laboratory tests were collected.
Results:
Forty-one patients (Male/Female: 18/23, age of onset: 34.5±15.9) accepted WES and were categorized into four scenarios: (1) patients with a positive WES result, (2) patients with an inconclusive WES result but supporting clinical data, (3) negative findings from WES, but a final diagnosis after further work-up, and (4) undetermined etiology from WES and in further work-ups. The yield rate of the initial WES was 63.4% (26/41). Among these, seventeen patients had positive WES result, while the other nine patients had inconclusive WES result but supporting clinical data. Notably, in the fifteen patients with negative findings from WES, four patients (26.7%) achieved a diagnosis after further workup: tumor-induced osteomalacia, metabolic myopathy with pathogenic variants in mitochondrial DNA, microsatellite expansion disease, and vasculitis-related neuropathy. The etiologies remained undetermined in eleven patients (myopathy: 7, neuropathy: 4) after WES and further workup.
Conclusions:
It is essential to design genotype-guided molecular studies to correlate the identified variants with their clinical features. For patients who had negative findings from WES, acquired diseases, mitochondrial DNA disorders and microsatellite expansion diseases should be considered.
INTRODUCTION
Neuromuscular disorders (NMDs) are a clinically and genetically heterogeneous group of disorders affecting peripheral nerves or muscles. Over the past decades, next-generation sequencing (NGS) has revolutionized the diagnostic paradigm in genetic neuromuscular disorders, and whole-exome sequencing (WES) is one of these powerful diagnostic tools. WES investigates protein-coding genes, approximately 3% of the whole genome, and the protein-coding genes accounts for up to 60% of disease-causing genomic variation [1]. The comprehensive interpretation of clinical manifestations, pathology, electrophysiological studies, and WES reports leads to accurate diagnosis of NMDs.
Previous studies have shown that the early utilization of WES facilitates more rapid and accurate NMD diagnosis, especially in monogenetic diseases [2–4]. The overall diagnosis yield rate ranges from 40–60% in myopathic patients and 30–40% in neuropathy patients, with a higher yield rate in pediatric patients and a lower yield rate in single proband studies [5–10]. The negative-exome results may be caused by somatic mosaicism, copy number variants, mitochondrial mutations, structural variants not properly captured through exome sequencing, and secondary causes not identified [11].
For exome-positive cases with known pathogenic variants that had been well investigated, the final diagnosis can be reached using phenotype-genotype correlations. However, careful interpretation along with further evaluation are essential for exome-positive cases without previously reported pathogenic variants and exome-negative cases [12]. Herein, we described our experience in integrating WES into a diagnostic approach in patients with NMDs. We focus on (1) advanced molecular tests in patients with variants found using WES and (2) a diagnostic approach for those with negative findings from WES.
METHODS
Patient recruitment
This retrospective cohort study (202109079RIBN) was approved by the Ethics Committee of the National Taiwan University Hospital (NTUH), Taipei, Taiwan. All procedures performed in studies involving human participants were in accordance with the ethical standards of the institutional and/or national research committee and with the 1964 Helsinki Declaration and its later amendments or comparable ethical standards. The inclusion criteria were: (1) an impression of hereditary NMDs, (2) no other etiology identified after selective genetic testing before the WES study, and (3) clinical data were available. We arranged WES to all the probands, and for the proband’s family, Sanger sequencing on the specific variants revealed by the proband-only WES was arranged if they agreed.
Clinical evaluations, laboratory study, and electrophysiological examinations
The clinical data, including age of disease onset, sex, and pedigree, were collected in all patients. In patients with myopathy, we additionally collected the serum creatinine kinase (CK) levels and the informations of muscle histopathology. According to previous studies, distribution of weakness and associated symptoms can be categorized into different patterns for clinical diagnosis. The presentation of our patients was categorized as follows: (1) limb-girdle weakness, (2) scapuloperoneal weakness, (3) distal arm and proximal leg weakness, (4) distal weakness, (5) ptosis with or without ophthalmoplegia, (6) episodic weakness/myalgia/rhabdomyolysis, and (7) asymptomatic hyperCKemia. The muscle histopathology was evaluated by the neurologist and pathologist together. Routine histochemical staining in our hospital encompassed hematoxylin and eosin staining, Gomori-Trichome staining, succinate dehydrogenase staining, nicotinamide adenine dinucleotide staining, and staining for myosin adenosine triphosphatase at pH 4.3 and 10.42. Based on clinical information and routine histochemistry results, specific immunohistochemistry would be arranged. Electron microscopy studies of muscle biopsy were also performed on some samples.
In patients with polyneuropathy, we collected clinical data and performed nerve conduction study (NCS). We used the electrophysiological criteria for chronic inflammatory demyelinating polyradiculoneuropathy to define demyelination and axonal loss.
Whole-exome sequencing
WES was performed using an exome capture kit probe (Agilent V6 (before Jan 2021) and Roche Hyperexome (after Feb 2021)). We then did sequencing with a NovaSeq6000 sequencer (Illumina). The protein-coding genes and flanking intronic sequences (50 bp) were studied with a 300-bp paired-end run and an average of > 150-fold coverage.
The human reference genome is GRCH38 and we used the Burrows–Wheeler Aligner (BWA) for sequence alignment and used the Genome Analysis Tool Kit (GATK V3.5, Broad Institute) [13] for variant calling. Variants were first annotated using ANNOVAR (http://wannovar.wglab.org/) [14]. Then, the annotated profiles were tagged with information of the inheritance pattern from OMIM (https://omim.org/), variant pathogenicity from ClinVar (https://www.ncbi.nlm.nih.gov/clinvar/), and the allele frequency from the Taiwan Biobank (https://taiwanview.twbiobank.org.tw/). The variant filtering criteria incorporated maximal minor allele frequency (included if < 0.01) and in-silico analysis of variant severity (nonsynonymous variant, nonsense, deletion, splicing, insertion, or splicing). We manually calssified the pathogenicity of variants according to American College of Medical Genetics and Genomics (ACMG) and Association for Molecular Pathology guidelines [15–19]. We further defined the results of WES as “positive”, “inconclusive” and “negative” based on ACMG criteria. The positive results were defined as the variants that had been asserted as pathogenic or likely pathogenic in ACMG criteria and definite genetic diagnosis according to the inheritance pattern of gene. That is, for those compound heterozygous variants, only both alleles showed pathogenic or likely pathogenic in ACMG criteria were defined as “positive”. The inconclusive results were those being classified as variants of uncertain significance in ACMG criteria. The results of the WES were defined as negative if (1) no possible culprit variant identified in the WES or (2) the identified variants were excluded by the segregation analysis. For copy number analysis (CNV), with SAM/BAM file as input, the GATK germline CNV (gCNV) caller can generate a VCF file containing the CNVs found during the variant calling process [20].
Clinical validation of WES results: Clinical-Molecular diagnosis
In patients with myopathy, the pathogenicity was defined as “definite” in two situations: (1) patients presented with the representative clinical phenotype with positive WES results, or (2) those who had a typical phenotype but with inconclusive WES results, the diagnosis validated by typical pathognomonic findings in clinical manifestations, pathology, or other examinations. Notably, there should be negative results for the other known genes with the similar phenotype in both situations [21]. Instead, pathogenicity was defined as “probable” if the criteria were not met. Among the patients with neuropathy or motor neuron disease, the clinical-molecular diagnosis was regarded “definite” only if the clinical manifestations, culprit variants, and electrophysiological study were consistent with the literature. Instead, pathogenicity was defined as “probable” if the above criteria were not met. For those with two allelic variants, the definite diagnosis required that compound heterozygous variants were confirmed. The final clinical-molecular diagnosis was made based on the genotype-phenotype correlation and was referred to Online Mendelian Inheritance in Man (OMIM) database.
RESULTS
In total, we recruited 41 patients with neuromuscular disorders who accepted the WES study. Most of the patients (n = 28) had a clinical diagnosis of myopathy, while the other 10 patients were clinically diagnosed with neuropathy, and 3 patients were diagnosed with motor neuronopathy. The clinical phenotypes, laboratory findings including electrophysiological and pathology, and genetic findings are summarized in Tables 1, 2, 3, & 4, Fig. 1, and supplementary. Overall, twenty-six patients (63.4%) had a likely diagnosis from the WES, meeting the clinical-molecular diagnosis [21], most of whom were diagnosed with myopathy, except four patients who were diagnosed with neuropathy or neuronopathy. Eight cases had biallelic variants, and compound heterozygosity was confirmed in four cases. Five patients could not meet the “definite” clinical-molecular diagnosis due to lack of segregation analysis. There were four scenarios among these 41 patients:
Fig. 1
Algorithm of the study.
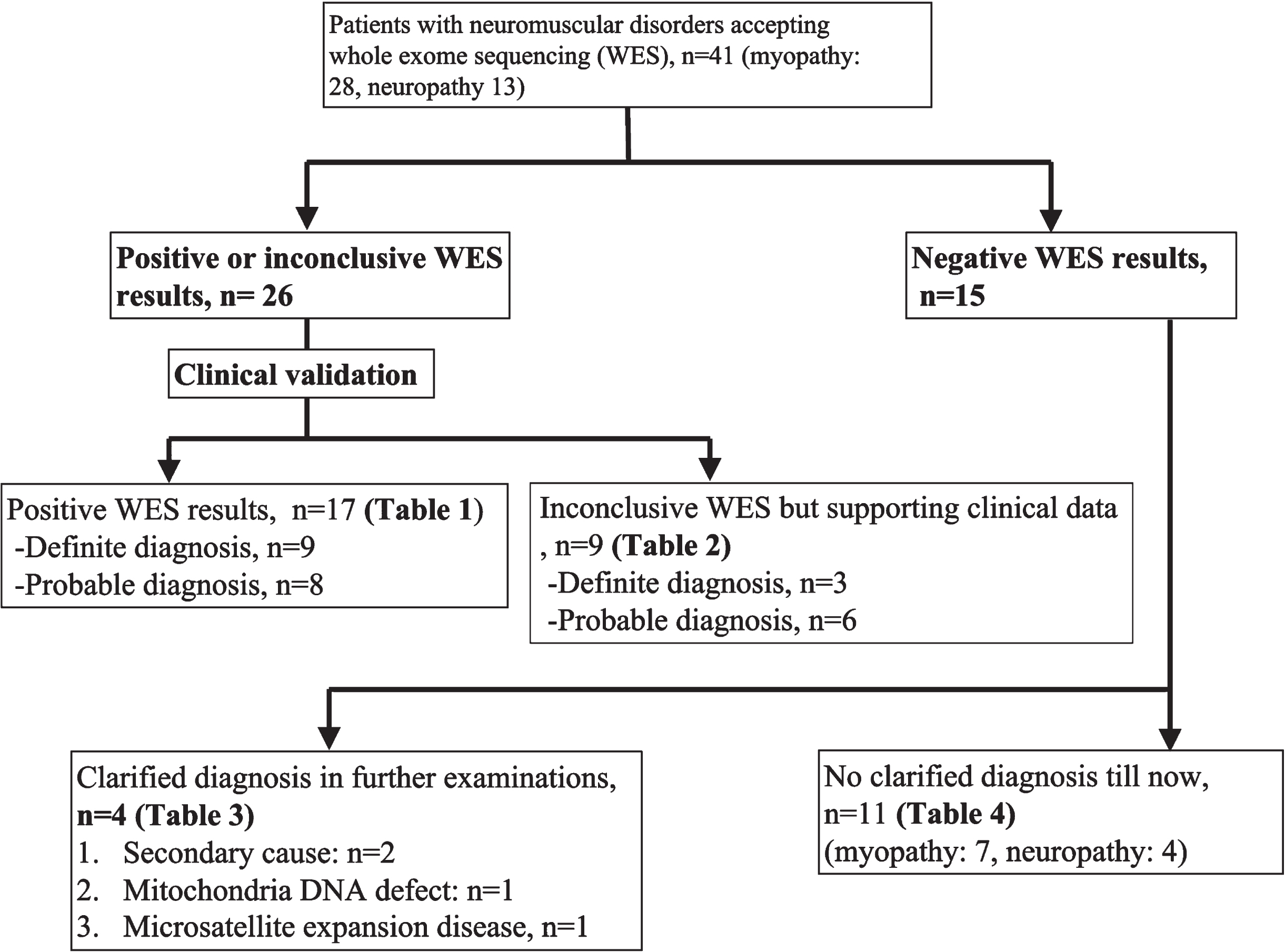
Table 1
Patients with a positive whole-exome sequencing result
Case /Age of onset/sex | Type of weakness | CK (U/L) | Pathology | Genetic diagnosis | Final diagnosis | Key points for definite/probable diagnosis | |||
Gene | Variants | Zygosity | ACMG class. | ||||||
Myopathy-definite diagnosis | |||||||||
1/23/F | Distal weakness | 171 | Myopathy change with rimmed vacuoles | GNE | c.[1618C>T];[829C>T] p.[His540Tyr];[Arg277Trp] | Het/Het | LP/P | GNE myopathy | Clinical manifestations and muscle biopsy |
2/40/F | Episodic pain and weakness | 63 | Lipid myopathy | ETFDH | c.[250G>A];[250G>A] p.[Ala84Thr];[Ala84Thr] | Hom | P | Multiple acyl-CoA dehydrogenase deficiency | Clinical manifestations, laboratory findings (tandem mass) and muscle biopsy |
3/11/M | Episodic pain and weakness | 192 | - | ETFDH | c.[250G>A]; [250G>A] p.[Ala84Thr]; [Ala84Thr] | Hom | P | Multiple acyl-CoA dehydrogenase deficiency | Clinical manifestations and laboratory findings (tandem mass) |
4/25/F | Distal Arm/Proximal Leg weakness | 253 | Nonspecific myopathy change | TCAP | c.23_24insCGAGGTGT p.Glu12_ArgfsTer20 | Hom | P | TCAP-related distal myopathy | Clinical manifestations and muscle biopsy |
5/18/M | Limb-girdle weakness | 655 | Myopathy change with rimmed vacuoles | TCAP | c.26_33dup p.Glu12_Argfs*20 | Hom | P | LGMD R7 | Clinical manifestations and muscle biopsy |
6/Child-hood/M | Episodic pain related to exercise | 3282 | Myogenic myopathy with partial loss of dystrophin staining | DMD | g.(?–31929601)_(32035615-?)del Exon 45–47 deletion | Het | P | Dystrophinopathy | Muscle biopsy. |
7/14/F | Distal weakness | 786 | Myopathy change with rimmed vacuoles | GNE | c.[1399C>T];[815T>G] p.[Gln467X];[Ile272Ser] | Het/Het | P/LP | GNE myopathy | Clinical manifestations and muscle biopsy |
Myopathy-probable diagnosis | |||||||||
8/Child-hood/M | Distal weakness | 105 | Chronic atrophic myocytes with predominant type I fibers | CLCN1 | c.[178C>T];[1444G>A] p.[Gln60Ter];[Gly482Arg] | Het/Het | P/LP | Myotonia congenita | Pathognomonic clinical manifestations, electrophysiology studies and muscle biopsy |
9/15/M | Episodic pain and weakness | 448 | Predominant type II fibers | CAPN3 | c.[1621C>T];[1621 =] p.[Arg541Trp];[Arg541 =] | Het | LP | LGMD D4 | Atypical clinical manifestations, need segregation analysis |
10/40/M | Limb-girdle weakness | 728 | - | TTN | c.[95136T>G];[95136 =] p.[Cys31712Trp];[Cys31712 =] | Het | LP | Muscular dystrophy | Uncommon phenotypes of TTN myopathy, need segregation analysis |
11/40/F | Limb-girdle weakness | 1586 | Dystrophic myopathy | DYSF | c.1277G>T(;)1667T>C p.Gly426Val(;)Leu556Pro | Het/Het | P/LP | LGMD R2 | Need segregation analysis to ascertain compound heterozygotes. |
12/47/F | Ptosis with ophthalmoplegia | 426 | Myopathy with combined neurogenic and myopathic features. Rimmed vacuoles, but no ragged-red fiber | SCN4A | c.[2297T>C];[2297T=] p.[Met766Thr];[Met766 =] | Het | LP | SCN4A-related myopathy (Single fiber EMG: normal jitter) | Atypical clinical manifestations, need segregation analysis and molecular studies. |
13/48/F | Scapulo-peroneal | 303 | Myogenic myopathy | FHL1 | c.[394T>C];[394 =] p.[Cys132Arg];[Cys132 =] | Het | LP | X-linked dominant scapuloperoneal myopathy | Unreported variant, need segregation analysis and molecular studies. |
Neuropathy-definite diagnosis | |||||||||
Case/Age of onset/sex | Clinical manifestations | Electrophysiology studies | Genetic diagnosis | Final diagnosis | Key points for definite/probable diagnosis | ||||
Gene | Variants | Zygosity | ACMG class. | ||||||
14/14/F | Distal numbness and weakness | Sensorimotor polyneuropathy with multiple conduction block | PMP22 | g.(?–15230907)_(15569020_?)del Total deletion of PMP22 gene | Het | P | HNPP | Clinical manifestations and nerve conduction studies | |
Neuropathy-probable diagnosis | |||||||||
15/50/M | Distal weakness | Motor neuropathy/motor neuronopathy | FBXO38 | c.[1389G>A];[1389G =] p.[Met463Ile];[Met463 =] | Het | LP | Probable neuronopathy, distal hereditary motor, type IID | Genetic susceptibility to neuronopathy; typical electrophysiology studies, need segregation analysis and molecular studies. | |
16/38/M | Distal numbness, parkinsonism | Sensory-predominant polyneuropathy or sensory neuronopathy | POLG | c.[3535T>C];[3535T =] p.[Phe1179Leu];[Phe1179 =] | Het | LP | POLG related parkinsonism with sensory neuronopathy | Gene related to parkinsonism and sensory neuronopathy, neuronopathy; typical electrophysiology studies, need segregation analysis and molecular studies. | |
17/46/F | Limb-girdle weakness | Motor-predominant polyneuropathy with minimal sensory findings | OPA1 | c.2600–2601del p.Val867Glufs* | Het | P | Probable OPA1-related motor dominant neuropathy | Gene related to motor-predominant polyneuropathy; neuronopathy; typical electrophysiology studies, need segregation analysis and molecular studies. |
Table 2
Patients with an inconclusive whole-exome sequencing result but supporting clinical data
Case /Age of onset/sex | Type of weakness | CK (U/L) | Pathology | Genetic diagnosis | Final diagnosis | Key points for definite/probable diagnosis | |||
Gene | Variants | Zygosity | ACMG class. | ||||||
Myopathy-definite diagnosis | |||||||||
18/43/F | Distal weakness | 704 | Myofibrillar myopathy | DES | c.1189_1206del | Het | VUS | Myofibrillar Myopathy 1 | Clinical manifestations and muscle biopsy |
p.397_402del | |||||||||
19/44/F | Limb-girdle weakness | 2701 | Myogenic myopathy with partial loss of dystrophin staining | DMD | g.(?_32287478)_(32699344-?)dup | Het | VUS | Dystrophinopathy | Muscle biopsy |
Exon 8∼43 duplication | |||||||||
20/37/F | Limb-girdle weakness | 201 | Myopathy change with some ragged red fibers | TK2 | c.[367C>G];[157– 2A>C] | Het/Het | VUS/LP | TK2-related myopathy | Muscle biopsy, mitochondria DNA sequencing and muscle electron transfer chain activity assay |
p.[R123G];[splice site] | |||||||||
Myopathy-probable diagnosis | |||||||||
21/13/M | Proximal leg weakness | 43 | Mild atrophic myocytes | SLCO2A1 | c.T1069C(;) G1106A | Het/Het | VUS/LP | Hypertrophic osteoarthropathy | Pathognomonic clinical manifestations, need segregation analysis to ascertain compound heterozygotes. |
p.Tyr357His(;)Gly369Asp | |||||||||
22/40/F | Limb-girdle weakness | 564 | Myogenic myopathy, subsarcolemmal accumulation, abnormal Z-lines and myofibrils | TTN | c.[1800 + 1G>A];[1800 + 1 =] | Het | VUS | Hereditary myopathy with early respiratory failure (HMERF) | Unreported variant in the region not typically associated to HMERF. |
23/20/F | Ptosis with ophthalmoplegia | 195 | - | POLG | c.2653A>C(;)924G>C | Het/Het | VUS/LP | CPEO | Need segregation analysis to ascertain compound heterozygotes. |
p.Thr885Pro(;)Gln308His | |||||||||
Neuropathy-probable diagnosis | |||||||||
Case/Age of onset/sex | Type of weakness | Electrophysiology studies | Genetic diagnosis | Final diagnosis | Key points for probable diagnosis | ||||
Gene | Variants | Zygosity | ACMG class. | ||||||
24/17/F | Isolated upper limbs weakness | Motor neuropathy/motor neuronopathy | NEFH | c.[G559T];[G559 =] | Het | VUS | Brachial amyotrophic diplegia | Genetic susceptibility to neuronopathy; typical electrophysiology studies; molecular research should be done | |
p.[Asp187Tyr];[Asp187 =] | |||||||||
25/47/F | Distal numbness and weakness | Grossly normal | NEFH | c.2230_2247del | Het | VUS | Probable PLS | Genetic susceptibility to neuronopathy; molecular research should be done | |
p.744_749del | |||||||||
26/30/F | Ptosis, hands numbness and gait disturbance | Motor-predominant polyneuropathy | SCO2 | c.544C>T(;)358C>T | Het/Het | P/VUS | CMT4 | Gene related to CMT4; neuronopathy; typical electrophysiology studies; need segregation analysis and molecular studies. | |
p.Gln182Ter(;)Arg120Trp |
Table 3
Patients with negative WES result, but with final clinical diagnosis after further work-up
Age of onset | Clinical symptoms | CK (U/L) | Pathology | Clinical diagnosis | Genetic diagnosis | Final diagnosis | |||
Gene | Variants | Zygosity | ACMG class. | ||||||
Myopathy-definite diagnosis | |||||||||
27/29/M | Limb-girdle weakness | 51 | - Muscle pathology: nonspecific findings | Chronic myopathy, hypophosphastemia | Not found | - | - | - | Tumor-induced osteomalacia |
- Tumor pathology: phosphaturic mesenchymal tumor | |||||||||
28/40/F | Episodic weakness related to exercise | 248 | Mitochondrial or lipid myopathy | Metabolic myopathy | MT-TL1 | m.3243A>G | Heteroplasmy level: 33.41% | P | Mitochondrial myopathy |
Neuropathy-definite diagnosis | |||||||||
Case/Age of onset/sex | Type of weakness | Electrophysiology studies | Clinical diagnosis | Genetic diagnosis | Final diagnosis | ||||
Gene | Variants | Zygosity | ACMG class. | ||||||
29/61/M | Sensory dominant polyneuropathy with nystagmus | Sensory-predominant polyneuropathy with axonal degeneration features | Hereditary sensory autonomic neuropathy | ATXN3 | CAG repeat numbers: 28/63* (Pathogenic≧52) | Het | P | ATXN3-related sensory neuropathy and cerebellar ataxia | |
Neuropathy-probable diagnosis | |||||||||
Case/Age of onset/sex | Type of weakness | Electrophysiology studies | Clinical diagnosis | Genetic diagnosis | Final diagnosis | ||||
Gene | Variants | Zygosity | ACMG class. | ||||||
30/27/F | Episodic numbness and weakness | Sensorimotor polyneuropathy with axonal degeneration features and asymmetric distribution | Suspect mononeuropathy multiplex, or HNPP | Not found | - | - | - | Mononeuropathy multiplex, suspect vasculitis related |
Scenario 1: Patients with a positive WES result
Among these 17 patients with positive WES result (Table 1), most presented with a typical clinical phenotype. We made the diagnosis with genotype-phenotype correlation. In the group of definite diagnosis, two cases with atypical presentations (Cases 6 and 7). Case 6 was a male with exercise intolerance and high hyperCKemia. The work-up of metabolic myopathy and mitochondrial myopathy was normal. However, the patient had partial loss of dystrophin staining in muscle biopsy, supporting the diagnosis of dystrophinopathy. Case 7 had hand weakness as an initial symptom but later developed lower limb distal weakness, which is rarely reported in GNE myopathy.
There were 8 patients classified as members of the probable diagnosis group (myopathy: 5, neuropathy: 3). Three patients (CAPN3, TTN, and SCN4A) with atypical or uncommon clinical phenotypes could not meet the definite diagnosis criteria, and segregation analysis and molecular studies were needed. Four patients (FHL1, FBXO38, POLG, and OPA1) had variants that had been asserted as pathogenic or likely pathogenic in ClinVar database, and one patient (DYSF) need a segregation analysis to ascertain compound heterozygote.
Scenario 2: Patients with an inconclusive WES result but supporting clinical data
There were 9 patients showing inconclusive WES result but having supporting clinical data. Of note, 3 patients with myopathy who carried the new variants fulfilled the proposed definite diagnosis criteria. Their diagnosis was validated by (1) typical pathognomonic findings yielded from the clinical manifestations or muscle biopsy (e.g., distal myopathy and atrophic myocytes with desmin aggregates in DES-related myofibrillar myopathy 1; limb-girdle weakness with partial loss of dystrophin staining in muscle biopsy), or (2) further molecular examinations (e.g., mitochondria DNA sequencing and muscle electron transfer chain activity assay in TK2-related myopathy [22]).
There were another 6 patients classified in the probable diagnosis group (myopathy: 3, neuropathy: 3). Three patients (SLCO2A1, POLG, and SCO2) with representative clinical phenotypes could not meet the definite diagnosis criteria due to the lack of segregation analysis to ascertain compound heterozygote. Notably, in a patient with SLCO2A1-related hypertrophic osteoarthropathy we found a nonspecific myopathic change in the proximal muscles, which has not been previously reported. The patient with variant in TTN gene had early respiratory failure when she could still ambulate. However, we classified the case as a probable diagnosis because the variant was not located in the common region related to hereditary myopathy with early respiratory failure (HMERF). Although the patient had no necklace body in the muscle pathology, the abnormal desmin and dystrophin subsacrolemmal in light microscopy and Z-disk alterations in electron microscopy are compatible with the pathological diagnosis of myofibrillar myopathy, which has been reported in TTN-related HMERF [23, 24]. Two patients had variants in the NEFH gene. We made the diagnosis of brachial amyotrophic diplegia, primary lateral sclerosis, neuronopathy, distal hereditary motor neuropathy, and type IID based on the clinical presentation, electrophysiological studies, and WES results.
Scenario 3: Patients with negative WES result, but with final clinical diagnosis after further work-up
Notably, in the 15 patients without a molecular diagnosis from WES, four patients (26.7 %) had a diagnosis after further work-up. The details of these four patients are described below.
Case 27: Tumor-induced osteomalacia: A 32-year-old man presented with a 3-year history of progressive proximal muscle weakness, atrophy, and generalized back soreness. Electromyography performed at other hospitals showed myopathic changes with spontaneous activities and a right proximal tibial tumor. WES was performed but only found some benign variants. The clinical evaluation revealed a reduction in body height from 185 cm to 183 cm, kyphosis, and a palpable tender lump at the right proximal lateral tibia. Laboratory tests showed markedly reduced phosphate (1.3 mg/dL; normal range 2.5–5 mg/dL) and normal creatine kinase levels. The workup for the hypophosphatemia revealed normal calcium and iPTH, decreased 25-OH vitamin D (16 ng/mL; normal range 25–80 mg/mL), and elevated alkaline phosphatase (439 U/L; normal range 34–104 U/L). The urine analysis showed a markedly elevated urinary phosphate level (5332 mg/24 hrs). Furthermore, the bone scan showed a bone tumor with markedly increased osteoblastic activity at the right proximal femur. Combined with the above information, tumor-induced osteomalacia was highly suspected. Elevated serum fibroblast growth factor 23 (FGF23) confirmed the diagnosis. The pathology of the bone tumor was consistent with a phosphaturic mesenchymal tumor. The patient received tumor excision, his FGF23 was undetectable five hours after the operation, and his serum phosphate returned to normal three days after the surgery (Fig. 2). On follow-up, his bone pain and whole back soreness resolved. He could walk with a cane for more than 100 meters three months later and could stand without assistance for more than 10 minutes at the 5-month follow-up. (Supplementary Video)
Fig. 2
Muscle and bone histopathology in patient 28 with tumor-induced osteomalacia. The staining of hematoxylin and eosin (H&E) (A) and myosin adenosine triphosphatase at pH 4.3 (B) showed compacted muscle fibers with scattered atrophic fibers in the biopsy at left biceps brachii. The bone tumor pathology showed spindle tumor cells in myxohyalinized fibrotic stroma with eosinophilic matrix-like materials accompanying the bony components in H&E staining. (C&D) Focal bony destruction, woven bone formation and grungy calcification were also noted. The immunohistochemical staining of CD56 (E) and SATB2 (F) was strongly positive. Whole-body bone scintigraphy revealed a bone tumor with markedly increased osteoblastic activity at the right proximal femur. (G) Additionally, there were multiple posttraumatic changes in the thoracic cage, pubic bones, and bilateral femurs.
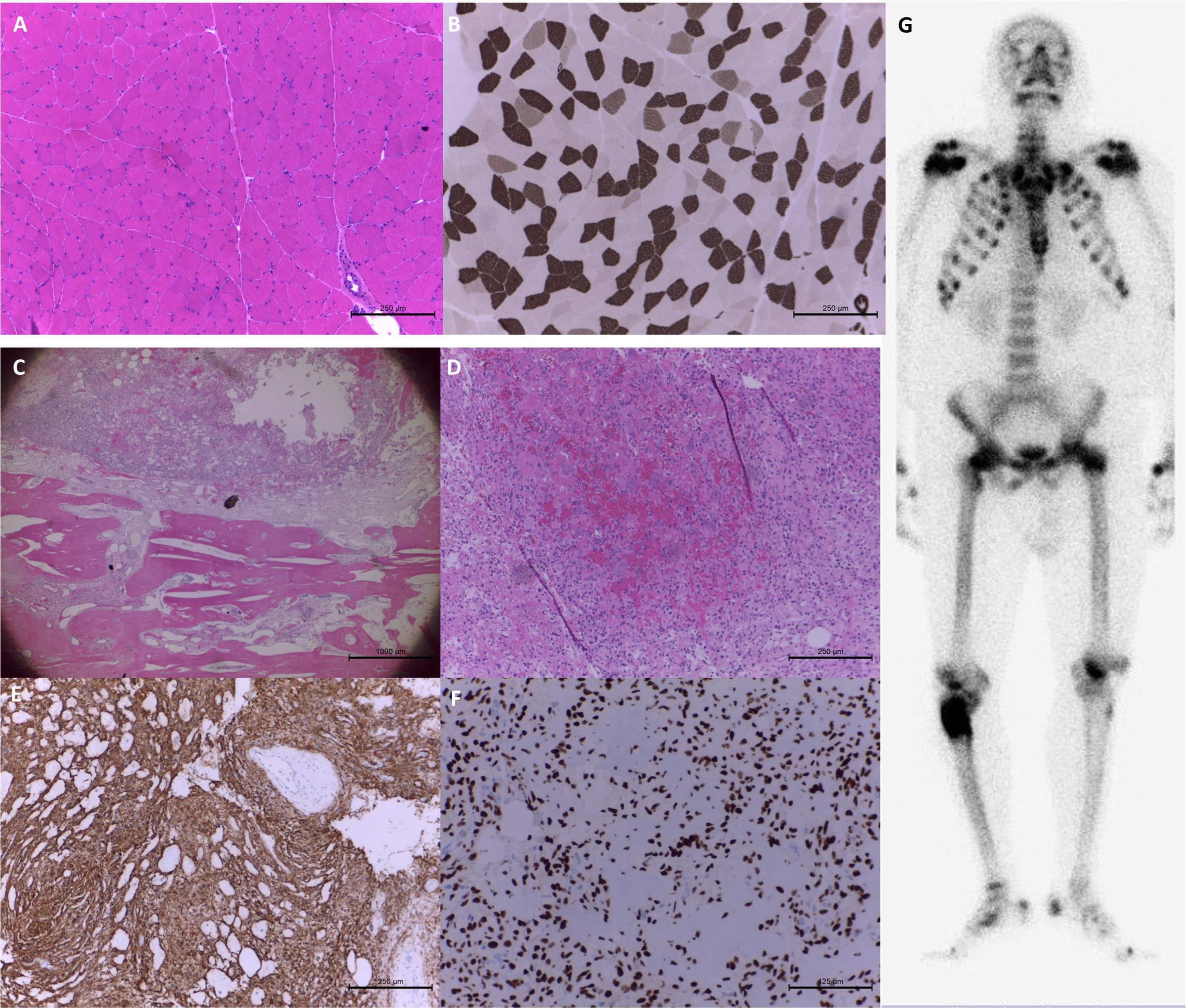
Case 28: Mitochondrial myopathy: A 41-year-old woman with no systemic disease visited our hospital for a one-month history of progressive proximal muscle weakness along with generalized myalgia. In addition, she lost eight kg in two months. She first received steroid therapy and her muscle strength improved in the first three months but later worsened even under treatment with prednisolone and azathioprine. Moreover, she developed obvious exercise intolerance. Her lactate level was 6.77 mmol/L at baseline (normal range 0.5–2.2 mmol/L). and increased to 11.96 mmol/L after exercise. Large amounts of lactate and pyruvate were noted in the urine as well as the presence of TCA cycle intermediates. The histopathology of the left biceps brachii revealed excessive lipid accumulation. (Fig. 3) Given the impression of metabolic myopathy, WES was ordered but yielded only benign variants. Based on the clinical information, we further arranged mitochondrial DNA (mtDNA) next-generation sequencing and a pathogenic variant (m.3243A>G) was found, confirming the diagnosis of mitochondrial myopathy.
Fig. 3
Muscle histopathology in patient 29 with mitochondrial myopathy. Hematoxylin and eosin staining showed variated muscle fiber sizes, degenerative and atrophic muscle fiber sizes, and nuclear internalization in the biopsy at the left biceps brachii. There were muscle fibers with variated cytoplasmic vacuoles. The vacuole was strongly positive for Oil Red O staining (B). In the staining of nicotinamide adenine dinucleotide-tetrazolium reductase (NADH-TR) (C) and succinate dehydrogenase (D), dark staining was noted in some muscle fibers (arrowhead). Vacuoles were also seen in the NADH-TR staining ( *).
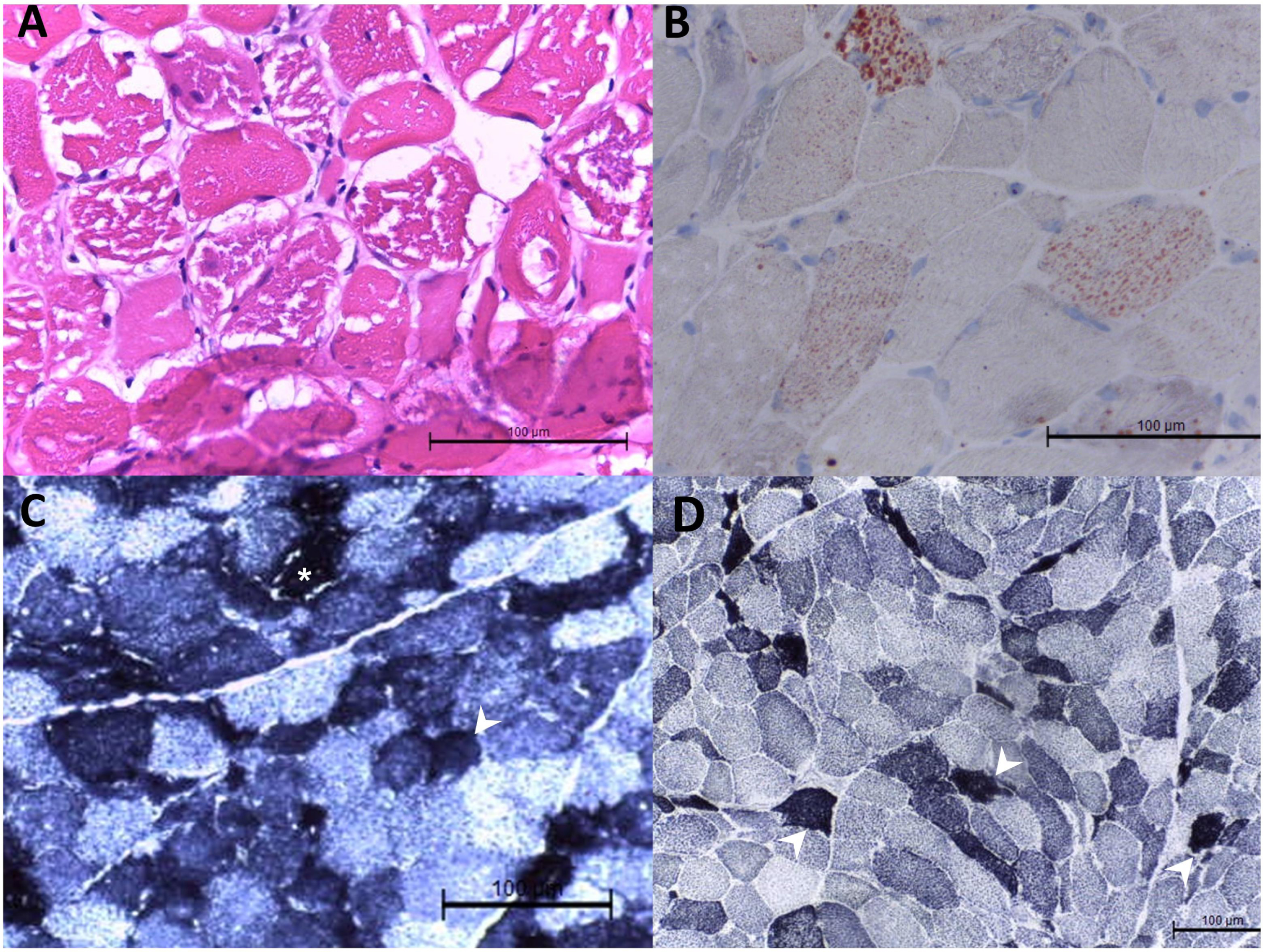
Case 29: Microsatellite expansion disease: A 65-year-old man with congenital deafness developed unsteady gait at the age of 61. Bilateral upper limb clumsiness, dysmetria, and dysphagia appeared successively from the age of 63. With disease progression, he became unable to walk without assistance and was brought to our hospital for help. He reported a family history of gait problems in his mother and siblings. The neurological examination showed saccadic pursuit, dysphagia, decreased muscle strength in all four limbs, areflexia, positive Romberg test, and ataxic gait. The brain and spine MRI findings were normal. NCS revealed sensorimotor polyneuropathy. With multisystem involvement, WES was arranged but showed negative results. Notably, a heterozygous CAG repeat expansion (repeat number: 28 and 63) was noted in the ATX3 gene, and compatible with Machado-Joseph Disease.
Case 30: Mononeuropathy multiplex, vasculitis related: The 37-year-old woman presented with several episodes of mononeuropathy in all four limbs with or without complete recovery from the age of 17. The NCS disclosed absent or decreased CMAP in the bilateral median, ulnar, tibial, and right peroneal nerves. Additionally, there was absent or reduced sensory nerve action potential (SNAP) in the bilateral median, ulnar, sural, superficial peroneal, and left superficial radial nerves. The laboratory tests and CSF studies were within normal limits except for elevated rheumatoid factor. The combined biopsy at the right superficial peroneal nerve and right peroneal brevis muscle did not reveal evidence of vasculitis (Fig. 4). Under the impression of mononeuropathy multiplex with unknown cause, whole genome sequencing was arranged, yielding negative findings. Nevertheless, the patient-reported symptoms improved after receiving pentoxifylline and a short duration of oral steroids. Based on the clinical findings, the final diagnosis was vasculitis-related mononeuropathy multiplex.
Fig. 4
Combined muscle and nerve biopsy in patient 31. The staining of hematoxylin and eosin (H&E) (A) and nicotinamide adenine dinucleotide-tetrazolium reductase (B) showed grouped atrophy in the right peroneal brevis muscle. The biopsy in the right superficial peroneal nerve did not show thickening of small vessels, lymphocyte infiltration, red blood cell extravasation in H&E (C-E) and Masson’s trichrome staining (F).
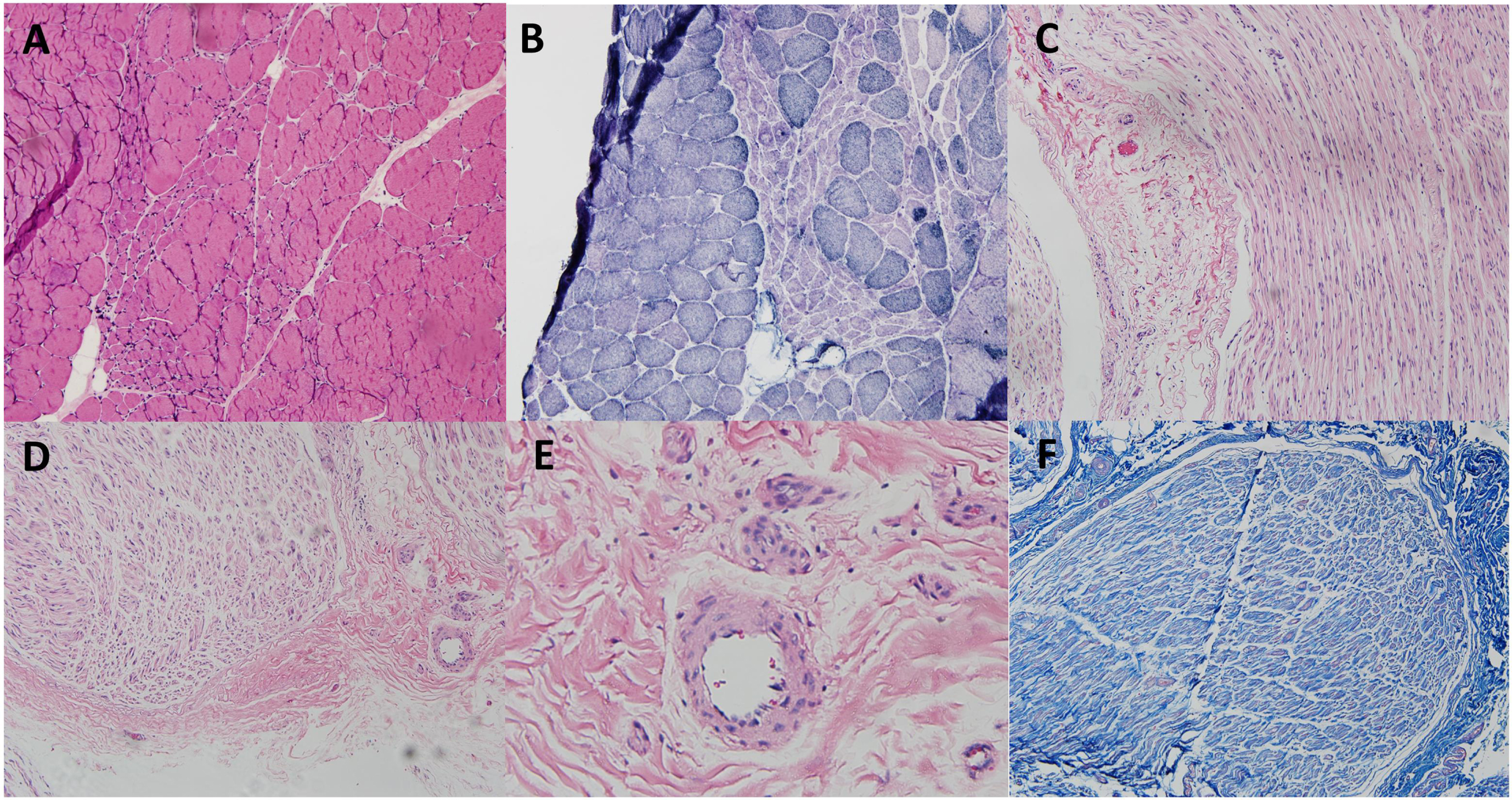
Scenario 4: Patients with negative WES result and undetermined etiology in further work-ups
For the remaining 11 patients with a negative molecular diagnosis from WES, most (n = 7) had a clinical diagnosis of myopathy, while others (n = 4) were clinically diagnosed with neuropathy. The detailed clinical features of patients without definite diagnosis are presented in Table 4. Among these, some had negative findings from NGS. Two had suspected variants at first but were later excluded by segregation analysis. WGS may be helpful for these patients. For patients with unreported pathological variants of ANO5 and FKRP mutations, the definite diagnosis may be verified after segregation analysis and muscle biopsy with molecular studies. Currently, the patient is still hesitant about this plan. Some had suspected variants but without reported phenotypes, such as POLG-related Charcot-Marie-Tooth disease type 1 (CMT1) and ACAD9-related mitochondrial myopathy. Segregation analysis and further molecular diagnosis are needed.
Table 4
Patients with negative WES result and undetermined etiology in further work-ups
Age of onset | Type of weakness | CK (U/L) | Pathology | Clinical diagnosis | Genetic diagnosis | Further workup | |||
Gene | Variants | Zygosity | ACMG class. | ||||||
Myopathy | |||||||||
31/10/M | limb-girdle weakness | 352 | - | Suspect mitochondrial disease | Not found | - | - | - | Blood mtDNA NGS: no culprit variants found; Consider muscle mtDNA sequencing, long-read sequencing, RNAseq or WGS |
32/33/F | 3 episodes of rhabdomyolysis | 304 | Variation of fiber size; most atrophic and angular fibers are type II, mild endomysial fibrosis | Suspect mitochondrial disease | Not found | - | - | - | Blood mtDNA NGS: no culprit variants found; Consider muscle mtDNA sequencing or WGS |
33/55/F | Proximal Arm (Scapular winging) | 43 | Myogenic myopathy, rare ragged-red fibers | mitochondrial myopathy | ACAD9 | c.[797G>A];[797G=] p.[Arg266Gln];[Arg266 =] | Het | LP | Blood mtDNA NGS: no culprit variants found; Consider muscle electron transfer chain assay/muscle mtDNA sequencing |
34/26/M | Limb-girdle weakness | 145 | - | Suspect hereditary hypophosphatemic rickets | Not found | Reanalysis WES 1-2 years later or check long-read sequencing, RNAseq, or WGS | |||
35/19/M | Limb-girdle weakness | 437 | Congenital myopathy with myofibrillar abnormalities | Suspect muscular dystrophy, mitochondrial or other congenital myopathy | Not found | - | - | - | Reanalysis WES 1-2 years later or check long-read sequencing, RNAseq, or WGS |
36/25/F | Limb-girdle weakness | 52 | Dystrophic myopathy | Suspect muscular dystrophy | Not found | - | - | - | Reanalysis WES 1-2 years later or check long-read sequencing, RNAseq, or WGS |
37/52/M | No definite weakness, subjectively felt smaller size in right leg | fluctuate between 300 U/L to 1000 U/L/ | - | Asymptomatic hyperCKemia | ANO5 | c.191dupA p.Asn64LysfsTer15 | Het | P | Segregation analysis and muscle biopsy was suggested, but patient hesitated. |
FKRP | c.[826C>A]; [826 =] p.[Leu276Ile]; [Leu276 =] | Het | P | ||||||
Neuropathy | |||||||||
Age of onset | Initial symptoms | Electrophysiology studies | Clinical diagnosis | Genetic diagnosis | Further workup | ||||
Gene | Variants | Zygosity | ACMG class. | ||||||
38/42/F | Distal numbness and weakness | Sensorimotor polyneuropathy with demyelinating features | CMT1 | POLG | c.[2890C>T],[2890C=] p.[Arg964Cys]; [Arg964 =] | Het | P | Molecular study for the variants into CMT1 cell/animal model. | |
Reanalysis WES 1-2 years later or check long-read sequencing, RNAseq, or WGS | |||||||||
39/52/M | Distal numbness | Sensorimotor polyneuropathy with demyelinating features. Autonomic dysfunction | CMT1 | SPTLC1 | c.[766C>T];[766C=] p.[Pro256Ser];[Pro256 =] | Het | VUS | Reanalysis WES 1-2 years later or check long-read sequencing, RNAseq, or WGS | |
*The segregation analysis: not cosegregation | |||||||||
40/Child-hood/M | Distal numbness | Sensorimotor polyneuropathy with axonal degeneration features | CMT2 | HARS | c.1255_1256delinsCT p.Lys419Leu | Het | VUS | Reanalysis WES 1-2 years later or check long-read sequencing, RNAseq, or WGS | |
*The segregation analysis: not cosegregation | |||||||||
41/79/M | Abnormal sensation below ankle | Motor-predominant polyneuropathy | Sensorimotor polyneuropathy. Left ventricular hypertrophy. | Not found | Reanalysis WES 1-2 years later or check long-read sequencing, RNAseq, or WGS |
DISCUSSION
WES is a powerful diagnostic approach for identifying pathogenic variants in patients with genetic diseases. Even when obtaining a positive finding from WES, the physician still needs to correlate the identified variants with the clinical phenotypes. Clinical challenges remain after obtaining a negative or undetermined WES. We focus our discussion on (1) designing molecular tests in patients with positive or inconclusive WES results and (2) a diagnostic approach for those with negative WES results.
In this study, our yield rate of WES is 63.4%, which is higher than the previous reports (yield rate of myopathic patients: 40–60% ; yield rate of neuropathy patients: 30–40% [5–10]). Our WES pipeline is same as most of the study, and reports having different pipelines such as using higher minor allele frequency < 0.02 or 0.05 as variant filtering criteria did not get higher yield rates. Several possibilities may explain this slightly higher yield rate. First, we considered the WES as the second-tier genetic test. After excluding common secondary cause, we would perform first-tier single gene test according to the deep phenotyping (e.g., D4Z4 repeats in the chromosome 4q in scapuloperoneal weakness and Ala117Ser in TTR gene in late-onset pan-modality neuropathy in Taiwanese). WES was served as the second-tier genetic test when the results of the first-tier test was negative. Second, we applied the segregation analysis with Sanger sequencing on the specific variants revealed by the proband-only WES, the cost of which is much lower than the cost of trio-WES. The procedure is mandatory for the cases with two variants but uncertain allele or the cases with only one previously unidentified variant. In our cohort, this procedure accompanied with muscle biopsy or further molecular examinations upgraded the patients with inconclusive WES result to definite clinical-molecular diagnosis in one case (Case 20), and exclude the variants as the pathogenic variants in two cases (Case 39 and 40). Of note, four patients could only meet the criteria of probable clinical-molecular diagnosis due to uncertainty of compound heterozygosity. Third, we arranged the specific genotype-guided molecular studies to investigate and confirm the proposed pathomechanism of different genetic diagnosis. The process of extensive clinical, molecular, and pathological correlation may give us additional yield [12].
For those cases with positive WES result, the final diagnosis could be reached by phenotype-genotype correlations. Interestingly, PMP22 deletion was found using WES in one patient who had negative results from extensive workups before receiving WES. In his previous genetic test, PMP22 deletion screening was performed using PCR restriction fragment length polymorphism. However, since the patient had a DNA deletion that included the restriction site, the results of gel electrophoresis may miss the deletion [25]. In Case 10, he presented weakness and hyperCKemia, and had positive family history of early respiratory failure. The TTN gene variant c.95136T>G (p.Cys31712Trp) was identified from our patient, and this variant had been reported in one Korean family with clinical features of HMERF [26]. However, our patient has not developed with respiratory failure currently and refused the muscle biopsy. He was categorized into a probable diagnosis. In summary, a validation with clinical manifestations, pathology, or other examinations is still essential in patients with positive WES results.
Among the 9 patients with inconclusive WES result, three patients (DES, DMD and TK2) achieved a final diagnosis via further pathology or molecular studies. All had a typical clinical phenotype. In case 18, the deletion from 1189 to 1206 base pairs of the DES gene is a formerly unreported new variant. With atrophic myocytes with desmin aggregates in scattered fibers in the muscle pathology, the patient was diagnosed with myofibrillar myopathy-1 according to the concordance of genotype, phenotype, and pathology [27, 28]. In addition to the typical pathognomonic findings, in case 20 with TK2 deficiency, we made a definite diagnosis using molecular examinations such as mitochondrial DNA sequencing and muscle electron transfer chain activity assays [29–31]. With a definite diagnosis, we could offer the patients more accurate genetic consultation, prognosis prediction, and the possibility of participation of clinical trial.
Not all patients or their families agreed to accept muscle biopsies or blood tests. Definite clinical-molecular diagnoses in our cohort were not achieved because of uncertain compound heterozygote (Cases 11, 21, 23, and 26), because of atypical manifestations according to literatures (Case 9, 10, and 12), because of unreported variants in the ClinVar database (Case 13 and 22) or because of current data limitations (Cases15, 16, 17, 24 and 25). For example, in Case 9, we only identified a variant in CAPN3 gene, which had been reported in patients with both autosomal dominance inheritance and autosomal recessive inheritance [32, 33]. However, the details of the phenotype of the variant with autosomal dominance inheritance was not clear in the literature [32]. Whether the single variant could lead to his symptoms, milder than typical LGMD R2, requires segregation analysis and further molecular studies. Another example is two variants of the NEFH gene noted in two patients (Cases 24 and 25) with a clinical diagnosis of ALS or probable PLS. The NEFH gene encodes the heavy neurofilament protein playing a role in intracellular transport to axons and dendrites. In molecular and animal studies, mutation of the NEFH gene affects the efficiency of survival of motor and sensory axons, while in human studies, deletions of the NEFH tail have been found in approximately 1% of sporadic motor neuron diseases [34, 35]. The above findings support the hypothesis that the NEFH variant is associated with susceptibility to ALS. Apart from the above reasons, Case 16, with a diagnosis of POLG-related parkinsonism with sensory neuronopathy, was grouped into the probable diagnosis category. The function of the enzyme encoded by POLG is the repair and replication of mitochondrial DNA. Pathogenic variants in the POLG gene may cause variable clinical manifestations including neuropathy, parkinsonism, cerebellar ataxia, epilepsy, and chronic progressive external ophthalmoplegia [36]. Although the case matched none of the POLG-related syndromes, he indeed presented with two of the main features: sensory neuronopathy and parkinsonism [37]. Clinical or tissue evidence of mitochondrial dysfunction warrants further mitochondrial DNA testing. In summary, a genotype-guided molecular studies were warranted to ascertain the pathogenicity of the identified variants.
In cases with negative WES results, further pursuit of a diagnosis is challenging. For example, in Case 27 myopathy was suspected but WES did not yield a diagnosis. Clinical observation of decreased body height and kyphosis led to a finding of osteoporosis and renal wasting hypophosphatemia. Finally, we linked right tibial tumor, renal wasting-related hypophosphatemia, muscle weakness, and osteoporosis together and arrived at an impression of tumor-induced osteomalacia. Further evaluation of serum FGF23 and tumor biopsy confirmed our diagnosis. Careful evaluation of clinical findings and searching for acquired causes should be performed before performing WES. If the WES result is undetermined, the acquired cause should be sought again.
Other than secondary causes, mitochondrial disease and microsatellite expansion disease should be kept in mind when WES is negative. Pathogenic variants in either the mitochondrial DNA or the nuclear DNA for mitochondria could lead to mitochondrial disease, but only nuclear DNA variants can be detected using WES. Therefore, it is crucial to consider mitochondrial disease in patients with maternally inherited patterns, exercise intolerance, ophthalmoplegia, or multisystemic disorders [38]. Of note, if a blood mtDNA yielded negative results, we would further arrange a muscle mtDNA test since heteroplasmy may be present. WES is incapable of detecting large-fragment copy number variations. Therefore, microsatellite expansion disease should still be put into the differential diagnosis when WES obtains negative findings. Additionally, a thorough evaluation of nerve system is essential to capture the deficits other than the peripheral nerve system. Of note, for those who had typical clinical presentation but an inconclusive pathology study, observation of treatment response could be helpful, for instance in our Case 30 with mononeuropathy multiplex.
The etiologies remained undetermined in eleven patients after WES and further workup. There are several possibilities. First, there should be secondary causes or culprit variants beyond our current knowledge that have not been evaluated. Second, under financial limitations, we checked mitochondrial genes only when clinically highly suspect. Although we did our best to evaluate all potential patients who had mitochondrial disease via detailed history taking, lactate exercise tests, muscle biopsies, and so on, a likelihood remains of missing patients with atypical phenotypes. Finally, the technological limitations of WES prevented us from detecting pathogenic variants in introns. Whole-genome sequencing or transcriptome studies in affected tissues may help to reach a final diagnosis for these patients.
Our study had limitations. First, this is a single-center study with a small cohort. Second, we did not set up a standard protocol for WES arrangement. To reduce selection bias, we recruited patients from only two attending physicians who reached a consensus about the inclusion criteria. Third, our study did not include pediatric patients. Expanding our findings to pediatric patients would require further study. Finally, the diversity of molecular studies for different genes was unavailable and resulted in many patients being placed into the probable diagnosis category.
CONCLUSION
WES facilitates the diagnosis of hereditary neuromuscular disorders. However, it is essential to pursue further molecular studies to correlate identified variants with clinical features. For patients who had negative findings from WES, secondary causes such as mitochondria and microsatellite expansion disease should be considered. Moreover, observation of treatment response, segregation analysis and further molecular evaluation should be beneficial to the final diagnosis.
DECLARATIONS
Consent publication
All authors have read and agreed the contents of this manuscript submitted to Journal of Neuromuscular Diseases.
AVAILABILITY OF DATA AND MATERIAL
The datasets generated during analysis and/or during the current study are available from the corresponding author on reasonable request.
COMPETING INTERESTS
None.
FUNDING
None.
AUTHOR’S CONTRIBUTIONS
All authors contributed to data acquisition and analysis. P.-S.C., N.-C.L., H.-W.H., and C.-C.Y. wrote the manuscripts with input from all authors.
ACKNOWLEDGMENTS
We confirm that we have read the Journal’s position on issues involved in ethical publication and affirm that this report is consistent with those guidelines.
SUPPLEMENTARY MATERIAL
[1] The supplementary material is available in the electronic version of this article: https://dx.doi.org/10.3233/JND-230013.
REFERENCES
[1] | Ross JP , Dion PA , Rouleau GA . Exome sequencing in genetic disease: recent advances and considerations. FRes. (2020) ;9: . |
[2] | Westra D , et al. Panel-based exome sequencing for neuromuscular disorders as a diagnostic service. J Neuromuscul Dis. (2019) ;6: (2):241–58. |
[3] | Haskell GT , et al. Diagnostic utility of exome sequencing in the evaluation of neuromuscular disorders. Neurol Genet. (2018) ;4: (1):e212. |
[4] | Ababneh NA , et al. The utility of whole-exome sequencing in accurate diagnosis of neuromuscular disorders in consanguineous families in Jordan. Clin Chim Acta. (2021) ;523: :330–8. |
[5] | Hartley T , et al. Whole-exome sequencing is a valuable diagnostic tool for inherited peripheral neuropathies: Outcomes from a cohort of 50 families. Clin Genet. (2018) ;93: (2):301–9. |
[6] | Walsh M , et al. Diagnostic and cost utility of whole exome sequencing in peripheral neuropathy. Ann Clin Transl Neurol. (2017) ;4: (5):318–25. |
[7] | Fichna JP , et al. Whole-exome sequencing identifies novel pathogenic mutations and putative phenotype-influencing variants in Polish limb-girdle muscular dystrophy patients. Human Genomics. (2018) ;12: (1):34. |
[8] | Ghaoui R , et al. Use of whole-exome sequencing for diagnosis of limb-girdle muscular dystrophy: Outcomes and lessons learned. JAMA Neurol. (2015) ;72: (12):1424–32. |
[9] | Babić Božović I . et al. Diagnostic yield of exome sequencing in myopathies: Experience of a Slovenian tertiary centre. . PLoS One. (2021) ;16: (6):e0252953. |
[10] | Chae JH , et al. Utility of next generation sequencing in genetic diagnosis of early onset neuromuscular disorders. Journal of Medical Genetics. (2015) ;52: (3):208–16. |
[11] | Frésard L , Montgomery SB . Diagnosing rare diseases after the exome. Cold Spring Harb Mol Case Stud. (2018) ;4: (6). |
[12] | Thompson R , et al. Advances in the diagnosis of inherited neuromuscular diseases and implications for therapy development. The Lancet Neurology. (2020) ;19: (6):522–32. |
[13] | Wang K , Li M , Hakonarson H . ANNOVAR: Functional annotation of genetic variants from high-throughput sequencing data. Nucleic Acids Res. (2010) ;38: (16):e164. |
[14] | Richards S , et al. Standards and guidelines for the interpretation of sequence variants: A joint consensus recommendation of the American College of Medical Genetics and Genomics and the Association for Molecular Pathology. Genet Med. (2015) ;17: (5):405–24. |
[15] | Retterer K , et al. Clinical application of whole-exome sequencing across clinical indications. Genet Med. (2016) ;18: (7):696–704. |
[16] | Abou Tayoun AN , et al. Recommendations for interpreting the loss of function PVS1 ACMG/AMP variant criterion. Hum Mutat. (2018) ;39: (11):1517–24. |
[17] | Biesecker LG , Harrison SM . The ACMG/AMP reputable source criteria for the interpretation of sequence variants. Genet Med. (2018) ;20: (12):1687–8. |
[18] | Ghosh R , et al. Updated recommendation for the benign stand-alone ACMG/AMP criterion. Hum Mutat. (2018) ;39: (11):1525–30. |
[19] | Resource CG . Sequence variant interpretation.; Available from: https://clinicalgenome.org/working-groups/sequence-variant-interpretation/. |
[20] | Babadi M , et al. Abstract 2287: Precise common and rare germlineCNVcalling withGATK. Bioinformatics and Systems Biology, 2018. |
[21] | Biesecker LG , Nussbaum RL , Rehm HL . Distinguishing variant pathogenicity from genetic diagnosis: How to Know Whether a Variant Causes a Condition. Jama. (2018) ;320: (18):1929–30. |
[22] | Cheng C-Y , et al. Thymidine kinase 2 deficiency–induced adult-onset ptosis and proximal weakness. Neurology: Clinical Practice. (2021) ;11: (3):e379–e382. |
[23] | Ohlsson M , et al. Hereditary myopathy with early respiratory failure associated with a mutation in A-band titin. Brain. (2012) ;135: (Pt 6):1682–94. |
[24] | Palmio J , et al. Hereditary myopathy with early respiratory failure: Occurrence in various populations. J Neurol Neurosurg Psychiatry. (2014) ;85: (3):345–53. |
[25] | Hung CC , et al. Identification of deletion and duplication genotypes of the PMP22 gene using PCR-RFLP, competitive multiplex PCR, and multiplex ligation-dependent probe amplification: A comparison. Electrophoresis. (2008) ;29: (3):618–25. |
[26] | Yeo Y , Park JE , Kwon HS . A novel TTN gene variant c. 6T>G (Cys2Trp) and associated clinical characteristics in a family with suspected hereditary myopathy with early respiratory failure. Ann Lab Med. (2021) ;41: (6):604–7. |
[27] | Luo Y-B , et al. Expanding the clinico-genetic spectrum of myofibrillar myopathy: experience from a chinese neuromuscular Center. Frontiers in Neurology. (2020) )11. |
[28] | Selcen D . Myofibrillar myopathies. Neuromuscul Disord. (2011) ;21: (3):161–71. |
[29] | Wang J , El-Hattab AW , Wong LJC . TK2-Related Mitochondrial DNA Maintenance Defect, Myopathic Form, in GeneReviews(®), M.P. Adam, et al., Editors. 1993, University of Washington, Seattle. Copyright © 1993-2022, University of Washington, Seattle. GeneReviews is a registered trademark of the University of Washington, Seattle. All rights reserved.: Seattle (WA). |
[30] | El-Hattab AW , Scaglia F . Mitochondrial DNA depletion syndromes: Review and updates of genetic basis, manifestations, and therapeutic options. Neurotherapeutics. (2013) ;10: (2):186–98. |
[31] | El-Hattab AW , Craigen WJ , Scaglia F . Mitochondrial DNA maintenance defects. Biochimica et Biophysica Acta (BBA) - Molecular Basis of Disease. (2017) ;1863: (6):1539–55. |
[32] | Köken ÖY , et al. P.161 The molecular landscape of CAPN3 mutations in limb-girdle muscular dystrophy: Experience of tertiary center from Turkey. Neuromuscular Disorders. (2022) ;32: :S114. |
[33] | Özyilmaz B , et al. Impact of next-generation sequencing panels in the evaluation of limb-girdle muscular dystrophies. Annals of Human Genetics. (2019) ;83: (5):331–47. |
[34] | Skvortsova V , et al. Analysis of heavy neurofilament subunit gene polymorphism in Russian patients with sporadic motor neuron disease (MND). European Journal of Human Genetics. (2004) ;12: (3):241–4. |
[35] | Jacquier A , et al. Cryptic amyloidogenic elements in mutant NEFH causing Charcot-Marie-Tooth 2 trigger aggresome formation and neuronal death. Acta Neuropathologica Communications. (2017) ;5: (1):55. |
[36] | Hsieh PC , et al. POLG R964C and GBA L444P mutations in familial Parkinson’s disease: Case report and literature review. Brain Behav. (2019) ;9: (5):e01281. |
[37] | Rahman S , Copeland WC . POLG-related disorders and their neurological manifestations. Nature Reviews Neurology. (2019) ;15: (1):40–52. |
[38] | Orsucci D , et al. Mitochondrial Syndromes Revisited. J Clin Med. (2021) ;10: (6). |