Profiling Serum Antibodies Against Muscle Antigens in Facioscapulohumeral Muscular Dystrophy Finds No Disease-Specific Autoantibodies
Abstract
Background:
FSHD is caused by specific genetic mutations resulting in activation of the Double Homeobox 4 gene (DUX4). DUX4 targets hundreds of downstream genes eventually leading to muscle atrophy, oxidative stress, abnormal myogenesis, and muscle inflammation. We hypothesized that DUX4-induced aberrant expression of genes triggers a sustained autoimmune response against skeletal muscle cells.
Objective:
This study aimed at the identification of autoantibodies directed against muscle antigens in FSHD. Moreover, a possible relationship between serum antibody reactivity and DUX4 expression was also investigated.
Methods:
FSHD sera (N = 138, 48±16 years, 48% male) and healthy control sera (N = 20, 47±14 years, 50% male) were analyzed by immunoblotting for antibodies against several skeletal muscle protein extracts: healthy muscle, FSHD muscle, healthy and FSHD myotubes, and inducible DUX4 expressing myoblasts. In addition, DUX4 expressing myoblasts were analyzed by immunofluorescence with FSHD and healthy control sera.
Results:
The results showed that the reactivity of FSHD sera did not significantly differ from that of healthy controls, with all the tested muscle antigen extracts. Besides, the immunofluorescent staining of DUX4-expressing myoblasts was not different when incubated with either FSHD or healthy control sera.
Conclusion:
Since the methodology used did not lead to the identification of disease-specific autoantibodies in the FSHD cohort, we suggest that autoantibody-mediated pathology may not be an important disease mechanism in FSHD. Nevertheless, it is crucial to further unravel if and which role the immune system plays in FSHD pathogenesis. Other innate as well as adaptive immune players could be involved in the complex DUX4 cascade of events and could become appealing druggable targets.
INTRODUCTION
FSHD is a worldwide prevalent myopathy [1] usually inherited in an autosomal dominant fashion, although complex digenic patterns of inheritance have also been described [2]. Progressive muscle weakness and atrophy of face and humero-scapular girdles are the hallmarks of the disease, with eventual spreading of these symptoms to the pelvic and lower limb regions [2].
The FSHD genetic locus was linked to the macrosatellite repeat array D4Z4 at the subtelomeric region of chromosome 4 in the early nineties [3]. Subsequently, the “unifying genetic mechanism model” was accepted as the most accredited disease mechanism [4]. This model postulates that the loss of an epigenetic silencing pathway, either due to D4Z4 contraction to < 10 repeats units in 95% of cases (FSHD1) [5], or due to mutations in D4Z4 chromatin modifiers SMCHD1, DNMT3B or LRIF1 in 5% of cases (FSHD2) [6], results in a failure of repression of the gene encoding the germline and cleavage stage transcription factor Double Homeobox 4 (DUX4) [7]. DUX4, which is otherwise epigenetically silenced in skeletal muscles, poses toxicity to skeletal muscle cells [8].
DUX4 is an embryonic transcription factor with hundreds of downstream targets among which immune genes and cancer testis antigens (CTA) and these genes are abnormally activated in FSHD skeletal muscles [9, 10]. CTA, for instance, can become a target of the immune system when mis-expressed in cancer cells [11]. The elicited immune response could activate both cellular (cytokine mediated) and humoral (antibody mediated) immunity with consequent tissue damage and autoantigen expression, perpetuating a self-inflammatory loop [12].
In sporadic inclusion body myositis (IBM), a neuromuscular disorder that together with dermatomyositis (DM) and polymyositis (PM) belongs to the idiopathic inflammatory myopathy (IIM) family, the use of muscle tissue as antigenic material allowed us to identify specific autoantibodies to skeletal muscle antigens such as cytosolic 5’-nucleotidase 1A (cN1A) [13]. Although FSHD is not categorized as an inflammatory myopathy, inflammatory features are described in about 40% of FSHD muscle biopsies [14, 15]. These inflammatory infiltrates often mimic a myositis-like event [16], supporting the theory that an immune response may be associated with FSHD pathogenesis [17]. However, it is not clear whether the observed muscle inflammation may be related to the underlying primary cause (that is DUX4 expression and expression of its downstream target genes). Similarly, it remains unsettled whether muscle inflammation may promote disease progression with progressive fatty-fibrotic replacement of muscles.
This study aims at investigating the presence of antibodies directed against skeletal muscle antigens in a large series of well characterized FSHD patient sera with the objective to explain the possible nature of the inflammatory cellular infiltrates often found in FSHD muscle specimens [18]. We hypothesized that DUX4-induced aberrant expression of genes triggers a sustained autoimmune reaction against skeletal muscle cells (Fig. 1). To test this hypothesis, we tested the reactivity of FSHD sera and healthy control sera (as a source of antibodies) on several muscle tissue and myoblast/myotube cell line extracts (as a source of muscle antigens). In addition, we investigated whether a relationship exists between serum reactivity and DUX4 expression.
Fig. 1
Graphical representation of study hypothesis. DUX4-induced aberrant expression of genes triggers a sustained autoimmune reaction against skeletal muscle cells.
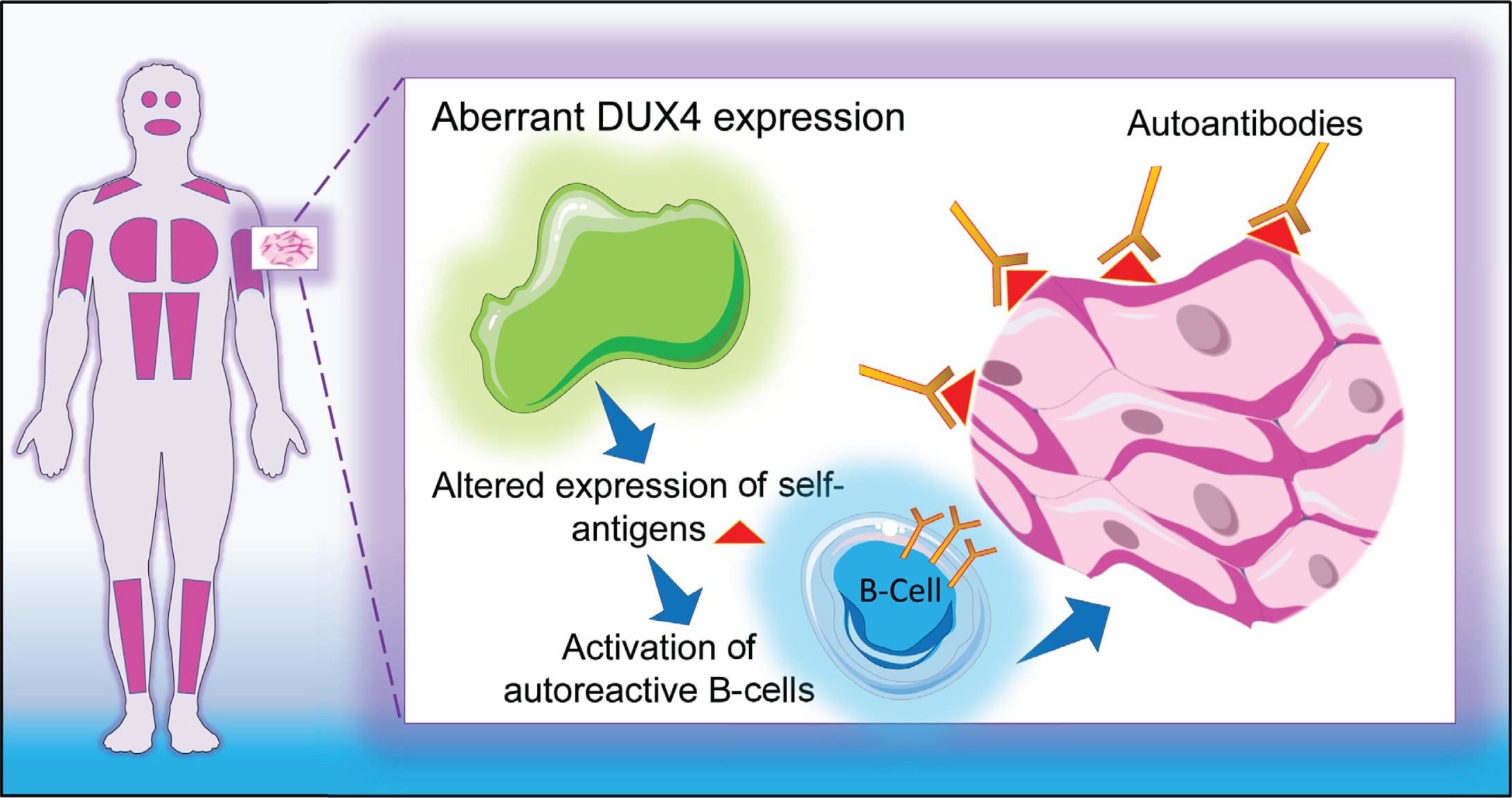
MATERIAL AND METHODS
Participants
Genetically confirmed FSHD patients (N = 138) were retrospectively recruited from the FSHD–FOCUS cohort study (Nijmegen, The Netherlands). Serum samples from all patients were provided by Radboud Biobank, Nijmegen, the Netherlands. Healthy control sera (N = 20) were either obtained from the Sanquin Blood Supply Foundation (Nijmegen, the Netherlands) or collected at the neurology out-patient clinic (Radboud university medical center, Nijmegen, The Netherlands) from healthy volunteers participating in the FSHD-Inflammation study (Nijmegen, The Netherlands). Gender and age at examination were recorded for both groups. Additionally, disease duration, Medical Research Council (MRC) sum score, Ricci Score, and 'Lamperti Score were recorded for the patient group. Disease duration was determined from age at disease onset. The MRC sum score ranges from 0 (complete paralysis) to 60 (normal muscle strength) and was obtained by summing the manual muscle testing scores of three upper limb muscles (shoulder abductors, elbow flexors, and wrist extensors) and three lower limb muscles (hip flexors, knee extensors, and foot dorsiflexors). The Ricci Score (0–10 scale) [19] and and the Lamperti Score (0–15 scale) [20] take into account the extent of weakness in various body regions and considers the descending spread of symptoms from face and shoulders to pelvic and leg muscles typical of FSHD. A subset of 109 patients out of 138 underwent a full leg magnetic resonance imaging (MRI) examination as described by Mul et al [21]. Briefly, transversal Dixon and short tau inversion recovery (STIR) sequences were acquired from the upper and lower leg to evaluate the degree of muscle fatty infiltration and inflammation, respectively. Finally, FSHD genetics characteristics (FSHD type and D4Z4 repeat length) were recorded as well from all FSHD patients. See Table 1 for additional details.
Table 1
Demographics and clinical characteristics of FSHD patients and healthy controls
FSHD patients | HC | |
Demographics | ||
Number of participants (% male) | 138 (48 %) | 20 (50 %) |
Age at examination mean y±SD | 48±16 | 47±14 |
Clinical features | ||
Disease duration mean y±SD | 25±15 | n/a |
MRC Sum Score (0–60) mean±SD | 49±10 | n/a |
Ricci Score (0–10) mean±SD | 5±3 | n/a |
Lamperti Score (0–15) mean±SD | 6±4 | |
Leg MRI STIR hyperintensity % | 47% (51/109) | n/a |
Genetics | ||
FSHD1 n/tot. n | 131/138 | n/a |
FSHD2 n/tot. n | 7/138 | n/a |
D4Z4 repeat units mean±SD | 6±2 | n/a |
This study was conducted according to the principles of the Declaration of Helsinki (version October 2013) and in accordance with the Medical Research Involving Human Subjects Act (WMO). The local Institutional Review Board (CMO region Arnhem-Nijmegen, The Netherlands) approved the study under file number CMO 2017–3119. All subjects provided written informed consent.
Workflow
The reactivity of FSHD sera and healthy control sera was tested with different classes of muscle tissue and myoblast/myotube cell line extracts (as a source of muscle antigens) following a stepwise workflow: 1) healthy human muscle, 2) FSHD muscle, 3) healthy control myotubes, 4) FSHD myotubes, and 5) inducible DUX4 myoblasts (Fig. 2).
Fig. 2
Muscle antigen preparations. Protein extracts were prepared from healthy control (A) and FSHD (B) skeletal muscle biopsies, from cultured healthy (C) and FSHD myotubes (D) and from DUX4-expressing myoblasts (E). The reactivity of FSHD and HC sera with each of these extracts was analyzed by immunoblotting. This figure was created adapting Servier Medical Art templates. Original images are licensed under a Creative Commons Attribution 3.0 Unported License.
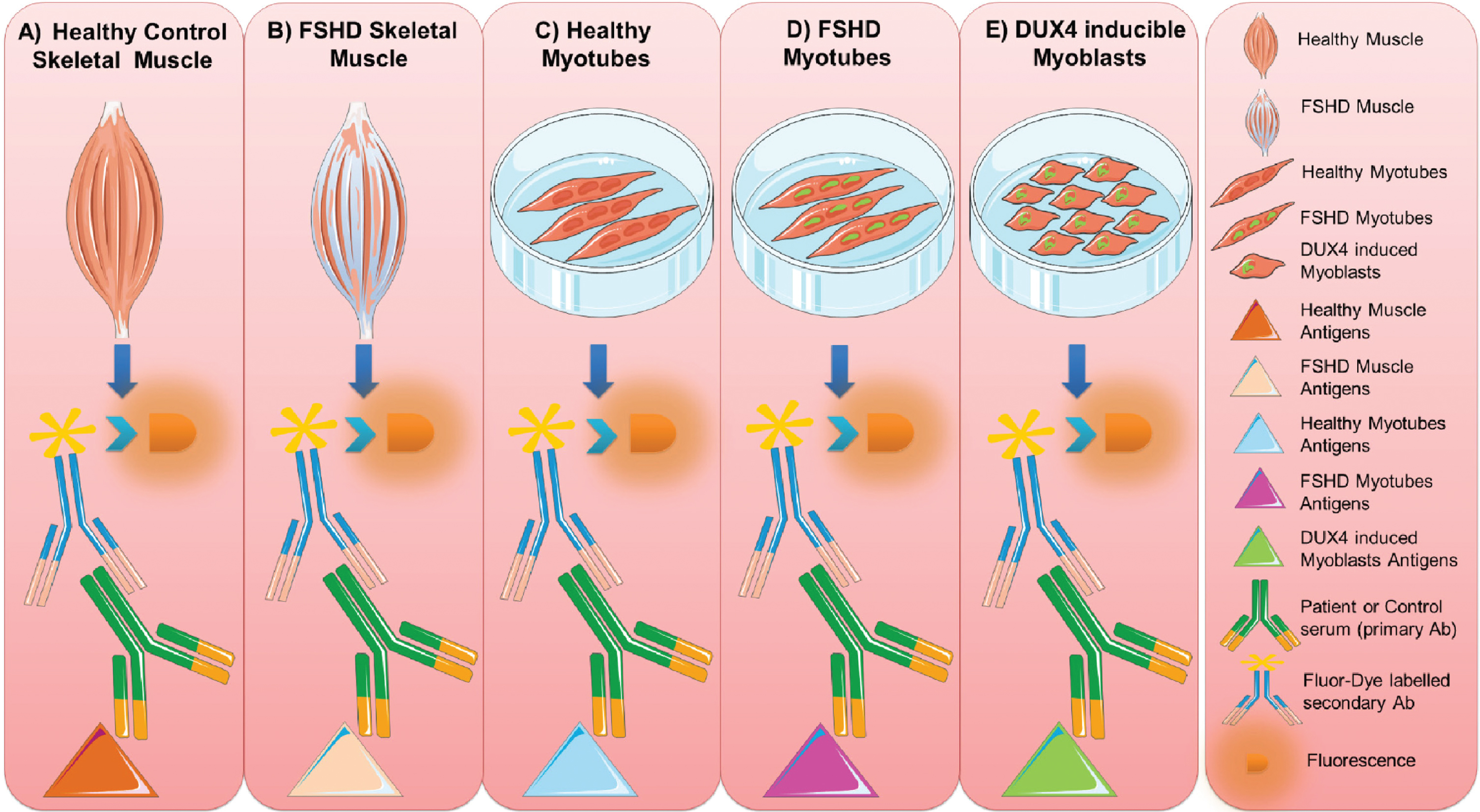
Muscle tissue protein extracts
Human healthy muscle specimens obtained from knee surgery leftovers and FSHD quadriceps needle muscle biopsies (see Table 2 for additional information) were snap frozen in liquid nitrogen and stored at –80 °C. Frozen healthy muscle pieces and FSHD muscle pieces were pulverized with a Microdismembrator (Braun Biotech International, Melsungen, Germany), resuspended in 10 volumes of RIPA buffer (50 mM Tris-HCl, pH 7.4, 150 mM NaCl, 0.1% SDS, 1% Nonidet P-40, 0.5% sodium deoxycholate (DOC), and Complete protease inhibitor cocktail), sonicated at 4 °C, and centrifuged for 15 minutes at 13.000 g. Healthy and FSHD muscle tissue protein extracts were separated by SDS-PAGE followed by western blotting.
Table 2
Muscle protein extracts
Status | Source | Identifier | D4Z4 length |
FSHD1 | VL muscle needle biopsy from a FSHD patient | FSHD124 | 3 |
FSHD1 | VL muscle needle biopsy from a FSHD patients | FSHD106 | 5 |
Control | human myotubes derived from a healthy subject | MT2417 | N/A |
FSHD1 | human myotubes derived form a FSHD patient | MT2402 | 3 |
FSHD2 | human myotubes derived form a FSHD patient | MT2338 | N/A |
Control | immortalized human myoblast cell lines with integrated inducible DUX4 construct | Imm1926DUX4iclone 5 | N/A |
VL = quadriceps muscle vastus lateralis.
Myotube protein extracts
Human healthy control (mHC), FSHD1 (mF1), and FSHD2 (mF2) myotubes (see Table 2 for additional information) were washed three times in cold PBS, resuspended in LDS buffer (NuPAGE™ LDS Sample Buffer, Invitrogen) (mHC and mF1) or RIPA buffer (mF2) for 30 minutes to ensure complete cell lysis, and centrifugated for 15 minutes at 13,000 g. mHC, mF1, and mF2 protein extracts were separated by SDS-PAGE followed by western blotting.
Inducible DUX4 myoblasts protein extracts
Primary myoblasts from a healthy control individual (see Table 2 for additional information) were first immortalized with hTERT and CDK4 transductions, followed by random insertion of codon-altered DUX4 cDNA sequence as described in detail by Jagannathan et al. [22]. In short, cells were transduced with lentivirus containing the plasmid pCW57.1-DUX4-CA (Addgene, #99281). Cells were selected and maintained in 1μg/ml puromycin containing medium. Individual clones were generated by single cell dilution cultures in 96-well format and clones were individually validated for DUX4-induction upon doxycycline treatment and DUX4-induced cytotoxicity within 24 hours. Inducible DUX4 myoblasts were next cultured in proliferation medium F-10, supplemented with 20% fetal bovine serum (FBS), 1% penicillin/streptomycin (GIBCO), 1μM dexamethasone (DEX), and 10 ng/ml FGF-basic and 1μg/ml puromycin at 37 °C in a 5% CO2 atmosphere. DUX4 induction was achieved by a 17 hours incubation of cultured myoblasts in above described proliferation medium, supplemented with 2μg/ml doxycycline (DOX). Protein extract from myoblasts with induced DUX4 expression (DOX+) and without DUX4 induction taken as negative control (DOX-) were used for immunoblotting as described above.
Immunoblotting
Muscle, myotubes, and inducible DUX4 myoblasts protein extracts prepared as described above, were separated by SDS-polyacrylamide gel electrophoresis (SDS-PAGE), followed by transfer to nitrocellulose membranes. Three millimeter wide strips were cut and blocked in phosphate-buffered saline, 0.1% Tween-20 (PBS-T), 5% non-fat dry milk, and incubated with FSHD patient or healthy control sera, 500-fold diluted in 5% milk in PBS-T. Subsequently, strips were incubated with the secondary antibody goat–anti-human immunoglobulin conjugated to IRDye800 (Rockland, Gilbertsville, PA) and visualized with the Odyssey system (LI-COR Biosciences, Lincoln, NE).
Immunofluorescence staining
A possible relationship between DUX4 expression and serum reactivity was investigated by immunofluorescence staining of DUX4 expressing myoblasts incubated with either FSHD patient or healthy control sera. Immunofluorescence staining was performed on an inducible DUX4 cell line (1926 iDUX4 cell line). Cells were fixed in 2% paraformaldehyde-PBS solution for 15 min at room temperature and washed twice with PBS. Subsequently, cells were permeabilized in 1% Triton X-100, PBS solution, washed with PBS, and incubated for 1 hour at room temperature with rabbit anti-DUX4 antibody (E5.5, ad124699, Abcam, 2000-fold diluted) and FSHD patient or healthy control sera (10-, 100-, or 500-fold diluted). Cells were then washed three times with PBS for 10 min at room temperature, followed by incubation with goat-anti-rabbit immunoglobulin conjugated to AlexaFluor 488 (400-diluted) and goat-anti-human antibody conjugated to AlexaFluor 568 (400-fold diluted). After 1 hour of incubation, cells were washed three times with PBS and incubated with DAPI for 10 minutes. Images were captured with a widefield fluorescence microscope (Leica LAS X).
Statistics
The sample size of this study was not based on a power statistical analysis. Immunoblots and immunofluorescent staining were qualitatively analyzed. Subject characteristics, observed reactivities frequencies, staining pattern and staining intensities were summarized through a descriptive statistical analysis conducted using GraphPad prism 5 (GraphPad software, La Jolla, CA).
RESULTS
Participants characteristics
Up to one hundred and thirty eight FSHD patient sera (48±16 years, 48% male) and twenty healthy control sera (47±14 years, 50% male) were included for antibody screening against skeletal muscular antigens. The total number of tested sera in each set of experiments is specified in the following sub-sections. Demographic, clinical, and genetics characteristics of patients and healthy controls are summarized in Table 1.
Reactivity of sera with healthy human skeletal muscle protein extract
In total, 138 sera of FSHD patients (FSHD1 = 131; FSHD2 = 7) and 11 sera of healthy controls were tested for autoantibody reactivities with healthy skeletal muscle protein extract by immunoblotting (Supplementary Figure 1). The results showed 5 relatively frequently occurring bands. Reactivity with a polypeptide of approximately 160 kDa was observed with 20 FSHD sera; reactivity with a polypeptide of approximately 98 kDa with 10 FSHD sera; reactivity with a polypeptide of approximately 73 kDa with 20 FSHD sera; reactivity with a polypeptide of approximately 48 kDa with 7 FSHD sera; and reactivity with a polypeptide of approximately 36 kDa with 26 FSHD sera. Additional immunoblots, in which the reactive FSHD sera (Fig. 3 A-E) and healthy control sera (Fig. 3F) were analyzed on flanking strips from the same blot revealed that similar reactivities were also seen with some healthy control samples. Additionally, we did not observe differences between FSHD1 and FSHD2 sera reactivities. We did not observe significant differences as well between the reactivity of FSHD patient sera reporting STIR hyperintensity on leg MRI and those without STIR hyperintensity. The frequencies by which these reactivities were observed in each group are summarized in Table 3.
Fig. 3
Reactivity of FSHD patient sera and HC sera with healthy human skeletal muscle protein extract. Healthy human skeletal muscle lysate was separated by SDS-PAGE and transferred to nitrocellulose membranes. Blot strips were incubated with 138 FSHD patient sera (A–E) and 11 healthy control sera (F), and bound antibodies were visualized by IRDye-labeled secondary antibodies. Patient sera are coded as sF followed by a number, and healthy control sera as sHC followed by a number. The left side of each panel indicates the position of molecular weight markers. Representative patient samples show reactivity at approximately 160 kDa (A), 98 kDa (B), 73 kDa (C), 48 kDa (D), and 36 kDa (E); representative HC samples show reactivity at 160, 98, 73, 48, and 36 kDa (F).
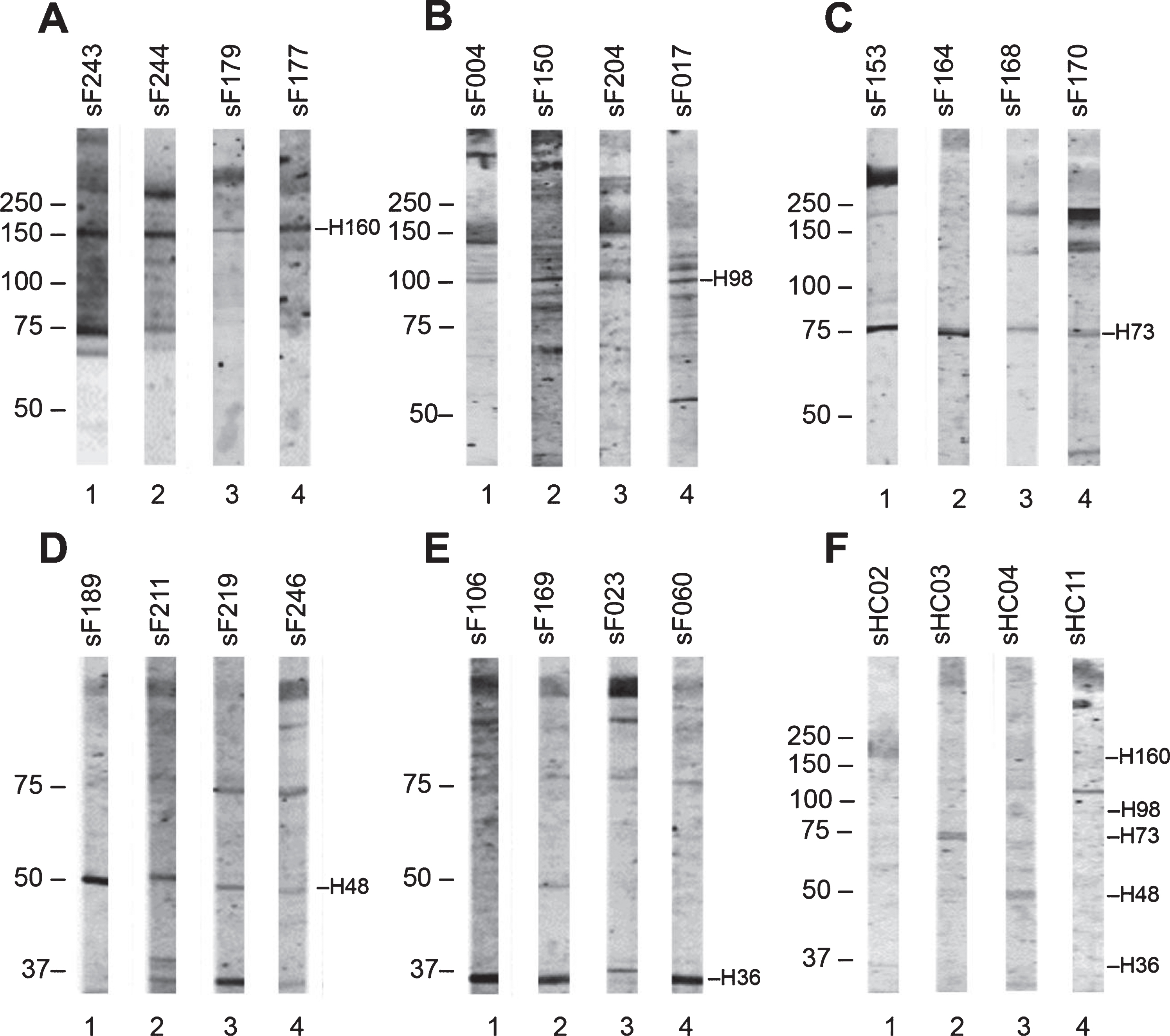
Table 3
Frequency of FSHD and HC sera reactive against healthy muscle protein extract (H)
Band kDa | FSHD Sera % n = 138 | FSHD Sera STIR+ % n = 51 | FSHD Sera STIR- % n = 58 | HC Sera % n = 11 |
H-160 | 15 (21/138) | 8 (4/51) | 12 (7/58) | 18 (2/11) |
H-98 | 7 (10/138) | 4 (2/51) | 4 (2/58) | 9 (1/11) |
H-73 | 14 (20/85) | 6 (3/51) | 7 (4/58) | 27 (3/11) |
H-48 | 5 (7/85) | 6 (3/51) | 3 (2/58) | 9 (1/11) |
H-36 | 14 (10/138) | 14 (7/51) | 9 (5/58) | 18 (2/11) |
Reactivity of sera with FSHD skeletal muscle protein extract
Since the protein composition of skeletal muscle tissue of FSHD patients may be partially different from that of healthy individuals, 85 FSHD sera (FSHD1 = 84; FSHD2 = 1) and 3 healthy control sera were subsequently analyzed with an extract of FSHD muscle biopsies (see Table 2 for additional details about FSHD muscle biopsies). The most frequently occurring reactivities on immunoblots were observed with polypeptides of approximately 60 kDa (6 FSHD sera; 1 healthy control serum), 45 kDa (3 FSHD sera; none of the healthy control sera) and 37 kDa (3 FSHD sera; 1 healthy control serum) (Supplementary Figure 2). A representative set of tested patient and control immunoblots are shown in Fig. 4. The frequencies by which these reactivities were observed are summarized in Table 4.
Fig. 4
Reactivity of FSHD patient sera and HC sera with FSHD quadriceps muscle protein extract. FSHD muscle lysate (F) and, as a reference an extract from healthy muscles (H), was separated by SDS-PAGE and transferred to nitrocellulose membranes. Blot strips were incubated with 85 FSHD patient sera (sF) (A) and with 3 healthy control sera (sHC) (B). Data for only a few representative samples are shown. The left side of each panel indicates the position of molecular weight markers.
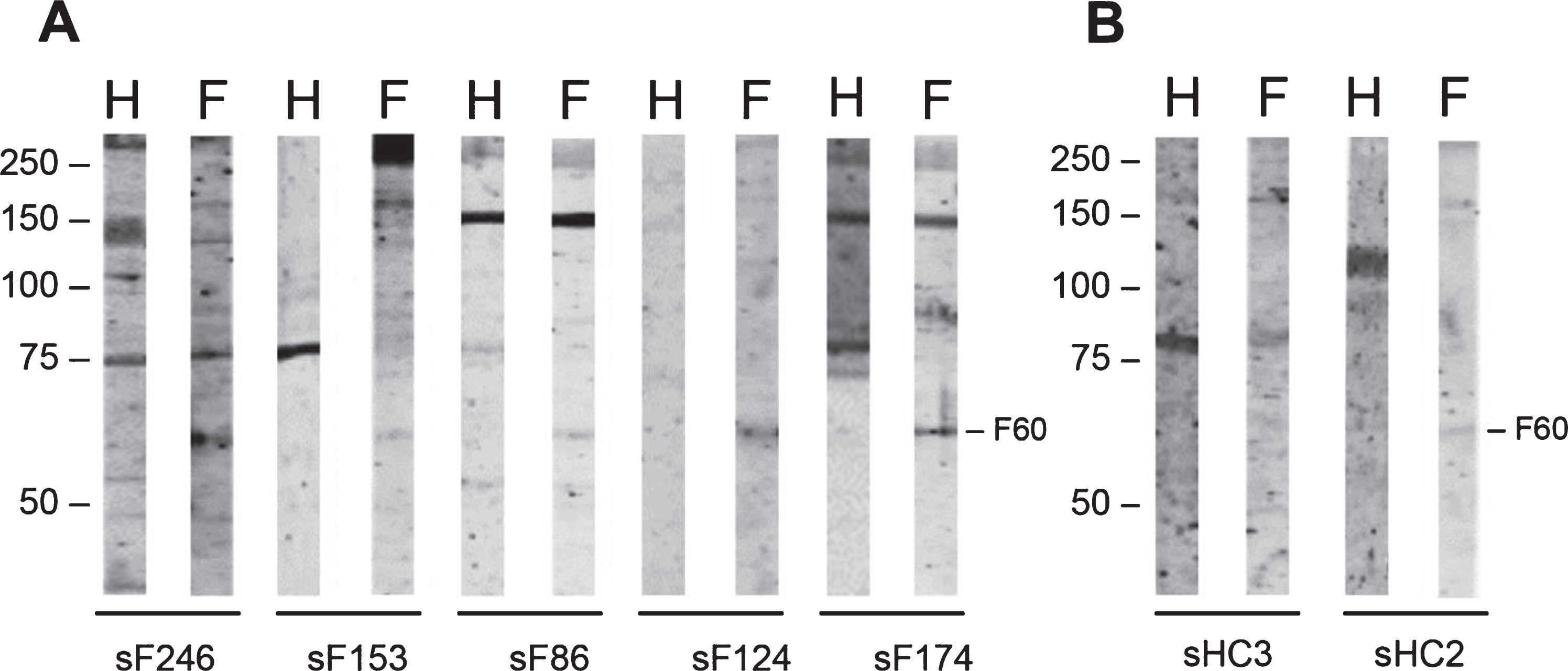
Table 4
Frequency of FSHD and HC sera reactive against FSHD muscle protein extract (F)
Band kDa | FSHD Sera % n = 85 | HC Sera % n = 3 |
F-60 | 7 (6/85) | 33 (1/3) |
F-45 | 2 (2/85) | – |
F-37 | 3 (3/85) | 33 (1/3) |
Reactivity of sera with protein extract from cultured myotubes
Since skeletal muscle biopsies not only contain muscle fibers but also other cell types, we decided to use also extracts from cultured myotubes as a source of antigens. The reactivity of 49 FSHD sera (FSHD1 = 48; FSHD2 = 1) and 3 healthy control sera was analyzed with extracts from (i) myotubes derived from a healthy individual (HC), (ii) myotubes derived from a FSHD1 patient (F1), and (iii) myotubes derived from a FSHD2 patient (F2), again by immunoblotting (Supplementary Figure 3). In spite of the appearance of reactive bands on both patient and control blot strips, a specific reactivity pattern was not observed. A polypeptide of approximately 60 kDa was relatively frequently detected with the FSHD1 and FSHD2 extracts (reactive with 6 FSHD sera), but not with the HC extract (Fig. 5). However, a 60 kDa polypeptide was also recognized by one of the three healthy control sera, although this was observed for all types of myotube extracts (Fig. 5). The frequencies of these reactivities are summarized in Table 5.
Fig. 5
Reactivity of FSHD patient sera and HC sera with FSHD myotubes protein extract. FSHD1 myotubes protein extract (mF1), FSHD2 myotubes protein extract (mF2), and healthy myotubes protein extract (mHC) were separated by SDS-PAGE and transferred to nitrocellulose membranes. Blot strips were incubated with 49 FSHD patient sera (sF) (A) and with 3 healthy control sera (sHC) (B). Panels contain a representative set of tested samples. On the left of each panel the positions of molecular weight markers are indicated.
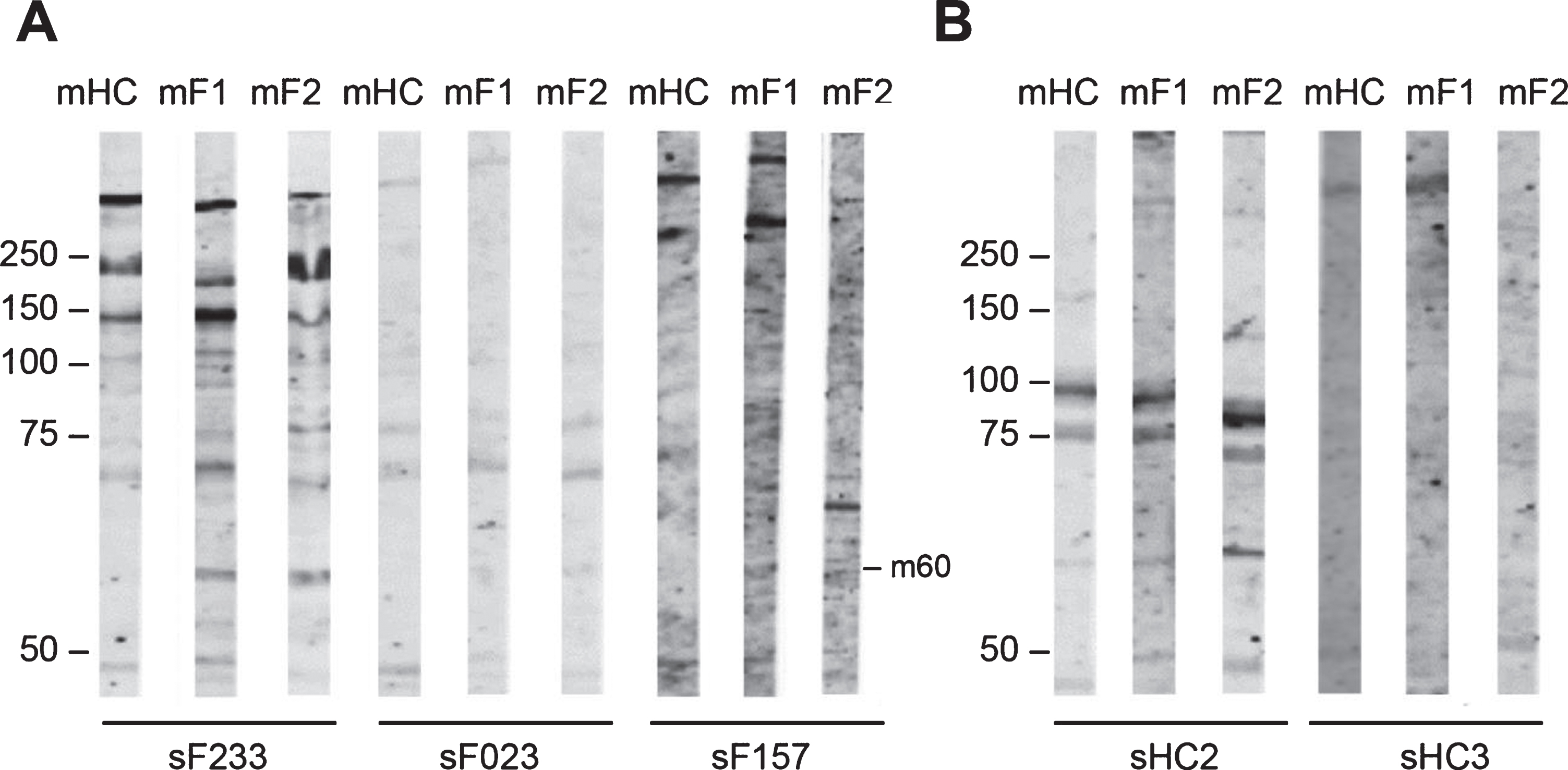
Table 5
Frequency of FSHD and HC sera reactive against myotube protein extract (m)
Band kDa | FSHD Sera % n = 49 mF1 | FSHD Sera % n = 49 mF2 | HC Sera % n = 3 mF1 | HC Sera % n = 3 mF2 |
m-250 | 4 (2/49) | – | – | – |
m-120 | 6 (3/49) | 2 (1/49) | – | – |
m-70 | 2 (1/49) | 4 (2/49) | – | – |
m-60 | 14 (7/49) | 12 (6/49) | 33 (1/3) | 33 (1/3) |
m-50 | 4 (2/49) | – | – | – |
m-45 | 6 (3/49) | 6 (3/49) | – | – |
m-37 | – | 12 (6/49) | 33 (1/3) | 33 (1/3) |
m-30 | – | 6 (3/49) | – | – |
Reactivity of sera with inducible DUX4 myoblasts protein extract
Although no potentially FSHD-specific autoantibody reactivities were detected with the FSHD myotubes protein extracts, we further explored the possibility that DUX4 induced the expression of autoantigenic proteins by using extracts from a myoblast cell line in which DUX4 was expressed. To investigate the presence of autoantibodies to these proteins in FSHD patient sera, 86 sera (FSHD1 = 84; FSHD2 = 2) and 3 healthy control sera were incubated with blot strips containing extracts from these myoblasts, in which DUX4 expression was induced by doxycycline (DOX+) (Supplementary Figure 4). Note that in these cultures DUX4 was detectably expressed in only a subset of nuclei (Fig. 6A).
Fig. 6
Reactivity of sera with DUX4-inducible myoblasts protein extract. Transfected myoblasts treated (DOX+) and non-treated with doxycycline (DOX-) were stained with DAPI (blue) and with an anti-DUX4 antibody (green) (A). DUX4-expressing myoblast protein extract (+) and control myoblast protein extract (–) were separated by SDS-PAGE and transferred to nitrocellulose membranes. Blot strips were incubated with 86 FSHD patient sera (sF) (B) and with 3 HC sera. Panel contains a representative set of tested samples. On the left the positions of molecular weight markers are indicated.
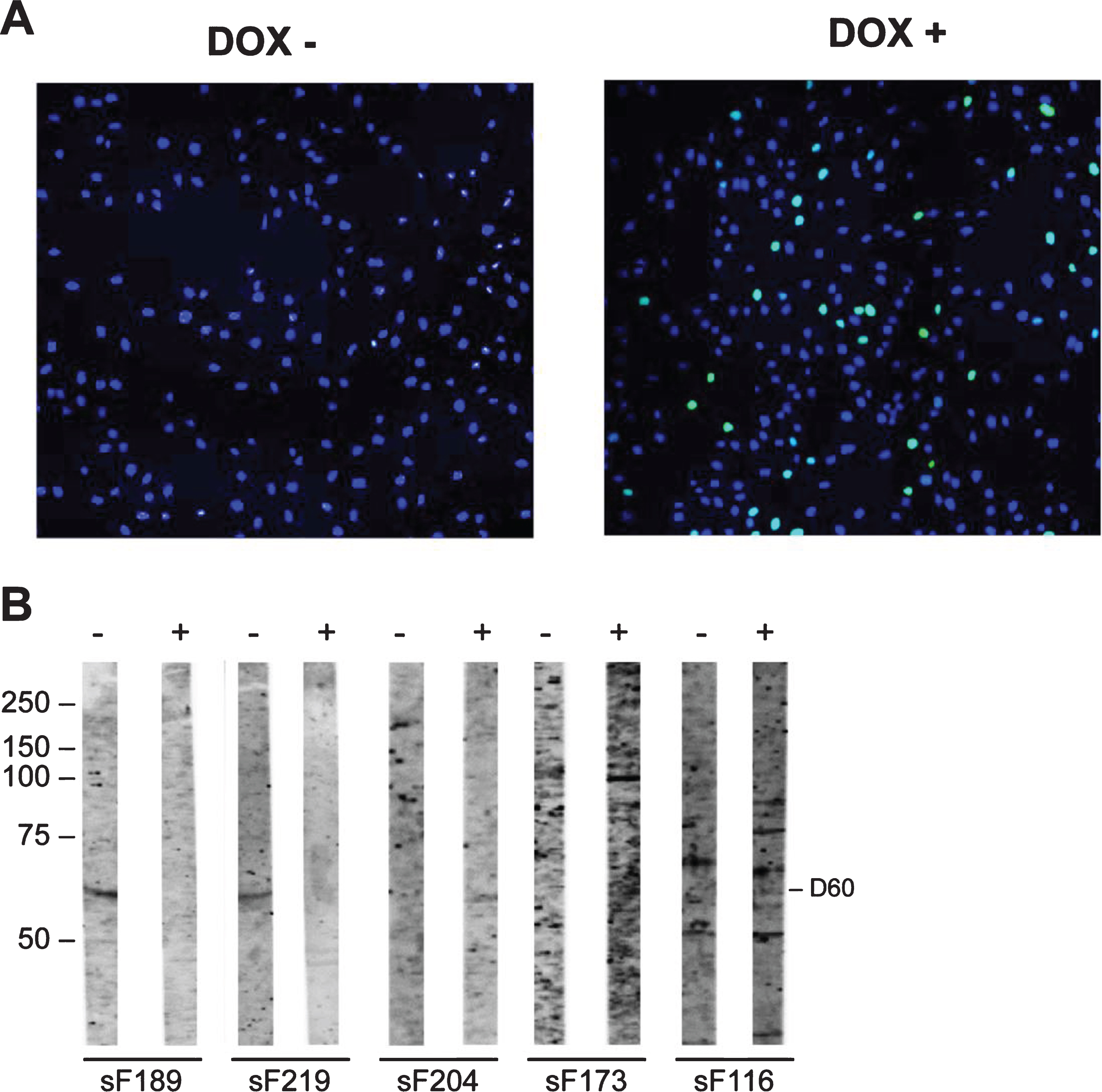
The reactivity with extracts from non-induced cells was analyzed in parallel. Reactivity with a 60 kDa band in the DUX4-deficient extract was observed in 7 FSHD sera, whereas this reactivity was not detected for the DUX4-containing extract. Conversely, 3 FSHD sera showed reactivity at 60 kDa with the DUX4-expressing myoblast extract, but not with the non-induced myoblast extract. Similarly, a 100 kDa band was detected with 2 FSHD sera only in the extract from DUX4-expressing myoblasts. Finally, a 75 kDa polypeptide was recognized by one FSHD sample in the DUX4-expressing myoblast extract and by two FSHD samples in the absence of DUX4. The frequencies of these reactivities are summarized in Table 6.
Table 6
FSHD serum reactivity against DUX4-expressing myoblasts protein extracts (D)
Band kDa | FSHD Sera % n = 86 DUX4+ | FSHD Sera % n = 86 DUX4- | HC Sera % n = 3 DUX4+ | HC Sera % n = 3 DUX4- |
D-100 | 2 (2/86) | – | – | 33 (1/3) |
D-75 | 1 (1/86) | – | – | – |
D-60 | 3 (3/86) | – | – | – |
D-30 | 2 (2/86) | – | – | – |
D-33 | 1 (1/86) | – | – | – |
D-75 | – | 2 (2/86) | – | – |
D-60 | – | 8 (7/86) | – | 33 (1/3) |
D-32 | – | 1 (1/86) | – | – |
D-28 | – | 1 (1/86) | – | – |
+ indicates a sample reactive only against the DOX+ myoblasts protein extract with DUX4 induction; - indicates a sample reactive only against the DOX- myoblasts protein extract without DUX4 induction.
Immunofluorescence analysis of FSHD sera with human DUX4-expressing immortalized myoblasts
The DUX4 inducible myoblast cell line allowed the analysis of FSHD sera by immunofluorescence and to explore the relationship between DUX4 expression and autoreactivity. Fixed cells were incubated with 85 FSHD and 14 healthy control sera (Supplementary Figure 5). DUX4 expression was visualized with an anti-DUX4 antibody, which showed that DUX4 was detectably expressed in only a subset of the nuclei, in agreement with previous observations (Fig. 6A). This phenomenon facilitated a comparison of the staining by patient autoantibodies in DUX4-expressing and non-expressing cells on the same specimen. After staining with a fluorescent secondary antibody, we observed cytoplasmic and (peri)nuclear staining in all samples (Fig. 7). However, the fluorescence intensity differed among samples and was graded as weak, medium, and strong. Besides, among and within the same samples we observed a variation in nuclear staining patterns comprising the following patterns: homogeneous, nuclear speckles, nucleolar, and peripheral. Nevertheless, staining intensity and staining pattern frequencies did not differ between patient and control sera (Fig. 7 A-B). Importantly, FSHD and healthy control sera were reactive in DUX4 expressing as well as non DUX4 expressing cells (Fig. 7 C-F) excluding in the tested cohort a DUX4 dependent relationship.
Fig. 7
Immunofluorescence analysis of the reactivity of FSHD and HC sera with DUX4-expressing myoblasts. DUX4-expressing myoblasts were fixed and incubated with either patient (N = 85) or control sera (N = 14) and with an anti-DUX4 antibody. Bound antibodies were visualized by fluorescent secondary antibodies. (A) Nuclear and cytoplasmic staining intensity frequencies scored as weak, medium, and strong. (B) (Peri)nuclear staining pattern frequencies. (C - F) White arrows indicate nuclear speckles; blue arrows indicate nucleolar staining; white arrowheads indicate homogeneous nuclear staining; blue arrowheads indicate perinuclear staining in the cytoplasm. sF = FSHD serum. sHC = healthy control serum. A representative set of samples is shown.
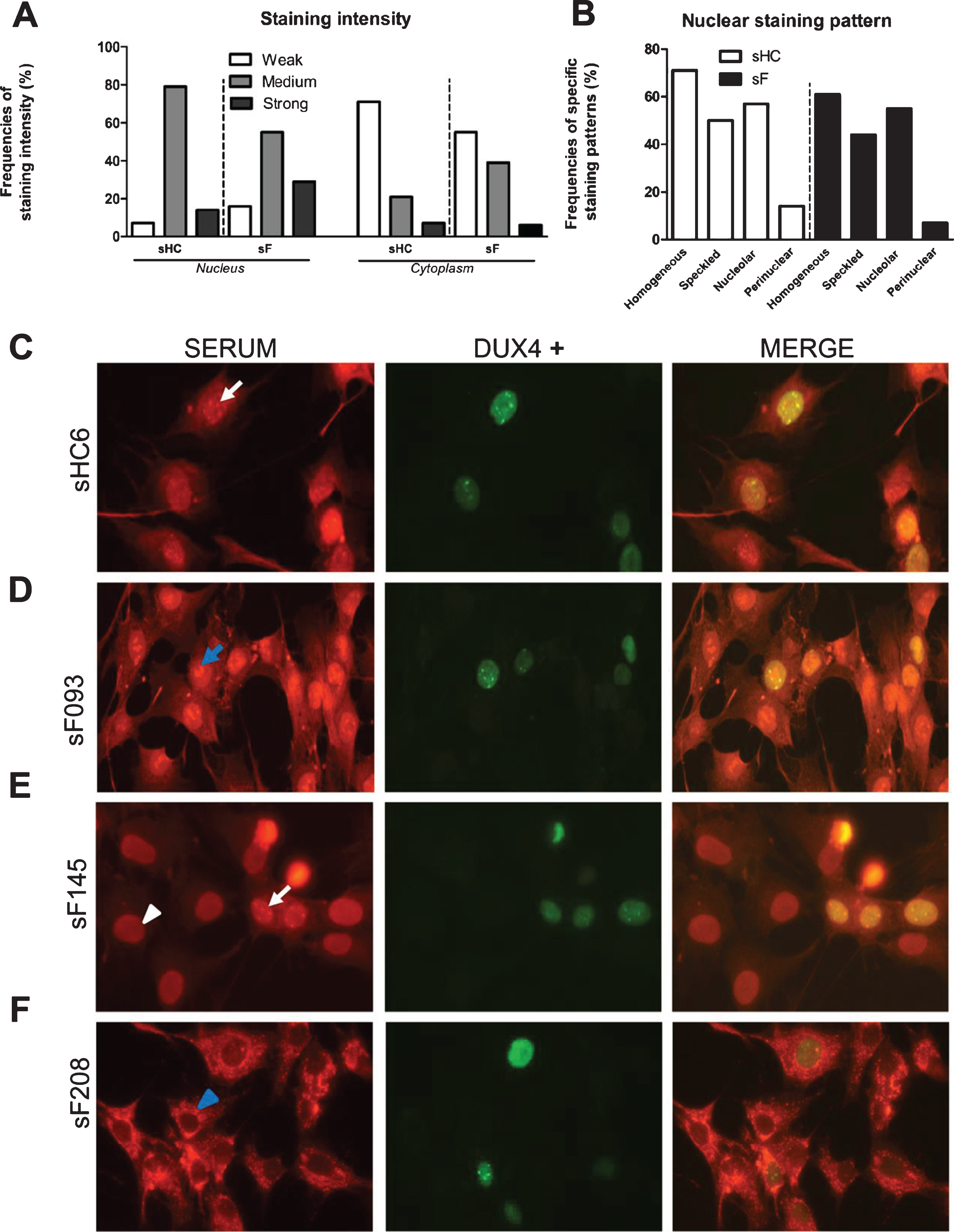
DISCUSSION
We hypothesized that DUX4-induced aberrant expression of genes in FSHD skeletal muscle cells might trigger the production of autoantibodies against muscle proteins (Fig. 1), thereby, at least in part, explaining the inflammatory component associated with the disease. To investigate this hypothesis, the presence of autoantibodies in FSHD sera was assessed with different sources of muscle antigens, following a stepwise workflow from healthy human skeletal muscle extracts to DUX4-expressing myoblast extracts (Fig. 2). Although a series of reactivities were observed, their frequency in FSHD sera was generally low and similar reactivities were also seen with healthy control sera. Moreover, we did not find a relationship between serum antibody reactivity and DUX4 expression, since both patient and healthy control sera showed similar reactivities with DUX4-expressing myoblasts, by immunoblotting as well as by immunofluorescence. Taken together, these data argue against the existence of a specific autoantibody reactivity targeting (DUX4-induced) muscle antigens.
The lack of evidence for specific FSHD autoantibodies in our cohort might suggest that muscular autoantibodies are specifically related to the pathogenesis of idiopathic inflammatory myopathies (myositis) like IBM, but are difficult to link to a neuromuscular disorder such as FSHD. Autoantibodies are indeed known to play a significant role in the pathogenesis of myositis [23]. Myositis-specific antibodies (MSAs) are commonly used for diagnosis and for follow-up of these disorders [24]. Anti-Jo-1, anti-Mi-2, and anti-signal recognition particle (SRP) are the most commonly found MSAs [25]. Autoantibodies can induce disease by different mechanisms. A well-known mechanism is the bursting of inflammation at the site of autoantibody deposition [26]. Similarly, antibodies against muscle antigens in such a complex disorder like FSHD, where multiple genetic, epigenetic, and immune pathways are known to interact [9, 27], were hypothesized to play a role in the pathogenesis of FSHD. Previously, our group tested [28] 97 sera of IIM patients and 107 sera of patients with a neuromuscular disorder, among which 21 FSHD sera, with a panel of common MSAs. MSAs appeared to be present in 25% of DM patients and 5% of PM patients, but in none of the FSHD patients or patients with another neuromuscular disorder. Similarly, Mammen et al. [29] screened 47 sera from patients with genetic muscle disorders (including 27 genetically proven FSHD sera) for the most common MSAs and compared the results with a DM patient cohort. Only one FSHD patient tested positive for the anti-Mi-2 autoantibody, a common MSA usually detected in DM. However, this FSHD patient had also a confirmed DM.
Taken together, these results indicate that MSAs might not be related to the pathogenesis of FSHD. Since the target proteins of MSAs are also present in skeletal muscle cells, our data confirm these observations in a large FSHD cohort and, in addition, demonstrate that there are no other muscle proteins that are frequently targeted by autoantibodies in FSHD patient sera.
Despite the absence of autoantibodies specifically related to FSHD, we observed several reactivities on both patient and healthy control blots. These findings may indicate the presence of (auto)antibodies naturally present in human serum, otherwise known as natural antibodies. The term natural antibodies was introduced in the early 1960 s by Boyden et al. [30] to define antibodies spontaneously produced without antigenic stimulation. Indeed, healthy human serum is well known to contain tissue autoantibodies naturally occurring against a broad variety of self-antigens, such as smooth muscle antigens and cytoskeletal antigens [31]. Natural antibodies are believed to be part of the first defense line of our immune system with a primary housekeeping function devoted to maintain homeostasis [32]. Therefore, considering the similar pattern of reactivity of patient and control sera against all the different tested sources of muscle antigens, we hypothesize the presence of naturally occurring antibodies in healthy controls as well as in FSHD patients, recognizing multiple muscle antigens without reaching pathological levels.
Ample evidence exists that DUX4 is able to induce ectopic activation of germline and cleavage stage genes in skeletal muscle cells [9, 22, 33], thereby sustaining the hypothesis of a break of tolerance with consequent autoimmune reaction. Nevertheless, our findings do not provide evidence for a DUX4-dependent induction of antibodies directed against skeletal muscle antigens. After immunofluorescence analysis of both patient and healthy control sera incubated with DUX4-expressing immortalized myoblasts, we observed four major patterns of antinuclear antibodies (ANA): homogeneous, nuclear speckles, nucleolar, and peripheral. ANA are a wide range of autoantibodies known to be associated with connective tissue diseases (CTD) [34], which include autoimmune disorders such as rheumatoid arthritis, scleroderma, and lupus[35]. Low titers of ANA are present in healthy people as well [36], which suggests that ANA could be part of the normal immune response [37]. In fact, together with the high false positive test rate of common immunofluorescent tests [38], ANA titer measurement is commonly recommended only in case of high clinical suspicion [39]. Hence, the observed ANA positivity in both patient and control sera in DUX4 expressing myonuclei as well as in non DUX4 expressing myonuclei suggest the presence of low titer antibodies, naturally reactive against nuclear antigens and, importantly, not DUX4 induced.
It is important to mention that muscle inflammation in FSHD is described as an intermittent process, affecting specific muscles at any given time [40]. The presence of active disease in FSHD patients can be radiologically characterized with MRI studies as STIR hyperintensity [41]. STIR is an MRI dedicated sequence that enables the detection of tissue edema or inflammation [42] and STIR hyperintensity is a well-known marker of active disease in FSHD patients [43]. We may speculate that low titers of circulating autoantibodies could be missed in patients with little active disease. However, our collection of tested patient sera includes a large percentage (47%) of patients with confirmed active disease in the muscles of the leg (Table 1). Since the MRI examination was restricted to the muscles of the leg, we cannot exclude the presence of active disease also in the patient subgroup reporting no STIR hyperintensity. This is a limitation of the study. Moreover, the FSHD quadriceps muscle protein extract (Fig. 4) used in this study was obtained from two vastus lateralis muscles with evidence of dystrophic changes, but without signs of active disease on MRI. This is a limitation of the study that might have contributed to the lack of evidence for specific autoantibodies to skeletal muscle components in FSHD patients.
Finally, it should be noted that the findings of this study are restricted to the screening of antibodies to muscle antigens, which does not exclude the involvement of other immune mechanisms. MRI-guided biopsies targeting STIR-positive FSHD muscles have indeed shown active inflammatory infiltrates [17], including CD8-positive T cells and macrophages, the most prominent mononuclear cells surrounding the endomysial spaces [17, 18]. Both T cells and macrophages endomysial infiltrates are not an FSHD-specific histological feature, since they are often described in IIM muscle specimens as well [44]. Macrophages are among the first immune cells recruited to the site of injury [45]. After recruitment, macrophages secrete inflammatory cytokines such as TNFα, IL-1β, and IL-6 which will orchestrate the appropriate T-cell activation [46]. However, the functional role of both macrophages and T-cells remains mainly conjectural in neuromuscular disorders such as FSHD. Therefore, based on the present study, these immune cells might be the object of investigation in further immunological studies.
Taken together, this study shows that there are no detectable differences in antibody reactivities towards muscle antigens between FSHD patient sera and healthy control sera. Importantly, these findings provide new insight in the understanding of muscle inflammation in FSHD, because our data strongly suggest that there is no major role for B cells in this process and autoantibody-mediated mechanisms may not be involved in FSHD pathophysiology. Alternatively, other immune pathways, including innate immune cell- and T-cell mediated mechanisms, might play a role and need to be investigated. Their involvement in disease development and/or progression may provide clues for novel druggable targets.
ACKNOWLEDGMENTS
This work was supported by the charitable Dutch foundation Prinses Beatrix Spierfonds, Grant number W.OR15-23 awarded to BGMvE, LABJ, and GJMP.
AUTHOR CONTRIBUTION
AG was involved in acquisition, analysis, and interpretation of data, drafting of the manuscript and figures. KRS was involved in data acquisition and analysis. KM and AvdH were involved in data acquisition and revised the manuscript. SMvdM, LABJ, and BGMvE were involved in interpretation of the data and revised the manuscript. GJMP conceived and supervised the study and revised the manuscript. All authors contributed significantly to the final version of the manuscript.
CONFLICT OF INTEREST
A. Greco, K. Straasheijm, K. Mul, A. van den Heuvel, S.M. van der Maarel, L.A.B Joosten, and G.J.M. Pruijn declared that no conflict of interest exists.
B.G.M van Engelen receives fees and non-financial support from Fulcrum. Facio, Avidity and Fondazione Telethon during the conduct of the study, and institutional support from the Radboud University Medical Centre and grant support from European Union’s Horizon 2020 research and innovation programme, European Union 7th Framework Programme (OPTIMISTIC), the Netherlands Organisation for Scientific Research (NWO), The Netherlands Organisation for Health Research and Development (ZonMw), Global FSH, Prinses Beatrix Spierfonds, Spieren voor Spieren, Association Francaise contre les Myopathies, and the Dutch FSHD Foundation.
SUPPLEMENTARY MATERIAL
[1] The supplementary material is available in the electronic version of this article: https://dx.doi.org/10.3233/JND-210653.
REFERENCES
[1] | Deenen JC , Arnts H , van der Maarel SM , Padberg GW , Verschuuren JJ , Bakker E , et al. Population-based incidence and prevalence of facioscapulohumeral dystrophy. Neurology. (2014) ;83: :1056–9. |
[2] | Tawil R , Kissel JT , Heatwole C , Pandya S , Gronseth G , Benatar M , et al. Evidence-based guideline summary: Evaluation, diagnosis, and management of facioscapulohumeral muscular dystrophy: Report of the Guideline Development, Dissemination, and Implementation Subcommittee of the American Academy of Neurology and the Practice Issues Review Panel of the American Association of Neuromuscular & Electrodiagnostic Medicine. Neurology. (2015) ;85: :357–64. |
[3] | Wijmenga C , Frants RR , Brouwer OF , Moerer P , Weber JL , Padberg GW . Location of facioscapulohumeral muscular dystrophy gene on chromosome 4. Lancet (London, England). (1990) ;336: :651–3. |
[4] | Tawil R , van Der Maarel SM , Tapscott S . Facioscapulohumeral dystrophy: The path to consensus on pathophysiology. Skeletal Muscle. (2014) ;4: :1–12. |
[5] | van Deutekom JC , Wijmenga C , van Tienhoven EA , Gruter AM , Hewitt JE , Padberg GW , et al. FSHD associated DNA rearrangements are due to deletions of integral copies of a 3.2 kb tandemly repeated unit. Human Molecular Genetics. (1993) ;2: :2037–42. |
[6] | van den Boogaard ML , Lemmers R , Balog J , Wohlgemuth M , Auranen M , Mitsuhashi S , et al. Mutations in DNMT3B Modify Epigenetic Repression of the D4Z4 Repeat and the Penetrance of Facioscapulohumeral Dystrophy. Am J Hum Genet. (2016) ;98: :1020–9. |
[7] | Daxinger L , Tapscott SJ , van der Maarel SM . Genetic and epigenetic contributors to FSHD. Curr Opin Genet Dev. (2015) ;33: :56–61. |
[8] | van der Maarel SM , Miller DG , Tawil R , Filippova GN , Tapscott SJ . Facioscapulohumeral muscular dystrophy: Consequences of chromatin relaxation. Current Opinion in Neurology. (2012) ;25: :614–20. |
[9] | Geng LN , Yao Z , Snider L , Fong AP , Cech JN , Young JM , et al. DUX4 activates germline genes, retroelements, and immune mediators: Implications for facioscapulohumeral dystrophy. Developmental Cell. (2012) ;22: :38–51. |
[10] | Jagannathan S , Ogata Y , Gafken PR , Tapscott SJ , Bradley RK . Quantitative proteomics reveals key roles for posttranscriptional gene regulation in the molecular pathology of facioscapulohumeral muscular dystrophy. Elife. 2019;8. |
[11] | da Silva V , Fonseca A , Fonseca M , da Silva T , Coelho A , Kroll J , et al. Genome-wide identification of cancer/testis genes and their association with prognosis in a pan-cancer analysis. Oncotarget. (2017) ;8: :92966–77. |
[12] | Al-Khadairi G , Decock J . Cancer Testis Antigens and Immunotherapy: Where Do We Stand in the Targeting of PRAME? Cancers (Basel). 2019;11. |
[13] | Pluk H , van Hoeve BJ , van Dooren SH , Stammen-Vogelzangs J , van der Heijden A , Schelhaas HJ , et al. Autoantibodies to cytosolic 5’-nucleotidase 1A in inclusion body myositis. Annals of Neurology. (2013) ;73: :397–407. |
[14] | Wulff JD , Lin JT , Kepes JJ . Inflammatory facioscapulohumeral muscular dystrophy and coats syndrome. Annals of Neurology. (1982) ;12: :398–401. |
[15] | Munsat TL , Piper D , Cancilla P , Mednick J . Inflammatory myopathy with facioscapulohumeral distribution. Neurology. (1972) ;22: :335–335. |
[16] | Hengstman GJD , van Engelen BGM , van Venrooij WJ . Myositis specific autoantibodies: Changing insights in pathophysiology and clinical associations. Curr Opin Rheumatol. (2004) ;16: :692–9. |
[17] | Frisullo G , Frusciante R , Nociti V , Tasca G , Renna R , Iorio R , et al. CD8(+) T cells in facioscapulohumeral muscular dystrophy patients with inflammatory features at muscle MRI. J Clin Immunol. (2011) ;31: :155–66. |
[18] | Statland JM , Odrzywolski KJ , Shah B , Henderson D , Fricke AF , van der Maarel SM , et al. Immunohistochemical Characterization of Facioscapulohumeral Muscular Dystrophy Muscle Biopsies. Journal of Neuromuscular Diseases. (2015) ;2: :291–299. |
[19] | Ricci E , Galluzzi G , Deidda G , Cacurri S , Colantoni L , Merico B , et al. Progress in the molecular diagnosis of facioscapulohumeral muscular dystrophy and correlation between the number of KpnI repeats at the 4q35 locus and clinical phenotype. Annals of Neurology. (1999) ;45: :751–7. |
[20] | Lamperti C , Fabbri G , Vercelli L , D’Amico R , Frusciante R , Bonifazi E , et al. A standardized clinical evaluation of patients affected by facioscapulohumeral muscular dystrophy: The FSHD clinical score. Muscle Nerve.. (2010) ;42: :213–7. |
[21] | Mul K , Vincenten S , Voermans N , Lemmers R , van der Vliet P , van der Maarel S , et al. Adding quantitative muscle MRI to the FSHD clinical trial toolbox. Neurology.. (2013) ;14: :2057–65. |
[22] | Jagannathan S , Shadle SC , Resnick R , Snider L , Tawil RN , van der Maarel SM , et al. Model systems of DUX4 expression recapitulate the transcriptional profile of FSHD cells. Human Molecular Genetics. (2016) ;25: :4419–31. |
[23] | Casciola-Rosen L , Nagaraju K , Plotz P , Wang K , Levine S , Gabrielson E , et al. Enhanced autoantigen expression in regenerating muscle cells in idiopathic inflammatory myopathy. J Exp Med. (2005) ;201: :591–601. |
[24] | Satoh M , Tanaka S , Ceribelli A , Calise SJ , Chan EK . A Comprehensive Overview on Myositis-Specific Antibodies: New and Old Biomarkers in Idiopathic Inflammatory Myopathy. Clin Rev Allergy Immunol. (2017) ;52: :1–19. |
[25] | Gunawardena H , Betteridge ZE , McHugh NJ . Myositis-specific autoantibodies: Their clinical and pathogenic significance in disease expression. Rheumatology (Oxford). (2009) ;48: :607–12. |
[26] | Fujio K , Okamura T , Sumitomo S , Yamamoto K . Regulatory T cell-mediated control of autoantibody-induced inflammation. Front Immunol. (2012) ;3: :28. |
[27] | Greco A , Goossens R , van Engelen B , van der Maarel SM . Consequences of epigenetic derepression in facioscapulohumeral muscular dystrophy. Clinical genetics. 2020. |
[28] | Hengstman GJ , van Brenk L , Vree Egberts WT , van der Kooi EL , Borm GF , Padberg GW , et al. High specificity of myositis specific autoantibodies for myositis compared with other neuromuscular disorders. J Neurol. (2005) ;252: :534–7. |
[29] | Mammen AL , Casciola-Rosen L , Christopher-Stine L , Lloyd TE , Wagner KR . Myositis-specific autoantibodies are specific for myositis compared to genetic muscle disease. Neurol Neuroimmunol Neuroinflamm. (2015) ;2: :e172. |
[30] | Boyden SV . Natural Antibodies and the Immune Response. 1966:1-28. |
[31] | Lutz H , Wipf G . Naturally occurring autoantibodies to skeletal proteins from human red blood cells. J Immunol. (1982) ;128: :1695–9. |
[32] | Palma J , Tokarz-Deptula B , Deptula J , Deptula W . Natural antibodies - facts known and unknown. Cent Eur J Immunol. (2018) ;43: :466–475. |
[33] | Knopp P , Krom YD , Banerji CR , Panamarova M , Moyle LA , den Hamer B , et al. DUX4 induces a transcriptome more characteristic of a less-differentiated cell state and inhibits myogenesis. J Cell Sci. (2016) ;129: :3816–31. |
[34] | Agmon-Levin N , Damoiseaux J , Kallenberg C , Sack U , Witte T , Herold M , et al. International recommendations for the assessment of autoantibodies to cellular antigens referred to as anti-nuclear antibodies. Ann Rheum Dis. (2014) ;73: :17–23. |
[35] | Reddy BY , Hantash BM . Cutaneous Connective Tissue Diseases: Epidemiology, Diagnosis, and Treatment. Open Dermatol J. (2009) ;3: :22–31. |
[36] | Marin GG , Cardiel MH , Cornejo H , Viveros ME . Prevalence of antinuclear antibodies in 3 groups of healthy individuals: Blood donors, hospital personnel, and relatives of patients with autoimmune diseases. J Clin Rheumatol.. (2009) ;15: :325–9. |
[37] | Grygiel-Górniak B , Rogacka N , Rogacki M , Puszczewicz M . Antinuclear antibodies in autoimmune and allergic diseases. Reumatologia. (2017) ;55: :298–304. |
[38] | O’Sullivan M , McLean-Tooke A , Loh RK . Antinuclear antibody test. Aust Fam Physician. (2013) ;42: :718–21. |
[39] | Habash-Bseiso DE , Yale SH , Glurich I , Goldberg JW . Serologic testing in connective tissue diseases. Clin Med Res. (2005) ;3: :190–3. |
[40] | Haynes P , Bomsztyk K , Miller DG . Sporadic DUX4 expression in FSHD myocytes is associated with incomplete repression by the PRC2 complex and gain of H3K9 acetylation on the contracted D4Z4 allele. Epigenetics Chromatin. (2018) ;11: :47. |
[41] | Leung DG . Magnetic resonance imaging in facioscapulohumeral muscular dystrophy. Muscle Nerve. (2018) ;57: :872–4. |
[42] | Friedman SD , Poliachik SL , Otto RK , Carter GT , Budech CB , Bird TD , et al. Longitudinal features of STIR bright signal in FSHD. Muscle Nerve. (2014) ;49: :257–60. |
[43] | Wang LH , Friedman SD , Shaw D , Snider L , Wong CJ , Budech CB , et al. MRI-informed muscle biopsies correlate MRI with pathology and DUX4 target gene expression in FSHD. Human Molecular Genetics. (2019) ;28: :476–86. |
[44] | Tripoli A , Marasco E , Cometi L , De Stefano L , Marcucci E , Furini F , et al. One year in review Idiopathic inflammatory myopathies. Clin Exp Rheumatol. (2020) ;38: :1–10. |
[45] | Arnold L , Henry A , Poron F , Baba-Amer Y , van Rooijen N , Plonquet A , et al. Inflammatory monocytes recruited after skeletal muscle injury switch into antiinflammatory macrophages to support myogenesis. J Exp Med. (2007) ;204: :1057–69. |
[46] | Olazabal IM , Martin-Cofreces NB , Mittelbrunn M , Martinez del Hoyo G , Alarcon B , Sanchez-Madrid F . Activation outcomes induced in naive CD8 T-cells by macrophages primed via “phagocytic” and nonphagocytic pathways. Mol Biol Cell. (2008) ;19: :701–10. |