Night Activity Reduction is a Signature Physiological Biomarker for Duchenne Muscular Dystrophy Dogs
Abstract
Background:
Duchenne muscular dystrophy (DMD) is an X-linked lethal muscle disease. Dystrophic dogs are excellent models to test novel therapies for DMD. However, the use of the dog model has been hindered by the lack of an effective method to evaluate whole-body mobility. We recently showed that night activity is a good indicator of dog mobility. However, our published method relies on frame-by-frame manual processing of a 12-hour video for each dog. This labor-intensive and time-consuming approach makes it unrealistic to use this assay as a routine outcome measurement.
Objective:
To solve this problem, we developed an automatic video-capturing/imaging processing system. The new system reduces the data analysis time over 1,000 fold and also provides a more detailed activity profile of the dog.
Methods:
Using the new system, we analyzed more than120 twelve-hour recordings from 12 normal and 22 affected dogs.
Results:
We observed similar activity profiles during repeated recording of the same dog. Throughout the night, normal dogs were in motion 10.4 ± 0.9% of the time while affected dogs were in motion 4.6 ± 0.2% of the time (p < 0.0001). Further, normal dogs made significantly more movements (p < 0.0001) while affected dogs rested significantly longer (p < 0.0001) during the period of recording (from 6 pm to 6 am next day). Importantly, statistical significance persisted irrespective of the coat color, gender and mutation type.
Conclusions:
Our results suggest that night activity reduction is a robust, quantitative physiological biomarker for dystrophic dogs. The new system may be applicable to study mobility in other species.
INTRODUCTION
Duchenne muscular dystrophy (DMD) is a devastating muscle-wasting disease that affects boys and young men and leads to premature death [1]. DMD is caused by the loss of a subsarcolemmal cytoskeletal protein called dystrophin. Dystrophin is an essential component of the dystrophin-associated glycoprotein (DGC) complex that preserves the integrity of the muscle cell membrane. In the absence of dystrophin, the cell membrane becomes fragile and cannot sustain shearing stress generated during muscle contraction. This results in membrane damage and myofiber degeneration. Eventually the dead muscle cells are replaced by connective tissue and affected individuals lose their mobility.
Current treatments for DMD are limited to corticosteroids and symptom management [2]. Tremendous progress has been made over the last decade in the development of novel pharmacologic and genetic therapies for DMD [3–5]. Most of these experimental treatments show promising efficacy in dystrophin-null mdx mice. However, mouse results often translate poorly to DMD patients due to the lack of the dystrophic phenotype in mdx mice, differences in immune responses between mice and humans, and/or the failure to scale-up from mice to large mammals [6]. Dystrophin-deficient dogs show characteristic symptoms of muscular dystrophy and dogs also have the scale-up advantage [7]. Results from studies performed in the canine DMD model may better inform the design of human trials.
Dystrophin deficiency has been described in more than 20 different dog breeds. Experimental DMD dog colonies have also been established in many places around the world [7]. However, there are limited tools to study muscle function in dogs. We recently developed an in situ protocol to evaluate contractility of a single dog muscle [8]. Kornegay and colleagues reported an assay for measuring hindlimb force in dogs [9]. Yet, there is no method to objectively study whole body mobility in dogs. To address this issue, we recently tested the hypothesis that spontaneous movement at night can serve as a physiological biomarker to objectively evaluate dog mobility. As a pilot study, we recorded night activity in a litter of three affected and four normal dogs [10]. We then converted the video to images (one frame every three seconds) and determined dog movement by manually quantifying differences between neighboring frames. This pilot study, though labor-intensive and time-consuming, has provided the critical preliminary data supporting our hypothesis that night activity is a useful endpoint for studying dog mobility.
Several questions were not answered in our pilot study. Specifically, it was not clear whether reproducible data could be obtained from the same dog on different days, whether dogs of different kindreds could yield consistent results, and whether reduced activity is a common finding in all dystrophic dogs irrespective of the gender and genotype type. The pilot study also had several inherent constraints such as the small sample size, cumbersome method and limitedoutput parameters. To address all these issues, we developed a computer-based system that can automatically record and analyze spontaneous movements at night in canines. The new system allows quantification of dog mobility using a comprehensive set of metrics. The results from the new system confirm and expand our initial observation [10]. Together these data suggest that night activity is a robust whole body mobility biomarker for dogs. The new system will greatly facilitate ongoing and future translational studies in the canine DMD model [11].
MATERIALS AND METHODS
Animals
Animal housing and experiments were approved by the Animal Care and Use Committee of the University of Missouri and were performed in accordance with guidelines of the National Institutes of Health and Department of Defense. All experimental dogs were on a mixed genetic background of golden retriever, Labrador retriever, beagle, and Welsh corgi. There are three different types of dystrophin gene mutations in affected dogs including type G (intron 6 point mutation identical to that of golden retriever muscular dystrophy dogs), type L (intron 19 insertion identical to that of Labrador retriever muscular dystrophy dogs) and type W (intron 13 insertion identical to that of Welsh corgi muscular dystrophy dogs) [12–15]. Experimental dogs were generated by in house artificial insemination. Specifically, fresh or frozen semen from an affected dog (genotype YG, YL, YW) were injected into the uterus of a carrier (genotype XG, XL, XW) twice at 48 h and 72 h post-ovulation. The dog coat color was determined according to Wikipedia (https://en.wikipedia.org/wiki/Coat_% 28dog% 29). The genotype was determined by polymerase chain reaction as we previously described [12, 13]. The diagnosis was further confirmed by the significantly elevated serum creatine kinase (CK) level in affected dogs. Night activity in 12 normal male (mean age, 14.8 ± 1.9 months; range, 8.0 to 22.9 months), 11 affected male (mean age, 18.2 ± 1.3 months; range, 9.7 to 23.3 months) and 11 affected female (mean age, 12.6 ± 1.8 months; range, 6.8 to 19.9 months) dogs were recorded and analyzed. These dogs were from 27 different litters. All experimental dogs were housed in specific-pathogen free animal care facilities and kept under a 12-hour light/12-hour dark cycle.
Video recording equipment
A low-light charge-coupled device (CCD) camera (PC164C, Supercircuits Inc., Austin, TX) was used for overnight video recording. The video camera was equipped with an infrared lens (Part #12VM412ASIR, Tamron, Commack, NY) and a near infrared (NIR) light source (Part # IR045, Clover electronics USA, Cerritos, CA).
Video recording conditions
The dog was housed individually in a kennel (181 cm×122 cm) with an elevated floor (Fig. 1A). The video camera was centered and secured to the ceiling on top of the kennel through a custom-made mounting bracket. Rubber dampers were added to the bracket to isolate any vibration from the ceiling to the camera. The camera and light source were positioned at 210 cm above the kennel floor to ensure a full view and even illumination of the cage floor (Fig. 1A). Spontaneous movement of the dog was recorded during the dark cycle from 6 pm to 6 am next day. During this period, there was no interference from environment cues or animal caregivers. Temperature and humidity were controlled and monitored during the recording period. The dog had free accessed to water but food was removed from the kennel.
Video recording
The CCD camera was controlled by the Overnight Dog Recording software, a program developed in Labview (National Instrument Inc., Austin, TX) (Fig. 1A). The program automatically started the recording system at 5:30 pm to warm up the hardware. Each session of recording was started at 6 pm and continued for 12 hrs. The frame rate was set to 3 frames per second. The recorded video was streamed to the hard drive of the control computer. The motion signal S(t) was defined as the signal difference between every two consecutive images. It was computed according to the following formula
(1)
Processing of the recorded video
The recorded S(t) was analyzed by the Overnight Dog-Video Analyzer, a software program developed in Matlab (Mathworks Inc., Natick, MA) (Fig. 1A). Ideally, the baseline should be zero when the dog is not moving. However the recorded baseline always fluctuated and drifted with time due to various noises from the video recording system and environment (such as air ventilation, building vibration and thermal noise of the electronics). To correct the baseline drift, the signal baseline was extracted by removing all motion-induced signal peaks from the raw signal. The missing baseline segments corresponding to the removed motion signals were reconstructed using a piecewise cubic interpolation algorithm. The resulting baseline was further smoothed by applying a 7th order Savitzky-Golay filter followed by a median filter, both with a window size of 1.1 sec (33 data points). This reconstructed baseline was then subtracted from the raw signal to create a flat baseline. Finally, a motion threshold was applied to remove noisy spikes that were not considered as true motion signals (Fig. 1B). Signals that are above this threshold were considered as motion spikes.
Definition of the movement and rest
The processed motion signals showed three types of motion spike(s) including a single motion spike, a stretch of continuous non-interrupted spikes and a stretch of spikes with ≤10 sec interruptions between neighbouring spikes (Fig. 1C). The 10 sec value was chosen based on a pilot study. Each identifiable type of spike (or spikes) was defined as a single movement. The interval between two consecutive movements was defined as rest. By definition, a rest is always longer than 10 sec.
Motion quantification metrics
A series of metrics were used to comprehensively evaluate dog night activity. These included the percentage of time in motion (% ; the percentage of the time the dog was in motion during the entire 12-hour recording period), amplitude of the movement (arbitrary unit; relative signal strength of a motion spike), number of movement per hour (Nm/hr), average duration of a single movement (sec) and average duration of a single rest (sec). Based on the duration, a movement wasfurther divided into short-duration movement (≤10s) and long-duration movement (>10s). To gain more detailed understanding on the movement, we further quantify the frequency of short and long-duration movements per hour and expressed these parameters as “number of short movement/hour” and “number of long movement/hour”, respectively.
Statistical analysis
Data are presented as mean ± standard error of mean. Statistical difference was assessed with the Student’s t-test followed by Bonferroni correction to correct familywise error caused by multiple two-group comparisons. The p value is presented in the figure legend.
RESULTS
Night activity analyzer is a robust system to evaluate dog mobility
To objectively evaluate dog mobility, we intentionally performed recording at night when there was neither human nor environment interference. To eliminate potential influences from changes in temperature and humidity, we also maintained a constant temperature (23.8 ± 0.2°C) and humidity (40.2 ± 1.5% ) in the kennel.
To determine whether our newly developed software can consistently record and analyze dog activity, we performed repeated recording. Specifically, the same dog was recorded multiple times during an 11-day period (2 to 10 times, either daily or in different days). The percentage of time in motion was quantified for each recording and compared with the first night recording. Consistent activity was observed for both normal and affected dogs during repeated recording (Fig. 2). For normal dogs, the difference (compared with the result of the first night recording) was ≤28% when the same dog was recorded at different nights. For affected dogs, the difference (compared with the result of the first night recording) was ≤18% when the same dog was recorded at different nights.
Dystrophin deficiency significantly reduced night activity in affected dogs
To determine whether night activity can serve as a robust physiological biomarker for DMD dogs, we initiated a comprehensive study with a large cohort of crossbred dogs. These included 12 male normal dogs (age 14.7 ± 1.9 months) and 22 mixed-gender affected dogs (age 15.4 ± 1.24 months). They came from 27 different litters. Figure 3A showed representative processed 12-hr recordings from a normal and an affected dog. Throughout the night, normal dogs were in motion 10.4 ± 0.9% of the time while affected dogs were in motion only 4.6 ± 0.2% of the time (p < 0.00001) (Fig. 3B). In other words, normal dogs moved approximately 75 min and affected dogs moved approximately 33 min during 12-hr recording. On average, normal dogs moved 28.3 ± 1.3 times per hour while affected dogs only moved 14.1 ± 0.4 times per hour (p < 0.00001) (Fig. 3C). Additional analysis showed that normal dogs not only made significantly more short movements but also made significantly more long movements (p < 0.00001) (Fig. 3D and E). Interestingly, the average amplitude of movement was similar between normal (0.60 ± 0.08) and affected (0.56 ± 0.04) dogs (p = 0.68) (Fig. 3F). Further, there was no significant difference in the average duration of movement between normal and affected dogs (Fig. 3G). In contrast to the duration of movement, the average duration of rest in affected dogs (243.1 ± 0.6 sec) was significantly longer than that of normal dogs (111.5 ± 4.5 sec) (p < 0.00001) (Fig. 3H).
As a consequence of crossbreeding, our study dogs displayed different coat colors which produced different pixel intensities in the video. To determine whether the coat color influenced the outcome, we grouped normal and affected dogs based on their color. Affected dogs consistently showed reduced activity irrespective of the coat color (Supplementary Figure 1).
Gender had minimal impact on night activity
The normal group consisted of male dogs only while the affected group included both male and female dogs. To determine whether gender had confounded our initial analysis (Fig. 3), we removed female dogs from the affected group and re-analyzed the data (Fig. 4). Impressively, normal male and affected male comparison resulted in a pattern nearly identical to that of the initial analysis (Figs. 3 and 4).
To further characterize the potential influence of the gender, we compared results of male affected and female affected dogs (Fig. 5). Affected male dogs showed slightly more movement than affected female dogs (the percentage of time in motion was 5.1 ± 0.4% and 4.1 ± 0.3% for affected male and female, respectively; p = 0.03) (Fig. 5A). This appeared to have resulted from a slightly longer movement time in males (the average duration of a single movement was 17.1 ± 1.4 sec and 13.8 ± 0.8 sec for affected male and affected female, respectively; p = 0.05). No difference was noticed in other parameters between affected male dogs and affected female dogs.
Affected dogs showed reduced night activity irrespective of the mutation type
There are three different types of dystrophin gene mutations in our affected dogs including type G, L and W [13–15]. In our study, all affected males carried type G mutation in their X-chromosome. However, all affected females carried two different types of dystrophin gene mutations, one on each chromosome. Comparison between male and female affected dogs (Fig. 4) suggests that the type of gene mutation had minimal impact on night activity. To confirm this observation, we performed detailed analysis in female affected dogs. Among 11 affected females, six had the genotype of LW and four had GW. No difference was seen in any metrics between LW and GW affected dogs (Fig. 6).
DISCUSSION
In this study, we developed a robust automatic video capturing/processing system to quantify dog mobility at night. A large cohort of crossbred normal and DMD dogs were evaluated using this system. We found that normal dogs had significantly more movements and shorter rests. In contrast, affected dogs displayed a significantly reduced night activity irrespective of the coat color, gender and type of mutations in the dystrophin gene.
Dystrophin-deficient dogs have been considered an excellent large animal model for DMD because of their striking clinical and pathological similarity to human patients. However, the research use of affected dogs has been limited by the lack of physiological assays that can reliably quantify muscle function. To address this issue, investigators have begun to adopt in situ protocols that are commonly used in rodent studies [8, 9, 16, 17]. While these methods allow detailed characterization of the contractile profile of a single muscle or a group of muscles, there are important limitations. First, these assays require the subject be anesthetized. Unfortunately, DMD patients and affected dogs are especially vulnerable to anesthesia-associated risks such as sudden cardiac arrest and rhabdomyolysis [18–21]. Second, these assays are often limited to limb muscle and cannot provide information on whole body mobility. Third, some of these assays (such as single muscle in situ force measurement) are terminal assays and can only be performed once in a subject before euthanization [8]. Non-invasive goniometry and accelerometry were developed recently to evaluate the range of motion of a joint and the gait pattern [10, 22–25]. These non-invasive assays had minimum risks and they can also be performed repeatedly in the same dog to monitor disease progression or responses to experimental therapy. However, the results obtained from kinematic studies could be influenced by training, environment, experiment conditions (such as the use of treats) and people involved in the study (caregivers and investigators). These interferences may introduce subjective bias. A truly objective assay would require evaluating dog mobility in its natural status (without the need of dog training and without interference from the environment and people). We reasoned that monitoring dog activity at night might accomplish this goal. We hypothesized that muscle degeneration and inflammation should compromise night movement in affected dogs. To test this hypothesis, we conducted in a pilot study and quantified night video recordings frame-by-frame manually [10]. To minimize the labor and the potential influence of the genetic background and age, we only evaluated three affected and four normal dogs from the same litter. Despite the small sample size, we found that the relative movement of affected dogs was significantly reduced [10].
To confirm this preliminary finding, we decided to expand the study to a large cohort of adult dogs from different litters. In preparation for this large sample-size study and also to facilitate easy application of the protocol by other investigators in the future, we streamlined the video recording and analysis procedures. Instead of manual recording and analysis, we developed the Overnight Dog Recording software and the Overnight Dog-Video Analyzer to control the entire process of video recording and to perform subsequent video quantification (Fig. 1). These software programs greatly reduce the labor. Further, they yield a set of metrics for comprehensive analysis. We can now not only quantify overall movement (percent of time in motion and number of movements) during the entire 12-hour recording period but also perform a detailed analysis of each individual movement (such as the type, amplitude and duration of the movement). A robust reliable protocol should yield consistent results on repeated measurements. We tested the same dog at different days (Fig. 2). Despite some changes (especially for the normal dog), overall there were minimal day-to-day variations suggesting our new system is highly reliable.
The ultimate goal of our study is to determine whether the muscle health of the dog correlates with night activity. We studied 34 dogs from 27 litters of different kindreds. As expected for diurnal animals, dogs were in sleep (without movement) most part of the night (Fig. 3A and B). Consistent with the data of the pilot study [10], night mobility of affected dogs was significantly reduced (Fig. 3). This is reflected in the total amount of time in motion, number of movements and duration of the rest. Overall, the percent of time in motion and number of movements was reduced by 50% while the duration of the rest was doubled in affected dogs. Surprisingly, the amplitude and the duration of the movements were not altered in affected dogs. The exact reasons for these observations are not clear but they may relate to neuronal control of sleep (hence cannot be influenced by muscle health). Alternatively, they may reflect inherent limitations of the software. For example, the current version of the software cannot discriminate a small-scale fast movement (such as the rapid wriggling of the dog tail) and a large-scale slow movement (such as when a dog moves its entire body slowly over a short distance).
DMD is a worldwide disease. It affects all human races. While the vast majority of DMD patients are male, female could also be affected if the dystrophin gene in both X-chromosomes is mutated or when random X-chromosome inactivation is skewed in a carrier [26–28]. Further, more than 7,000 different mutations have been reported in the dystrophin gene [29]. A robust assay for DMD should differentiate normal versus affected irrespective of their skin color, gender and the type of mutation the patient has. To this end, we analyzed on the impact of the coat color, sex and gene mutation (Figs. 4 to 6, Supplementary Figure 1). None of these factors significantly changed the outcome.
There are also several limitations. First, the loss of dystrophin also affects cognitive functions. It is possible that the observed differences may reflect a combined effect of dystrophin deficiency in muscle as well as in the central nerve system. Second, the muscle groups involved in different types of movement (such as short movement versus long movement, single spike versus continuous spikes) remain to be defined. Third, the results are obtained from a single colony. Additional studies are needed to see if similar findings exist in other dystrophic dog colonies. Fourth, we observed statistically significant differences between normal and affected dogs. However, our study did not establish the overnight activity as a valid surrogate end point. Future studies are needed to determine if a therapy that increases muscle force also results in improvement in overnight activity.
In summary, our results suggest that night activity reduction is a signature functional biomarker for DMD dogs. The newly developed video system is an easy-to-use, cost-effective and highly reliable platform to objectively study dog (and potentially other species) mobility. The night activity monitoring should be included in future studies to help assess disease progression and therapeutic intervention in the canine DMD model.
COMPETING FINANCIAL INTERESTS
D.D. is a member of the scientific advisory board for Solid GT, LLC, a venture company founded to advance gene therapy for DMD.
ACKNOWLEDGMENTS
This work was supported by grants (to D.D.) from National Institutes of Health NS-90634, Department of Defense MD130014, Jesse’s Journey-The Foundation for Cell and Gene Therapy, and Hope for Javier. Authors thank Duan lab members for the help of caring for affected dogs.
Appendices
The supplementary figure is available in the electronic version of this article: http://dx.doi.org/10.3233/JND-150114.
REFERENCES
1 | O’Brien KF, Kunkel LM (2001) Dystrophin and muscular dystrophy: Past, present, and future Mol Genet Metab 74: 1-2 75 88 |
2 | Bushby K, Finkel R, Birnkrant DJ, Case LE, Clemens PR, Cripe L (2010) Diagnosis and management of Duchenne muscular dystrophy, part 1: Diagnosis, and pharmacological and psychosocial management Lancet Neurol 9: 1 77 93 |
3 | Rodino-Klapac LR, Mendell JR, Sahenk Z (2013) Update on the treatment of Duchenne muscular dystrophy Curr Neurol Neurosci Rep 13: 3 332 |
4 | Leung DG, Wagner KR (2013) Therapeutic advances in muscular dystrophy Ann Neurol 74: 3 404 411 |
5 | Verhaart IE, Aartsma-Rus A (2012) Gene therapy for Duchenne muscular dystrophy Curr Opin Neurol 25: 5 588 596 |
6 | Duan D (2011) Duchenne muscular dystrophy gene therapy: Lost in translation? Res Rep Bio 2: 31 42 |
7 | McGreevy JW, Hakim CH, McIntosh MA, Duan D (2015) Animal models of Duchenne muscular dystrophy: From basic mechanisms to gene therapy Dis Model Mech 8: 3 195 213 |
8 | Yang HT, Shin JH, Hakim CH, Pan X, Terjung RL, Duan D (2012) Dystrophin deficiency compromises force production of the extensor carpi ulnaris muscle in the canine model of Duchenne muscular dystrophy PLoS One 7: 9 e44438 |
9 | Childers MK, Grange RW, Kornegay JN (2011) In vivo Canine muscle function assay J Vis Exp 50 pii 2623 |
10 | Shin JH, Greer B, Hakim CH, Zhou Z, Chung YC, Duan Y (2013) Quantitative phenotyping of Duchenne muscular dystrophy dogs by comprehensive gait analysis and overnight activity monitoring PLoS One 8: 3 e59875 |
11 | Duan D (2015) Duchenne muscular dystrophy gene therapy in the canine model Hum Gene Ther Clin Dev 26: 1 57 69 |
12 | Fine DM, Shin JH, Yue Y, Volkmann D, Leach SB, Smith BF (2011) Age-matched comparison reveals early electrocardiography and echocardiography changes in dystrophin-deficient dogs Neuromuscul Disord 21: 7 453 461 |
13 | Smith BF, Yue Y, Woods PR, Kornegay JN, Shin JH, Williams RR (2011) An intronic LINE-1 element insertion in the dystrophin gene aborts dystrophin expression and results in Duchenne-like muscular dystrophy in the corgi breed Lab Invest 91: 2 216 231 |
14 | Sharp NJ, Kornegay JN, Van Camp SD, Herbstreith MH, Secore SL, Kettle S (1992) An error in dystrophin mRNA processing in golden retriever muscular dystrophy, an animal homologue of Duchenne muscular dystrophy Genomics 13: 1 115 121 |
15 | Smith BF, Wrighten R (2010) Animal models for inherited muscle diseases Duan D Muscle gene therapy 1 21 New York Springer Science + Business Media, LLC |
16 | Hakim CH, Wasala NB, Duan D (2013) Evaluation of muscle function of the extensor digitorum longus muscle ex vivo and tibialis anterior muscle in situ in mice J Vis Exe 72 50183 |
17 | Hakim CH, Li D, Duan D (2011) Monitoring murine skeletal muscle function for muscle gene therapy Methods Mol Biol 709: 75 89 |
18 | Segura LG, Lorenz JD, Weingarten TN, Scavonetto F, Bojanic K, Selcen D (2013) Anesthesia and Duchenne or Becker muscular dystrophy: Review of 117 anesthetic exposures Paediatr Anaesth 23: 9 855 864 |
19 | Hayes J, Veyckemans F, Bissonnette B (2008) Duchenne muscular dystrophy: An old anesthesia problem revisited Paediatr Anaesth 18: 2 100 106 |
20 | Girshin M, Mukherjee J, Clowney R, Singer LP, Wasnick J (2006) The postoperative cardiovascular arrest of a 5-year-old male: An initial presentation of Duchenne’s muscular dystrophy Paediatr Anaesth 16: 2 170 173 |
21 | Nathan A, Ganesh A, Godinez RI, Nicolson SC, Greeley WJ (2005) Hyperkalemic cardiac arrest after cardiopulmonary bypass in a child with unsuspected Duchenne muscular dystrophy Anesth Analg 100: 3 672 674 |
22 | Barthelemy I, Barrey E, Thibaud JL, Uriarte A, Voit T, Blot S (2009) Gait analysis using accelerometry in dystrophin-deficient dogs Neuromuscul Disord 19: 11 788 796 |
23 | Marsh AP, Eggebeen JD, Kornegay JN, Markert CD, Childers MK (2010) Kinematics of gait in golden retriever muscular dystrophy Neuromuscul Disord 20: 1 16 20 |
24 | Gaiad TP, Silva MB, Silva GC, Caromano FA, Miglino MA, Ambrosio CE (2011) Physical therapy assessment tools to evaluate disease progression and phenotype variability in golden retriever muscular dystrophy Res Vet Sci 91: 2 188 193 |
25 | Gaiad TP, Araujo KP, Serrao JC, Miglino MA, Ambrosio CE (2014) Motor physical therapy affects muscle collagen type I and decreases gait speed in dystrophin-deficient dogs PLoS One 9: 4 e93500 |
26 | Leyser M, Marques FJ, Elias MA, Diniz Gonsalves MC, da Silva OSJr, Carvalho RS (2013) Classic manifestations of Duchenne dystrophy in a young female patient: A case report Eur J Paediatr Neurol 17: 2 212 218 |
27 | Seemann N, Selby K, McAdam L, Biggar D, Kolski H, Goobie S (2011) Symptomatic dystrophinopathies in female children Neuromuscul Disord 21: 3 172 177 |
28 | Schanzer A, Rau I, Kress W, Kohler A, Neubauer B, Hahn A (2012) Duchenne muscular dystrophy in a 4-year-old girl due to heterozygous frame shift deletion of the dystrophin gene and skewed X-inactivation Klin Padiatr 224: 4 256 258 |
29 | Bladen CL, Salgado D, Monges S, Foncuberta ME, Kekou K, Kosma K (2015) The TREAT-NMD DMD global database: Analysis of more than 7000 Duchenne muscular dystrophy mutations Hum Mutat 36: 4 395 402 |
Figures and Tables
Fig.1
Dog night activity evaluation system. A, Overview of the system. The experimental dog was housed in the recording kennel. The CCD camera was installed to the ceiling allowing full visualization of the entire floor. Dog activity was recorded from 6 pm to 6 am next day by the “overnight dog recording” system. The signals were sent to the “overnight dog-video analyzer” for activity evaluation. B, Video processing outline. First, the baseline drift of the raw motion signals was corrected. Then noisy spikes were eliminated by the motion threshold (gray dotted line). C, A cartoon illustration of three types of motion spike(s): a single isolated spike, a stretch of continuous spikes and a stretch of spikes with short (≤10 s) interruptions.
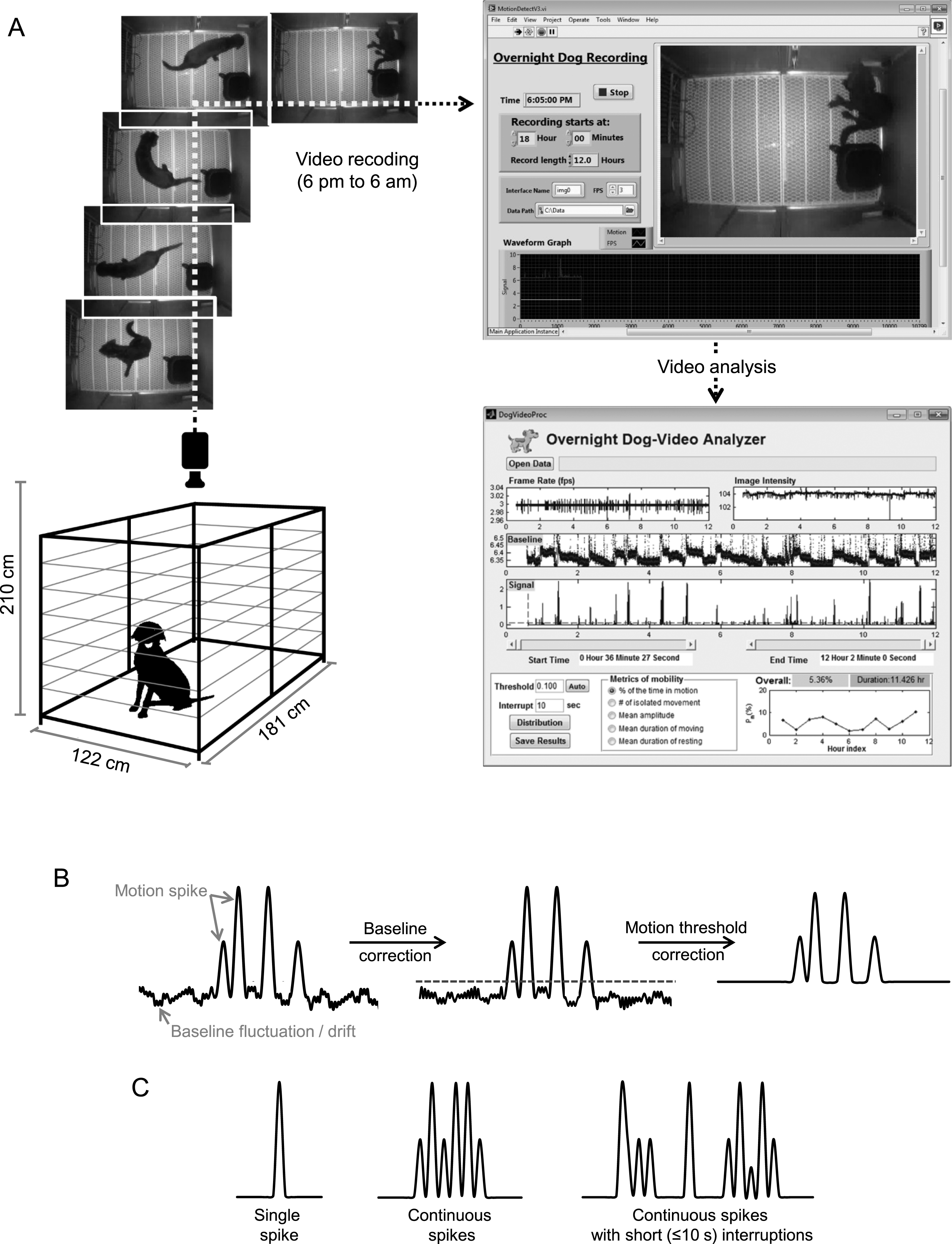
Fig.2
Repeated recording yielded consistent results from the same dog. The dog was recorded at different nights (either continuously every night or at alternating nights). The results of subsequent recordings were compared to that of the first night. The graph shows repeated recording results from 11 normal dogs and 10 affected dogs.
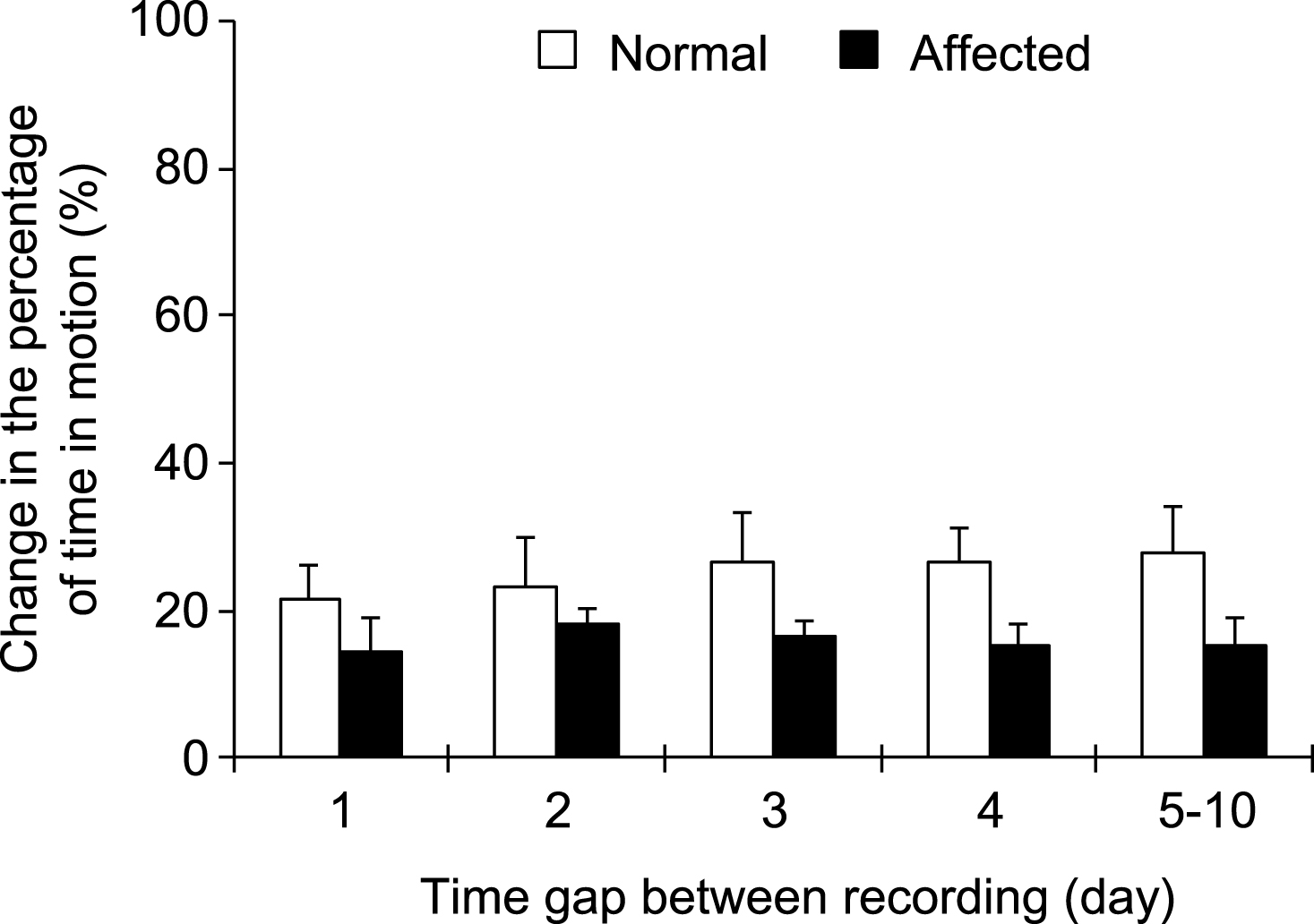
Fig.3
Night activity was significantly reduced in affected dogs. A, Representative processed motion signals from a normal (top panel) and an affected dog (bottom panel). The normal dog showed more activity than the affected dog. Dotted line, motion threshold. B to H, A quantitative comparison of indicated night activity property between normal (n = 12) and affected (n = 22) dogs. Asterisk, p < 0.0001.
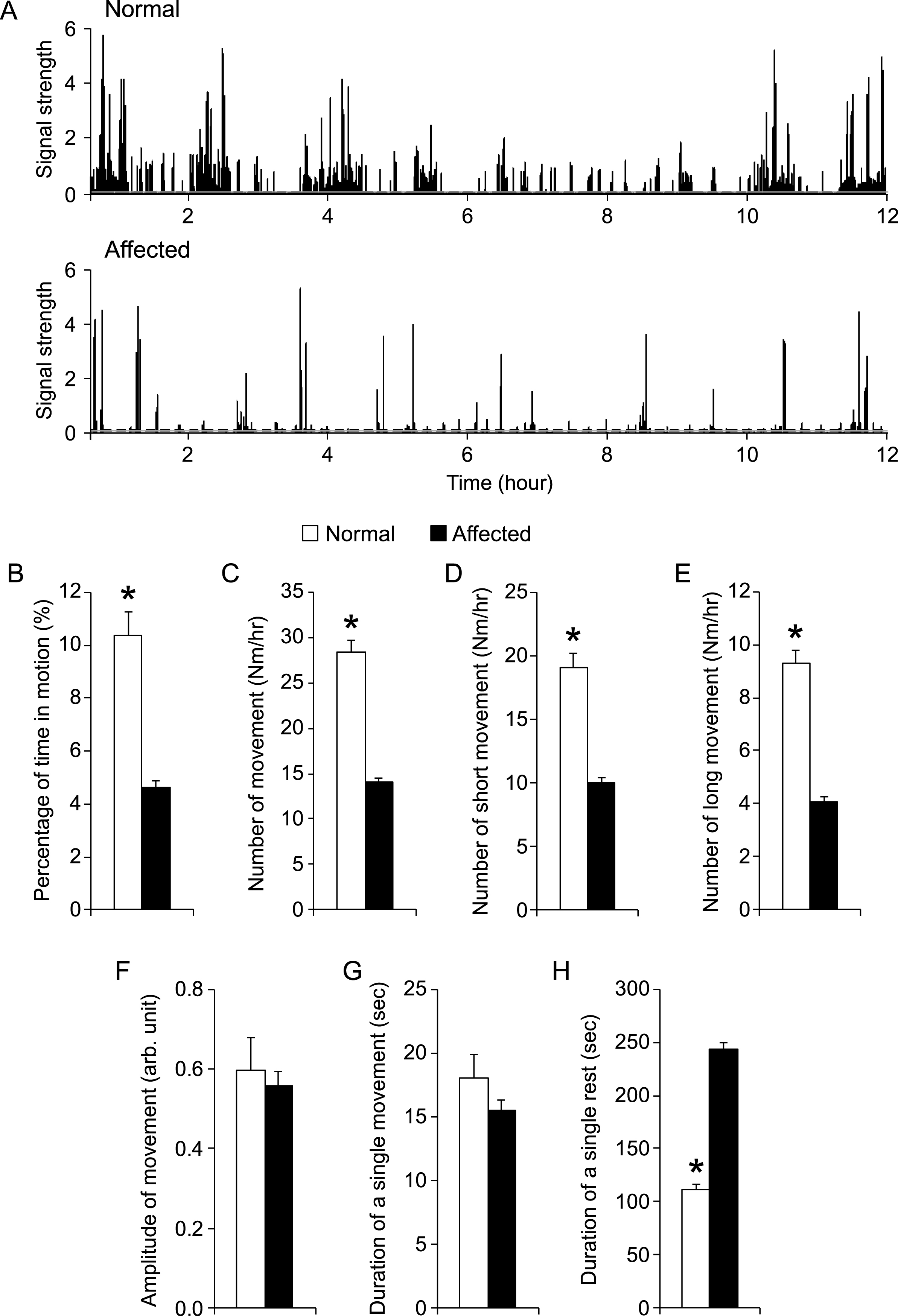
Fig.4
Comparison of night activity between normal and affected male dogs. n = 12 for normal male, n = 11 for affected male. Asterisk, p < 0.0001.
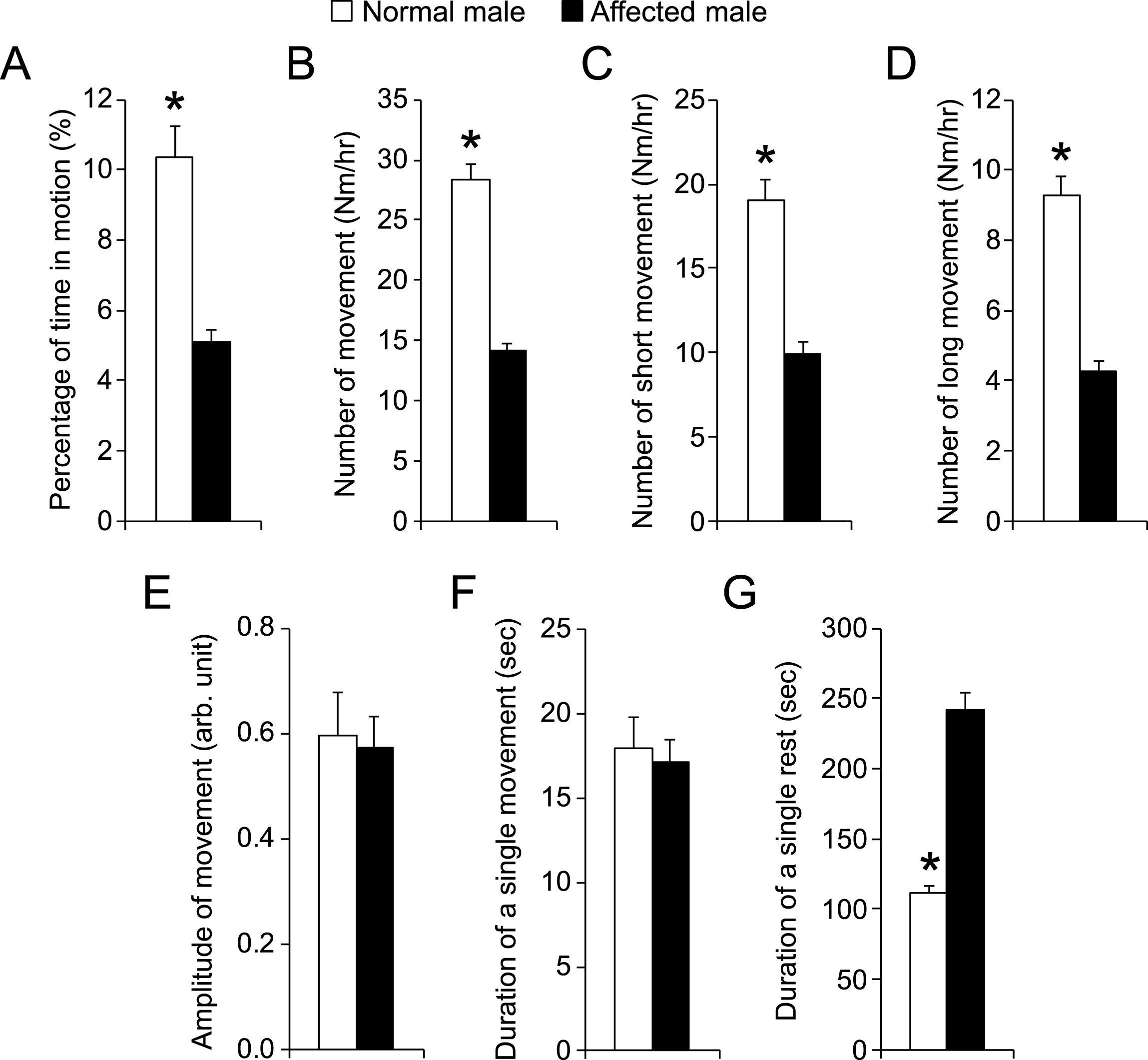
Fig.5
Comparison of night activity between male and female affected male dogs. n = 11 for affected male, n = 11 for affected female. Asterisk, p < 0.0001.
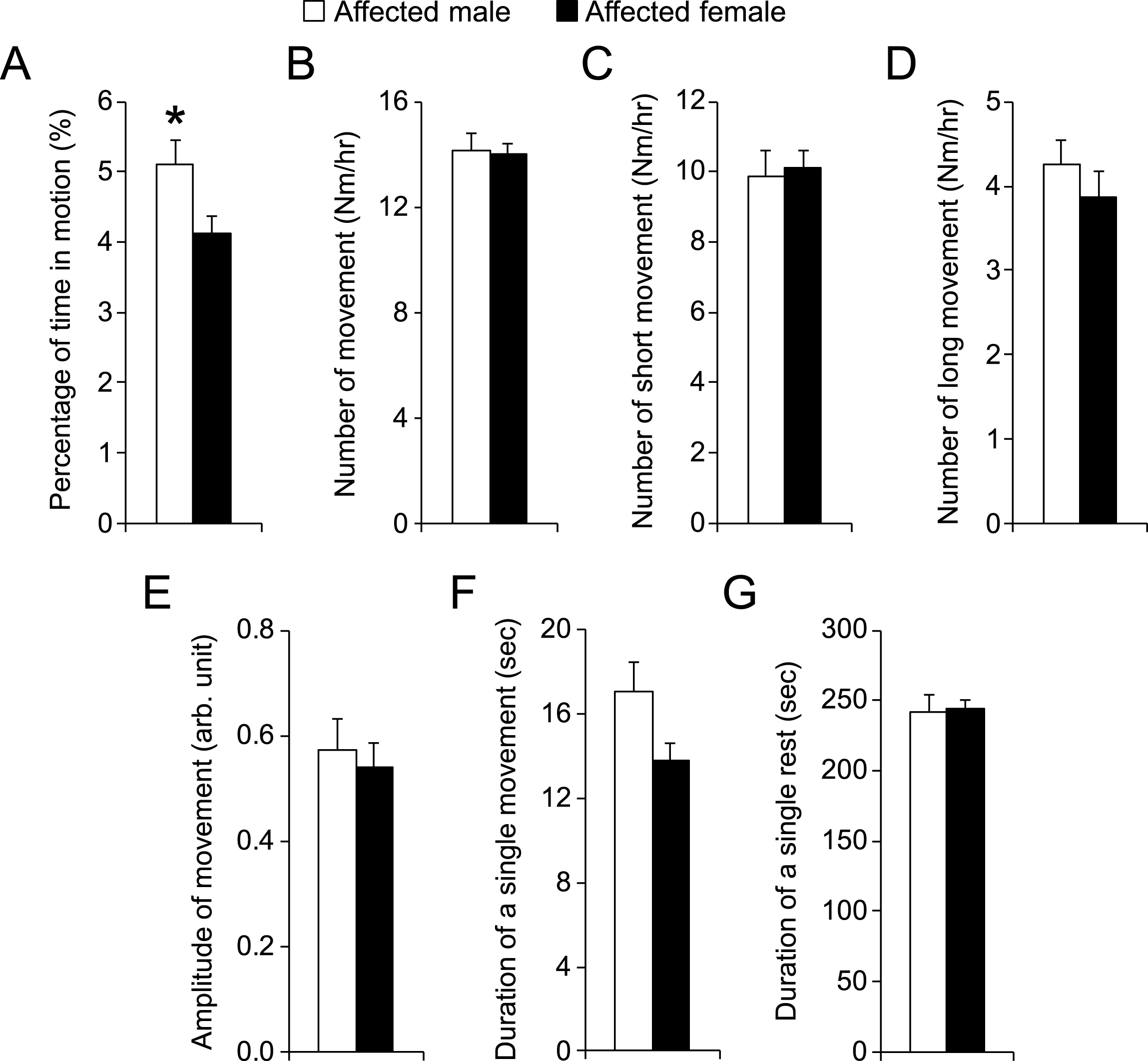
Fig.6
Comparison of night activity in female affected dogs that had different mutations in the dystrophin gene. LW, affected female dogs with one X-chromosome carrying intron 19 insertion and another X-chromosome carrying intron 13 insertion. GW, affected female dogs with one X-chromosome carrying intron 6 point mutation and another X-chromosome carrying intron 13 insertion. Asterisk, p < 0.0001.
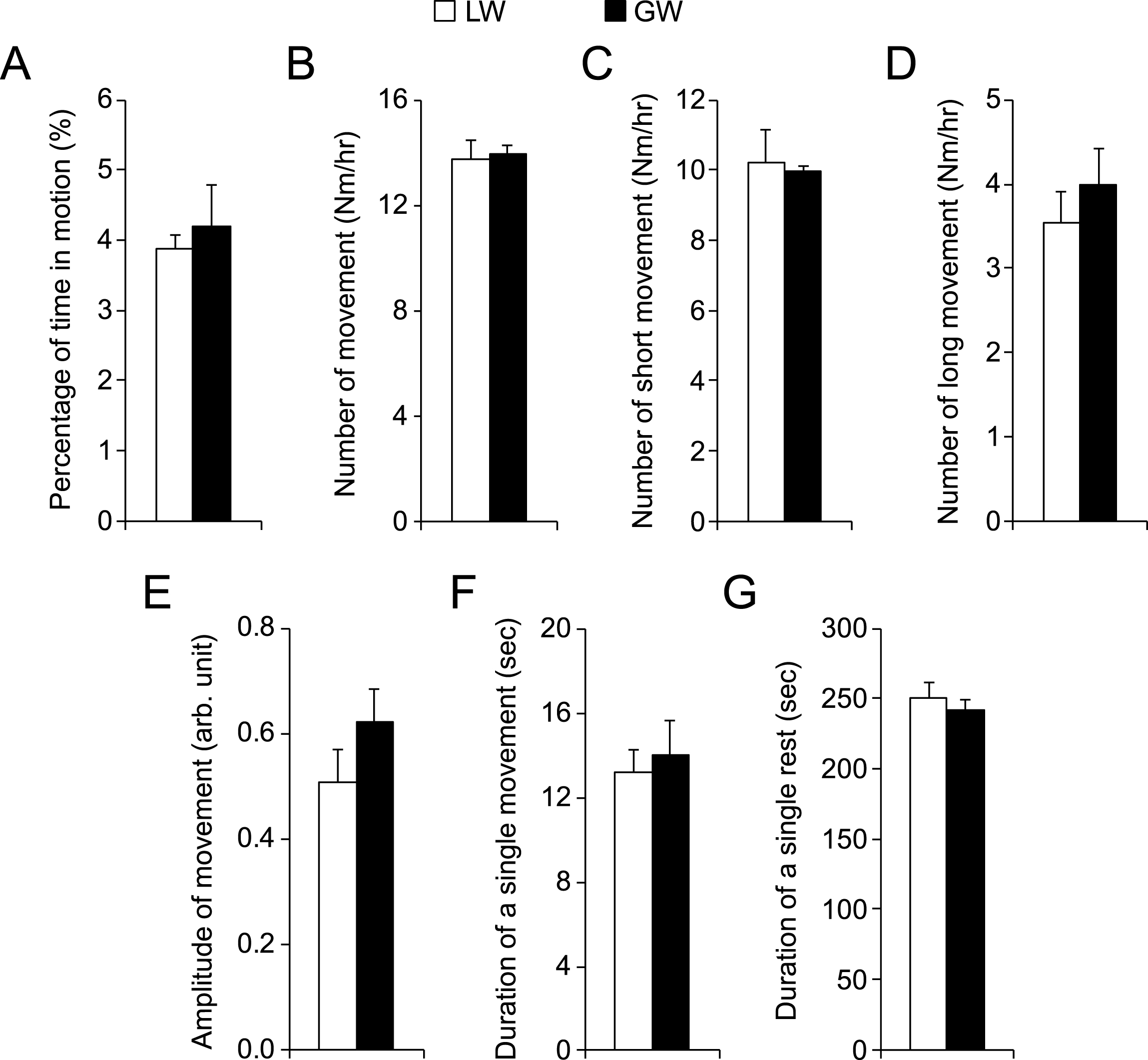