The effect of meconium exposure on the expression and differentiation of amniotic fluid mesenchymal stem cells
Abstract
BACKGROUND:
The goal of this study was to determine if exposure to meconium would alter the phenotype of amniotic fluid mesenchymal stem cells (AF-MSCs) and the ability of these cells to be differentiated into distal airway type cells.
METHODS:
Meconium was collected, lyophilized and resuspended in PBS at 3 different concentrations (high, medium, and low). AF-MSCs were cultured in the presence of this meconium suspension for 8 hours and then analyzed for changes in gene expression. Additionally, AF-MSCs exposed to meconium were differentiated for 14 days using modified small airway growth medium (mSAGM) and gene expression was determined. As a spontaneous differentiation control, meconium exposed AF-MSCs were cultured in amniotic fluid stem cell medium (AF medium).
RESULTS:
After 8 hours of exposure in culture, AF-MSCs had increased expression of distal airway genes aquaporin 5 (AQP5) and surfactant protein c (SPC) when cultured in AF medium containing meconium. These gene expression levels were similar to that of AF-MSCs that were differentiated in mSAGM for 14 days. Furthermore, there was an up regulation of pluripotency genes NANOG and OCT4 in response to low meconium concentration for 8 hours. Following 14 days of culture in mSAGM, there was an upregulation of TTF1, SPC and AQP5 expression in the control, as well as in the low and medium meconium exposed groups indicating that these cells were still able to be differentiated. High meconium concentration did, however, appear to influence the level of distal airway gene expression after 14 days in mSAGM. After 14 days in AF medium, there was significant downregulation in pluripotency and mesenchymal markers as well as distal airway gene expression in all groups.
CONCLUSION:
The phenotype of AF-MSCs is modulated by meconium exposure; however, the cells were still able to differentiate into distal airway gene and protein expression. This result supports the hypothesis that progenitor cells exist in the amniotic fluid and the presence of meconium may affect their initial phenotype. However, these cells were still able to be differentiated to a distal lung phenotype.
1Background
Meconium aspiration syndrome (MAS) is a life-threatening disease that affects some neonates born with meconium staining [1]. Despite the 10% to 16% incidence of meconium staining in neonates, the actual rate of MAS is quite low [2, 3]. This finding suggests that important predisposing factors, other than meconium itself, contribute to the development of MAS [1, 4]. There has been no correlation in the amount or timing of meconium exposure on the severity of MAS. [5–13]. At delivery, when meconium is present in the airways, it has been shown to inactivate surfactant and cause an inflammatory response [14]. The direct effect of meconium on the phenotype of amniotic fluid stem cells has not been studied and is important in understanding how this exposure will affect the potential use of these cells in patients.
Amniotic Fluid Mesenchymal Stem Cells (AF-MSCs) have been described as a source of autologous multipotent cells that could potentially be used to treat neonatal lung injury [15–17]. We and others have demonstrated the ability to isolate culture and differentiate AF-MSCs into distal airway cells [18–23]. The goal of this study was to evaluate the effect of meconium exposure on AF-MSCs and their subsequent ability to be differentiated into distal lung type cells. The ability to harvest AF-MSCs at or prior to birth provides a cell therapy option that could be used to treat lung injury in neonates including the injury caused by MAS. However, no studies have evaluated the effects of meconium on AF-MSCs.
2Materials and methods
2.1Culturing of AF-MSCs
Clonal populations of amniotic fluid mesenchymal stem cells were obtained, isolated and characterized as previously described [18] from 3 separate patients ranging from 36–38 weeks gestation who were undergoing amniocentesis for lecithin sphingomyelin assessment. Informed consent was obtained and this protocol was approved by the Hartford Hospital IRB committee (IRB#FINC003364HU). Each patient’s AF-MSCs were cultured separately in Amniotic Fluid (AF) Medium, which consisted of MEM Alpha (Life Technologies, Grand Island, NY), 18% Chang B Medium (Irvine Scientific, Santa Ana CA), 2% Chang C Supplement (Irvine Scientific, Santa Ana CA), 1X Penicillin/Streptomycin (Life Technologies, Grand Island, NY) and 1X Glutamax (Life Technologies, Grand Island, NY). Medium was changed every other day and cells were allowed to grow to approximately 70–80% confluence prior to splitting. Cells were split at a ratio of 1:3–1:4 not exceeding passage 10 for this study. Cells had a normal karyotype (data not shown). Clonal populations obtained from these AF samples were only utilized for this study if they expressed pluripotent and mesenchymal stem cell markers and did not express hematopoietic markers. Clonal AF-MSCs isolated from each patient were cultured separately for this study, so clonal populations from different patients were not mixed or combined.
2.2Characterization of AF-MSCs via flow cytometry
Cells were removed from tissue culture dishes using TrypLE (Life Technologies, Grand Island, NY). Cells were stained with fixable viability dye e450 according to manufacturer’s protocol (Affymatrix Santa Clara, CA). Cells were then fixed in 4% PFA for 5–10 minutes prior to staining cells. Cells were re-suspended in staining buffer (phosphate buffered saline & 2% fetal bovine serum) and incubated for 30 minutes on ice with antibodies that are summarized in Table 1. Cells were then rinsed and incubated for 20–30 minutes in staining buffer containing 0.1% Tween 20 (Sigma-Aldrich St. Louis, MO). Antibody against nuclear marker OCT 3/4 (Affymatrix Santa Clara, CA) was then added and incubated for an additional 30 minutes. Cells were then analyzed on a BD LSRII flow cytometer using BD FACS ARIA software (BD Bioscience San Jose, CA). For analysis, only viable cells were included and percent positivity for each marker was calculated and graphed using GraphPad Prism (GraphPad Software La Jolla, CA).
Table 1
Summary of stem cell markers
Marker | Conjugation | Company | |
SSEA4 | PE | Affymatrix | Pluripotent stem cell marker |
OCT3/4 | e660 | Affymatrix | Pluripotent stem cell marker |
TRA160 | PerCP e710 | Affymatrix | Pluripotent stem cell marker |
CD90 | PE-Cy7 | Affymatrix | Mesenchymal stem cell marker |
CD105 | PE | Affymatrix | Mesenchymal stem cell marker |
CD29 | FITC | Affymatrix | Mesenchymal stem cell marker |
CD44 | APC | Affymatrix | Mesenchymal stem cell marker |
2.3Patient enrollment
Patients were enrolled in this research study after meeting all inclusion criteria and after informed consent was obtained. Patients that were less than 18 years of age were excluded from the study for the ease of obtaining consent. Furthermore mothers could not have hypertension, gestational diabetes or pre-existing diabetes or suffering from substance abuse. In addition, prenatal labs had to be normal, which meant a negative result for HIV, RPR (Syphilis), HSV (Herpes Simplex), HBsAg (Hepatitis B Virus). The mothers must also have delivered at greater than or equal to 37 weeks gestation and been exclusively breast fed prior to the meconium collection. Patients were excluded from the study if they had a prenatal testing abnormality, had multiple births, used formula prior to the first meconium stool, were diagnosed with oligohydramnios or gave birth preterm (prior to 37 weeks).
2.4Collection and processing of meconium samples
Meconium samples were collected within the first 24 hours of life from infants after the mother met all inclusion and exclusion criteria detailed above. Meconium was first lyophilized to remove any moisture and to generate a powder that could be measured. Each sample was lyophilized separately; however, they were pooled prior to reconstitution. Lyophilization was performed using a freeze drying system (Labconco Kansas City, MO) and checked daily for up to 4 days. Following freeze drying, supernatants were generated by mixing meconium in phosphate buffered saline (PBS) at varying concentrations: Light meconium staining supernatant contained 3 mg of pooled meconium per ml of PBS (LMS), moderate meconium staining contained 6 mg of pooled meconium per ml of PBS (MMS) and thick meconium staining contained 12 mg of pooled meconium per ml of PBS (TMS) as previously described [24]. Samples were then spun in a Sorvall RC-26 Plus ultracentrifuge (Thermofisher Scientific Waltham, MA) at 10,000 RPM for 20 minutes. The supernatant was removed and used for treatment of cells.
2.5Exposure of AF-MSCs to meconium
AF-MSCs were seeded at 10,000 cells/cm2 on Matrigel coated 6-well plates as previously described [18]. Cells were allowed to reach approximately 70% confluence prior to initiating meconium exposure. Cell culture medium was aspirated and cells were rinsed once with PBS prior to application of meconium supernatant. Approximately 2mls of meconium supernatant from each concentration (LMS, MMS, and TMS) was added to wells in duplicate and allowed to incubate at 37°C for 8 hours. Control cells were treated with PBS alone for the same period of time. Cells were rinsed 2–3 times with PBS and Day 0 samples were collected for analysis. Treated and non-treated cells were then differentiated as described below. A diagram detailing the experimental procedure is illustrated in Fig. 1.
Fig.1
Schematic Describing the Meconium Exposure and Differentiation of AF-MSCs.
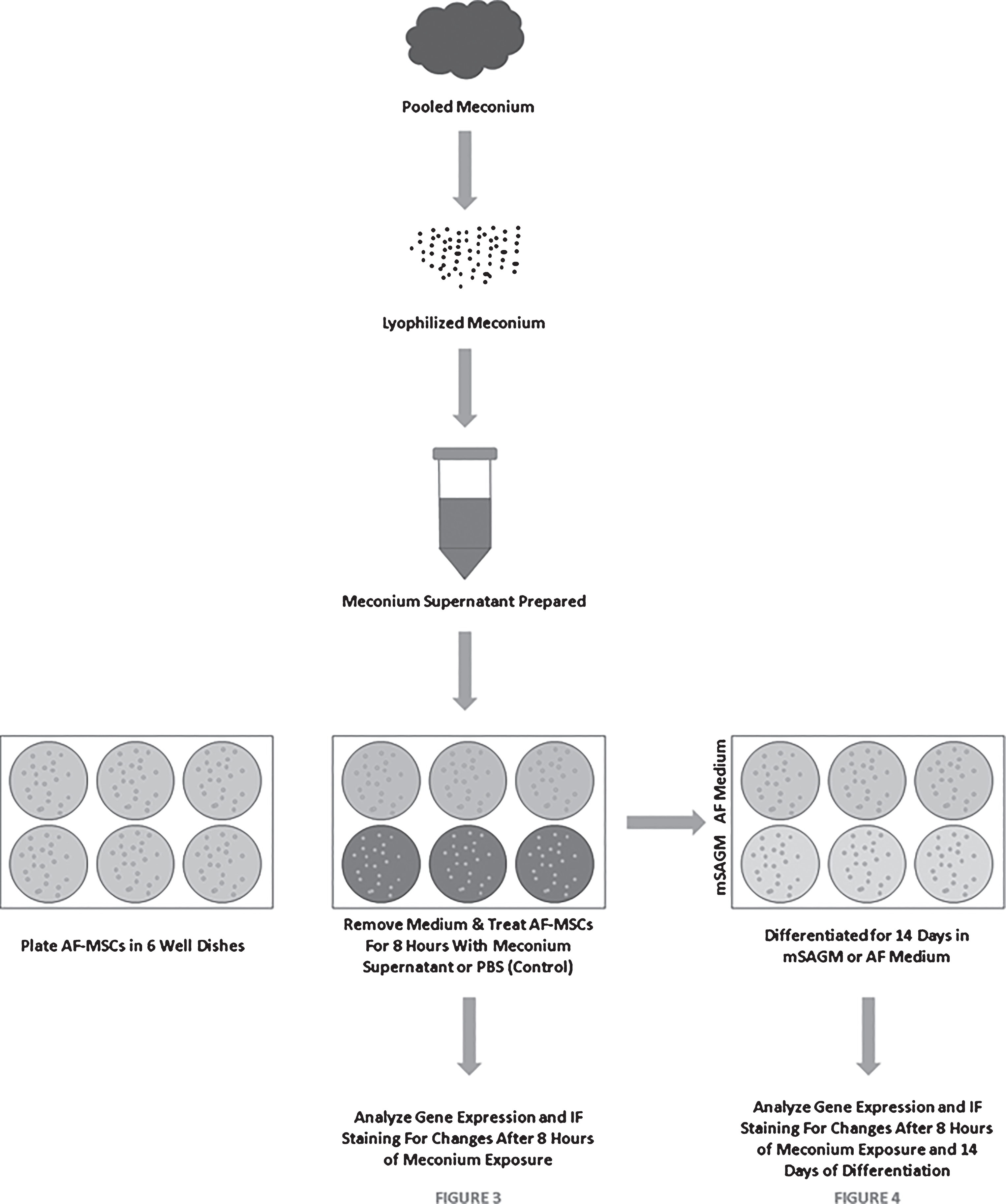
2.6Viability following meconium exposure
Following exposure to various concentrations of meconium, cells were trypsinized using TrypLE (Thermo Fisher Scientific Waltham, MA) and stained using fixable viability dye e780 (Affymatrix Santa Clara, CA) according to manufacturer’s instructions. Cells were strained using 50-micron mesh and analyzed for viability using an LSRII flow cytometer with BD FACS Aria Software (BD Biosciences San Jose, CA). Graphs and statistics were performed using GraphPad Prism software (GraphPad Software La Jolla, CA). Viability was run in triplicate for all conditions and for each patient cell line. Control conditions were treated with PBS that did not contain meconium.
2.7Distal airway cell differentiation
AF-MSCs were differentiated using a modified recipe of a commercially available small airway growth medium (mSAGM) (Lonza Walkersville, MD) as previous described to generate phenotypic distal airway-like cells (SPC, AQP5 and TTF1+) [18]. Medium was changed every other day for a total of 14 days of differentiation. As a spontaneous differentiation control, cells were also incubated in 14 days of AF medium. After 14 days of differentiation cells were collected for analysis by immunofluorescence and qRT-PCR.
2.8Quantitative real-time PCR
Cells were washed once with PBS prior to collection of RNA using Qiagen RNEasy mini kit (Qiagen Valencia, CA) according to the supplier’s protocol. RNA was quantified on a NanoDrop spectrophotometer (Thermo Fisher Scientific Waltham, MA) and 200 ng of cDNA was generated using iSCRIPT cDNA synthesis kit (Biorad Hercules, CA) according to the supplier’s protocol. Quantitative real-time PCR was performed on an ABI 7900HT Real-Time PCR System using Taqman PCR Mastermix (Thermo Fisher Scientific Waltham, MA). Primers utilized are shown in Table 2. The natural log of the calculated fold change relative to PBS treated AF-MSCs at Day 0 were graphed with standard deviations and statistics using GraphPad Prism (GraphPad Software La Jolla, CA).
Table 2
Quantitative real time PCR primer list
Target | Sequence or catalog number |
NANOG | Hs04399610 (ABI) |
POUF51 (OCT4) | Hs04260367 (ABI) |
ENG (CD105) | Hs00923996 (ABI) |
THY1 (CD90) | Hs00264235 (ABI) |
GAPDH | Hs02728991 (ABI) |
Pro-SPC | Fwd-GGCCAGGAACAAACAGGCTTCAAA |
Rev-ATCTTGCTGCAGGTGCTATGCTCT | |
TTF1 | Fwd-CGGCATGAACATGAGCGGCAT |
Rev-GCCGACAGGTACTTCTGTTGCTTG | |
AQP5 | Fwd-ACTGGGTTTTCTGGGTAGGG |
Rev-ATGGTCTTCTTCCGCTCTTC |
2.9Immunofluorescence
Cells were washed once with PBS and then fixed for 10 minutes with 4% PFA (Thermo Fisher Scientific Waltham, MA). Cells were then rinsed twice with PBS and incubated in Block/Perm solution consisting of 1X PBS with 0.1% Triton-X100 and 2% Fetal Bovine Serum (FBS) for 45 minutes. Cells were allowed to incubate overnight at 4°C in Block/Perm solution containing primary antibodies in a humidified chamber. Primary antibodies used included: NANOG (sc-33759: SantaCruz Biotechnology Dallas, TX), OCT 3/4 (sc-9081: SantaCruz Biotechnology Dallas, TX), SPC (sc-13979: SantaCruz Biotechnology Dallas, TX), AQP5 (sc-9890: SantaCruz Biotechnology Dallas, TX), KI67 (556003: BD Bioscience San Jose, CA), T1α (sc-59347: SantaCruz Biotechnology Dallas, TX). Cells were rinsed twice in PBS prior to incubating with Secondary Antibodies for 1 hour in the dark at room temperature: Goat Anti-Rabbit Alexa 546, Goat Anti-Mouse Alexa 546, Rabbit Anti-Goat Alexa 546 (Thermo Fisher Scientific Waltham, MA). Cells were then rinsed twice with PBS and counterstained with DAPI (Thermo Fisher Scientific Waltham, MA) diluted in PBS for 10 minutes prior to visualization and image capturing using a Zeiss Inverted Fluorescence Microscope and ZEN Blue Software (Carl Zeiss Pleasanton, CA).
3Results
3.1Phenotypic characteristics of AF-MSCs:
Clonal populations of AF-MSCs were assessed via flow cytometry where over 90% of AF-MSCs express mesenchymal stem cell markers (CD90, CD105, CD29, CD44) and stem cell marker Oct4 while a smaller portion (approximately 25–30%) express pluripotent stem cell markers (SSEA4, TRA160) (Fig. 2A). These cell lines have a normal karyotype, which has been previously published by our research group [18]. Furthermore, we have shown that these clonal populations of AF-MSCs can differentiate into adipogenic and osteogenic lineages, as previously published [18].
Fig.2
A. Freshly harvested third trimester AF-MSCs clonal populations from three patients (n = 3) demonstrate presence of pluripotent (Oct4, SSEA4, TRA160) and mesenchymal (CD90, CD105, CD29, CD44) phenotypes by flow cytometry analysis B: Viability of AF-MSCs following 8 hours of meconium exposure remained between 60–80% across all 3 patients in this study when analyzed in triplicate (n = 9).
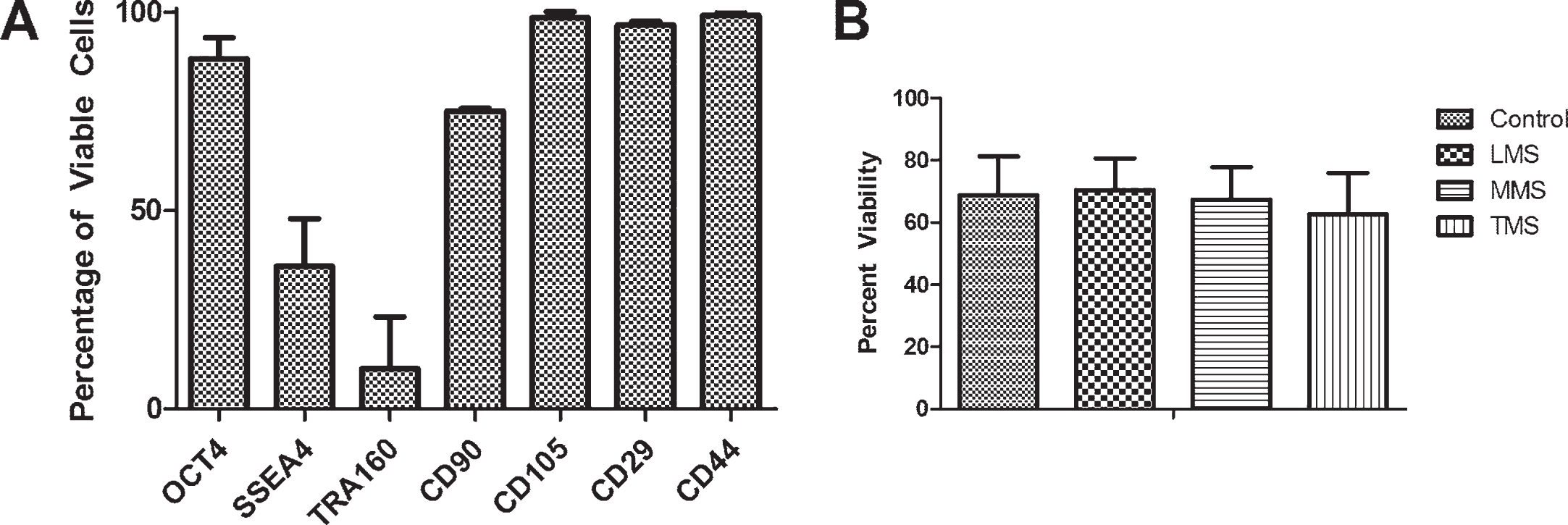
3.2Cell viability following exposure to meconium
After AF-MSCs were exposed for eight hours to three different concentrations of meconium (LMS- 3 mg meconium/1 ml PBS, MMS- 6 mg meconium/1 ml PBS, TMS- 12 mg meconium/1mlPBS) or PBS alone as a control, cell viability was evaluated by fixable viability dye and flow cytometry. There was no significant difference across the meconium exposed groups as compared to the PBS control (Fig. 2B). Statistical analysis was performed using one-way ANOVA with Bonferroni post-tests and a p-value≤0.05. The analysis demonstrated no significant differences in viability between the treatment groups after the 8 hour exposure. Data was obtained from 3 separate third trimester patients and each experimental condition was evaluated in triplicate for each patient.
3.3Expression of pluripotent and distal airway markers after exposure to meconium
Following eight hours of exposure to low meconium staining (LMS), there was increased expression of OCT4 and NANOG gene expression as compared to control cells (Fig. 3A). Mesenchymal markers CD90 and CD105 had minimal variation in their expression after meconium exposure. There was increased TTF1 expression following exposure to MMS, however, levels were down regulated in LMS and TMS conditions (Fig. 3B). There was minimal changes in SPC and AQP5 expression compared to PBS control. (Fig. 3B). None of the gene expression changes were statistically significant. AF-MSCs were still proliferative after 8 hours of meconium exposure at different concentrations as evident by KI67 staining, albeit qualitatively less so in the TMS condition (Fig. 3C). Distal airway markers AQP5 and T1α appeared to maintain their phenotypic expression when compared to control (Fig. 3C).
Fig.3
Gene Expression of AF-MSCs after 8 Hours of Exposure to Meconium compared to Controls (8 hours of exposure to PBS). A: Gene expression of pluripotency markers NANOG and OCT4 were increased after LMS exposure compared to PBS control, but decreased significantly in the MMS and TMS groups. CD90 and CD105 expression has no appreciable change after meconium exposure. B: Distal airway markers SPC and AQP5 were slightly elevated following meconium exposure but did not reach significance. TTF-1 had variable expression depending on the concentration of meconium. (* = P≤0.05 ** = P≤0.01) C: Immunofluorescence of Pluripotent Markers after 8 Hours of Meconium Exposure as Compared to PBS treated Control Cells. Qualitatively there is a decrease in the number of OCT4 and NANOG expressing cells with higher concentrations of meconium similar to flow cytometry results (Fig. 2A). Expression of T1alpha and AQP5 persists throughout all conditions. Magnification 400X.
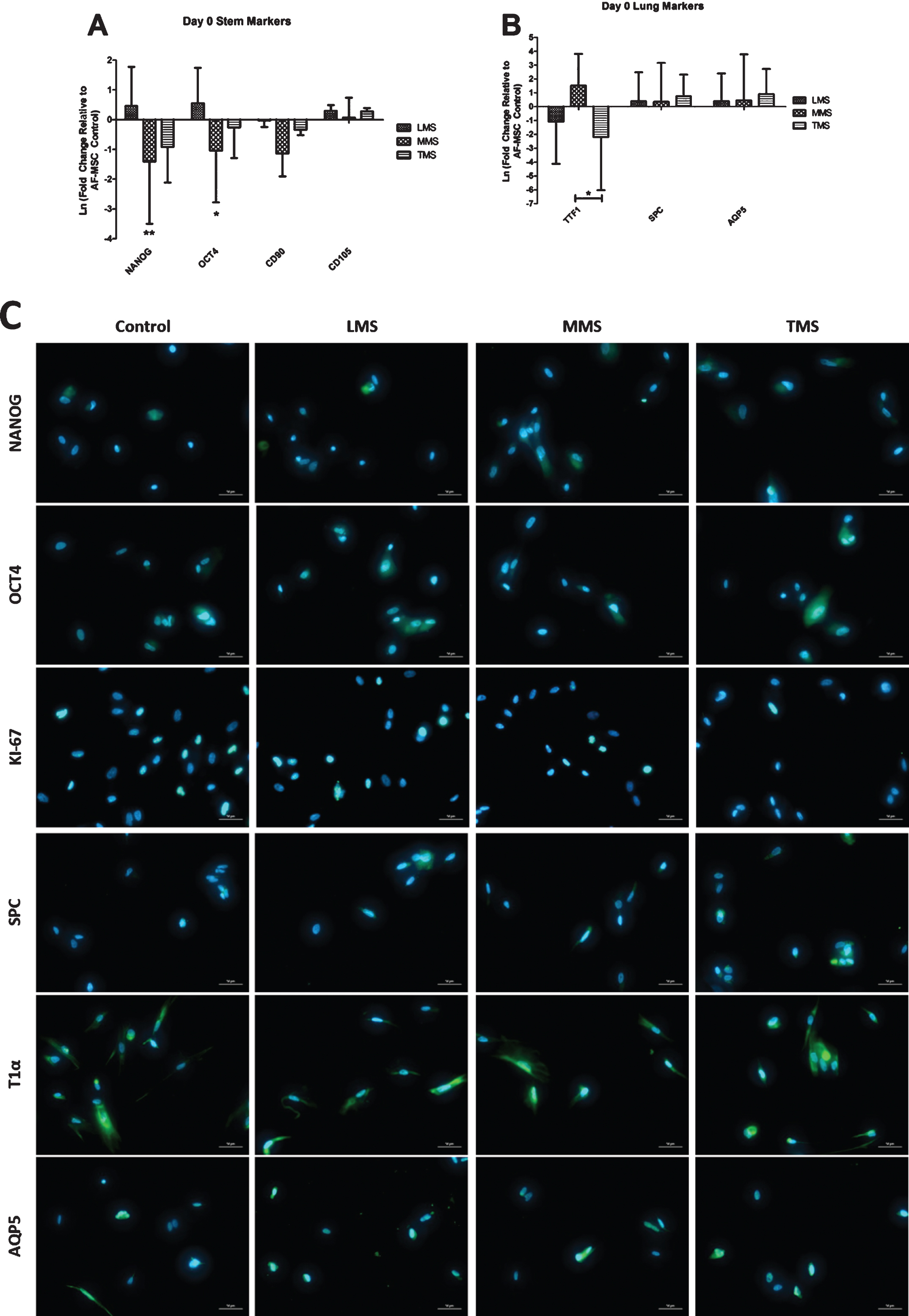
3.4Expression of distal airway and pluripotent genes after differentiation following exposure to meconium
Following 8 hours of exposure to various meconium concentrations or PBS as a control, cells were then differentiated for 14 days in modified small airway growth medium (mSAGM) to determine their ability to generate phenotypic distal airway cells. AF medium was used as a control to evaluate the AF-MSCs ability to differentiate into distal lung type cells spontaneously.
After initial meconium exposure and 14 days in AF Medium there was upregulation of pluripotency gene OCT4 in the LMS conditions compared to cells treated only with PBS (Fig. 4A). Furthermore, there appeared to be a decrease in mesenchymal gene expression for CD90 in all meconium conditions when compared to PBS treated controls, however, it was not significant. Expression of CD105 was minimally upregulated after 14 days in AF media (Fig. 4A). After 14 days in AF medium, AF-MSCs demonstrated downregulation of distal airway markers TTF1, SPC, and AQP5 (Fig. 4B). None of these conditions were statistically significant.
Fig.4
Gene Expression of Meconium exposed AF-MSCs after 14 Days of Differentiation in either AF media or mSAGM. A: All conditions were normalized to AF-MSCs that were exposed to PBS for 8 hours but were not differentiated. AF-MSCs exposed to meconium were cultured for 14 days in AF medium to evaluate for spontaneous differentiation. In separate experiments, AF-MSCs exposed to meconium were cultured in mSAGM to induce differentiation to distal airway phenotypes. In AF medium, exposure to meconium (LMS, MMS, and TMS) results in significant decrease in gene expression for pluripotency markers OCT4 and NANOG and minimal changes in mesenchymal marker expression when compared to PBS exposed AF-MSCs (controls). B: In AF media, gene expression of phenotypic distal airway markers was decreased throughout. C: Cells that were cultured in small airway growth medium demonstrated increased NANOG expression in the MMS and TMS conditions. There were some minor qualitative increases in expression of CD105 and CD90, but they were not consistent between groups. D: Lastly, cells that were cultured in small airway growth medium for 14 days had increased expression of distal airway markers SpC and AQP5 except in the TMS condition. TTF-1 expression was variable among the groups. E: Immunofluorescence staining of cells following 14 days in AF Medium or mSAGM differentiation demonstrate qualitatively more staining for AQP5 and SPC in the MMS and TMS conditions compared to control. Magnification 400X.
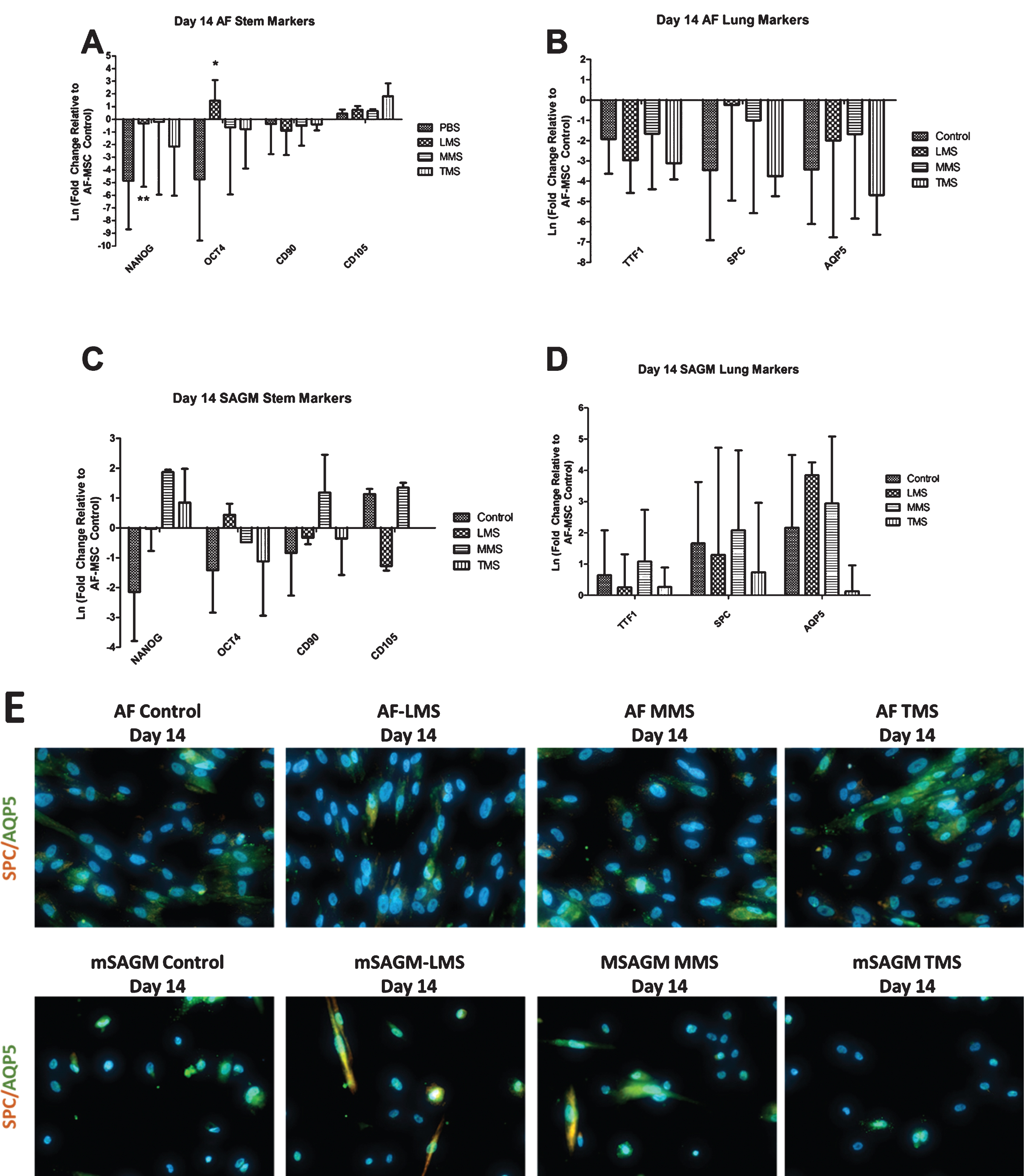
Cells that were cultured in mSAGM for 14 days demonstrated increased NANOG expression in the MMS and TMS conditions (Fig. 4C). There were some minor qualitative increases in expression of CD105 and CD90, but they were not consistent between groups (Fig. 4C). Lastly, cells that were cultured in mSAGM for 14 days had increased expression of all phenotypic distal airway markers (Fig. 4D). On immunofluorescence, AF-MSCs that were differentiated in mSAGM demonstrated expression of distal airway markers AQP5 and SPC in the LMS and MMS conditions but not in the TMS condition (Fig. 4E). AF-MSCs cultured in AF medium had persistent expression of AQP5 in all cultures (Fig. 4E). Gene expression of AF-MSCs when directly compared between mSAGM and AF differentiation shows an increase in TTF1 expression following culture in mSAGM in both meconium exposed and PBS treated cells (Fig. 5A). Gene expression of SPC was significantly increased in both meconium exposed and PBS treated groups when incubated for 14 days in mSAGM compared to AF medium (Fig. 5B). Gene expression of SPC was also significantly elevated in meconium exposed cells that were differentiated in mSAGM for 14 days compared to AF-MSCs treated only with PBS in AF Medium (Fig. 5B). Lastly, gene expression of distal airway gene AQP5 was also significantly upregulated in cells that were exposed to meconium or just PBS following differentiation for 14 days in mSAGM compared to AF medium (Fig. 5C). Additionally, AQP5 gene expression was significantly higher in cells that were exposed to meconium and differentiated in mSAGM compared to cells that were not meconium exposed and incubated in AF Medium (Fig. 5C). This data demonstrates that meconium exposure does not affect the ability of AF-MSCs to upregulate gene expression of distal airway genes after 14 days in mSAGM culture.
Fig.5
Comparison of Distal Airway Gene Expression after 14 Days in Culture Following Meconium Exposure. A. Gene expression of TTF1 was increased after 14 days in mSAGM compared to cells incubated in AF medium. The same result was seen with or without 8 hours of exposure to meconium. B. Gene expression of SPC was significantly increased after 14 days in mSAGM compared to cells cultured in AF Medium irrespective of exposure to meconium. SPC Gene expression of cells exposed to MMS and then cultured in 14 days of mSAGM was significantly higher than non-treated cells cultured in AF Medium. C. Gene expression of AQP5 was significantly increased after 14 days in mSAGM with or without exposure to meconium. Furthermore, cells cultured for 14 days in mSAGM after meconium exposure demonstrated significantly higher AQP5 expression compared to AF cells culture in AF medium with no meconium exposure. (* = P≤0.05).

4Conclusions
The pathophysiology of meconium aspiration syndrome is complex and multifactorial. A bulk of evidence implies that meconium is a potent activator of inflammatory mediators, including cytokines, complement, prostaglandins and reactive oxygen species (ROS) [4, 14, 25–27]. Meconium consists of numerous substances of host origin mainly derived from the digestive tract [14]. New evidence suggests that those patients who exhibit severe signs of MAS at the time of birth had inhalation and exposure of the proximal and distal airways of the lung to meconium in utero long before delivery took place.
Current treatment for MAS focuses on supportive care ventilation and suctioning to remove as much meconium as possible [28]. Although these treatment strategies improve patient condition, response can be variable. Therefore, new patient-specific therapeutic approaches are being explored using stem cell therapy.
AF-MSCs provide a patient-specific therapeutic cell source that can be obtained at or before the time of birth. These cells have been shown to be immunomodulatory and anti-inflammatory with little engraftment seen after administration of cells [18, 21, 29–34]. Furthermore, these cells can be differentiated into distal airway type cells as previously described [18], which may help repair damaged tissue and reduce inflammation. The purpose of this study was to investigate the affect meconium may have on the phenotype of these cells and the potential of these cells to be differenitated into distal lung phenotypes. Since meconium stained fluid could still contain a patient-specific cell option it is imperative to understand how this exposure affects viability, cellular phenotype and their ability to differentiate into distal airway cells.
The exposure time chosen in this study for AF-MSCS to meconium was 8 hours. Clinically, rupturing of membranes needs to take place to diagnose initial meconium staining, so data prior to that event is not known. Data that is available details secondary meconium exposure with times ranging from 10 minutes to 8.5 hours [35]. Although the time frame varies greatly, we chose a timepoint of 8 hours for this study as this would be the worst case scenario based on this retrospective clinical data. In addition, meconium in this study was lyophliized to allow for accuracy in creation of meconium concentrations. Meconium contains many components including: intestinal epithelial cells, amniotic fluid, bile, water and mucus and recently it has been described that meconium is not sterile [36, 37]. Studies have shown that lyophilization of infant fecal samples will not alter the oligosacharride or bacterial composition of the sample which is important for this study [38]. In addition, it has been shown that lipid content can be preserved during lyophilization [39]. This is important as small amounts of cholesterol and fatty acids have been found in meconium[37].
After 8 hours, the viability of AF-MSCs exposed to various concentrations of meconium was not significantly different suggesting that meconium does not lead to immediate cell death. Proliferation was also not significantly altered by exposure to meconium. In our data, we have noticed a trend in gene expression patterns although no statistically significant. We postulate that these changes may be releated to inflammation as seen in previous studies were cells exposed to condition medium from inflammed cells had higher gene expression of pluripotent markers [39, 40]. Therefore, our study supports the concept that meconium exposure does effect the phenotype of AF-MSCs but the extent is not completely clear.
In addition to changes in expression of pluripotency genes, we noted some increased expression of distal airway cell markers SPC and AQP5 following meconium exposure. It has been previously described that lung progenitor cells in response to the inflammatory mileu have the ability to differentiate into distal airway cell types to mitigate damage [40, 41]. Therefore, it is reasonable to infer that inflammation caused by meconium exposure could select for AF-MSCs that express distal airway cell markers. Further studies are needed to determine the exact mechanism and populations of cells in the amniotic fluid. Additionally, obtaining AF-MSCs from a meconium stained birth would be helpful in determining exact intial phenotypes of AF-MSCs in this circumstance for comparison. AF-MSCs that were exposed to PBS and then differentiated for 14 days in AF Medium (as a spontaneous differentiation control) demonstrated a downregulation of pluripotency gene expression as well as CD90 gene expression. This result is not surprising as cells were confluent after 14 days and this likely contributed to the loss of pluripotency and mesenchymal gene expression. These cells normally are maintained at no greater than 70–80% confluence to maintain phenotype, so this overconfluence and downregulation of gene expression that was observed is a reasonble explanation. Futhermore, expression of any distal airway genes was drastically downregulated indicating that although distal airway genes were increased after 8 hours of exposure, this same result did not continue after 14 days of culture.
Lastly, cells that were exposed to meconium and subsequently cultured in mSAGM demonstrated increases in NANOG expression in the MMS and TMS conditions as well as increased OCT4 gene expression in the LMS condition. This result was different than that of cells culture in AF medium, which again suggests that confluency may have had a role in the changes in gene expression seen in the AF Medium group. AF-MSCs cultured in mSAGM for 14 days demonstrated increased expression of distal airway genes TTF1, SPC and AQP5.
Taken together, the data obtained from this descriptive study illustrates that 8 hours of meconium exposure does alter gene expression and phenotype of amniotic fluid mesenchymal stem cells. Further studies are needed to identify the mechanisms responsible for this phenotypic alteration. Lastly, AF-MSCs exposed to meconium still retain the ability to differentiate into distal airway phenotypes when cultured in mSAGM. This lends support for the ability of these cells to be exploited for translational applications despite meconium exposure.
Disclosures
Authors do not have any financial interests or disclosures to report.
Statement of financial support
This work was funded by Connecticut Children’s Medical Center Strategic Research Funding.
Acknowledgments
We would like to acknolwedge the NICU staff and research staff at Connecticut Children’s for helping to collect and transport the meconium samples for our studies.
References
[1] | Singh BS , Clark RH , Powers RJ , Spitzer AR . Meconium aspiration syndrome remains a significant problem in the NICU: Outcomes and treatment patterns in term neonates admitted for intensive care during a ten-year period. J Perinatol (2009) ;29: (7):497–503. |
[2] | Zhang X , Kramer MS . Variations in mortality and morbidity by gestational age among infants born at term. J Pediatr (2009) ;154: (3):358–62, 62.e1. |
[3] | Fanaroff AA . Meconium aspiration syndrome: Historical aspects. J Perinatol (2008) ;28: (Suppl 3):S3–7. |
[4] | Choi W , Jeong H , Choi SJ , et al. Risk factors differentiating mild/moderate from severe meconium aspiration syndrome in meconium-stained neonates. Obstet Gynecol Sci (2015) ;58: (1):24–31. |
[5] | Ross MG . Meconium aspiration syndrome–more than intrapartum meconium. N Engl J Med (2005) ;353: (9):946–8. |
[6] | Ghidini A , Spong CY . Severe meconium aspiration syndrome is not caused by aspiration of meconium. Am J Obstet Gynecol (2001) ;185: (4):931–8. PubMed PMID: 11641681. |
[7] | Hernández C , Little BB , Dax JS , Gilstrap LC , Rosenfeld CR . Prediction of the severity of meconium aspiration syndrome. Am JObstet Gynecol (1993) ;169: (1):61–70. |
[8] | Sunoo C , Kosasa TS , Hale RW . Meconium aspiration syndrome without evidence of fetal distress in early labor before elective cesarean delivery. Obstet Gynecol (1989) ;73: (5 Pt 1):707–9. |
[9] | Spong CY , Ogundipe OA , Ross MG . Prophylactic amnioinfusion for meconium-stained amniotic fluid. Am J Obstet Gynecol (1994) ;171: (4):931–5. |
[10] | Ahanya SN , Lakshmanan J , Morgan BL , Ross MG . Meconium passage in utero: Mechanisms, consequences, and management. Obstet Gynecol Surv (2005) ;60: (1):45–56; quiz 73-4. |
[11] | Turbeville DF , McCaffree MA , Block MF , Krous HF . In utero distal pulmonary meconium aspiration. South Med J (1979) ;72: (5):535–6. |
[12] | Brown BL , Gleicher N . Intrauterine meconium aspiration. Obstet Gynecol (1981) ;57: (1):26–9. |
[13] | Fischer C , Rybakowski C , Ferdynus C , Sagot P , Gouyon JB . A population-based study of meconium aspiration syndrome in neonates born between 37 and 43 weeks of gestation. Int J Pediatr (2012) ;2012: :321545. |
[14] | Mokra D , Mokry J . Glucocorticoids in the treatment of neonatal meconium aspiration syndrome. Eur J Pediatr (2011) ;170: (12):1495–505. |
[15] | Cananzi M , Atala A , De Coppi P . Stem cells derived from amnioticfluid: New potentials in regenerative medicine. Reprod BiomedOnline (2009) ;18: (Suppl 1):17–27. |
[16] | Antonucci I , Stuppia L , Kaneko Y , et al. Amniotic fluid as a rich source of mesenchymal stromal cells for transplantation therapy. Cell Transplant (2011) ;20: (6):789–95. |
[17] | De Coppi P , Bartsch G , Siddiqui MM , et al. Isolation of amniotic stem cell lines with potential for therapy. Nat Biotechnol (2007) ;25: (1):100–6. |
[18] | Vadasz S , Jensen T , Moncada C , et al. Second and third trimester amniotic fluid mesenchymal stem cells can repopulate a de-cellularized lung scaffold and express lung markers. J Pediatr Surg (2014) ;49: (11):1554–63. |
[19] | Sessarego N , Parodi A , Podestà M , et al. Multipotentmesenchymal stromal cells from amniotic fluid: Solid perspectivesfor clinical application. Haematologica (2008) ;93: (3):339–46. |
[20] | You Q , Cai L , Zheng J , Tong X , Zhang D , Zhang Y . Isolation of human mesenchymal stem cells from third-trimester amniotic fluid. Int J Gynaecol Obstet (2008) ;103: (2):149–52. |
[21] | Ma X , Zhang S , Shang Y , et al. [Involvement of human amniotic fluid colony derived stem cells in regeneration of mouse injured muscle]. Zhongguo Xiu Fu Chong Jian Wai Ke Za Zhi (2011) ;25: (7):842–7. |
[22] | Moorefield EC , McKee EE , Solchaga L , et al. Cloned, CD117 selected human amniotic fluid stem cells are capable of modulating the immune response. PLoS One (2011) ;6: (10):e26535. |
[23] | Bottai D , Cigognini D , Nicora E , et al. Third trimester amniotic fluid cells with the capacity to develop neural phenotypes and with heterogeneity among sub-populations. Restor Neurol Neurosci (2011) . |
[24] | Eidelman AI , Nevet A , Rudensky B , et al. The effect of meconium staining of amniotic fluid on the growth of Escherichia coli and group B streptococcus. J Perinatol (2002) ;22: (6):467–71. |
[25] | Lindenskov PH , Castellheim A , Saugstad OD , Mollnes TE . Meconium aspiration syndrome: Possible pathophysiological mechanisms and future potential therapies. Neonatology (2015) ;107: (3):225–30. |
[26] | Funai EF , Labowsky AT , Drewes CE , Kliman HJ . Timing of fetal meconium absorption by amnionic macrophages. Am J Perinatol (2009) ;26: (1):93–7. d. |
[27] | Mokra D , Calkovska A . How to overcome surfactant dysfunction in meconium aspiration syndrome? Respir Physiol Neurobiol (2013) ;187: (1):58–63. |
[28] | Swarnam K , Soraisham AS , Sivanandan S . Advances in the management of meconium aspiration syndrome. Int J Pediatr (2012) ;2012: :359571. |
[29] | Sedrakyan S , Da Sacco S , Milanesi A , et al. Injection of amnioticfluid stem cells delays progression of renal fibrosis. J Am Soc Nephrol (2012) ;23: (4):661–73. |
[30] | Yeh YC , Lee WY , Yu CL , et al. Cardiac repair with injectable cellsheet fragments of human amniotic fluidstem cells in an immune-suppressed rat model. Biomaterials (2010) ;31: (25):6444–53. |
[31] | Pederiva F , Ghionzoli M , Pierro A , De Coppi P , Tovar JA . Amniotic fluid stem cells rescue both in vitro and in vivo growth, innervation, and motility in nitrofen-exposed hypoplastic rat lungs through paracrine effects. Cell Transplant (2013) ;22: (9):1683–94. |
[32] | Wen ST , Chen W , Chen HL , et al. Amniotic fluid stem cells from EGFP transgenic mice attenuate hyperoxia-induced acute lung injury. PLoS One (2013) ;8: (9):e75383. |
[33] | Bottai D , Scesa G , Cigognini D , et al. Third trimester NG2-positive amniotic fluid cells are effective in improving repair in spinal cord injury. Exp Neurol (2014) ;254C: :121–33. |
[34] | Villani V , Milanesi A , Sedrakyan S , et al. Amniotic fluid stem cells prevent beta-cell injury. Cytotherapy (2014) ;16: (1):41–55. |
[35] | Incerti M , Locatelli A , Consonni S , Bono F , Leone BE , Ghidini A . Can placental histology establish the timing of meconium passage during labor? Acta Obstet Gynecol Scand (2011) ;90: (8):863–8. |
[36] | Jiménez E , Marín ML , Martín R , Odriozola JM , Olivares M , Xaus J , et al. Is meconium from healthy newbornsactually sterile? Res Microbiol (2008) ;159: (3):187–93. |
[37] | Davy J . On the composition of the meconium, and of the vernix caseosa, or lubricating matter of the new-born infant. Med Chir Trans (1844) ;27: :189–97. |
[38] | Lewis ZT , Davis JC , Smilowitz JT , German JB , Lebrilla CB , Mills DA . The impact of freeze-drying infant fecal samples on measures of their bacterial community profiles and milk-derived oligosaccharide content. PeerJ (2016) ;4: :e1612. |
[39] | Cavazos-Garduño A , Serrano-Niño J , Solís-Pacheco J , et al. Effect of pasteurization, freeze-drying and spray drying onthe fat globule and lipid profile of human milk. Food Nutr Res (2016) ;4: (5):296–302. |
[40] | Buckley S , Shi W , Carraro G , Sedrakyan S , Da Sacco S , Driscoll BA , et al. The milieu of damaged alveolar epithelial type 2 cells stimulates alveolar wound repair by endogenous and exogenous progenitors. Am J Respir Cell Mol Biol (2011) ;45: (6):1212–21. |
[41] | Pociask DA , Scheller EV , Mandalapu S , et al. IL-22 is essential for lung epithelial repair following influenza infection. Am J Pathol (2013) ;182: (4):1286–96. |