Early Amygdala Activation and Later Ventromedial Prefrontal Cortex Activation During Anger Induction and Imagery
Abstract
Background:
Neurobiological studies implicate the amygdala and related limbic/paralimbic structures, such as the ventromedial prefrontal cortex (VMPFC), in anger and aggression. Previous studies of self-generated anger using Positron Emission Tomography (PET) have consistently documented a lack of amygdala activation during anger.
Objective:
We investigated the hypothesis that a lack of amygdala activation during anger is due to differences in the time course of amygdala and VMPFC activation. Specifically, we explored whether the amygdala is involved in the early phases of anger experience which is later followed by increased VMPFC activation.
Methods:
Eighteen healthy control participants underwent fMRI. We adapted an anger induction paradigm previously used in our PET study, in which neutral and angry states were induced using autobiographical scripts. The hypothesized time course of amygdala and VMPFC activation during acute anger induction and imagery were modeled. Region of interest (ROI) analyses were used to identify significant a priori region activation, and correlations were run between signal values and VAS anger ratings.
Results:
Amygdala activation increased during the acute phase of anger induction and decreased during the later phase of anger imagery, whereas VMPFC activation decreased during anger induction and increased during anger imagery, compared to the neutral conditions. In addition, negative correlations were found between self-ratings of anger and bilateral VMPFC activation.
Conclusions:
Overall, our results suggest that the amygdala may be active at the initial onset of anger while the VMPFC is activated over time as the individual sustains and perhaps regulates that emotional state.
INTRODUCTION
Anger is a part of everyone’s life. Excessive feelings of anger, irritability and/or hostility, however, are associated with diminished quality of life, relationship difficulties, and adverse health effects such as increased risk for coronary artery disease [e.g., 1–4]. As many as 50% of outpatient psychiatric populations have reported moderate to severe levels of anger and aggression [5].
Anterior paralimbic and limbic brain regions are implicated in neurobiological studies of anger and impulsive aggression. Positron emission tomography (PET) studies have consistently shown increased regional cerebral blood flow in the ventromedial prefrontal cortex (VMPFC) during anger, imagined expression of anger, and exposure to anger in healthy controls [e.g., 6–9]. Individuals with a predisposition to anger and aggression (murderers, violent offenders, and people with antisocial personality disorder [APD]) exhibit decreased activation in the prefrontal cortex compared with healthy controls in PET and single-photon emission computed tomography (SPECT) studies [10–13]. A PET study by our group also found that patients with Major Depressive Disorder (MDD) and anger attacks demonstrate blunted VMPFC activation during anger induction compared to healthy controls and MDD patients without anger attacks [8].
Another brain region that has been implicated in anger and aggression is the amygdala. Among other functions, the amygdala appears to be involved in the initial assessment of threat and facilitation of fight or flight responses [14–17]. For example, following exposure to threatening visual stimuli, patients with APD show reduced potentiation of the startle reflex and impaired aversive conditioning [e.g., 18, 19] comparable to those observed in patients with amygdala lesions [20, 21]. Patients with APD and those with amygdala lesions also exhibit impairments in the processing of fearful facial expressions [e.g., 22–25]. A structural MRI study [26] of patients with temporal lobe epilepsy found that those with comorbid intermittent explosive disorder (IED) had substantially higher rates of amygdala atrophy or amygdala lesions than those without comorbid IED. Finally, a functional MRI study [27] using a memory task demonstrated that participants who scored higher on a scale of antisocial behavior demonstrated reduced amygdala activation while processing negatively valenced words compared with individuals who scored lower on the scale.
Although the amygdala has been consistently found activated in studies involving negative affective, particularly fearful, stimuli [28–31], there is a striking absence of findings involving amygdala activation during anger states in functional imaging studies using anger induction paradigms. One reason for this absence of amygdala activations in anger induction studies may lie in the nature of neural connectivity between the VMPFC and the amygdala, and the time course of amygdala activations. Specifically, there are known bidirectional connections between the prefrontal cortex (especially the medial prefrontal cortex) and the amygdala [32–34], and there is evidence in healthy controls that these two structures are mutually inhibitory, with increased activity in one structure inhibiting activity in the other structure [8, 35, 36]. fMRI studies investigating the temporal characteristics of the amygdala response to affective stimuli have shown that healthy samples exhibit rapid amygdala habituation to emotional stimuli [37–41].
Anger induction paradigms have primarily used PET to measure regional changes in cerebral blood flow associated with anger states. This imaging method is limited in temporal resolution. Changes in regional cerebral blood flow are averaged over longer periods of time (usually >60 seconds) to achieve acceptable signal to noise ratios. If anger induction is associated with an early initial, but not sustained, amygdala activation, it is possible that existing PET anger induction studies may have failed to capture this initial amygdala activation in response to induction of anger. The present study was designed to investigate this hypothesis. We conducted an anger induction experiment with healthy volunteers and assessed changes in VMPFC and amygdala activation using fMRI, which has a better temporal resolution than PET. Based on the above neuroimaging findings we hypothesized a negative correlation between amygdala and VMPFC activation during anger induction and imagery. Specifically, we hypothesized that the amygdala is involved in the early phases of anger experience which is later followed by increased VMPFC activation.
MATERIALS AND METHODS
Participants
All participants provided written informed consent in accordance with the guidelines of the Partners Human Research Committee (IRB). Study participants were eighteen right-handed healthy volunteers (7 male, 11 female) with a mean age of 29.17 years (SD = 8.35, range = 35). The mean years of education was 16.50 (SD = 1.47, range = 4). All participants had no history of psychiatric or neurological disorder as assessed by the Structured Clinical Interview for the Diagnostic and Statistical Manual of Mental Disorders (DSM-IV) [42]. The Trait Anger scale of the State-Trait Anger Expression Inventory (STAXI-2) indicated a slightly lower than average anger reactivity with a mean percentage of 31.5% and a standard deviation of 23.28% [43].
Scripts
Following the diagnostic evaluation, study participants prepared descriptions about anger provoking and neutral life events. These autobiographical scripts of participants’ past personal events were prepared according to a previously published procedure [8]. Briefly, each subject wrote 2 paragraphs of their most and second most angry experiences (e.g. the day I lost my job) and 2 autobiographical neutral events (e.g. going for a walk or cooking dinner). Subjects were also given a list of bodily responses (e.g. “heart racing,” “labored breathing,” “palms clenched,” etc.) and incorporated the bodily responses they had experienced into their paragraphs. Based on the information provided, the experimenters created four 30 second, second-person, present tense scripts, and recorded them in a neutral voice for playback in the scanner.
Anger induction paradigm
Subjects completed four functional runs. Subjects heard two neutral and two angry autobiographical scripts. Each script corresponded to one run. Prior to each run, subjects were instructed to close their eyes. Each run consisted of an initial 60 second resting period followed by the presentation of the autobiographical script. Subjects listened to each script (“induction”), and then continued to experience the induced feeling for additional 60 seconds (“imagery”) when they were asked to stop.
MRI acquisition
Participants were scanned using a Symphony/Sonata 1.5T MRI scanner equipped for echo planar imaging (Siemens Medical Systems, Iselin NJ) with a 3-axis gradient head coil. Head movements were restricted using foam cushions. Sound was projected using headphones, which also served to reduce the noise of the scanner. Following automated scout and shimming procedures, two high-resolution 3D MPRAGE sequences (TR = 7.25 ms, TE = 3 ms, flip angle = 7 degrees; voxel size 1.3×1×1 mm) were collected for positioning of subsequent scans. fMRI images (i.e. blood oxygenation level dependent signal or BOLD) were acquired using a T2* -weighted sequence (30 coronal slices perpendicular to the anterior/posterior commissures, 5 mm thickness, skip 1 mm, TE = 40 ms, TR = 3 s, flip angle = 90 degrees).
Visual analog scales (VAS)
After the MRI scan, for each run, subjects rated the degree to which they experienced anger, arousal, fear, disgust, happiness, sadness, surprise, guilt, and shame during each run on a 0–10 analog scale where 0 indicated a complete absence of the emotion and 10 indicated the maximum possible experience of that emotion.
fMRI data analyses
Data was pre-processed using SPM 5 software (Wellcome Department of Cognitive Neurology, London, UK). All scans showed acceptable levels of motion with less than 3 mm of movement in any direction. fMRI images were motion corrected and spatially normalized to the standardized space established by the Montreal Neurologic Institute (MNI; http://www.bic.mni.mcgill.ca). Images were then smoothed with a three-dimensional, 6 mm FWHM Gaussian filter. Structural images were co-registered with motion corrected functional images and normalized to MNI standardized space.
For each subject, we created contrast images representing each individual condition of interest (i.e., rest before anger, anger induction, anger imagery, rest after anger). For second level random effects analysis, we conducted a flexible factorial analysis using each individual subject’s contrast images. We then modeled the hypothesized time course of amygdala and VMPFC activation during anger induction and imagery (relative to the neutral conditions; Fig. 1). Specifically, we modeled an early increase of amygdala activation during anger induction followed by increased VMPFC activation during anger imagery.
Fig.1
We modeled the hypothesized time course of amygdala and ventromedial prefrontal cortex (VMPFC) activation during anger induction and imagery relative to the neutral conditions. Specifically, we modeled an early increase of amygdala activation during anger induction followed by increased VMPFC activation during anger imagery.
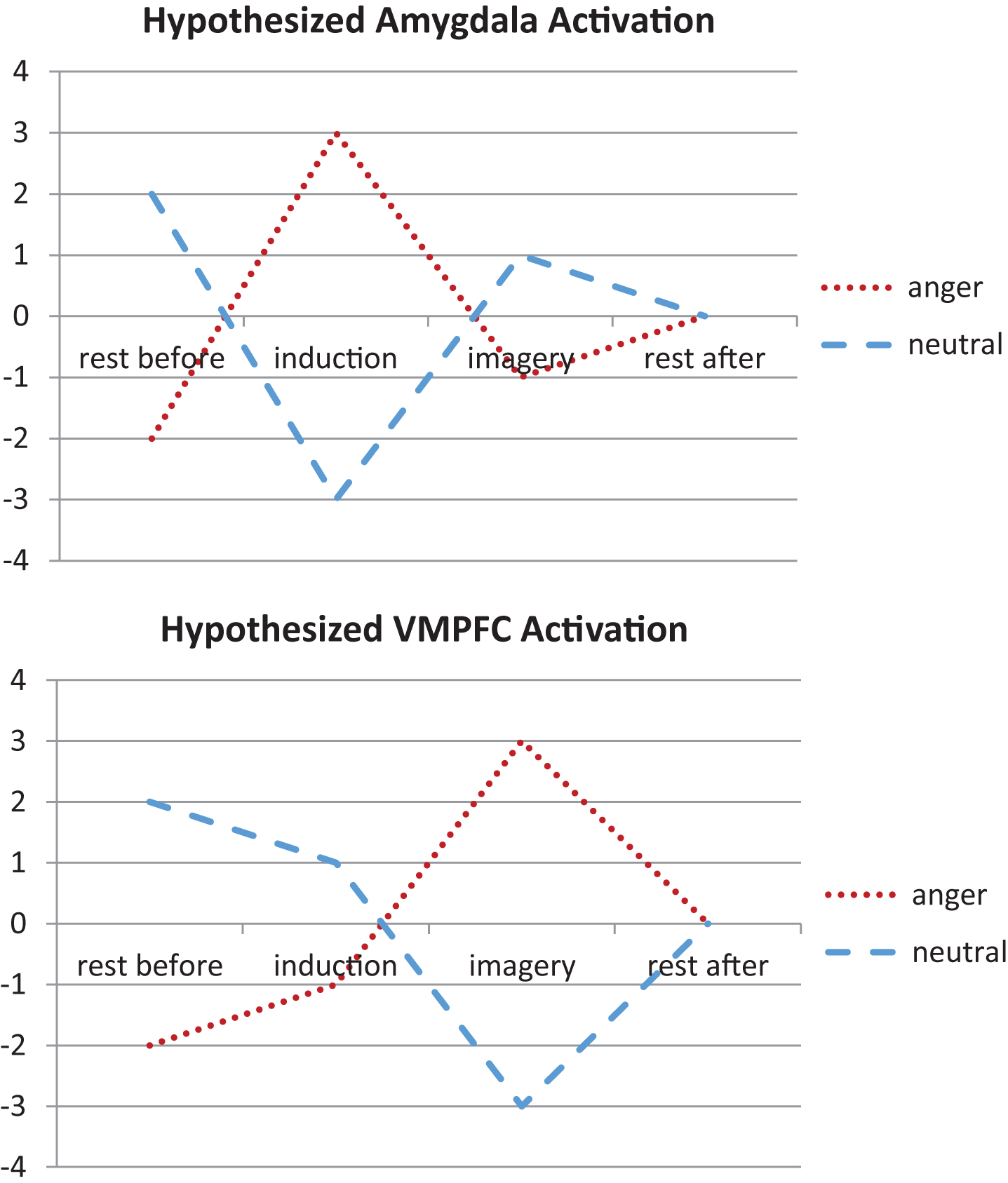
Region of interest (ROI) analyses were then completed for the amygdala (bilateral) as defined by anatomical masks from the Wake Forest University Pick Atlas [44]. To control for multiple statistical comparisons, we maintained a cluster-level false positive detection rate at p < 0.05 with a cluster (k) extent empirically determined by Monte Carlo simulations in the AFNI program AlphaSim (Ward, http://afni.nimh.nih.gov/afni/docpdf/alphasim.pdf). For the VMPFC, spherical ROIs (radius = 5 mm) were centered around coordinates from our previous findings using the same paradigm in PET (+/–8 62 –10) [8].
We also conducted exploratory psychophysiological interaction (PPI) analyses to understand changes in effective connectivity between a functional seed in the VMPFC and the amygdala going from the neutral imagery period to the anger imagery period.
Statistical analyses
Tests of normality on the visual analog scale ratings revealed that most of the ratings (e.g., arousal, sadness, and shame ratings during the neutral induction, arousal, fear, and disgust ratings during the anger induction, etc.) were not normally distributed. All of these ratings were log transformed. Visual analog scale ratings were then analyzed using a 2×2×9 repeated measures ANOVA with run (run 1 and run 2), induction type (anger and neutral), and emotion (anger, arousal, fear, disgust, happiness, sadness, surprise, guilt, and shame) as the within-subject factors. Follow-up pairwise comparisons were then run to understand if participants specifically felt more anger after the anger induction compared to the neutral induction period. To understand the relationship between VAS ratings and activation in the VMPFC and amygdala, correlational analyses were run. Correlation analyses were also run to understand the relationship between STAXI-2 Trait Anger scale scores and activation in the VMPFC and amygdala.
RESULTS
Behavioral data
The repeated measures ANOVA analyzing visual analog scale ratings revealed a main effect of emotion (F(1.69,21.92) = 35.31, p < 0.001). Simple contrasts revealed that subjects reported higher anger ratings during the anger induction compared to the neutral induction (F(1,13) = 17.84, p < 0.001).
fMRI data
We found significant clusters of activation within the amygdala and VMPFC which demonstrated our hypothesized time course of activation (Fig. 1). Specifically, we found significant bilateral activation in the amygdala that exceeded AlphaSim correction for multiple comparisons to preserve an α<0.05 (MNI coordinates for right = 18, 0, –16, k = 43, Z-score = 3.35; MNI coordinates for left = –24, –6, –12, k = 45, Z-score = 2.95; Fig. 2) that showed early increased activation during the anger induction relative to the neutral induction. However, during anger imagery, there was increased left VMPFC activation (MNI coordinates = –4, 60, –12, k = 3, Z-score = 2.98) that was trending in significance (preserving an α of 0.09 after AlphaSim correction for multiple comparisons). Correspondingly, the amygdala showed decreased activation during the anger imagery period. Our VMPFC finding was a replication of our result from our previous PET study [8]. For a list of all brain regions that demonstrated a similar pattern of increased activation during anger induction and decreased activation during anger imagery (and vice versa), see Table 1.
Fig.2
We found significant bilateral activation in the amygdala during anger induction followed by increased left ventromedial prefrontal cortex (VMPFC) activation during anger imagery. The VMPFC activation was trending in significance.
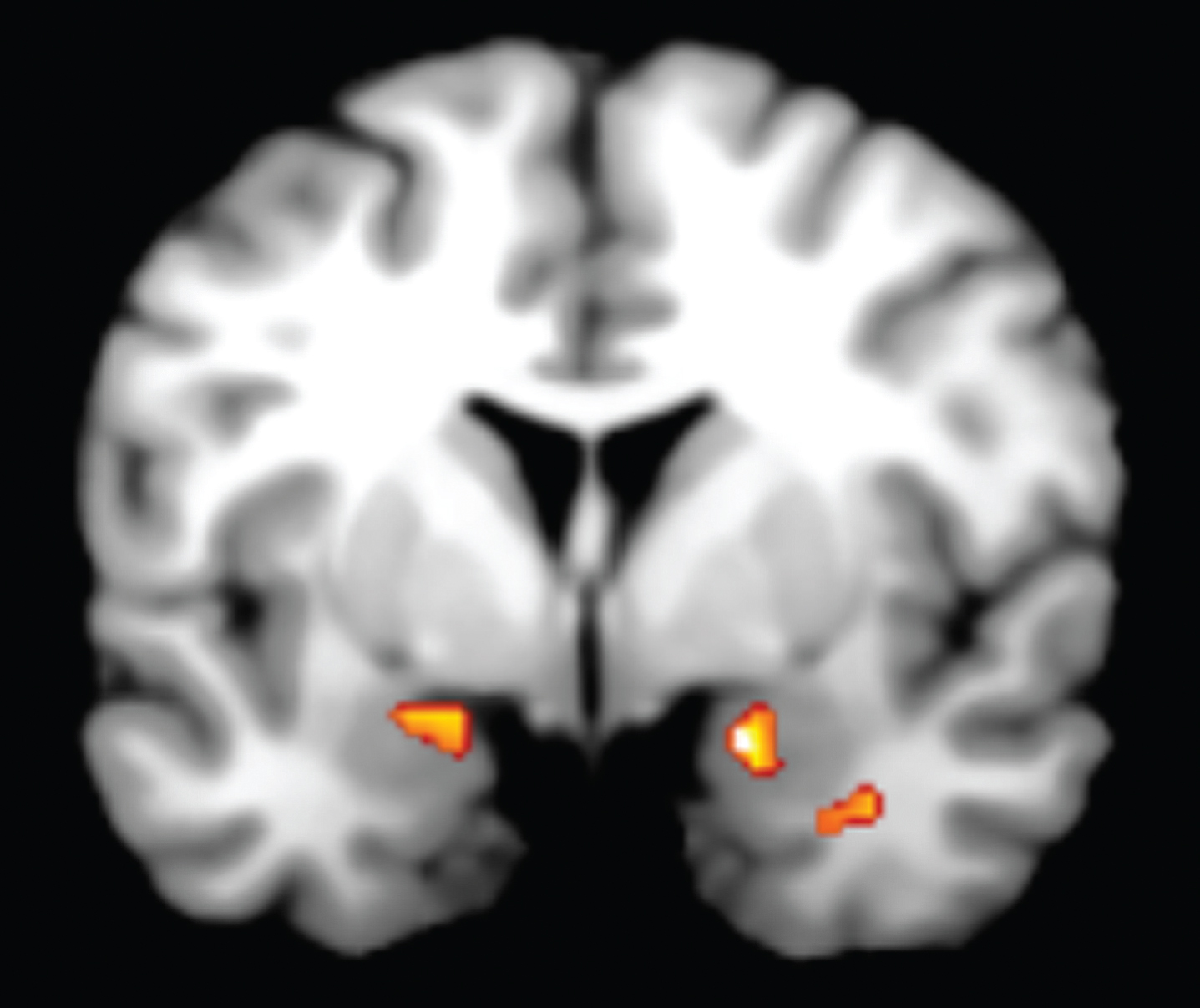
Table 1
All fMRI activations
Region | MNI Coordinates (x, y, z) | Cluster size (k) | T-score | Z-score |
Using the Hypothesized Amygdala Model Contrast | ||||
Midbrain | –14, –16, –14 | 117 | 3.98 | 3.80 |
Parahippocampal gyrus | 30, –16, –20 | 118 | 3.67 | 3.52 |
Superior frontal gyrus | 16, 54, 32 | 62 | 3.65 | 3.51 |
Corpus callosum | –8, 24, 0 | 41 | 3.62 | 3.48 |
Superior temporal gyrus | 54, –22, –2 | 49 | 3.37 | 3.26 |
Using the Hypothesized VMPFC Model Contrast | ||||
Midbrain | –14, –22, –22 | 130 | 5.35 | 4.95 |
Putamen | 32, 0, 2 | 61 | 5.34 | 4.95 |
Inferior frontal gyrus | –38, 32, –8 | 83 | 4.95 | 4.63 |
Precentral gyrus | –34, –22, 54 | 74 | 4.72 | 4.44 |
Medial frontal gyrus | –2, 26, 46 | 126 | 4.40 | 4.16 |
Sub-gyral | 26, 24, 40 | 72 | 4.33 | 4.11 |
Extra-nuclear | –8, –2, –10 | 108 | 4.11 | 3.92 |
Precentral gyrus | 36, –2, 30 | 110 | 4.09 | 3.90 |
Inferior frontal gyrus | 54, 12, 20 | 60 | 4.09 | 3.90 |
Culmen | –8, –36, –18 | 97 | 3.97 | 3.79 |
Inferior frontal gyrus | –26, 30, –10 | 56 | 3.93 | 3.76 |
Insula | 42, 22, –8 | 52 | 3.86 | 3.70 |
Anterior cingulate | 4, 28, 24 | 43 | 3.74 | 3.59 |
Right cerebrum | 16, 6, –18 | 48 | 3.63 | 3.49 |
Cingulate gyrus | 6, –4, 30 | 48 | 3.47 | 3.34 |
Sub-gyral | –38, 0, –26 | 58 | 3.39 | 3.27 |
Note: All clusters shown at p < 0.005 and k > 40 voxels.
Our exploratory PPI analyses revealed decreased coupling between the VMPFC functional seed and the left amygdala (MNI coordinates = –22, –8, –14, k = 4, Z-score = 2.27, p < 0.05; Fig. 3) when participants were experiencing anger (anger imagery period) compared to experiencing a neutral emotion (neutral imagery period).
Fig.3
Exploratory PPI analyses revealed decreased coupling between the VMPFC functional seed and the left amygdala when participants were experiencing anger (anger imagery period) compared to experiencing a neutral emotion (neutral imagery period).
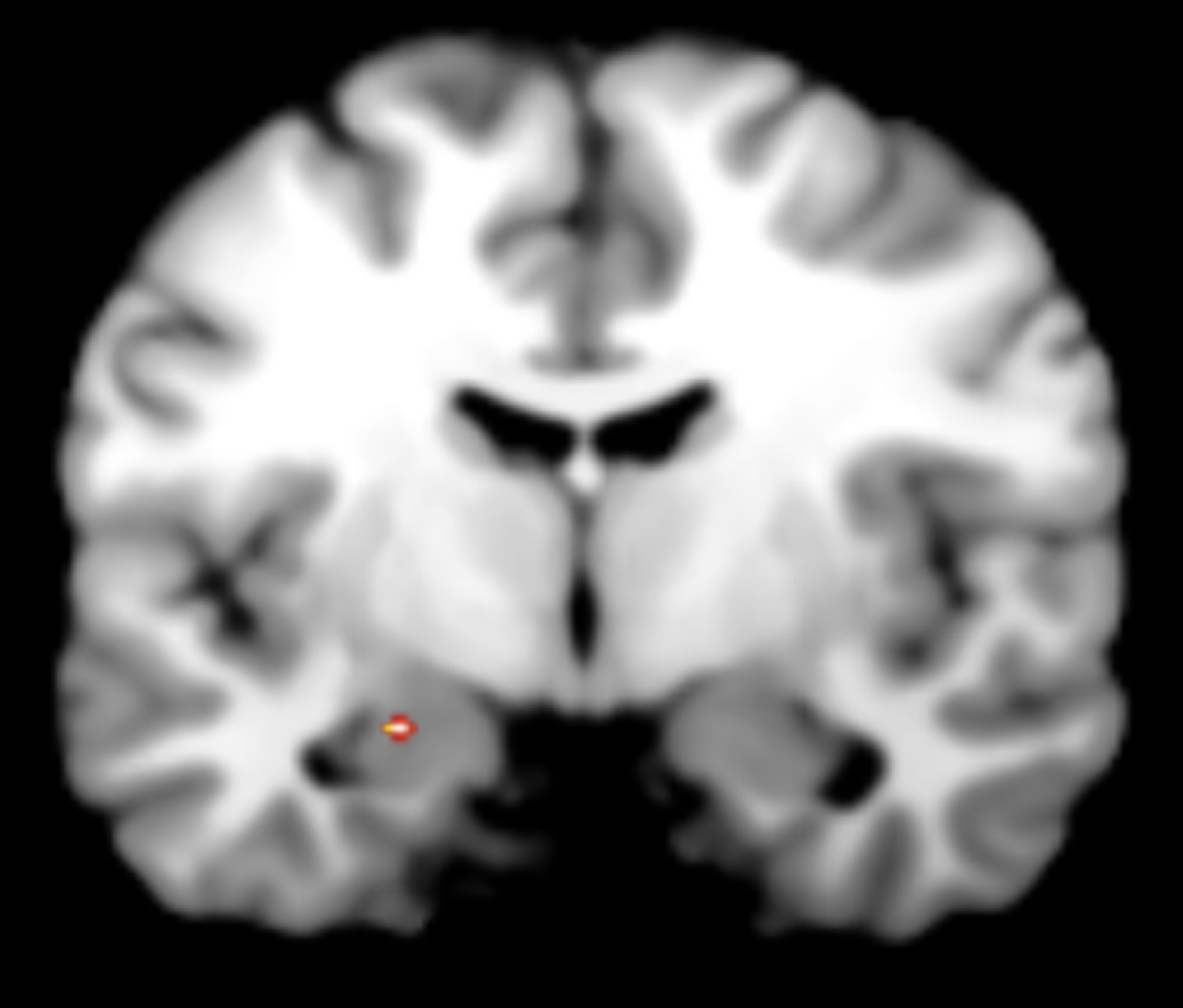
Correlations
For the first run there was a trend towards a negative correlation between VAS ratings and the right VMPFC (Pearson’s r = –0.42, p = 0.06) during the induction. In other words, as self-rated anger increased, activation in the right VMPFC decreased. There was also a negative correlation between VAS ratings and bilateral VMPFC activation (left VMPFC: Pearson’s r = –0.54, p = 0.015; right VMPFC: Pearson’s r = –0.47, p = 0.035) for the second run during induction indicating that increased self-rated anger was associated with lower VMPFC activation. In the second run imagery period, anger ratings were again negatively correlated with activation in left VMPFC (Pearson’s r = –0.57, p = 0.009).
DISCUSSION
Previously, most studies using autobiographical scripts to induce emotion were done in PET. This study successfully brought an emotion-induction paradigm from PET to fMRI and was able to use the greater temporal resolution of fMRI to clarify the temporal relation between the amygdala and VMPFC in the experience of anger. We had hypothesized that the lack of amygdala activation reported in neuroimaging studies could be due to an early but not sustained involvement of the amygdala during anger. We found that there was indeed an early increase of bilateral amygdala activation during anger induction, which was then followed by increased left VMPFC activation during the anger imagery period.
We also found negative correlations between self-rated anger and activation in the VMPFC. In other words, as self-rated anger increased, activation in the VMPFC decreased. These results support the idea that there is mutually inhibitory relationship between the amygdala and VMPFC [35, 36, 45]. Specifically, the amygdala’s early activation suggests that the amygdala might be more involved in the initial experience of anger while the VMPFC is more involved in the evaluation of that anger and in the inhibition of an impulsive response.
A unique feature of this study is that subjects were asked to provide self-relevant, autobiographical information for the anger induction and imagery paradigm. Most prior research has investigated the neural correlates of anger only when participants are viewing standard angry faces. Our use of self-relevant stimuli might have better ecological validity in terms of potentially studying the more realistic experience of self-generated and personally relevant anger processes. A clear future direction is to investigate the time course of amygdala and VMPFC activation during self-generated anger in psychiatric populations that have difficulty controlling their anger.
One limitation of the present study is that it was not designed to assess sex differences in anger. Future studies would be desirable to detect those differences. Also, the current sample scored slightly lower than average on anger reactivity; however, these scores did not significantly correlate with VMPFC or amygdala activation. Future work is needed to understand if this discrepancy was related to the fact that participants were asked to think of their most anger-provoking events and generate anger that may be an atypical experience for them in real life.
Overall, our study combined the advantages of using an actual event that has affected behavior with the superior temporal resolution of fMRI. Our findings suggest that it is important to look at the specific time course of amygdala activation when trying to understand the neural correlates of anger, which may not be possible with PET imaging. We also found that the VMPFC modulates early amygdala activation in healthy controls, and this modulation results in decreased self-rated anger.
CONFLICTS OF INTEREST
Dr. Dougherty has served as a consultant to and received honoraria from Medtronic, received travel support from Roche, and has received grant/research support from Medtronic, Cyberonics, Eli Lilly, and Roche.
Dr. Rauch has participated in research funded by Medtronic and Cyberonics. He has also received royalties for APPI and Oxford University Press.
Dr. Deckersbach’s research has been funded by NIMH, NARSAD, TSA, OCF, Tufts University and the Depression and Bipolar Disorder Alternative Treatment Foundation. He has received honoraria, consultation fees and/or royalties from the MGH Psychiatry Academy, BrainCells Inc., Systems Research and Applications Corporation, Boston University, the Catalan Agency for Health Technology Assessment and Research, the National Association of Social Workers Massachusetts, the Massachusetts Medical Society, Tufts University, NIDA, NIMH, and Oxford University Press. He has also participated in research funded by NIH, NIA, AHRQ, Janssen Pharmaceuticals, The Forest Research Institute, Shire Development Inc., Medtronic, Cyberonics, Northstar, and Takeda.
Ms. Chou and Dr. Buhlman have no conflicts of interest to report.
ACKNOWLEDGMENTS
This study was supported by Mentored Patient Oriented Research Career Development Award from the National Institute of Mental Health, Bethesda, MD (D.D., MH01735) and a Young Investigator Award by the National Alliance for Research in Schizophrenia and Depression (T.D.). This work was also supported by the National Science Foundation Graduate Research Fellowship Program (T.C., DGE1144152).
REFERENCES
[1] | Iribarren C , Sidney S , Bild DE , Liu K , Markovitz JH , Roseman JM , et al. Association of hostility with coronary artery calcification in young adults: The CARDIA study. Coronary Artery Risk Development in Young Adults. JAMA. (2000) ;283: :2546–51. |
[2] | Smith TW , Glazer K , Ruiz JM , Gallo LC . Hostility, anger, aggressiveness, and coronary heart disease: An interpersonal perspective on personality, emotion, and health. J Pers. (2004) ;72: (6):1217–70. |
[3] | Chen YY , Gilligan S , Coups EJ , Contrada RJ . Hostility and perceived social support: Interactive effects on cardiovascular reactivity to laboratory stressors. Ann Behav Med. (2005) ;29: (1):37–43. |
[4] | Julkunen J , Ahlstrom R . Hostility, anger, and sense of coherence as predictors of health-related quality of life. Results of an ASCOT substudy. J Psychosom Res. (2006) ;61: (1):33–9. |
[5] | Posternak MA , Zimmerman M . Anger and aggression in psychiatric outpatients. The Journal of Clinical Psychiatry. (2002) ;63: :665–72. |
[6] | Dougherty DD , Shin LM , Alpert NM , Pitman RK , Orr SP , Lasko M , et al. Anger in healthy men: A PET study using script-driven imagery. Biological Psychiatry. (1999) ;46: :466–72. |
[7] | Damasio AR , Grabowski TJ , Bechara A , Damasio H , Ponto LL , Parvizi J , et al. Subcortical and cortical brain activity during the feeling of self-generated emotions. Nature Neuroscience. (2000) ;3: :1049–56. |
[8] | Dougherty DD , Rauch SL , Deckersbach T , Marci C , Loh R , Shin LM , et al. Ventromedial prefrontal cortex and amygdala dysfunction during an anger induction positron emission tomography study in patients with major depressive disorder with anger attacks. Archives of General Psychiatry. (2004) ;61: :795–804. |
[9] | Nomura M , Ohira H , Haneda K , Iidaka T , Sadato N , Okada T , et al. Functional association of the amygdala and ventral prefrontal cortex during cognitive evaluation of facial expressions primed by masked angry faces: An event-related fMRI study. NeuroImage. (2004) ;21: :352–63. |
[10] | Goyer PF , Andreason PJ , Semple WE , Clayton AH , King AC , Compton-Toth BA , et al. Positron-emission tomography and personality disorders. Neuropsychopharmacology: Official publication of the American College of Neuropsychopharmacology. (1994) ;10: :21–8. |
[11] | Brower MC , Price BH . Neuropsychiatry of frontal lobe dysfunction in violent and criminal behaviour: A critical review. J Neurol Neurosurg Psychiatry. (2001) ;71: (6):720–6. |
[12] | Soderstrom H , Hultin L , Tullberg M , Wikkelso C , Ekholm S , Forsman A . Reduced frontotemporal perfusion in psychopathic personality. Psychiatry Res. (2002) ;114: :81–94. |
[13] | Yang Y , Raine A . Prefrontal structural and functional brain imaging findings in antisocial, violent, and psychopathic individuals: A meta-analysis. Psychiatry Res. (2009) ;174: (2):81–8. |
[14] | Whalen PJ . Fear, Vigilance, and ambiguity: Initial neuroimaging studies of the human amygdala. Current Directions in Psychological Science. (1998) ;7: :177–188. |
[15] | Armony JL , Dolan RJ . Modulation of spatial attention by fear-conditioned stimuli: An event-related fMRI study. Neuropsychologia. (2002) ;40: :817–26. |
[16] | Pourtois G , Grandjean D , Sander D , Vuilleumier P . Electrophysiological correlates of rapid spatial orienting towards fearful faces. Cerebral Cortex. (2004) ;14: :619–33. |
[17] | Deckersbach T , Dougherty DD , Savage C , McMurrich S , Fischman AJ , Nierenberg A , et al. Impaired recruitment of the dorsolateral prefrontal cortex and hippocampus during encoding in bipolar disorder. Biological Psychiatry. (2006) ;59: :138–46. |
[18] | Levenston GK , Patrick CJ , Bradley MM , Lang PJ . The psychopath as observer: Emotion and attention in picture processing. J Abnorm Psychol. (2000) ;109: (3):373–85. |
[19] | Flor H , Birbaumer N , Hermann C , Ziegler S , Patrick CJ . Aversive Pavlovian conditioning in psychopaths: Peripheral and central correlates. Psychophysiology. (2002) ;39: (4):505–18. |
[20] | Davis M . The role of the amygdala in fear and anxiety. Annu Rev Neurosci. (1992) ;15: :353–75. |
[21] | Angrilli A , Mauri A , Palomba D , Flor H , Birbaumer N , Sartori G , et al. Startle reflex and emotion modulation impairment after a right amygdala lesion. Brain: A Journal of Neurology. (1996) ;119. |
[22] | Blair RJR , Coles M . Expression recognition and behavioural problems in early adolescence. Cognitive Development. (2000) ;15: :421–34. |
[23] | Fine C , Blair RJR . The cognitive and emotional effects of amygdala damage. Neurocase. (2000) ;6: :435–50. |
[24] | Blair RJ , Colledge E , Murray L , Mitchell DG . A selective impairment in the processing of sad and fearful expressions in children with psychopathic tendencies. Journal of Abnormal Child Psychology. (2001) ;29: :491–8. |
[25] | Stevens D , Charman T , Blair RJ . Recognition of emotion in facial expressions and vocal tones in children with psychopathic tendencies. The Journal of Genetic Psychology. (2001) ;162: :201–11. |
[26] | van Elst LT , Woermann FG , Lemieux L , Thompson PJ , Trimble MR . Affective aggression in patients with temporal lobe epilepsy: A quantitative MRI study of the amygdala. Brain: A Journal of Neurology. (2000) ;123: (Pt 2):234–43. |
[27] | Kiehl KA , Smith AM , Hare RD , Mendrek A , Forster BB , Brink J , et al. Limbic abnormalities in affective processing by criminal psychopaths as revealed by functional magnetic resonance imaging. Biological Psychiatry. (2001) ;50: :677–84. |
[28] | Costafreda SG , Brammer MJ , David AS , Fu CH . Predictors of amygdala activation during the processing of emotional stimuli: A meta-analysis of 385 PET and fMRI studies. Brain Res Rev. (2008) ;58: (1):57–70. |
[29] | Sergerie K , Chochol C , Armony JL . The role of the amygdala in emotional processing: A quantitative meta-analysis of functional neuroimaging studies. Neurosci Biobehav Rev. (2008) ;32: (4):811–30. |
[30] | Fusar-Poli P , Placentino A , Carletti F , Landi P , Allen P , Surguladze S , et al. Functional atlas of emotional faces processing: A voxel-based meta-analysis of 105 functional magnetic resonance imaging studies. J Psychiatry Neurosci. (2009) ;34: (6):418–32. |
[31] | Lindquist KA , Wager TD , Kober H , Bliss-Moreau E , Barrett LF . The brain basis of emotion: A meta-analytic review. Behav Brain Sci. (2012) ;35: (3):121–43. |
[32] | Chiba T , Kayahara T , Nakano K . Efferent projections of infralimbic and prelimbic areas of the medial prefrontal cortex in the Japanese monkey, Macaca fuscata. Brain Research. (2001) ;888: :83–101. |
[33] | Stefanacci L , Amaral DG . Some observations on cortical inputs to the macaque monkey amygdala: An anterograde tracing study. The Journal of Comparative Neurology. (2002) ;451: :301–23. |
[34] | Price JL . Comparative aspects of amygdala connectivity. Annals of the New York Academy of Sciences. (2003) ;985: :50–8. |
[35] | Hariri AR , Bookheimer SY , Mazziotta JC . Modulating emotional responses: Effects of a neocortical network on the limbic system. Neuroreport. (2000) ;11: :43–8. |
[36] | Milad MR , Quirk GJ . Neurons in medial prefrontal cortex signal memory for fear extinction. Nature. (2002) ;420: :70–4. |
[37] | Wright CI , Fischer H , Whalen PJ , McInerney SC , Shin LM , Rauch SL . Differential prefrontal cortex and amygdala habituation to repeatedly presented emotional stimuli. Neuroreport. (2001) ;12: :379–83. |
[38] | Phan KL , Wager T , Taylor SF , Liberzon I . Functional neuroanatomy of emotion: A meta-analysis of emotion activation studies in PET and fMRI. NeuroImage. (2002) ;16: :331–48. |
[39] | Fischer H , Wright CI , Whalen PJ , McInerney SC , Shin LM , Rauch SL . Brain habituation during repeated exposure to fearful and neutral faces: A functional MRI study. Brain Research Bulletin. (2003) ;59: :387–92. |
[40] | Williams LM , Brown KJ , Das P , Boucsein W , Sokolov EN , Brammer MJ , et al. The dynamics of cortico-amygdala and autonomic activity over the experimental time course of fear perception. Cognitive Brain Research. (2004) ;21: :114–23. |
[41] | Strauss MM , Makris N , Aharon I , Vangel MG , Goodman J , Kennedy DN , et al. fMRI of sensitization to angry faces. NeuroImage. (2005) ;26: :389–413. |
[42] | First MB , Spitzer RL , Gibbon M , Williams JBW . Structured Clinical Interview for DSM-IV-TR Axis I Disorders, Research Version, Patient Edition (SCID-I/P) 2002. |
[43] | Spielberger. STAXI-2 State-trait anger expression inventory-2; Professional manual; (1999) . |
[44] | Maldjian JA , Laurienti PJ , Kraft RA , Burdette JH . An automated method for neuroanatomic and cytoarchitectonic atlas-based interrogation of fMRI data sets. NeuroImage. (2003) ;19: :1233–9. |
[45] | Carmichael ST , Price JL . Limbic connections of the orbital and medial prefrontal cortex in macaque monkeys. The Journal of Comparative Neurology. (1995) ;363: :615–41. |