Seasonal changes in white strawberry: Effect on aroma, phenolic compounds and its biological activity
Abstract
BACKGROUND:
The native Chilean white strawberry (Fragaria chiloensis ssp. chiloensis f. chiloensis) is a semi-domesticated crop that has a characteristic aroma and flavor and a low production in southern Chile. However, edaphoclimatic conditions can influence on fruit quality attributes and its health benefits. Establishing a link between seasonal changes and aroma or biological activity require detailed research in exploring bioactive compounds.
OBJECTIVE:
The present work assessed how seasonal and local changes varied the content of bioactive compounds and therefore change their aromatic quality and the response of biological activity.
METHODS:
White Strawberry from two seasons and two locations were investigated; FCC1, FCC2 (Fragaria chiloensis from Contulmo, 2017 and 2018 season, respectively), FCP1, FCP2 (F. chiloensis from Purén, 2017 and 2018 season, respectively). Measurement of changes on volatile compounds were studied by SPME/GC-MS. Analyses of variations on phenolic compounds were investigated by HPLC-DAD-ESI-MSn with total polyphenolic content and antioxidant capacity by using DPPH• and ORAC assays by spectrophotometric and fluorimetric methods. The relationship between different concentrations of compounds and in vitro biological activity including inhibitory tests for α-glucosidase and acetylcholinesterase were analyzed.
RESULTS:
In the fruit extracts, 38 volatiles and 27 phenolic compounds were identified detecting differences among the samples, being affected by climatic conditions and location. The total content of ellagic acid and its derivatives was 6.54 mg 100 g–1 FW for FCC1, showing statistical differences with respect to the rest strawberries. Nonetheless, the antioxidant capacity tests revealed high antioxidant capacity for all samples, being FCP2 the significantly highest activity (3314μmol Trolox 100 g–1 FW by DPPH• assay) compared to the rest of locations and seasons. Additional inhibitory tests α-glucosidase and acetylcholinesterase showed statistically differences due to seasonal and location changes where was observed higher ellagic acid derivates content and bioactivity. The Chilean white strawberry extracts were effective inhibitors of α-glucosidase (non-competitive) and acetylcholinesterase (competitive) activities, respectively, presenting FCC1 the most potent inhibitory effects.
CONCLUSIONS:
A higher ellagic acid content in Chilean white strawberry, affected by seasonal and location changes, influenced on the biological activity potential. Therefore, the relatively high antioxidant capacity, phytochemical composition and biological activity potential, of these aromatic fruits, offer a great opportunity for the rural developments, however it will be necessary to implement good practices that would guarantee batch-to-batch replicability for quality and composition of these foods for the future.
1Introduction
In the last decades, strategies for the preservation of plant biodiversity and the genetic resources in every country, as well as establishing sustainable agriculture practices to face the world challenges of the future of food supply are of urgent need [1]. The current interest and diversification of human diets and the changing environments because of the global warming and climate changes, together with the rapid growth of the population is switching the perspective to native species and natural resources to be more adaptable for the new conditions [2]. In addition, over the past several years, a great interest in producing foods enriched in bioactive compounds, to provide additional health-promoting benefits to the nutritional value, has been also triggering the research [3, 4].
Regarding to this characteristic, inhibition of α-glucosidase, a strategic enzyme that metabolize carbohydrates in the digestion, could delay the breakdown of oligosaccharides reducing glucose absorption and therefore decreasing postprandial hyperglycemia [5, 6]. Polyphenols of berries have demonstrated inhibitory effects of this enzyme and have been proved on type II diabetes rodent models to significantly lower the post-prandial glycaemia and the insulin response [7]. Also, polyphenols can act on neurodegenerative diseases such as Alzheimer’s Disease (AD) since one of the key point for improving cholinergic activity in the brain is the inhibition of the enzymes responsible for the breakdown of acetylcholine (ACh), by the enzyme acetylcholinesterase (AChE) [8]. In this way, ACh is not transformed to acetyl-CoA and choline, thus increasing the accessibility of ACh to stimulate nicotinic and muscarinic receptors within the brain. Bioactive compounds from foods, specially the polyphenols, displayed bioactivity on AChE [9] as well as bioavailability in several types of body tissues [10]. Therefore, the study of the interaction of polyphenols with different enzymes helps us to predict the potentiality and biological activity of some foods.
Native fruits are a rich source of antioxidants but they are susceptible to get lost or disappear due to different causes [11–13]. Fragaria chiloensis ssp. is an endemic and wild species of Fragaria from southern Chile that produces light red or almost white strawberries. In addition, in the current conditions, this plant produces fruit only once a year. This fruit stands out for its pale color, pleasant taste and aroma and is known to be one of the progenitors of the worldwide known commercial red strawberry (Fragaria x ananassa Duch.) [13]. Volatile compounds represent an aspect of fruit quality since they are responsible for aroma and flavor, and in the case of white strawberries it has been poorly identified, in spite of being one of their most important organoleptic characteristics in this fruit [14]. In addition, this aroma has never been identified as a function of changes in compounds determined by edaphoclimatic conditions until now.
Related to phenolic compounds, several studies have characterized the phenolic profile of this native species [12, 13, 15], showing ellagitannins, ellagic acid, quercetin, kaempferol and isorhamnetin derivatives [15–18]. The ellagitannins, proanthocyanidins and flavonoids exhibit a wide variety of activities including radical scavenging [19], cholinesterase inhibition [20], and anti-hyperglycemic activity [7, 21]. However, differences in concentration of compounds according to the growing conditions such as environmental conditions and crop management could be found as well as in relation to its biological activity. Therefore, it is important to study the fruit present in different cultivation sites, countries and geographical areas [22, 23] in a climate change context since stress conditions have been correlated with changing in the activity of superoxide dismutase, contents of glutathione, phenols and anthocyanins [24]. Nevertheless, changes in the climate, mainly increasing temperatures and decreasing precipitations will produce changes in the production of Chilean white strawberry and variation of the bioactive compounds. Therefore, inclusion of native berries in agricultural production will be a new strategy to reconvert the production procedure in a more profitable system [25].
Considering climate conditions, we can hypothesize that the production of Chilean white strawberry will present a valuable chemical profile and also biological activity to continue to safe this value genetic material, however seasonal and location changes could vary its biological activity. Based on the above, consequently, in this study, the purpose was to update and identify the changes in volatile compounds and variations in profile of the hydromethanolic extract of F. chiloensis spp. chiloensis f. chiloensis collected from two different geographical places where it mainly growths and evaluating the effect on α-glucosidase and acetylcholinesterase inhibitors, their inhibition ratio and mechanisms studying their enzymatic kinetics. This information will contribute to deepen on the phytochemical changes that the crop undergoes depending on the location and the year and protect F. chiloensis setting the Chilean white strawberry in a best situation to avoid its total disappearance, benefit the rural sector and provide new knowledge for establishing F. chiloensis as a high value-added functional food by fulfilling a beneficial role for health.
2Materials and methods
2.1Plant material
Fruits of F. chiloensis ssp. chiloensis f. chiloensis (FCC1 as F. chiloensis ‘Contulmo’ 2017 and FCC2 as F. chiloensis ‘Contulmo’ 2018) were harvested in a commercial plantation located in Pichihuillinco, Contulmo, Province of Arauco, Biobío Region, Chile (S 38° 04’ 8.6”, W 73° 14’ 2.96”) at 605 m above sea level. Ripe fruits of F. chiloensis ssp. chiloensis f. chiloensis (FCP1 as F. chiloensis ‘Purén’2017 and FCP2 as F. chiloensis ‘Purén’2018) were harvested in a commercial plantation located in Manzanal Alto, Purén, Province of Malleco, Araucanía Region, Chile (S 38° 03’ 10.2”, W 73° 11’ 25.1”) at 500 m above sea level. All the cultivars, chilean strawberry used in this study were still produced by local farmer with limited agriculture management, without technification nor agrochemicals. Only fungicides were used in all treatments and in a very limited way. F. chiloensis ssp. chiloensis f. chiloensis fruits were harvested in maturity in December 2017 and December 2018. The samples were immediately frozen at –80°C until analyzed. The strawberries were arranged to remove damaged, poor quality fruit and to obtain a uniform sample in size and color. All the analysis described into the manuscript consisted in six replications for each treatment where 50 grams of homogenized sample (approximately 10 fruits) were used for each replication.
2.2Headspace solid phase micro extraction of Chilean white strawberry volatile compounds
The extraction of volatile compounds from Chilean white strawberry samples was accomplished by using the headspace solid phase micro extraction (HS-SPME), which was operated by the CombiPAL auto-sampler software (Agilent Technologies, Palo Alto, CA, USA). Briefly, 2 g of smashed fruit was placed in 20 mL amber glass screw-capped SPME vials followed by an incubation in a shaking heated cube at 400 rpm at 40°C for 20 min. A Divinylbenzene/Carboxen/Polydimethylsiloxane (DVB/CAR/PDMS) SPME fiber (50/30μm film thickness and 1 cm length; Supelco, Bellefonte, PA, USA) was used. An initial pre-condition of the SPME fiber was conducted at 270°C for 30 min. The fiber was injected into an Agilent 7890B gas chromatograph (Agilent, Palo Alto, CA, USA) containing a 30 m×0.25 mm fused silica HP-5 ms column. The chromatographic conditions used were inlet 250°C; column 40°C for 2 min followed by ramping at 5°C min–1 to 250°C. Mass spectral analyses were carried out with an Agilent 5977A instrument (Agilent, Palo Alto, CA, USA). The scan mass range extended from m/z 20 to 400. Mass spectra of VOCs were compared with those obtained from the NIST library, and identifications were confirmed using commercially available standard compounds. Chemicals (with purities above 99%) were purchased from Sigma-Aldrich (St. Louis, Missouri, USA) to identify some compounds released.
2.3Extraction of phenolic compounds
Chilean white strawberries fruit sepals were removed and 50 g of berries were cut into small pieces and homogenized in a disperser Ultra-Turrax at room temperature. 0.5 g of processed fruits were weighed and was thoroughly mixed with 5 mL of extractive solvent methanol: water: formic acid (25:24:1, v/v/v). The extraction was assisted by ultrasound bath for 60 min, followed by a maceration with stirring for 24 h in the dark at –20°C to return to the ultrasound bath for 60 min more. The resulting extracts were centrifuged, and the supernatant was collected and filtrated through a 0.45μm size pore membrane [26].
2.4Bioactive components analysis
2.4.1Determinations of Total Phenolic Content
Total phenolics content (TPCs) were determined by the Folin and Ciocalteu’s reagent method [27]. Briefly, the samples were prepared adding 750μL of Folin Ciocalteu 1N reagent, 750μL of 20% sodium carbonate and 500μL of the extracts of chilean strawberries. In addition, a blank was also prepared using distilled water to replace the sample. All of them were maintained for two hours in the dark and absorbance was measured through a Thermo Scientific UV-Vis Orion AquaMate 8000 spectrophotometer (Madrid, Spain). Results were expressed as mg of gallic acid equivalents per 100 grams of fresh fruits (mg GAE 100 g–1 FW).
2.4.2Identification of phenolics compounds by HPLC-DAD-ESI-MSn and quantification by HPLC-DAD
Analyses of phenolic compounds from Chilean white strawberry were carried out on a Luna C18-100A column (250×5 mm. 5μm particle size; Phenomenex, Macclesfield, UK). Formic acid 1% and acetonitrile were used as mobile phases A and B, respectively, with a flow rate of 1 mL min–1. Chromatographic conditions and mass spectrometry analyses were carried out as described by Gironés-Vilaplana et al.[9]. The quantification of compounds was through a HPLC system equipped with a Chromolith® HighResolution RP-18 endcapped column (100×4.6 mm; Merckmillipore, Darmstadt, Germany) and the same conditions for HPLC-DAD-ESI-MSn. The injection volume was 20μL. Chromatograms were recorded at 254, 280, 320, 360 and 520 nm and the derivatives of ellagic acid, flavonols and anthocyanins were quantified using ellagic acid, quercetin and pelargonidin 3-O-glucoside as standard. The quantified compounds were expressed as mg equivalents per 100 grams of fresh fruits (mg Eq 100 g–1 FW). All reagents were of analytical HPLC grade Merck, Darmstadt, Germany) and standards were from Sigma Chemical Co. (St Louis, MO, USA).
2.4.3Determination of Antioxidant Capacity
The antioxidant capacity of berry extracts was evaluated in vitro by two methods.
DPPH• Assays:
DPPH• (2,2-diphenyl-1-picrylhydrazyl radical) reduction test was measured as previously informed [28]. A 0.1 mL aliquot of sample or Trolox was mixed with DPPH• radical in methanol. The mixture was incubated at 25°C in the dark for 1 h, and the absorbance of the methanolic DPPH•-dye was measured spectrophotometrically at 517 nm. The results were expressed as micromoles of Trolox equivalents per 100 grams of fresh fruit (μmol TE 100 g–1 FW).
ORAC Assays:
The achievement of ORAC test is based on a previously reported method with light modifications [29]. Briefly, 25μL of sample or Trolox were mixed with 150μL of Fluorescein and the mixture was incubated at 37°C in the dark for 30 min. Finally, 25μL of AAPH (2,2′-Azobis(2-methylpropionamidine)) were added. Fluorescence was analyzed for 60 min (excitation wavelength was set at 485 nm; emission wavelength at 528 nm) at 37°C. Measurements were taken in triplicate in a Synergy HTX Multi-Mode Microplate Reader (Vermont, U.S.A). The results were calculated as ORAC values using the differences between the blank and the sample areas under the fluorescein decay curve. Results were expressed as micromoles of Trolox equivalents per 100 grams of fresh fruit (μmol TE 100 g–1 FW).
2.5Inhibition of α-glucosidase activity
α-Glucosidase inhibitory was evaluated by modification of a previously reported method [30]. Briefly, each well contained 170μL potassium phosphate buffer (100 mmol L–1, pH 7.5), 25μL of extract (diluted 1:10 in buffer) and 25μL of the enzyme solution (1 U mL–1) from Saccharomyces cerevisiae (G5003; EC 3.2.1.20). All reagents were from Sigma Chemical Co. (St Louis, MO, USA). Measurements assay were taken in triplicate by using 96-well micro plates in a Synergy HTX Multi-Mode Microplate Reader (Vermont, U.S.A). The plates were incubated at 37°C for 20 min. The reaction was initiated by the addition of 30μL of 2.5 mmol L–1 4-nitrophenyl α-D-glucopyranoside (PNP-G) and then reaction mixture was incubated for 10 min at 37°C. The absorbance of 4-nitrophenol released from 4-nitrophenyl α-D-glucopyranoside at 405 nm was measured and a control was used without inhibitor. The inhibition was expressed as mg mL–1. In order to study the inhibitory effects of hydromethanolic extracts of F. chiloensis on α-glucosidase, various concentrations ranged from 0.7 to 0.05 mmol L–1 of substrate in the presence of 0.1 U mL–1 α-glucosidase were used. The absorbance was read at 405 nm every minute for 20 min. Then the double reciprocal Lineweaver-Burk plots were used to determine the inhibition mode by calculating the inhibition Michaelis-Menten constant (Km) and maximum reaction rate (Vmax) through linear regression.
2.6Inhibition of acetylcholinesterase activity
Acetylcholinesterase (AChE) inhibitory activity was assessed by modification of a previously reported procedure by Ellman et al. [31]. Briefly, each well contained 105μL potassium phosphate buffer (100 mmol L–1, pH 7.5), 25μL of extract (diluted 1:10 in buffer) 50μL of DNTB solution (3.5 mmol L–1) (5,5′-Dithiobis(2-nitrobenzoic acid)) and 30μL of the enzyme solution (0.75 U mL–1) from Electrophorus electricus (C3389; EC 3.1.1.7). All reagents were from Sigma Chemical Co. (St Louis, MO, USA). Measurements assay were taken in triplicate by using 96-well micro plates in a Synergy HTX Multi-Mode Microplate Reader (Vermont, U.S.A). The plates were incubated at 37°C for 20 min. The reaction was initiated by the addition of 40μL of 1.8 mmol L–1 acetylthiocholine iodide and then reaction mixture was incubated for 15 min at 37°C. The absorbance was read at 405 nm and a control was used without inhibitor. The inhibition was expressed as mg mL–1. In order to study the inhibitory effects of hydromethanolic extracts of F. chiloensis on acetylcholinesterase, various concentrations (0.7, 0.5, 0.3, 0.1, and 0.05 mmol L–1) of substrate in presence of 0.09 U mL–1 acetylcholinesterase were used. The absorbance was read at 405 nm every minute for 20 min. Then the double reciprocal Lineweaver-Burk plots were used to determine the inhibition mode by calculating the inhibition Michaelis-Menten constant (Km) and maximum reaction rate (Vmax) through linear regression.
2.7Statistical analysis
IBM SPSS Statistics 25 software package (SPSS Inc., Chicago, IL, USA) was used for the statistical analysis, using one-way analysis of variance (ANOVA), with a completely random design (n = 4), and Tukey test at a level of significance P < 0.05. Principal Component Analysis (PCA) was determined using the correlation matrix and varimax analysis with Kaiser normalization; subsequent comparison of scores for principal components (PCs) was carried out by t-test or ANOVA with Tukey’s multiple comparison test. For the inhibition enzyme assay calculated use the GraphPad Prism v. 6.1 software (GraphPad Prism® software, Inc., US). Each experiment was repeated six times and the results reported are the means of the trials±SD.
3Results and discussion
3.1Identification of volatiles from F. chiloensis by SPME/GC-MS
Determination of volatile compounds from F. chiloensis shows an interesting consumer trait since this fruit presents a characteristic and pleasant aroma compared to red strawberries [32]. Volatile compounds identified in Chilean white strawberries are presented in Table 1. Ten alcohols, 2 ketones, 5 aldehydes, 6 esters, 11 terpenes and others compounds until 38 were identified for each sample. From the volatile compounds already described, it was found that 1-hexanol, 1-octanol and hexyl acetate, benzyl acetate and 2-heptanone have been reported [14] for the development and ripening of the fruit. In addition, Chilean strawberry has volatile compounds in common with some varieties of F. x ananassa such as hexanal, linalool, 1-octanol, 2 phenylethanol, L-α-terpineol and γ-decalactone.
Table 1
Changes in volatiles from F. chiloensis by SPME/GC-MS during two seasons in Purén and Contulmo locations
Peak a | RT (min) | Identified Compounds | |||
FCC1 | FCC2 | FCP1 | FCP2 | ||
nd | * | nd | nd | 6.1 | Octamethylcyclotetrasiloxane# |
* | * | * | * | 6.8 | Thiophene |
* | * | * | nd | 7.3 | Hexanal |
* | * | * | * | 8.6 | Decamethylcyclopentasiloxane# |
* | * | * | * | 9.5 | 2-Heptanone |
nd | * | * | nd | 9.7 | D-Limonene |
nd | * | * | nd | 10.2 | Eucalyptol |
* | * | * | * | 10.4 | 2-Hexenal |
* | * | * | nd | 11.2 | Styrene |
nd | * | * | * | 11.5 | Hexyl acetate |
* | * | * | * | 12.8 | Diethyldi(4-acetylphenoxy)silane# |
nd | nd | nd | * | 13.3 | γ-Terpinene |
* | * | * | * | 13.7 | 1-Hepten-3-one |
* | * | * | * | 13.9 | 3,6-dimetoxy-9-(2-phenylethinyl)-Fluoren-9-ol# |
* | * | * | nd | 14.7 | 1-Hexin-3-ol |
* | * | * | * | 15.0 | 1-Hexanol |
* | nd | * | nd | 16.6 | 1-Octen-3-ol |
* | * | * | * | 17.0 | p-Mentan-3-one |
* | * | * | * | 18.1 | Tetradecamethylcycloheptasiloxane# |
* | * | nd | * | 18.4 | 2-ethyl-1-hexanol |
* | * | * | * | 19.2 | Benzaldehyde |
* | * | * | * | 22.1 | Linalool |
* | nd | nd | nd | 23.4 | (E)-2-nonenal |
* | nd | nd | nd | 24.5 | Isobornyl acetate |
* | * | * | nd | 26.9 | 1-octanol |
* | nd | * | nd | 28.4 | Methyl decanoate |
* | * | * | * | 29.5 | Estragole |
nd | nd | * | nd | 31.7 | (E)-2-nonen-1-ol |
* | nd | nd | * | 36.3 | trans-Dihydrocarvone |
* | nd | * | nd | 36.6 | α-Amorphene |
* | nd | * | * | 38.7 | L-α-Terpineol |
* | * | * | * | 40.9 | Benzyl acetate |
* | * | * | * | 43.3 | D-Carvone |
* | nd | * | nd | 44.5 | Methyl dodecanoate |
* | * | * | * | 45.8 | Anethole |
* | nd | * | * | 47.1 | Ethyl dodecanoate |
* | * | * | * | 48.5 | Benzyl alcohool |
* | nd | * | * | 50.0 | 2-Phenylethanol |
* | nd | * | nd | 50.5 | Phenol |
* | * | * | * | 51.6 | 3-Phenylpropanol |
* | nd | * | * | 53.4 | 1,1’-[methylenbis(oxy)]bis-hexane |
nd | nd | * | nd | 54.0 | Cyclododecane |
* | * | * | * | 55.4 | 3-Phenylpropanal |
* | nd | * | * | 57.6 | γ -Decalactone |
* | nd | * | nd | 58.4 | Hept-4-il isobuthyl phtalate# |
nd: not detected, *: detected; aFCC1 is F. chiloensis ‘Contulmo’ 2017, FCC2 is F. chiloensis ‘Contulmo’ 2018, FCP1 is F. chiloensis ‘Purén’ 2017, FCP2 is F. chiloensis ‘Purén’ 2018. #Putative compounds from the column and fiber.
Among the concentrations in volatile compounds from Chilean strawberry (Table 1), it was observed that 2-heptanone, 1-hepten-3-one, 1-hexin-3-ol, 1-hexanol and estragole were detected in higher concentrations for the FCC2 strawberry. In contrast, for the FCC1, the major volatile compounds detected were D-carvone, anethole, benzyl alcohol, 3-phenylpropanol and 3-phenylpropanal, a similar case for the FCP1, both harvested in the same season. For FCP2 the main volatile compounds found were 2-hexenal, 1-hepten-3-one, benzyl alcohol, 3-phenylpropanol and 3-phenylpropanal. Thus, the main compounds for FCP1 and FCP2 were benzyl alcohol, 3-phenylpropanol and 3-phenylpropanal as well as for the FCC1. In addition, it is quite significant to note that some compounds are only detected during the first season such as 1-octen-3-ol, methyl decanoate, α-amorphene, methyl dodecanoate and phenol. Consequently, a reduction of the content of volatiles with excessive rain in the second season was observed. Others compounds strongly depended on locality and seasonality. With these results, the changes in concentrations for the different volatile compounds are presented. Therefore, location and season affected to composition of the aroma in white strawberry varying its aromatic properties.
3.2Bioactive compounds analysis
3.2.1Identification and quantification of polyphenolic compounds in Fragaria chiloensis
Twenty-nine signals were identified by comparing their relative retention times and spectral data (Supplementary Data 1). Twenty-four compounds were identified for F. chiloensis ssp. chiloensis f. chiloensis, some of these already described by Simirgiotis et al. [15] and Thomas-Valdés et al. [18]. However, changes in the concentrations were found in this study influenced by seasonal and location conditions.
The content of the major anthocyanins of F. chiloensis (Table 2) was cyanidin 3-O-glucoside with 1.23 to 5.66 mg Eq 100 g–1 FW followed by pelargonidin 3-O-glucoside 0.10 to 0.43 mg Eq 100 g–1 FW. The latter anthocyanin did not present significant differences for FCC1 and FCC2 nor FCP1 and FCP2. The cyanidin 3-O-glucoside content presented significant differences among season 1 and season 2. The two samples harvested in the second season, FCC2 and FCP2, without statistically differences among them, presented the highest values (5.23 and 5.66 mg Eq 100 g–1 FW). Also, 1.15 mg 100 g–1 of cyanidin 3-O-glycoside and 0.04 mg 100 g–1 of pelargonidin 3-O-glycoside had been reported for F. chiloensis.
Table 2
Main anthocyanins, ellagic acid and derivatives, quercetin and derivatives content (mg Eq 100 g–1 FW) by HPLC-DAD of four white strawberry (F. chiloensis spp. chiloensis f. chiloensis of two seasons and two locations)*
Compound | FCC1 | FCC2 | FCP1 | FCP2 |
Cyanidin 3-O-glucoside | 1.23±0.16c | 5.23±0.24a | 3.24±0.56b | 5.66±0.28a |
Pelargonidin 3-O-glucoside | 0.10±0.02b | 0.43±0.08b | 0.19±0.05b | 0.18±0.01b |
Cyanidin malonyl-glucoside | 0.26±0.06c | 0.98±0.07b | 0.55±0.18b | 1.85±0.07a |
Pelargonidin malonyl-glucoside | nq | 0.06±0.01b | nq | 0.17±0.03a |
Total Anthocyanins | 1.59 | 6.70 | 3.98 | 7.86 |
Ellagic acid pentoside | 0.14±0.09c | 5.93±1.52a | 0.25±0.10c | 3.21±0.58b |
Ellagic acid rhamnoside | 4.36±0.12ab | 3.44±0.48b | 4.35±0.25b | 7.00±1.99a |
Ellagic acid | 6.54±0.30a | 3.66±0.62b | 4.11±0.31b | 4.86±0.99b |
Quercetin pentoside | 1.51±0.61a | 1.88±0.75a | 1.93±0.50a | 1.83±0.52a |
Quercetin glucuronide | 2.10±0.07c | 4.90±0.28a | 0.60±0.11d | 3.18±0.66b |
Sum of Compounds | 16.24 | 26.49 | 15.21 | 27.91 |
nq; not quantifiable; *FCC1 is F. chiloensis ‘Contulmo’ 2017, FCC2 is F. chiloensis ‘Contulmo’ 2018, FCP1 is F. chiloensis ‘Purén’ 2017, FCP2 is F. chiloensis ‘Purén’ 2018. Different superscript letters in the same row mean significant differences at (P≤0.05).
The values of total anthocyanins in white strawberries (Table 2) ranged from 1.59 mg EC 100 g–1 FW (FCC1) to 7.86 mg EC 100 g–1 FW (FCP2). The highest values in white strawberry of total anthocyanins were for FCP2 and FCC2 (7.86 and 6.70 mg EC 100 g–1 FW respectively), being cyanidin 3-O-glucoside, cyanidin malonyl-glucoside and pelargonidin 3-O-glucoside the anthocyanins found in greater concentrations. These values obtained during the second year of harvest are considered high for the white strawberry, which is in accordance with the effect of the edaphoclimatic conditions that were experienced in this second year (low accumulated precipitations and high temperature, see Supplementary Data 2). Investigations have reported for F. chiloensis ssp. chiloensis f. chiloensis values around 2.3 and 2.2 mg EC 100 g–1 FW, respectively [15, 16].
The ellagic acid content was approximately higher for F. chiloensis (ranged from 3.66 to 6.54 mg Eq 100 g–1 FW) than described for F. x ananassa. Statistically differences were found between FCC1 and the rest of the samples, being the first season and in the Contulmo location where the ellagic acid content was superior (6.54 mg Eq 100 g–1 FW). Simirgiotis et al. [15] reported that the content of free ellagic acid was about 11 times higher for F. chiloensis than F. x ananassa directly influenced by edaphoclimatic conditions.
3.2.2Total Phenolics Content (TPC) and the Antioxidant Capacity of Fragaria chiloensis
Phenolic content in the hydromethanolic extract of F. chiloensis ssp. chiloensis f. chiloensis are shown in Table 3. The phenolic content for FCC2 and FCP2 presented significant differences harvested in the same season. These results obtained for white berries were similar to those previously reported [15–17].
Table 3
Total phenolics (TP) content and antioxidant capacity of four white strawberry (F. chiloensis spp. chiloensis f. chiloensis of two seasons and two locations) *
White Strawberry | TP (mg GAE 100 g–1 FW) | DPPH• (μmol TE 100 g–1 FW) | ORAC (μmol TE 100 g–1 FW) |
FCC1 | 145.8±20.9c | 1054±125c | 2707±196ab |
FCC2 | 218.3±14.3b | 2512±201b | 2934±351ab |
FCP1 | 146.6±15.0c | 1052±166c | 2675±194b |
FCP2 | 262.1±21.3a | 3314±334a | 3126±365ab |
*FCC1 is F. chiloensis ‘Contulmo’ 2017, FCC2 is F. chiloensis ‘Contulmo’ 2018, FCP1 is F. chiloensis ‘Purén’ 2017, FCP2 is F. chiloensis ‘Purén’ 2018. FW is fresh weight, TE is Trolox equivalent, DPPH• is 2,2-diphenyl-1-picrylhydrazyl radical reduction test and ORAC is Oxygen radical absorbance capacity. Different superscript letters in the same column mean significant differences at (P≤0.05).
Related to antioxidant capacity FCP2 had the highest antioxidant capacity by DPPH• (Table 3), showing 3314μmol TE 100 g–1 FW, while the lowest antioxidant capacity was observed in FCC1 and FCP1 with 1052–1054μmol TE 100 g–1 FW respectively. It is observed in Tables 2 and 3 that the higher the content of cyanidin 3-O-glucoside, the greater the antioxidant capacity for white strawberry, which indicates the differences that exist between the different compounds. Although not only that anthocyanin is remarkable, but also ellagic acids and derivatives and the flavonols presented by the FCC2 and FCP2 samples. As mentioned above, the ellagic acid content and ellagitannin derivatives were high values for all the samples for F. chiloensis finding the sum of all these compounds higher for FCP2. In a study carried out by Cervantes et al. [33], the antioxidant capacity was 1811μmol TE 100 g–1 FW for F. x ananassa. Other investigations reported values of around 2986μmol TE 100 g–1 FW for F. x ananassa being values inferior than FCP2.
It is observed that there is a direct relationship between total polyphenols and antioxidant capacity, observing how it was influenced by the lack of rain in second season FCC2, FCP2, (Supplementary Data 2), and therefore presenting a higher antioxidant capacity.
3.3Inhibition of α-Glucosidase activity
Inhibition of α-glucosidase activity revealed that all diluted extracts of strawberry significantly inhibited the enzyme as shown in Table 4. For white strawberries, the inhibition of α-glucosidase ranged from 1.04 (FCP2) to 1.35 mg mL–1 (FCC1). The α-glucosidase inhibition values of diluted extracts of FCP2 and FCC1 presented statistically significant difference showing FCC1 the most potent inhibitory effect. The IC50 values for drugs commonly used for diabetes such as amaryl (glimerid) was 0,88 mg mL–1, for betanorm (glicazide) was 1,40 mg mL–1 and glucobay (acarbose) was 0,75 mg mL–1 [35]. However, in studies by Yang et al. [21] and Thomas-Valdes et al. [18] indicated that the enzyme inhibition was mainly attributed to polyphenols from F. x ananassa and F. chiloensis spp. chiloensis f. chiloensis, respectively. Other works, showed that α-glucosidase inhibitors, such as purified phenolic compounds such as ellagic acid and derivatives [36], ellagitannins [37], anthocyanins [38, 39] and gallotannins [40] manifested an inhibitory effect on the enzyme.
Table 4
Inhibition of α-glucosidase and acetylcholinesterase activity (mg mL–1) of four white strawberry (F. chiloensis spp. chiloensis f. chiloensis of two seasons and two locations)*
White Strawberry | α-glucosidase | Acetylcholinesterase |
FCC1 | 1.35±0.14b | 5.57±0.88b |
FCC2 | 1.24±0.05ab | 3.01±0.86a |
FCP1 | 1.11±0.15ab | 3.93±0.88ab |
FCP2 | 1.04±0.12a | 2.88±0.53a |
*FCC1 is F. chiloensis ‘Contulmo’ 2017, FCC2 is F. chiloensis ‘Contulmo’ 2018, FCP1 is F. chiloensis ‘Purén’ 2017, FCP2 is F. chiloensis ‘Purén’ 2018. Different superscript letters in the same column mean significant differences at (P≤0.05).
In our case, it would be the high concentrations of ellagic acid obtained in the first season in the FCC1 sample, which has the greatest inhibitory power on this enzyme, not observing this result for other phenolic compounds. Hence, Fragaria chiloensis is a rich source of phenolic compounds and aromatic volatiles and offer a prospective alternative for the management of postprandial hyperglycemia. Kam et al. [41] suggest that a phenolic compound structure, particularly the presence of the trihydroxybenzoic acid backbone, may influence the selective inhibition of α-glucosidase. Tadera et al. [42] showed that other flavonoids such as quercetin, kaempferol, and catechin had inhibitory effect on the activity of this enzyme. The α-glucosidase inhibitory activity is considered an effective strategy for diabetes control because of the decrease in glucose absorption in the body [43] and phenol extracts from strawberry can potentially reduce hyperglycemia in diabetes by inhibiting α-glucosidase. Seasonal and local changes and therefore difference in bioactive compound contents, presented changes on inhibition of the α-glucosidase activity and therefore should be taken into account.
3.4Inhibition of acetylcholinesterase activity
Inhibition of acetylcholinesterase activity revealed that all diluted extracts of strawberry significantly inhibited the enzyme as shown in Table 4. For white strawberries, the inhibition of glucosidase ranged from 2.88 (FCP2) to 5.57 mg mL–1 (FCC1). Nevertheless, acetylcholinesterase inhibition values of all diluted extracts of strawberries had significant difference between FCC1 and the rest of samples. As in the previous case, FCC1 presented a higher concentration of ellagic acid, and therefore it could be that the biological activity was directly related to this compound in the white strawberry. In this case, seasonal and local changes and therefore variations in bioactive compounds, involved changes on inhibition of the acetylcholinesterase activity. The IC50 values for drugs to inhibit AChE were: physostigmine 1.8×10–4μg mL–1, rivastigmine 1.1×10–3μg mL–1, donepezil 2.5×10–3μg mL–1 and tacrine 1.5×10–3μg mL–1 [44]. Although, the values obtained from inhibition were low, it is important to note that all were tested in diluted extracts.
The common approach to treating neurodegenerative diseases is to inhibit the activity of acetylcholinesterase in order to increase the level of cholinergic neurotransmitters in the brain. In fact, inhibitors of this enzyme are used clinically to increase the time of action of acetylcholine in the synaptic space [45]. Inhibition of acetylcholinesterase was used to determine the neuroprotector effect of extracts of strawberries as an approximation. Acetylcholinesterase inhibition activity has been previously reported from various plant extracts [46]. This effect has long been attributed to polyphenols. Several studies reported that compounds such as quercetin, kaempferol derivatives [47, 48] and ellagic acid [49] manifested an inhibitory effect on acetylcholinesterase. Therefore, phenols present in Chilean white strawberry extract can potentially have other biological activities that they have not been considered inhibitors against of acetylcholinesterase and could be used as nutraceuticals or functional foods.
3.5The inhibition mode and inhibition kinetics of α-glucosidase and acetylcholinesterase
The inhibition kinetics of the two enzymes was calculated to elucidate inhibition mode. As shown in Fig. 1a, b, c, d, all inhibition kinetics curves crossed X axis, therefore, the inhibition type of all strawberries for α-glucosidase was non-competitive. From the Michaelis-Menten equation in Table 5, the value of the transverse axis intercept (Km) remained unchanged with all strawberries, indicating that all diluted extracts were non-competitive inhibitors for α-glucosidase. Therefore, there are combined actions between inhibitor and enzyme as well as between inhibitor and enzyme-substrate complex.
Fig. 1
Lineweaver-Burk plot of different diluted extracts from strawberries on α-glucosidase. Four white strawberry, F. chiloensis spp. chiloensis f. chiloensis (a FCC1; b FCC2; c FCP1; d FCP2).
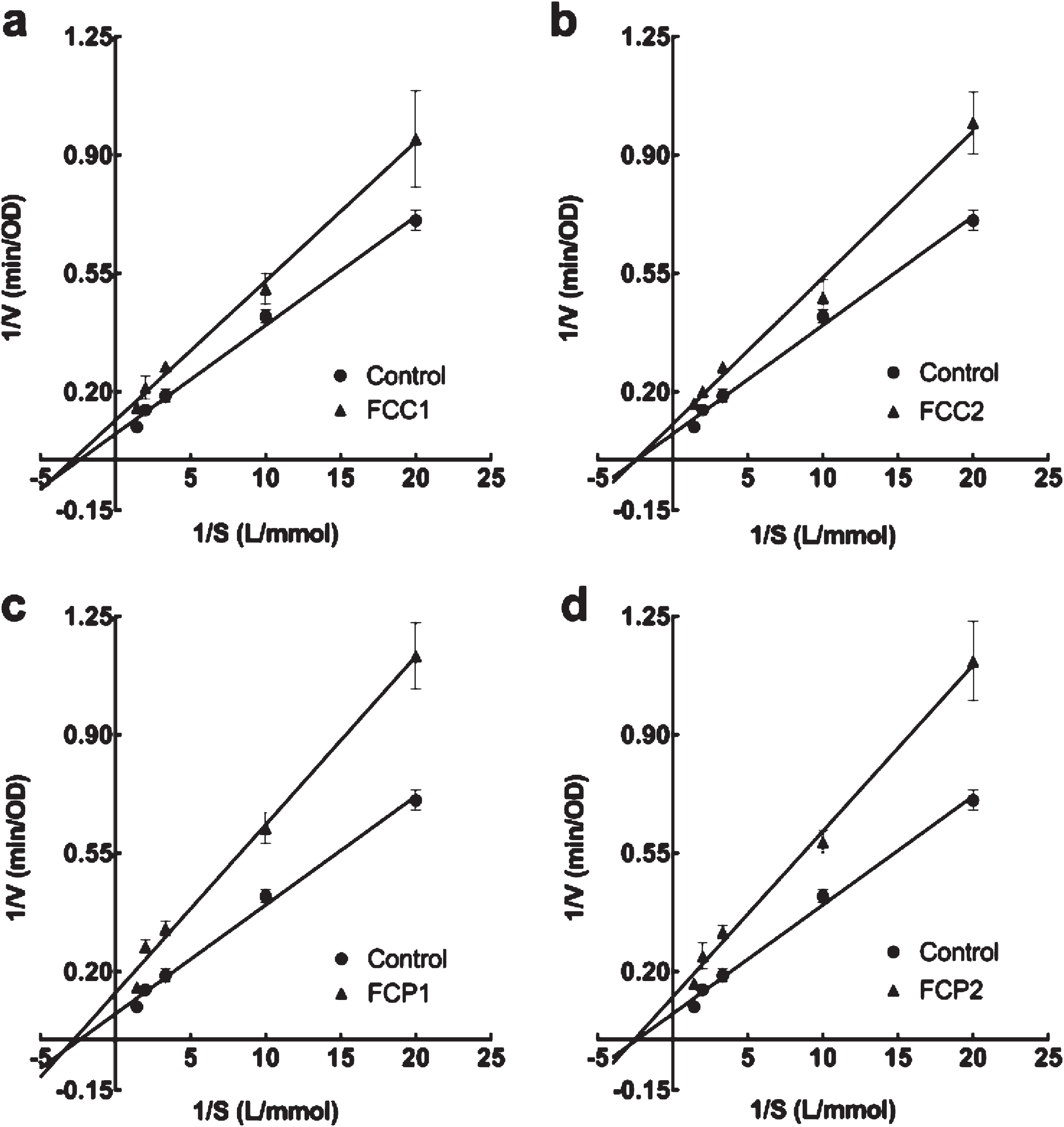
Table 5
Related parameters on inhibition kinetics of four white strawberry (F. chiloensis spp. chiloensis f. chiloensis of two seasons and two locations) on α-glucosidase
Double reciprocal equation | R2 | Km (L mmol–1) | Vmax (OD min–1) | Inhibition type | |
Control | y = 0.032x + 0.075 | 0.99 | 0.42±0.02 | 13.25±1.15* | – |
FCC1 | y = 0.041x + 0.116 | 0.99 | 0.38±0.03 | 8.64±1.37* | Non-competitive |
FCC2 | y = 0.043x + 0.104 | 0.99 | 0.42±0.02 | 9.62±1.93* | Non-competitive |
FCP1 | y = 0.049x + 0.137 | 0.99 | 0.39±0.02 | 7.32±1.78* | Non-competitive |
FCP2 | y = 0.049x + 0.124 | 0.99 | 0.40±0.02 | 8.06±1.20* | Non-competitive |
FCC1 is F. chiloensis ‘Contulmo’ 2017, FCC2 is F. chiloensis ‘Contulmo’ 2018, FCP1 is F. chiloensis ‘Purén’ 2017, FCP2 is F. chiloensis ‘Purén’ 2018. “x” represents the reciprocal of substrate concentration, “y” represents the reciprocal of rate of catalysis reaction rate. *Indicates significant differences with respect to the control (P≤0.05).
In Fig. 2a, b, c, d, all inhibition kinetics curves crossed Y axis, so the inhibition type of all strawberries for acetylcholinesterase was competitive. From the Michaelis-Menten equation in Table 6, the value of the vertical axis intercept (1/Vmax) remained unchanged with all strawberries, indicating that all diluted extracts were competitive inhibitors for acetylcholinesterase. Therefore, there are actions between inhibitor and enzyme.
Fig. 2
Lineweaver-Burk plot of different diluted extracts from strawberries on acetylcholinesterase. Four white strawberry, F. chiloensis spp. chiloensis f. chiloensis (a FCC1; b FCC2; c FCP1; d FCP2).
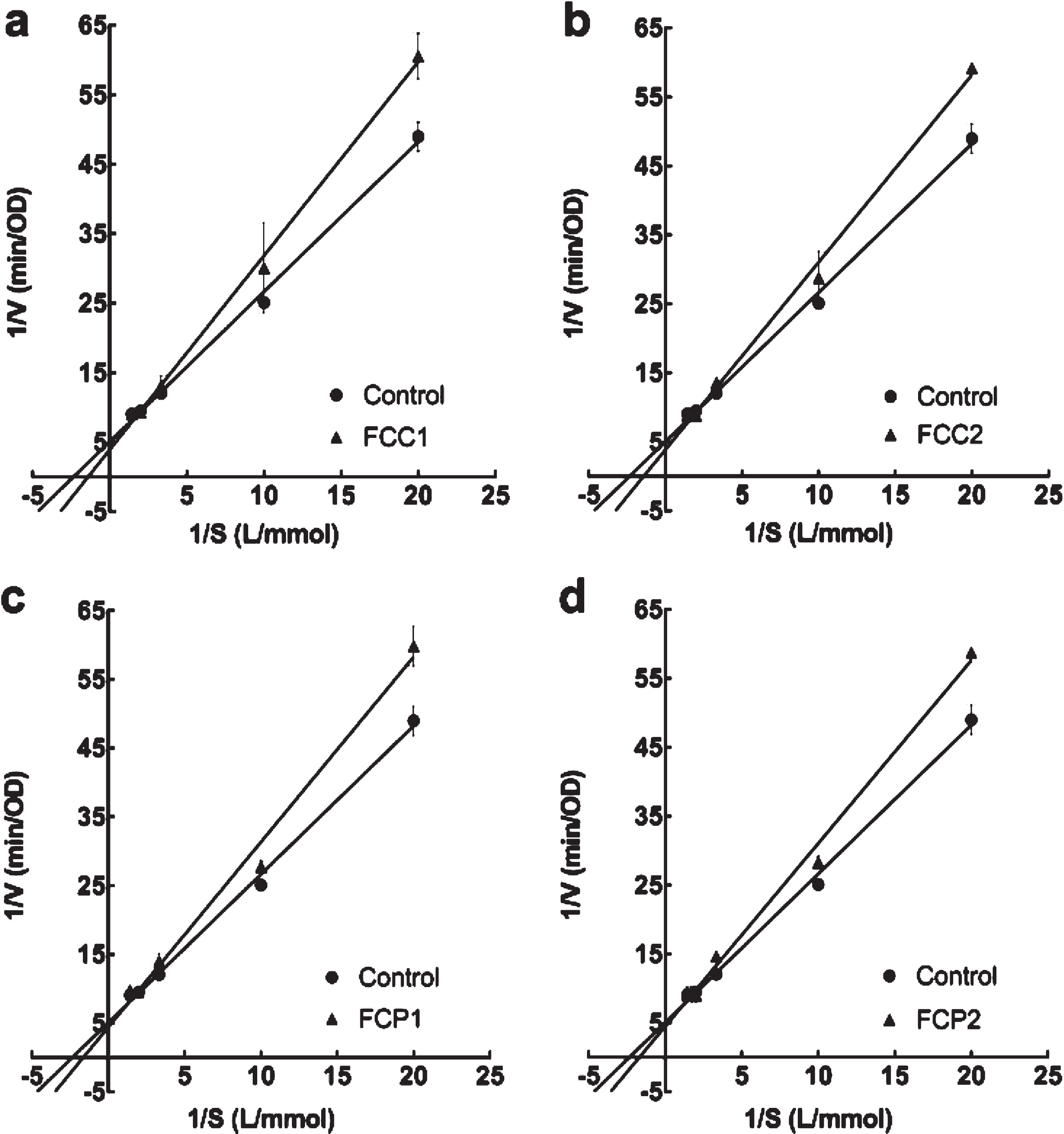
Table 6
Related parameters on inhibition kinetics of extracts of four white strawberry (F. chiloensis spp. chiloensis f. chiloensis of two seasons and two locations) on acetylcholinesterase
Double reciprocal equation | R2 | Km (L mmol–1) | Vmax (OD min–1) | Inhibition type | |
Control | y = 2.16x + 5.05 | 0.99 | 0.43±0.01 | 0.20±0.02 | – |
FCC1 | y = 2.79x + 3.88 | 0.99 | 0.72±0.03* | 0.26±0.05 | Competitive |
FCC2 | y = 2.72x + 3.85 | 0.99 | 0.71±0.03* | 0.26±0.09 | Competitive |
FCP1 | y = 2.69x + 4.37 | 0.99 | 0.62±0.09* | 0.23±0.06 | Competitive |
FCP2 | y = 2.66x + 4.47 | 0.99 | 0.60±0.03* | 0.22±0.09 | Competitive |
FCC1 is F. chiloensis ‘Contulmo’ 2017, FCC2 is F. chiloensis ‘Contulmo’ 2018, FCP1 is F. chiloensis ‘Purén’ 2017, FCP2 is F. chiloensis ‘Purén’ 2018. “x” represents the reciprocal of substrate concentration, “y” represents the reciprocal of rate of catalysis reaction rate. * Indicates significant differences with respect to the control (P≤0.05).
In both cases, we can check that despite having different biological activity due to seasonal and local changes and therefore variations in bioactive compounds, the mode of action did not vary. That is to say, that these results illustrate that the behavior of white strawberry extracts (mode of action) on these two enzymes would not be so affected by seasonal or local changes. However, the percentage of enzyme inhibition would be related to differences in concentrations of the analyzed compounds and these would be influenced by seasonal changes and location.
3.6Principal component analysis
Principal component analysis (PCA) was applied to assess the relationships among Fragaria chiloensis samples grown in two season and two different geographical places from Chile regarding their phytochemical composition and biological activity. Three PCs explained up to 89.4% of the total variance. The first PC (PC1) accounted for 53.1% of the total variability and had positive loadings from pelargonidin malonyl-glucoside, cyanidin malonyl-glucoside, total phenolics, DPPH•, ORAC, ellagic acid rhamnoside and cyanidin 3-O-glucoside. The second PC (PC2), representing 19.7% of the total variance, was positively associated pelargonidin 3-O-glucoside, ellagic acid pentoside and quercetin glucuronide. The third PC (PC3), representing 16.7% of the total variance, was positively associated to α-glucosidase, acetylcholinesterase, ellagic acid and quercetin pentoside (Fig. 3). The two geographical regions and two seasons considered exhibited some common patterns. Hence, it can be seen that a large part of flavonols and phenolic acids were correlated with in vitro assays (TP, DPPH• and ORAC). However, this did not occur with ellagic acid, since this compound was correlated, although in a low percentage, with the biological activity potential, α-glucosidase inhibition and acetylcholinesterase inhibition. This is especially relevant since ellagic acid was one of the compounds that was affected by seasonal changes and the sample FCC1 presented a higher concentration of this compound. In addition, the biological activity was directly related to concentration of ellagic acid. Therefore, this analysis confirms that seasonal and local changes and therefore variations in bioactive compounds, involved changes on inhibition of the acetylcholinesterase and α-glucosidase activity.
Fig. 3
Principal component analysis (with varimax rotation) of the phytochemical composition of F. chiloensis spp. chiloensis f. chiloensis of two season and two different localities from Chile. Loading plot of PC1 versus PC2 versus PC3; In the loading plot: TP, total phenolics; C3g, cyanidin 3-O-glucoside; P3g, pelargonidin 3-O-glucoside; Cmg, cyanidin malonyl-glucoside; Pmg, pelargonidin malonyl-glucoside; EAp, ellagic acid pentoside; EAr, ellagic acid rhamnoside; EA, ellagic acid; Qp, quercetin pentoside; Qgluc, quercetin glucuronide.
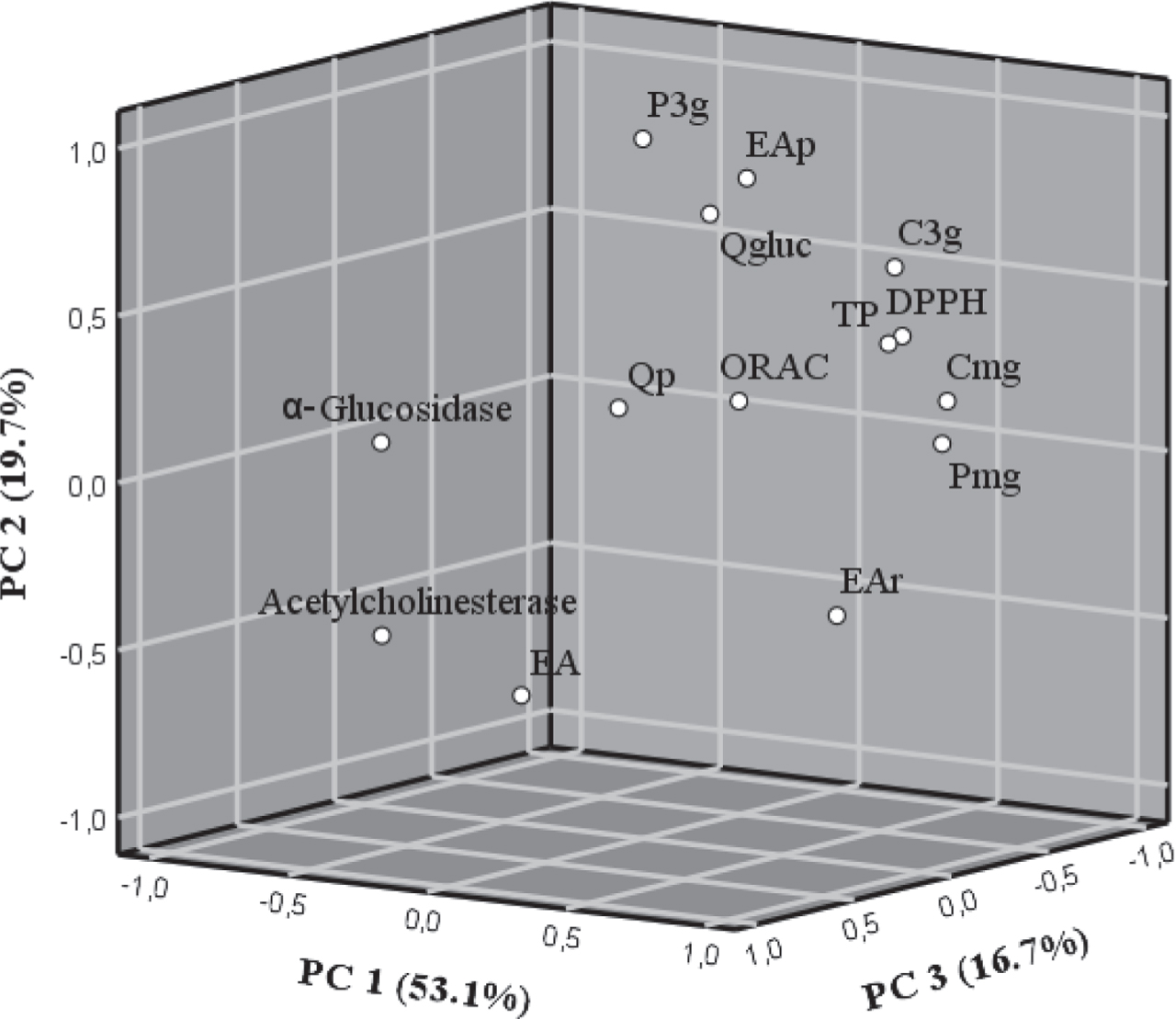
4Conclusions
F. chiloensis presented seasonal changes in the aroma due at least in part to the edaphoclimatic conditions where the plants are growing (e.g. reduced content of volatiles with excessive rain is present, season 2018). In addition, in F. chiloensis twenty-four different phenolic compounds were identified, with varying concentrations depending on geographical location and season. The major compounds quantified in the hydromethanolic extracts of F. chiloensis were ellagic acid and its derivatives, as well as cyanidin 3-O-glucoside. The same extracts were also tested for antioxidant capacity that were also lower in rainy season. Looking into potential for health-promoting effects, the studied extracts were effective inhibitors of α-glucosidase (non-competitive) and acetylcholinesterase (competitive). However, the inhibition on the enzymes was influenced by changes in chemical composition and therefore, seasonal and location changes. Fragaria chiloensis as rich source of phenolic compounds and aromatic volatiles offer a prospective alternative for the management of postprandial hyperglycemia. To the best of our knowledge, this is one of the first reports of anticholinesterase inhibitory properties of Fragaria spp. Further studies would help in improving the efficacy of the inhibition (better IC50) with other formulations enriched in the bioactive molecules more related to this inhibitory activity.
For the future of food supply and security, Chilean white strawberry is a promising resource for managing metabolic (e.g. diabetes mellitus) and cognitive (e.g. neurodegenerative diseases) impairments of the aging population worldwide. Further investigations are needed to improve the bioactivity-compound relationships in the context of dietary advice and future intervention studies for functional food developments. On the other hand, valuable data for establishing good production practices for rural development are also obtained in order to provide fruits with a standardized quality of composition according to the area of production.
Conflict of interest
The authors have no conflict of interest to report.
Supplementary materials
[1] The supplementary material is available in the electronic version of this article: https://dx.doi.org/10.3233/JBR-200585.
Acknowledgments
The authors gratefully acknowledge financial support of FONDECYT 1160899.
References
[1] | Esquinas-Alcázar J . Protecting crop genetic diversity for food security: political, ethical and technical challenges. Nat Rev Genet. (2005) ;6: :946–53. https://doi.org/10.1038/nrg1729 |
[2] | Frison E . After Vavilov: Collecting germplasm in the 21st century. Collecting plant genetic diversity: Technical guidelines. 2011 update, Rome, Italy.: Bioversity International; 2011, pp. 2. |
[3] | Alothman M , Bhat R , Karim AA . Effects of radiation processing on phytochemicals and antioxidants in plant produce. Trends in Food Sci & Tech. (2009) ;20: :201–12. https://doi.org/10.1016/j.tifs.2009.02.003 |
[4] | Cassileth B . Complementary Therapies, Herbs, and Other OTC Agents. Oncol. (2010) ;24: :1202. |
[5] | Rubilar M , Jara C , Poo Y , Acevedo F , Gutierrez C , Sineiro J , et al. Extracts of Maqui (Aristotelia chilensis) and Murta (Ugni molinae Turcz.): Sources of Antioxidant Compounds and α-Glucosidase/α-Amylase Inhibitors. J Agric Food Chem. (2011) ;59: :1630–7. https://doi.org/10.1021/jf103461k |
[6] | Gironés-Vilaplana A , Baenas N , Villaño D , Moreno DA . Iberian-American fruits rich in bioactive phytochemicals for nutrition and health. 2014. |
[7] | Rojo LE , Ribnicky D , Logendra S , Poulev A , Rojas-Silva P , Kuhn P , et al. In vitro and in vivo anti-diabetic effects of anthocyanins from Maqui Berry (Aristotelia chilensis). Food Chem. (2012) ;131: :387–96. https://doi.org/10.1016/j.foodchem.2011.08.066 |
[8] | López MD , Campoy FJ , Pascual-Villalobos MJ , Muñoz-Delgado E , Vidal CJ . Acetylcholinesterase activity of electric eel is increased or decreased by selected monoterpenoids and phenylpropanoids in a concentration-dependent manner. Chemico-Biological Interactions. (2015) ;229: :36–43. https://doi.org/10.1016/j.cbi.2015.01.006 |
[9] | Gironés-Vilaplana A , Valentão P , Moreno DA , Ferreres F , Garcıa-Viguera C , Andrade PB . New Beverages of Lemon Juice Enriched with the Exotic Berries Maqui, Açaı, and Blackthorn: Bioactive Components and in Vitro Biological Properties. J Agric Food Chem. (2012) ;60: :6571–80. https://doi.org/10.1021/jf300873k |
[10] | Kawabata K , Mukai R , Ishisaka A . Quercetin and related polyphenols: new insights and implications for their bioactivity and bioavailability. Food Funct. (2015) ;6: :1399–417. https://doi.org/10.1039/C4FO01178C |
[11] | Speisky H , López-Alarcón C , Gómez M , Fuentes J , Sandoval-Acuña C . First Web-Based Database on Total Phenolics and Oxygen Radical Absorbance Capacity (ORAC) of Fruits Produced and Consumed within the South Andes Region of South America. J Agric Food Chem. (2012) ;60: :8851–9. https://doi.org/10.1021/jf205167k |
[12] | Tomé-Carneiro J , Visioli F . Polyphenol-based nutraceuticals for the prevention and treatment of cardiovascular disease: Review of human evidence. Phytomedicine. (2016) ;23: :1145–74. https://doi.org/10.1016/j.phymed.2015.10.018 |
[13] | Retamales J , Caligari P , Carrasco B , Saud G . Current Status of the Chilean Native Strawberry and the Research Needs to Convert the Species into a Commercial Crop. HortScience. (2005) ;40: :1633–4. https://doi.org/10.21273/HORTSCI.40.6.1633 |
[14] | González M , Gaete-Eastman C , Valdenegro M , Figueroa CR , Fuentes L , Herrera R , et al. Aroma development during ripening of Fragaria chiloensis fruit and participation of an alcohol acyltransferase (FcAAT1) gene. J Agric Food Chem. (2009) ;57: :9123–32. https://doi.org/10.1021/jf901693j |
[15] | Simirgiotis MJ , Theoduloz C , Caligari PDS , Schmeda-Hirschmann G . Comparison of phenolic composition and antioxidant properties of two native Chilean and one domestic strawberry genotypes. Food Chem. (2009) ;113: :377–85. https://doi.org/10.1016/j.foodchem.2008.07.043 |
[16] | Cheel J , Theoduloz C , Rodriguez JA , Caligari PDS , Schmeda-Hirschmann G . Free radical scavenging activity and phenolic content in achenes and thalamus from Fragaria chiloensis ssp. chiloensis, F. vesca and F. x ananassa cv. Chandler. Food Chemistry. 2007. https://doi.org/10.1016/j.foodchem.2006.04.036 |
[17] | Simirgiotis MJ , Schmeda-Hirschmann G . Determination of phenolic composition and antioxidant activity in fruits, rhizomes and leaves of the white strawberry (Fragaria chiloensis spp. chiloensis form chiloensis) using HPLC-DAD-ESI-MS and free radical quenching techniques. J Food Compos Anal. (2010) ;23: :545–53. https://doi.org/10.1016/j.jfca.2009.08.020 |
[18] | Thomas-Valdés S , Theoduloz C , Jiménez-Aspee F , Burgos-Edwards A , Schmeda-Hirschmann G . Changes in polyphenol composition and bioactivity of the native Chilean white strawberry (Fragaria chiloensis spp. chiloensis f. chiloensis) after in vitro gastrointestinal digestion. Food Res Int. (2018) ;105: :10–8. https://doi.org/10.1016/j.foodres.2017.10.074 |
[19] | Benvenuti S , Pellati F , Melegari M , Bertelli D . Polyphenols, Anthocyanins, Ascorbic Acid, and Radical Scavenging Activity of Rubus, Ribes, and Aronia. J Food Sci. (2004) ;69: :164–9. https://doi.org/10.1111/j.1365-2621.2004.tb13352.x |
[20] | Papandreou MA , Dimakopoulou A , Linardaki ZI , Cordopatis P , Klimis-Zacas D , Margarity M , et al. Effect of a polyphenol-rich wild blueberry extract on cognitive performance of mice, brain antioxidant markers and acetylcholinesterase activity. Behav Brain Res. (2009) ;198: :352–8. https://doi.org/10.1016/j.bbr.2008.11.013 |
[21] | Yang D , Xie H , Jiang Y , Wei X . Phenolics from strawberry cv. Falandi and their antioxidant and α-glucosidase inhibitory activities. Food Chem. (2016) ;194: :857–63. https://doi.org/10.1016/j.foodchem.2015.08.091 |
[22] | Miliauskas G , Venskutonis PR , van Beek TA . Screening of radical scavenging activity of some medicinal and aromatic plant extracts. Food Chemistry. (2004) ;85: :231–7. https://doi.org/10.1016/j.foodchem.2003.05.007 |
[23] | Fredes C , Montenegro G , Zoffoli JP , Santander F , Robert P . Comparison of the total phenolic content, total anthocyanin content and antioxidant activity of polyphenol-rich fruits grown in Chile. Ciencia e Investigación Agraria. (2014) ;41: :49–60. https://doi.org/10.4067/S0718-16202014000100005 |
[24] | Keutgen AJ , Pawelzik E . Modifications of Strawberry Fruit Antioxidant Pools and Fruit Quality under NaCl Stress. J Agric Food Chem. (2007) ;55: :4066–72. https://doi.org/10.1021/jf070010k |
[25] | Ruiz A , Sanhueza M , Gómez F , Tereucán G , Valenzuela T , García S , et al. Changes in the content of anthocyanins, flavonols, and antioxidant activity in Fragaria ananassa var. Camarosa fruits under traditional and organic fertilization. Journal of the Science of Food and Agriculture. (2019) ;99: :2404–10. https://doi.org/10.1002/jsfa.9447 |
[26] | Ferreres F , Gomes D , Valentão P , Gonçalves R , Pio R , Chagas EA , et al. Improved loquat (Eriobotrya japonica Lindl. ) cultivars: Variation of phenolics and antioxidative potential. Food Chemistry. (2009) ;114: :1019–27. https://doi.org/10.1016/j.foodchem.2008.10.065 |
[27] | Singleton VL , Rossi JA . Colorimetry of Total Phenolics with Phosphomolybdic-Phosphotungstic Acid Reagents. Am J Enol Vitic. (1965) ;16: :144–58. |
[28] | Mena P , García-Viguera C , Navarro-Rico J , Moreno DA , Bartual J , Saura D , et al. Phytochemical characterization for industrial use of pomegranate (Punica granatum L. ) cultivars grown in Spain. J Sci Food Agric. (2011) ;91: :1893–906. https://doi.org/10.1002/jsfa.4411 |
[29] | Huang D , Ou B , Hampsch-Woodill M , Flanagan JA , Prior RL . High-throughput assay of oxygen radical absorbance capacity (ORAC) using a multichannel liquid handling system coupled with a microplate fluorescence reader in 96-well format. J Agric Food Chem. (2002) ;50: :4437–44. https://doi.org/10.1021/jf0201529 |
[30] | Costamagna MS , Zampini IC , Alberto MR , Cuello S , Torres S , Pérez J , et al. Polyphenols rich fraction from Geoffroea decorticans fruits flour affects key enzymes involved in metabolic syndrome, oxidative stress and inflammatory process. Food Chem. (2016) ;190: :392–402. https://doi.org/10.1016/j.foodchem.2015.05.068 |
[31] | Ellman GL , Courtney KD , Andres V , Featherstone RM . A new and rapid colorimetric determination of acetylcholinesterase activity. Biochemical Pharmacology. (1961) ;7: :88–95. https://doi.org/10.1016/0006-2952(61)90145-9 |
[32] | Prat L , Espinoza MI , Agosin E , Silva H . Identification of volatile compounds associated with the aroma of white strawberries (Fragaria chiloensis). J Sci Food Agric. (2014) ;94: :752–9. https://doi.org/10.1002/jsfa.6412. |
[33] | Cervantes L , Ariza MT , Gómez-Mora JA , Miranda L , Medina JJ , Soria C , et al. Light exposure affects fruit quality in different strawberry cultivars under field conditions. Sci Hortic. (2019) ;252: :291–7. https://doi.org/10.1016/j.scienta.2019.03.058 |
[34] | Mandave PC , Pawar PK , Ranjekar PK , Mantri N , Kuvalekar AA . Comprehensive evaluation of in vitro antioxidant activity, total phenols and chemical profiles of two commercially important strawberry varieties. Scientia Horticulturae. (2014) ;172: :124–34. https://doi.org/10.1016/j.scienta.2014.03.002 |
[35] | Onal S , Timur S , Okutucu B , Zihnioğlu F . Inhibition of alpha-glucosidase by aqueous extracts of some potent antidiabetic medicinal herbs. Prep Biochem Biotechnol. (2005) ;35: :29–36. https://doi.org/10.1081/PB-200041438 |
[36] | Yin P , Yang L , Xue Q , Yu M , Yao F , Sun L , et al. Identification and inhibitory activities of ellagic acid- and kaempferol-derivatives from Mongolian oak cups against α-glucosidase, α-amylase and protein glycation linked to type II diabetes and its complications and their influence on HepG2 cells’ viability. Arab J Chem. (2018) ;11: :1247–59. https://doi.org/10.1016/j.arabjc.2017.10.002 |
[37] | Pinto M da S , de Carvalho JE , Lajolo FM , Genovese MI , Shetty K . Evaluation of Antiproliferative, Anti-Type 2 Diabetes, and Antihypertension Potentials of Ellagitannins from Strawberries (Fragaria×ananassa Duch.) Using In Vitro Models. J of Med Food. (2010) ;13: :1027–35. https://doi.org/10.1089/jmf.2009.0257 |
[38] | You Q , Chen F , Wang X , Luo PG , Jiang Y . Inhibitory effects of muscadine anthocyanins on α-glucosidase and pancreatic lipase activities. J Agric Food Chem. (2011) ;59: :9506–11. https://doi.org/10.1021/jf201452v |
[39] | Zhang J , Sun L , Dong Y , Fang Z , Nisar T , Zhao T , et al. Chemical compositions and α-glucosidase inhibitory effects of anthocyanidins from blueberry, blackcurrant and blue honeysuckle fruits. Food Chemistry. (2019) ;299: :125102. https://doi.org/10.1016/j.foodchem.2019.125102 |
[40] | Yue LM , Lee J , Zheng L , Park YD , Ye ZM , Yang JM . Computational prediction integrating the inhibition kinetics of gallotannin on α-glucosidase. Int J Biol Macromol. (2017) ;103: :829–38. https://doi.org/10.1016/j.ijbiomac.2017.05.106 |
[41] | Kam A , Li KM , Razmovski-Naumovski V , Nammi S , Shi J , Chan K , et al. A comparative study on the inhibitory effects of different parts and chemical constituents of pomegranate on α-amylase and α-glucosidase. Phytother Res. (2013) ;27: :1614–20. https://doi.org/10.1002/ptr.4913 |
[42] | Tadera K , Minami Y , Takamatsu K , Matsuoka T . Inhibition of alpha-glucosidase and alpha-amylase by flavonoids. J Nutr Sci Vitaminol. (2006) ;52: :149–53. https://doi.org/10.3177/jnsv.52.149 |
[43] | Ghani U . Re-exploring promising α-glucosidase inhibitors for potential development into oral anti-diabetic drugs: Finding needle in the haystack. Eur J Med Chem. (2015) ;103: :133–62. https://doi.org/10.1016/j.ejmech.2015.08.043 |
[44] | Ogura H , Kosasa T , Kuriya Y , Yamanishi Y . Comparison of inhibitory activities of donepezil and other cholinesterase inhibitors on acetylcholinesterase and butyrylcholinesterase in vitro. Methods Find Exp Clin Pharmacol. (2000) ;22: :609–13. https://doi.org/10.1358/mf.2000.22.8.701373 |
[45] | Chopra K , Misra S , Kuhad A . Current perspectives on pharmacotherapy of Alzheimer’s disease. Expert Opin Pharmacother. (2011) ;12: :335–50. https://doi.org/10.1517/14656566.2011.520702 |
[46] | Amessis-Ouchemoukh N , Madani K , Falé PLV , Serralheiro ML , Araújo MEM . Antioxidant capacity and phenolic contents of some Mediterranean medicinal plants and their potential role in the inhibition of cyclooxygenase-1 and acetylcholinesterase activities. Industrial Crops and Products. (2014) ;53: :6–15. https://doi.org/10.1016/j.indcrop.2013.12.008 |
[47] | Nguyen DH , Seo UM , Zhao BT , Le DD , Seong SH , Choi JS , et al. Ellagitannin and flavonoid constituents from Agrimonia pilosa Ledeb. with their protein tyrosine phosphatase and acetylcholinesterase inhibitory activities. Bioorg Chem. (2017) ;72: :293–300. https://doi.org/10.1016/j.bioorg.2017.04.017 |
[48] | Sousa GF de , Aguilar MG de , Takahashi JA , Alves TMA , Kohlhoff M , Vieira Filho SA , et al. Flavonol triglycosides of leaves from Maytenus robusta with acetylcholinesterase inhibition. Phytochem Lett. (2017) ;19: :34–8. https://doi.org/10.1016/j.phytol.2016.10.024 |
[49] | Jha AB , Panchal SS , Shah A . Ellagic acid: Insights into its neuroprotective and cognitive enhancement effects in sporadic Alzheimer’s disease. Pharmacol Biochem Behav. (2018) ;175: :33–46. https://doi.org/10.1016/j.pbb.2018.08.007 |