Red raspberry (Rubus idaeus) consumption restores the impaired vasoconstriction and vasorelaxation response in the aorta of the obese Zucker rat, a model of the Metabolic Syndrome
Abstract
Metabolic Syndrome (MetS) increases the risk of cardiovascular disease. Whole red raspberry (WRR) consumption on vascular function was investigated in the obese Zucker rat (OZR), model of MetS. Male OZR and their lean littermates (LZR) were placed on a control (C) or an 8% w/w WRR-enriched diet for 8 weeks. Phenylephrine (Phe)-induced vasoconstriction and acetylcholine (Ach)-induced vasorelaxation were measured in aortic rings in the presence or absence of L-N-monomethyl-arginine (L-NMMA) and mefenamic acid (MFA). Phe-induced vasoconstriction was lower in the OZR-C compared to LZR-C (p < 0.05). The WRR diet partially restored aortic response in the OZR-WRR aorta (p < 0.05) compared to OZR- C. The OZR-WRR group pre-treated with L-NMMA increased compared to OZR-C (p < 0.05). Pre-treatment with L-NMMA, maximal relaxation response was higher in the OZR compared to the LZR (p < 0.05). With L-NMMA, maximal relaxation response in OZR-WRR (p < 0.05) was lower compared to the OZR-C. Prostacyclin I2 concentration was higher in the OZR compared to the LZR (p < 0.05) and was attenuated in the OZR-WRR (p < 0.05). Aortic expression of eNOS and COX-2 were downregulated in the OZR-WRR (p < 0.05). In conclusion, WRR restores the impaired vascular tone of the OZR by enhancing Phe-induced vasoconstriction and attenuating Ach-induced vasorelaxation.
1Background
The Metabolic Syndrome (MetS) is a combination of interrelated conditions including abdominal obesity, dyslipidemia, insulin resistance, glucose intolerance, and hypertension resulting in an increased risk for cardiovascular disease (CVD) and type-2-diabetes mellitus (T2DM) [1]. Genetics and environmental factors such as a Western-style diet and physical inactivity are major factors that contribute to the development of MetS [2]. There is a plethora of evidence which demonstrates that dietary intervention prevents and/or reverses the development of MetS [3].
Vascular function a major component of vascular health and disease, is regulated by the major pathways of nitric oxide synthase (NOS) and cyclooxygenase (COX) [4, 5]. In healthy blood vessels these pathways produce vasoactive mediators such as nitric oxide (NO), thromboxane A2 (TXA2), and prostacyclin (PGI2) [6]. Nitric oxide, a product of NOS, is a vasodilator that suppresses vascular smooth muscle cell proliferation, blood cell adhesion, and lipid peroxidation [7, 8]. The COX pathway is responsible for prostanoid formation including TXA2 and PGI2. Prostacyclin, is a vasorelaxant agent that acts synergistically with NO to inhibit platelet aggregation [9], whereas TXA2 is an endothelium-dependent vasoconstrictor and a potent inducer of platelet aggregation [10]. Alterations in the production of these endothelially-derived factors leads to vascular dysfunction observed in individuals with MetS, initiating and promoting the pathogenesis of early vascular events such as atherosclerotic lesions [11, 12].
Numerous studies have shown the vasoprotective effects of polyphenols, acting through major pathways of vascular function [6]. Studies have reported improved vascular function with the consumption of 8% wild blueberries (WB) in the Sprague-Dawley rat after 7 [13] and 13 weeks [14], the spontaneously hypertensive rat after 8 weeks [5, 15], and the obese Zucker rat after 8 weeks [16]. Additionally, WB remodeled the composition and structure of the aortic extracellular matrix by shifting glycosaminoglycan structure to a less atherogenic profile, both in the Sprague-Dawley [17] and in the spontaneously hypertensive rat [18].
Red raspberries (Rubus idaeus) are unique compared to other berries due to their distinct phenolic profile and are good dietary sources of hydrolysable tannins [19]. Their main components are ellagitannins and anthocyanins as well as vitamin C, manganese, fiber and other nutrients [20]. Whole red raspberries and their extracts have been shown to have various potential health-promoting benefits such as anti-inflammatory [21–23], anti-angiogenic [24], anti-neurodegenerative [25], and anti-carcinogenic [26]. Recent studies demonstrate that a red raspberry-supplemented diet at 2% for 12 weeks lowered cardiometabolic risk factors in male Zucker Fatty rats on a “western-style diet” by up-regulating expression of myocardial adiponectin receptor 1 and apolipoprotein E and down-regulating lipoprotein lipase [27]. Also, Syrian hamsters on an atherogenic diet that consumed a raspberry juice containing anthocyanins and ellagitannins, equivalent to 218–305μg/mL–1 and 45–75μg/mL–1, respectively for 12 weeks, lowered plasma biomarker levels of early atherosclerosis such as triglycerides, total cholesterol, and low-density lipoprotein [28]. The vascular effects of red raspberries in healthy human subjects that received 200 g and 400 g of frozen red raspberries, improved flow-mediated dilation up to 24 hours [29]. Thus, literature shows that red raspberries have an indirect benefit related to vascular function. However, there are no documented studies that demonstrate a mechanistic effect of whole role red raspberries directly on vascular function in a MetS model.
The obese Zucker rat (OZR) is an ideal model for MetS since it expresses similar characteristics to that of human MetS. The OZR has a mutation in the leptin receptor gene (Lepr) (fa/fa). This mutation causes early-onset morbid obesity, hyperphagia, reduced energy expenditure, alteration in glucose homeostasis, dyslipidemia, and inflammation [30]. Additionally, the OZR develops endothelial dysfunction and increased vascular oxidative stress [31].
2Objective
As mentioned above, there is limited information available for red raspberries and their role on vascular function and mechanism of action associated with the MetS. Thus, to our knowledge this is the first study that investigates the effect of a red raspberry-enriched diet on phenylephrine (Phe)-induced vasoconstriction and acetylcholine (Ach)-induced vasodilation, aortic effluent concentration of PGI2 and TXA2, gene expression of aortic inducible NOS (iNOS), endothelial NOS (eNOS) and cyclooxygenase-2 (COX-2) in male obese Zucker rats (OZR), a model of MetS [32].
3Methods
3.1Animals
At 8 weeks of age, 32 obese Zucker rats (OZR) (fa/fa) and 32 lean controls (LZR) (Fa/Fa) were purchased from Charles River Laboratories (Raleigh, North Caroline). The rats were housed individually in stainless steel mesh-bottom cages in the Small Animal Facility at the University of Maine in an environmentally controlled room maintained at room temperature and light cycle (12:12 hours light:dark). Body weights were measured weekly, to monitor growth and possible weight differences among groups [5]. The experimental protocol was approved by the University of Maine Institution Animal Care and Use Committee (IACUC: A2017-01-06). Animals were treated in accordance with Guide for the Care and Use of Laboratory Animals (8th edition, National Academies Press).
3.2Diets
The rats were randomly assigned to a either a control diet AIN93G (C), or a whole red raspberry-enriched diet (WRR) (8% w/w) substituted for cornstarch in the C diet for 8 weeks. Supplementation of red raspberry powder is equivalent to a daily human dose of a cup and a half of fresh red raspberry [33]. Both diets were prepared at Dyets Inc. (Bethlehem, PA) and kept at -80°C in vacuum-packed UV protective bags until use. Freeze-dried WRR powder was provided by FutureCeuticals (Momence, IL). FutureCeuticals standardized the powder to a total 0.5% anthocyanins, 1.5% phenolics and 0.01% ellagic acid. Water and food were provided ad libitum. Food consumption was measured daily to monitor possible differences between the groups.
3.3Sample collection
At the end of the 8-week feeding period, animals were fasted overnight. They were anesthetized with CO2 for approximately 2 minutes and were exsanguinated by cardiac puncture. Blood was collected in a tube containing 200μl of a 5% EDTA solution and centrifuged immediately for 15 minutes at 2300 g for plasma separation and stored at –80°C until subsequent analysis.
The thoracic aorta was quickly harvested and placed in a physiological salt solution (PSS) (composed of: 118 mM NaCl, 4.7 mM KCl, 25 mM NaHCO3, 1.18 mM KH2PO4, 1.17 mM MgSO4, 11 mM dextrose, 1.25 mM CaCl), and was cleaned of blood clots and connective tissue, as previously described [15]. The middle segment of the aorta was sectioned into four rings (approximately 3 mm length) and used immediately for the evaluation of vasoconstriction or vasorelaxation. The remaining end of the aorta was sectioned into three pieces (approximately 1 mm length) and used for effluent measurements. The arch of the aorta was snap-frozen in liquid nitrogen and stored at –80°C until further analysis.
3.4Endothelial function
Vascular studies were conducted on isolated aortic ring preparations to measure Phe-induced vasoconstriction and Ach-induced vasodilation. The four aortic rings were suspended between two stainless-steel triangles and submerged in 20 mL Radnoti tissue baths (Radnoti Glass Technology Inc., Monrovia, CA) that contained PSS at 37°C aerated with 95% O2/5% CO2 gas mix (pH 7.4). The bottom triangle was fixed to the tissue bath and the top triangle was connected to a tissue force analyzer (TFA Model 410; Micro-Med Inc., Louisville KY) that recorded the force (g) developed by the aortic ring using DMSI-450 software (version 1.01: Micro-Med Inc.).
The isolated rings were pre-loaded with a baseline tension of 1.50±0.01 g and pre-conditioned with a single dose of Ach (10–8 M) and Phe (10–8 M). Each aortic ring was randomly assigned to either a treatment that inhibits nitric oxide (NO) synthase with L-N-monomethyl-arginine (L-NMMA) or a treatment which inhibits cyclooxygenase (COX1 and COX2) with mefenamic acid (MFA); or a treatment with no inhibitor.
3.5Phenylephrine (Phe)-induced vasoconstriction
A Phe dose-response curve was generated using the four aortic rings from each animal to evaluate vasoconstriction, as previously described [16]. The aortic rings were exposed to six cumulative Phe doses (from 10–8 to 3×10–6 M) and were allowed to reach maximum vasoconstriction force for 6 minutes. A single dose of Ach (3×10–6 M) was added following the last dose of Phe to determine endothelial integrity through the vasorelaxation response. For each ring, the maximum force of contraction (Fmax), the effective concentration at 50% of maximum response (EC50), and the vessel sensitivity (pD2, -log10 EC50) were determined.
3.6Acetylcholine (Ach)-induced vasodilation
An Ach dose-response curve was generated using four aortic rings per rat to evaluate vasodilation, as previously described [16]. Each aortic ring was exposed to single dose of Phe (3×10–6 M) for 10 minutes which allowed the vessel to reach maximum vasoconstriction. Then six cumulative Ach doses (from 10–8 to 3×10–6 M) were introduced to each of the tissue baths, to allow the aortic rings to reach maximum vasorelaxation for 6 minutes after each dose. The maximum relaxation value at each dose of Ach was expressed as a percentage from the Phe-induced preconditioned force (0% vasorelaxation). The effective concentration at 50% of vasorelaxation response (EC 50) and vessel sensitivity (pD2, -log10 EC 50) were determined.
3.7Aortic effluent: Prostacyclin I2 (PGI2) and Thromboxane A2 (TXA2)
The remaining segment of the aorta from each animal was incubated in a 2 mL Radnoti tissue bath under the same conditions mentioned above. The aorta pieces were allowed to equilibrate for 20 minutes before being exposed to a single dose of Phe (10–6 M) for 10 minutes followed by Ach (10–6 M) for 10 minutes to stimulate prostanoid release, as previously described [16]. The medium was collected and stored at –80°C until further analysis. The aortic tissues were dried at room temperature and weighed. Estimated levels of PGI2 (competitive ELISA kit 6-keto- PGF1α (6 kPGF1α) (Cayman, 515211)) and TXA2 (competitive ELISA kit Thromboxane B2 (TXB2) (Cayman, 501020)) were determined. Results were normalized to the dry weight of the aorta section used.
3.8Real-time reverse transcription polymerase chain reaction (PCR)
Total RNA from frozen aortic tissue was isolated with RNeasy Mini Kit (Qiagen, Valencia, CA) and RNeasy Lipid Tissue Mini Kit (Qiagen, Valencia, CA), respectively following the manufacturer’s protocol. Samples were analyzed by custom RT2 Profiler PCR Array (Qiagen, Valencia, CA). Briefly, cDNA was prepared from purified RNA using RT2 First Strand Kit. cDNA was added to RT2 SYBR Green MasterMix. This mixture was aliquoted into the custom 96-well plate that contained species-specific primers for experimental genes (iNOS, eNOS, and COX2) and controls (housekeeping genes (β-actin and lactate dehydrogenase A) (Qiagen). Relative gene expression of iNOS, eNOS, and COX2 were determined by the ΔΔCt method as described by Livak and Schmittgen [34], relative to the average of the housekeeping genes β-actin and lactate dehydrogenase A, and expressed as the fold-variation following WRR treatment compared to the C animals.
3.9Plasma nitric oxide
Plasma samples were analyzed for NO concentration in plasma samples using Total Nitric Oxide and Nitrate/Nitrite Parameter Kit (R&D Systems, KGE001), following the instructions provided by the manufacturer. The colorimetric assay is based on a spectrophotometric detection of a Griess Reaction performed at 540 nm with a correction length at 690 nm on a Synergy 2 multi-detection microplate reader, BioTek Instruments, Inc. (Winooski, VT).
3.10Statistical analysis
A two-way analysis of variance (ANOVA) was used to examine the influence of dietary treatment (C vs. WRR) and animal model (LZR vs. OZR). The differences between means were further analyzed using the Tukey’s Honestly Significant Difference (HSD) test. Results were expressed as±SEM and considered significant at p < 0.05. Statistical analysis was performed using GraphPad Prism 7 version 7.0 d statistical software version (GraphPad Software, La Jolla, CA).
4Results
4.1Animal weight and food intake
The average weight of the animals at 8-weeks of age was significantly higher in the OZR (394±47 g) compared to their litter mates (265±36 g) (p < 0.05). The average weight of the animals at 16 weeks of age was significantly higher in the OZR (608±74 g) compared to the LZR group (434±68 g) (p < 0.05). There was no significant difference within each diet group between the C and WRR animals.
Average daily food consumption was significantly higher in the OZR (36.3±1.054 g) compared to the LZR group (26.5±0.729 g) (p < 0.05). Within each diet group (C vs. WRR) the daily food consumption was not significant.
4.2Phe-induced vasoconstriction
The Phe-induced vasoconstriction dose response curve is shown in Fig. 1. In the absence of inhibitors, the maximal force of contraction induced by Phe was significantly lower in the OZR-C (Fmax 0.75±0.05 g) compared with the LZR-C (Fmax 1.15±0.05 g, p < 0.05) (Fig. 1A). Consumption of WRR partially restored Phe-induced vasoconstrictor responses in OZR, with a significant increase in the maximal force of contraction (Fmax 0.98±0.03 g p < 0.05) (Fig. 1A). The overall maximal tension of aorta rings with the pre-treatment of NO inhibitor, L-NMMA increased (Fig. 1B). The Phe-induced vasoconstrictor response significantly increased the maximal force of the OZR assigned to a WRR diet compared to OZR-C (Fmax 2.03±0.12 g and Fmax 1.82±0.14 g, p < 0.05 respectively) (Fig. 1B). Following pre-treatment of rings with MFA, a COX pathway inhibitor, the vasoconstrictor response was significantly lower in the OZR-C (Fmax 0.95±0.14 g) compared to LZR-C ((Fmax 1.34±0.09 g, p < 0.05) (Fig. 1C). No significant differences were observed between treatment group in the OZR (C vs WRR).
Fig. 1
(A-C): Phenylephrine (Phe)-induced vasoconstriction dose response curve generated in the lean Zucker rat (LZR) and obese Zucker rat (OZR). A. Phe-induced vasoconstriction response in the absence of the inhibitor. B. Phe-induced vasoconstriction response with the pre-treatment of L-N-monomethyl-arginine (L-NMMA). C. Phe-induced vasoconstriction response with the pre-treatment of Mefenamic Acid (MFA). Note: Physiological Salt Solution (PSS); L-N-monomethyl-arginine (L-NMMA); Mefenamic Acid (MFA). The values are expressed as means±SEM (n = 8 rats per treatment group). aSignificant effect of animal type, LZR-C vs OZR-C (p < 0.05). bSignificant effect of diet, OZR-C vs. OZR-WRR (p < 0.05)
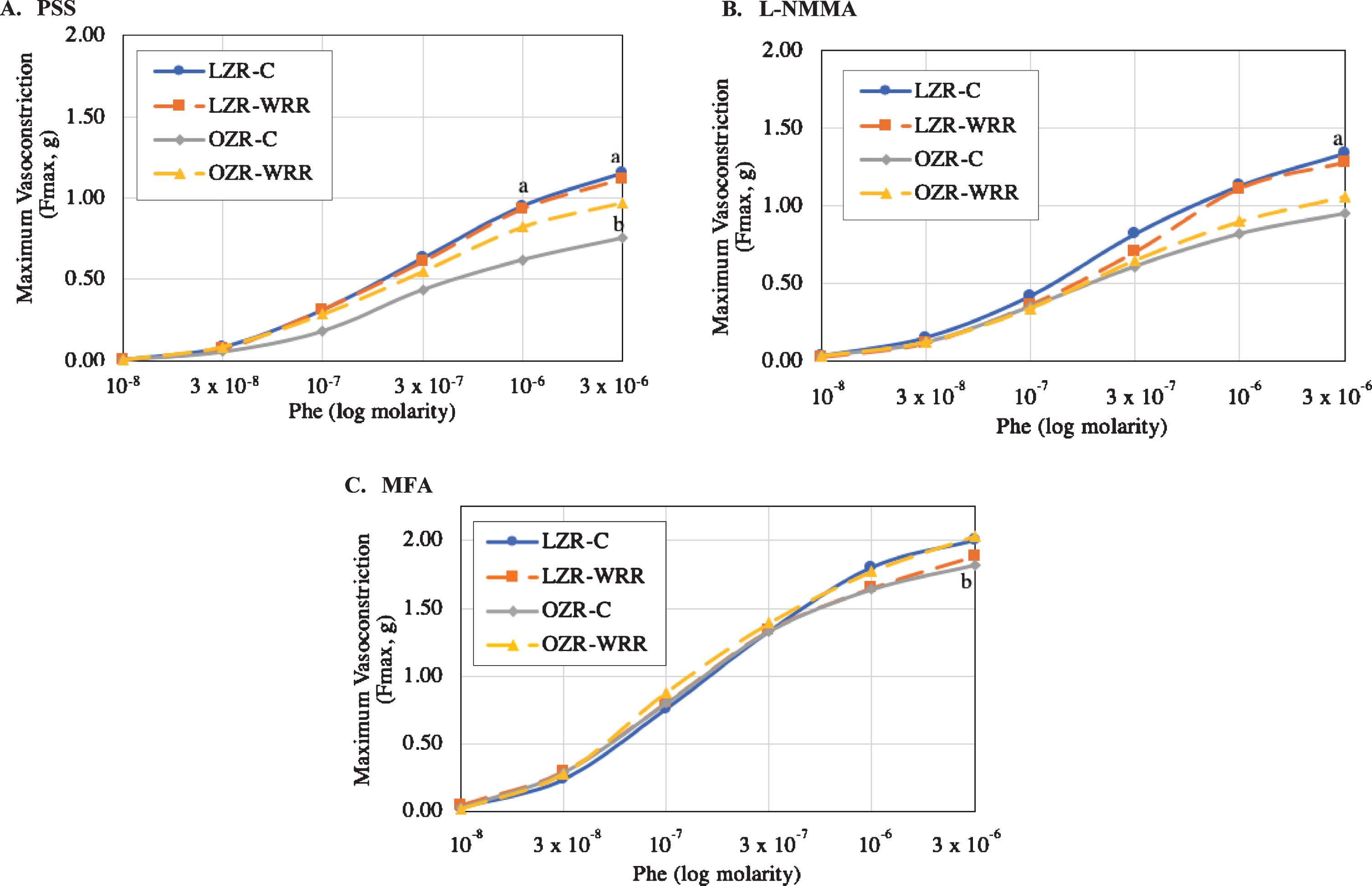
When animals were treated with PSS, vessel sensitivity (pD2), shown in Table 1, was similar between animal type and was unaffected by diet. Pre-treatment with L-NMMA significantly increased the vessel sensitivity in both LZR and OZR (p < 0.05) and the LZR-WRR. No significant differences were observed either with animal type or diet with MFA pre-treatment.
Table 1
Vasoconstriction Vessel Sensitivity (pD2) in the lean Zucker rat (LZR) and obese Zucker rat (OZR) with pre-treatment of no inhibitor (PSS), L-N-monomethyl-arginine (L-NMMA). Mefenamic Acid (MFA)
Treatment | LZR-C | LZR-WRR | OZR-C | OZR-WRR |
PSS | 6.54±0.06a | 6.56±0.07a | 6.61±0.04a | 6.68±0.08 |
PSS+L-NMMA | 6.81±0.05 | 6.88±0.06 | 6.91±0.04 | 6.89±0.07 |
PSS+MFA | 6.66±0.06 | 6.61±0.04 | 6.79±0.07 | 6.69±0.06 |
Note: PSS (physiological salt solution); L-NMMA (L-N-monomethyl-arginine); MFA (mefenamic acid). The values are expressed as means±SEM (n = 8 rats per treatment group). aSignificant effect with treatment, PSS vs L-NMMA (p < 0.05)
4.3Ach-induced vasorelaxation
The Ach-induced vasorelaxation dose response curve is illustrated in Fig. 2. In the absence of inhibitors, the OZR-C exhibited amplified relaxation response to Ach compared to the LZR-C at the first two doses, 10–8 and 3×10–8M (61.64±4.88 % and 92.30±1.98 % vs. 29.19±3.84 % and 71.40±4.58 %, p < 0.05, respectively) (Fig. 2A). An attenuated Ach-induced vasorelaxation response was observed in the OZR-WRR at 10–8 and 3×10–8M (42.12±5.02 % and 80.69±4.41 %, p < 0.05, respectively) compared with OZR-C in the absence of inhibitors (Fig. 2A). Following pre-treatment with L-NMMA, the maximum relaxation response was higher in the OZR-C compared to the LZR-C (80.37±2.95 % vs. 60.65±4.67 %, p < 0.05, respectively) (Fig. 2B). The maximal vasorelaxation response in OZR-WRR (70.99±3.02 %, p < 0.05) was lower compared to the OZR-C in the presence of L-NMMA (Fig. 2B). An amplified vascular response of the OZR-C was observed compared to the LZR-C at 10–8, 3×10–8 and 10–7M (26.09±3.44 %, 56.30±2.03 % and 82.76±1.17 % vs 45.65±6.13 %, 76.53±4.04 % and 95.90±1.44 %, p < 0.05, respectively) following pre-treatment of MFA (Fig. 2C). Consumption of WRR in the OZR partially attenuated the amplified response observed in the OZR-C (30.08±3.66 %, 55.58±4.28 % and 82.66±2.78 %, p < 0.05, respectively) as shown in Fig. 2C.
Fig. 2
(A-C): Acetylcholine (Ach)-induced dose response curve generated in the lean Zucker rat (LZR) and obese Zucker rat (OZR). A. Phe-induced vasoconstriction response in the absence of the inhibitor. B. Phe-induced vasoconstriction response with the pre-treatment of L-N-monomethyl-arginine (L-NMMA). C. Phe-induced vasoconstriction response with the pre-treatment of Mefenamic Acid (MFA). Note: Physiological Salt Solution (PSS); L-N-monomethyl-arginine (L-NMMA); Mefenamic Acid (MFA). The values are expressed as means±SEM (n = 8 rats per treatment group). aSignificant effect of animal type, LZR-C vs OZR-C (p < 0.05). bSignificant effect of diet, OZR-C vs OZR-WRR (p < 0.05)
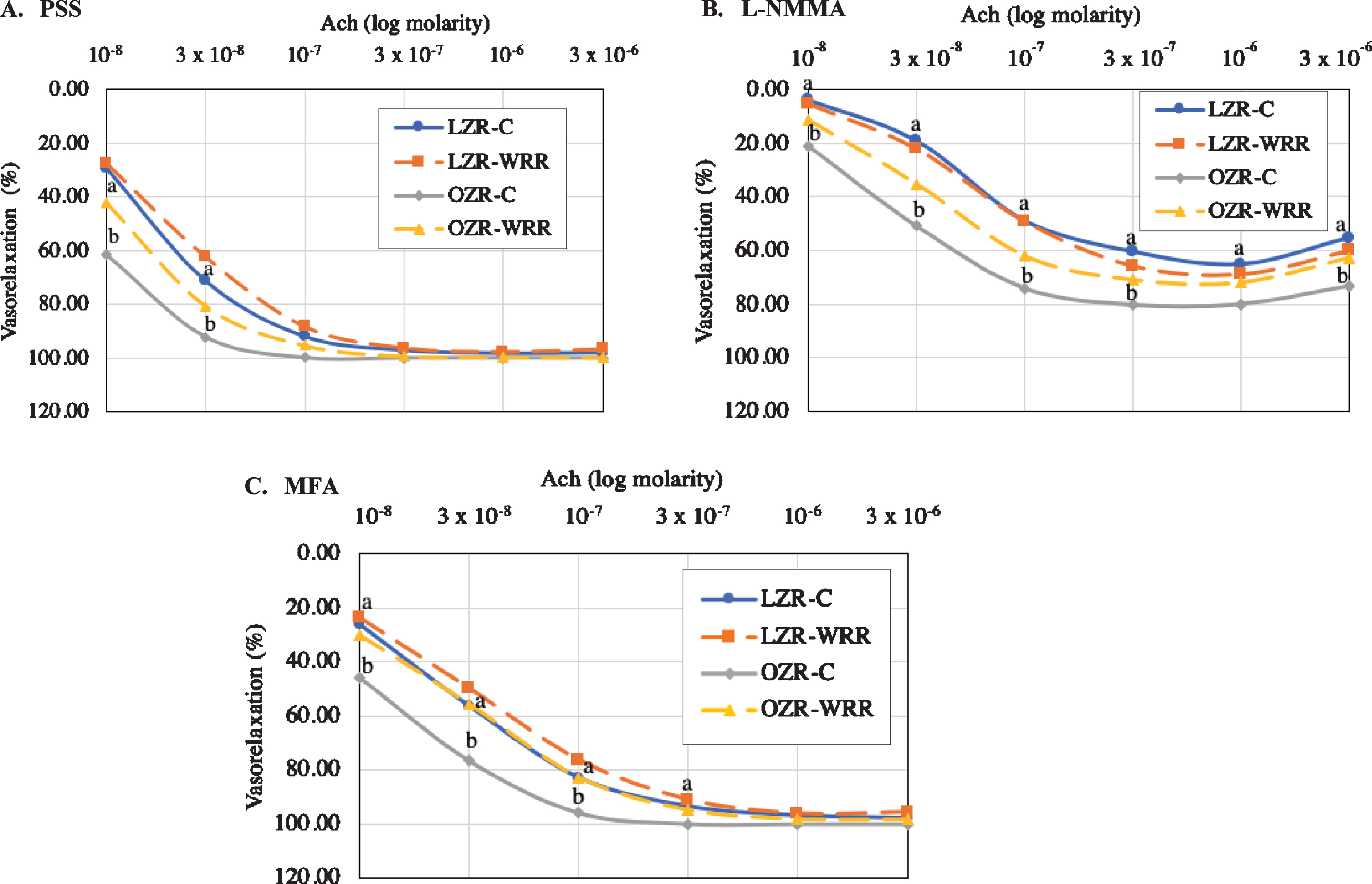
Shown in Table 2, the pD2 of Ach-induced vasorelaxation was higher in the OZR compared with LZR, in the absence of the inhibitors (7.63±0.58 vs 7.28±0.04, p < 0.05) and following L-NMMA treatment (7.09±0.11 vs 6.45±0.16, p < 0.05). The pD2 was significantly higher in the absence of the inhibitors compared with the pre-treatment of L-NMMA in all groups (p < 0.05). Additionally, the OZR assigned to a WRR diet had a significantly lower pD2 with the pre-treatment of MFA (7.15±0.08, p < 0.05) compared to the treatment with no inhibitors.
Table 2
Vasorelaxation Vessel Sensitivity (pD2) in the lean Zucker rat (LZR) and obese Zucker rat (OZR) with pre-treatment of no inhibitor (PSS), L-N-monomethyl-arginine (L-NMMA). Mefenamic Acid (MFA)
Treatment | LZR-C | LZR-WRR | OZR-C | OZR-WRR |
PSS | 7.28±0.04a,b | 7.27±0.06b | 7.63±0.58b | 7.48±0.09b,c |
PSS+L-NMMA | 6.45±0.16 | 6.48±0.10 | 7.10±0.11 | 6.90±0.10 |
PSS+MFA | 7.21±0.10 | 6.94±0.12 | 7.39±0.52 | 7.15±0.08 |
Note: PSS (physiological salt solution); L-NMMA (L-N-monomethyl-arginine); MFA (mefenamic acid). The values are expressed as means±SEM (n = 8 rats per treatment group). aSignificant effect of animal type, LZR-C vs OZR-C (p < 0.05). bSignificant effect between treatment, PSS vs L-NMMA (p < 0.05). cSignificant effect between treatment, PSS vs MFA (p < 0.05)
4.4Prostanoids and NO
Aortic effluent prostanoids and plasma NO concentrations are reported in Table 3. In the aortic effluent, 6kPGF1α was significantly higher in the OZR compared to the LZR group (p < 0.05). The concentration of 6ketoPGF1α significantly decreased in the OZR assigned a WRR diet (p < 0.05). The aortic effluent concentration of TXB2 and the NO plasma levels were unaffected among animal type or diet groups.
Table 3
Concentration of aortic effluent prostanoids (6kPGF1α, TXB2) and plasma NO concentrations of lean (LZR) and obese (OZR) Zucker rats assigned a control (C) or whole red raspberry (WRR) diet
LZR-C | LZR-WRR | OZR-C | OZR-WRR | |
6kPGF1α (pg/mg of aorta) | 5523.36±359.29a | 5789.34±574.32 | 9644.71±1460.37b | 4905.91±550.02 |
TXB2 (pg/mg of aorta) | 47.83±5.43 | 44.84±11.38 | 61.36±16.15 | 43.59±12.34 |
Plasma NO (μmol/L) | 9.97±1.01 | 13.28±1.41 | 16.61±2.67 | 15.04±2.31 |
Note: 6kPGF1α (6-keto-prostaglandin F1α); TXB2 (Thromboxane B2); NO (Nitric Oxide). The values are expressed as means±SEM (n = 8 rats per treatment group). aSignificant effect of animal type, LZR-C vs OZR-C (p < 0.05). bSignificant effect of diet, OZR-C vs OZR-WRR (p < 0.05)
4.5Aortic mRNA expression
In Fig. 3A, there was no effect on aortic iNOS expression with animal type or diet intervention. The aortic expression of eNOS (Fig. 3B) and COX-2 (Fig. 3C) was upregulated in OZR-C compared with LZR-C (p < 0.05). Following consumption of WRR, aortic expression of eNOS (Fig. 3B) and COX-2 (Fig. 3C) were downregulated in the OZR-WRR (p < 0.05).
Fig. 3
(A-C): Relative gene expression of the aorta of lean (LZR) and obese (OZR) Zucker rats assigned a control (C) or whole red raspberry (WRR) diet: A. inducible nitric oxide (iNOS), B. endothelial nitric oxide (eNOS) and C. cyclooxygenase-2 (COX-2). Note: iNOS (inducible nitric oxide synthase); eNOS (endothelm nitric oxide); COX-2 (cyclooxygenase-2). The values are expressed as means±SEM (n = 8 rats per treatment group). aSignificant effect of animal type, LZR-C vs OZR-C (p < 0.05). bSignificant effect of diet, OZR-C vs OZR-WRR (p < 0.05)
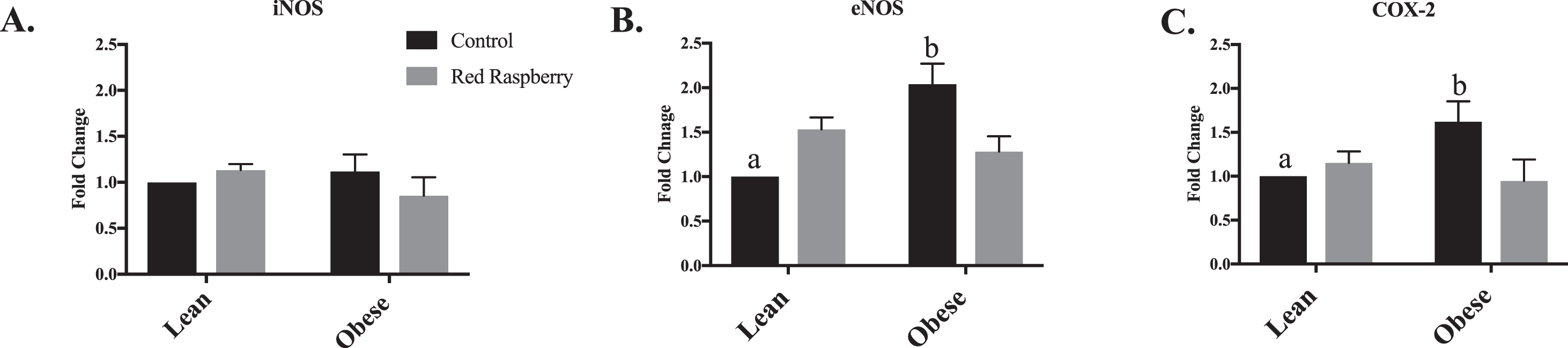
5Discussion
These results demonstrated that 8-weeks of WRR consumption partially normalized the vascular function of the OZR aorta by restoring the impaired Phe-induced constrictor response and attenuating the amplified Ach-induced relaxation response. To our knowledge, this is the first study to investigate the role of WRR on vascular function.
It has been previously demonstrated that OZRs develop a dysfunctional vasoconstriction and vasorelaxation mechanical response compared to their lean littermates independent of vessel type and age [16, 35–42]. However, endothelial-derived relaxing factors (EDRF) and endothelial-derived constricting factors (EDCF) including NO and prostanoids are inconsistent among studies.
In our study, the OZR exhibited an attenuated Phe-induced vasoconstrictor response, developing a lower maximal force compared to LZR, with no significant difference in vessel sensitivity. Other studies have also demonstrated inhibition of Phe-induced vasoconstriction response in the OZR in the thoracic aorta at 16 weeks of age [16, 41], in thoracic, coronary and mesenteric arteries at 16 to 40 weeks of age [36], and in pulmonary arteries at 17 to 18 weeks of age [42]. The WRR diet partially restored the impaired vasoconstrictor response in the OZR-C in the absence of inhibitors. L-NMMA pre-treatment increased the maximal force of contraction in the OZR, suggesting their involvement on the NO pathway, while no significant change was observed with MFA pre-treatment, suggesting that red raspberry involvement on the COX pathway may be secondary to the NO pathway.
With regard to Ach-induced vasorelaxation, the OZR-C exhibited an amplified relaxation response compared to LZR-C at the first two doses 10–8 and 3×10–8M. Vendrame et al. documented similar OZR and LZR responses [16]. A WRR diet decreased the maximal relaxation in the OZR. With the inhibition of the NO pathway with L-NMMA, the OZR that consumed a WRR-enriched diet exhibited a lower relaxation response, indicating the involvement of the NO pathway. An amplified vascular function of the OZR-C was observed compared to the LZR-C at the first three doses 10–8, 3×10–8 and 10–7M following pre-treatment of MFA. With consumption of the WRR diet, vascular function in the OZR at these three doses improved but not completely, suggesting the partial involvement of the COX pathway. Similar results to our study were reported in aortic rings of 16-weeks of age OZR with supplementation of wild blueberries [16]. A possible explanation for the decreased vasoconstriction response to Phe and the heightened vasorelaxation response in the OZR may be due to compensatory mechanisms of the dysfunctional endothelium of the OZR that agrees with the proteomic and genomic results of this study. Ellagic acid, a compound found in abundance in red raspberries increased vasorelaxation activity of aorta of 10–12 week old male Wistar rat [43]. Moreover, ethyl acetate extract from red raspberries decreased blood pressure of spontaneously hypertensive rats at 15 weeks of age [44]. With the consumption of WRR, the OZR partially restored vascular response in the presence and absence of the inhibitors suggesting a partial involvement of both the NOS and COX pathways.
Nitric oxide is a powerful vasodilator synthesized by eNOS which is expressed mainly in endothelial cells [45], inhibits platelet and leukocyte aggregation and adhesion, DNA synthesis, mitogenesis, and proliferation of vascular smooth muscle cells thus promoting blood fluidity and preventing thrombosis [46]. No changes were observed in plasma levels of NO and expression of iNOS between animal type and diet group but gene expression of aorta eNOS, was significantly upregulated in the OZR compared to LZR. It has been documented that in conditions of inflammatory and oxidative stress of cardiac function, there is an overproduction of NO [47]. Human umbilical vein endothelial cells exposed to chronic and acute flow conditions resulted in elevated eNOS mRNA expression and protein levels [48]. It has been proposed that the overexpression of eNOS may enhance blood flow by attenuating the vasoconstrictor response during heart failure to increase cardiac output and survival [45]. Following the consumption of WRR, expression of aortic eNOS in the OZR was significantly down-regulated, similar to that of the LZR. This result is in line with our observations that WRR consumption improved the vasoconstriction and vasorelaxation response in the OZR. Activity of eNOS within the endothelial cells is modulated by several factors including reactive oxygen species (ROS) [49]. Previous studies have demonstrated that anthocyanin-enriched extracts of blackberry, black raspberry, blueberry, cranberry, red raspberry and strawberry possess free radical scavenging activity due to their anti-oxidant profiles [50]. Dihydrobenzofuran-type enantiomeric neolignans isolated from red raspberries attenuated ROS generation in SH-SY5Y cells [51]. Additionally, red raspberry leaf extract has been shown to modulate blood platelet reactivity and aggregation in ADP-stimulated blood, possibly via the modulation of the redox status [52]. These studies in addition to our data suggest that the antioxidant activity of WRR may modulate factors associated with endothelial dysfunction by positively influencing the activity of eNOS from a compensatory to a regulatory role.
Nitric oxide and prostacyclin, a product of the COX pathway, work closely together for maintenance of vascular tone [46]. Compromised release of one of these molecular entities places results in a dysfunctional endothelium, increasing the risk of cardiovascular events [46]. The OZR presented an increase in COX-2 activity compared to its lean littermate. Moreover, the expression of COX-2 in the OZR assigned a WRR diet was down-regulated. Anthocyanins have been shown to down-regulate expression and activity of COX-2 [53–57]. In particular, the anthocyanin fraction of red raspberries containing cyanidin-3-sophoroside, cyanidin-glucoside, cyanidin-rutinose and cyanidin-glucosylrutinose demonstrated a 47% COX-2 inhibition when tested against a COX-inhibitory assay [53]. Ellagic acid suppressed COX-2 expression in human monocytes pre-treated with lipopolysaccharide [57]. As we expected with the shift to COX-2, the concentration of PGI2 was elevated in the OZR-C compared to LZR in the aortic effluent. With the consumption of WRR, PGI2 in the aortic effluent was attenuated in the OZR. Supplementation of anthocyanin extract from black rice composed of predominately cyanidin-3-glucoside decreased serum TXA2 and PGI2 ratio in rats fed a high-fat diet [58]. In our study, aortic effluent concentration of TXA2 did not change. Plasma concentration of TXA2 was not altered with supplementation of 8% wild blueberries in spontaneously hypertensive rats [5]. One explanation for these observations is that the COX pathway is responsible from the production of prostanoids through COX-1 and COX-2 activity. COX-1 is responsible for the production of TXA2, whereas the activation of COX-2 by inflammatory stimuli shifts prostanoid synthesis to favor PGI2 and PGE2 [59, 60]. It has been documented in numerous studies that COX-2 is upregulated with the MetS and in coronary arteries from insulin-resistant obese Zucker rat [61] and HUVECs stimulated with IL-1β [62]. It has been proposed that MetS up-regulates COX-2 by enhancing the release of relaxant prostaglandins thus playing a protective role on endothelial function [61].
These results of upregulation of EDRF’s, eNOS and COX-2 and increase in PGI2 concentration, mirror our observations on vascular tone of a lessened vasoconstriction and an heighten vasorelaxation response of the OZR compared to the LZR, independent of diet. Supplementation of WRR was able to modulate vascular tone in the OZR by down-regulating eNOS and COX-2 and decreasing PGI2 concentration in the aortic effluent. We speculate that the observed results are due to WRR’s antioxidant and vasodilatory properties; acting as activators or inhibitors of multiple signaling pathways of the vasculature, protecting vessels against endothelial dysfunction.
Results from this study suggest that the dysfunctional aorta of the OZR is protected by COX-2 production of vasodilator prostaglandins as a compensatory response. The consumption of WRR improved vascular function in the OZR by down-regulating the expression of eNOS and COX-2 in the aorta. The decrease in COX-2 activity resulted in a decrease in the synthesis of PGI2. This aligns with this study’s mechanistic observations in this study of a heightened Phe-induced vasoconstriction response and an attenuated Ach-induced vasorelaxation response in the OZR that consumed the WRR diet.
To our knowledge this is the first study to demonstrate that 8-week consumption of WRR improves the vascular mechanical properties of the impaired vasoconstriction and vasodilation function of the OZR and reveals that red raspberries operate through the involvement of NO pathway and COX pathway. These results combined with previous research document that supplementation of diets with WRR may play an important role in improving vascular dysfunction, associated with the MetS.
Conflict of interest:
The authors have no conflict of interest to report.
Acknowledgments
This work was supported by the National Processed Raspberry Council. This project was also supported by the USDA National Institute of Food and Agriculture Hatch project number #ME0-31910 through the Maine Agricultural and Forest Experiment Station publication number 3677. Additionally, the red raspberry powder was generously provided by FutureCeuticals (Momence, IL).
References
[1] | Aquilante CL , Vande Griend JP . Metabolic Syndrome: Clinical and Policy Implications of the New Silent Killer. Pharmacother Self-Assessment Program, 6th Ed 2017;109-128. |
[2] | Lam D , LeRoith D . Metabolic Syndrome. nternational J Biol Sci. (2015) ;7: :1003. |
[3] | Klimis-Zacas D , Vendrame S , Kristo AS . Wild blueberries attenuate risk factors of the metabolic syndrome. J Berry Res. (2016) ;6: :225–36. |
[4] | Steinberg H , Baron A . Vascular function, insulin resistance and fatty acids. Diabetologia. (2002) ;45: :623–34. |
[5] | Kristo AS , Kalea AZ , Schuschke DA , et al. Attenuation of alpha-adrenergic-induced vasoconstriction by dietary wild blueberries (Vaccinium angustifolium) is mediated by the NO-cGMP pathway in spontaneously hypertensive rats (SHRs). Int J Food Sci Nutr. (2013) ;64: :979–87. |
[6] | Suganya N , Bhakkiyalakshmi E , Sarada DVL , et al. Reversibility of endothelial dysfunction in diabetes: role of polyphenols. Br J Nutr. (2016) ;116: :223–46. |
[7] | Kleinbongard P , Dejam A , Lauer T , et al. Plasma nitrite reflects constitutive nitric oxide synthase activity in mammals. Free Radic Biol Med. (2003) ;35: :790–6. |
[8] | Napoli C , de Nigris F , Williams-Ignarro S , et al. Nitric oxide and atherosclerosis: An update. Nitric Oxide - Biol Chem. (2006) ;15: :265–79. |
[9] | Vanhoutte PM . Endothelial control of vasomotor function: from health to coronary disease. Circ J. (2003) ;67: :572–5. |
[10] | Paul BZS , Jin J , Kunapuli SP . Molecular Mechanism of Thromboxane A2-induced Platelet Aggregation. J Biol Chem. (1999) ;274: :29108–14. |
[11] | Tziomalos K , Athyros VG , Karagiannis A , et al. Endothelial dysfunction in metabolic syndrome: Prevalence, pathogenesis and management. Nutr Metab Cardiovasc Dis. (2010) ;20: :140–6. |
[12] | Oak M-H , Auger C , Belcastro E , et al. Potential mechanisms underlying cardiovascular protection by polyphenols: Role of the endothelium. Free Radic Biol Med. Epub ahead of print 2018. DOI:10.1016/j.freeradbiomed.2018.03.018. |
[13] | Norton C , Kalea AZ , Harris PD , et al. Wild blueberry-rich diets affect the contractile machinery of the vascular smooth muscle in the Sprague-Dawley rat. J Med Food. (2005) ;8: :8–13. |
[14] | Kalea A , Clark K , Schuschke D , et al. Vascular reactivity is affected by dietary consumption of wild blueberries in the Sprague-Dawley rat. J Med Food. 2009;21-8. |
[15] | Kalea AZ , Clark K , Schuschke DA , et al. Dietary enrichment with wild blueberries (Vaccinium angustifolium) affects the vascular reactivity in the aorta of young spontaneously hypertensive rats. J Nutr Biochem. (2010) ;21: :14–22. |
[16] | Vendrame S , Kristo AS , Schuschke D a , et al. Wild blueberry consumption affects aortic vascular function in the obese Zucker rat. Appl Physiol Nutr Metab Appl Nutr Metab. (2014) ;39: :255–61. |
[17] | Kalea AZ , Lamari FN , Theocharis AD , et al. Wild blueberry (Vaccinium angustifolium) consumption affects the composition and structure of glycosaminoglycans in Sprague-Dawley rat aorta. J Nutr Biochem. (2006) ;17: :109–16. |
[18] | Kristo AS , Malavaki CJ , Lamari FN , et al. Wild blueberry (V. angustifolium)-enriched diets alter aortic glycosaminoglycan profile in the spontaneously hypertensive rat. J Nutr Biochem. (2012) ;23: :961–5. |
[19] | Cevik N , Turker G , Kizilkaya B . The Phenolic Compounds in Berries: Beneficial Effects on Human Health. New Knowl J Sci. 2013;52-5. |
[20] | Venketeshwer Rao A , Snyder DM . Raspberries and Human Health: A Review. J Agric Food Chem. (2010) ;58: :3871–83. |
[21] | Zhu MJ , Kang Y , Xue Y , et al. Red raspberries suppress NLRP3 inflammasome and attenuate metabolic abnormalities in diet-induced obese mice. J Nutr Biochem. (2018) ;53: :96–103. |
[22] | Noratto G , Chew BP , Ivanov I . Red raspberry decreases heart biomarkers of cardiac remodeling associated with oxidative and inflammatory stress in obese diabetic db/db mice. Food Funct. (2016) ;7: :4944–55. |
[23] | Noratto GD , Chew BP , Atienza LM . Red raspberry (Rubus idaeus L.) intake decreases oxidative stress in obese diabetic (db/db) mice. Food Chem. (2017) ;227: :305–14. |
[24] | Sousa M , MacHado V , Costa R , et al. Red Raspberry Phenols Inhibit Angiogenesis: A Morphological and Subcellular Analysis Upon Human Endothelial Cells. J Cell Biochem. (2016) ;117: :1604–12. |
[25] | Zhou L , Lou L-L , Wang W , et al. Enantiomeric 8-O-4′ type neolignans from red raspberry as potential inhibitors of β-amyloid aggregation. J Funct Foods. (2017) ;37: :322–9. |
[26] | Stoner GD , Wang LS , Seguin C , et al. Multiple berry types prevent N-nitrosomethylbenzylamine-induced esophageal cancer in rats. Pharm Res. (2010) ;27: :1138–45. |
[27] | Kirakosyan A , Seymour EM , Kondoleon N , et al. The intake of red raspberry fruit is inversely related to cardiac risk factors associated with metabolic syndrome. J Funct Foods. (2018) ;41: :83–9. |
[28] | Suh J-H , Romain C , González-Barrio R , et al. Raspberry juice consumption, oxidative stress and reduction of atherosclerosis risk factors in hypercholesterolemic golden Syrian hamsters. Food Funct. (2011) ;2: :400–5. |
[29] | Istas G , Feliciano RP , Weber T , et al. Plasma urolithin metabolites correlate with improvements in endothelial function after red raspberry consumption: A double-blind randomized controlled trial. Arch Biochem Biophys. Epub ahead of print 2018. DOI:10.1016/J.ABB.2018.05.016. |
[30] | Clément K , Vaisse C , Lahlou N , et al. A mutation in the human leptin receptor gene causes obesity and pituitary dysfunction. Nature. (1998) ;392: :398–401. |
[31] | Sista AK , O’Connell MK , Hinohara T , et al. Increased aortic stiffness in the insulin-resistant Zucker fa / fa rat. (2019) ;94305: :845–51. |
[32] | Aleixandre de Artiñano A , Miguel Castro M . Experimental rat models to study the metabolic syndrome. Br J Nutr. (2009) ;102: :1246. |
[33] | Reagan-Shaw S , Nihal M , Ahmad N . Dose translation from animal to human studies revisited. FASEB J. (2008) ;22: :659–61. |
[34] | Livak KJ , Schmittgen TD . Analysis of Relative Gene Expression Data Using Real-Time Quantitative PCR and the 2-ΔΔCT Method. Methods. (2001) ;25: :402–8. |
[35] | Oltman CL , Coppey LJ , Gellett JS , et al. Progression of vascular and neural dysfunction in sciatic nerves of Zucker diabetic fatty and Zucker rats. Am J Physiol Metab. (2005) ;289: :E113–E122. |
[36] | Oltman CL . Progression of coronary and mesenteric vascular dysfunction in Zucker obese and Zucker diabetic fatty rats. AJP Hear Circ Physiol. (2006) ;291: :H1780–H1787. |
[37] | Vessières E , Belin de Chantemèle EJ , Toutain B , et al. Cyclooxygenase-2 inhibition restored endothelium-mediated relaxation in old obese Zucker rat mesenteric arteries. Front Physiol. (2010) ;1: :1–10. |
[38] | Romanko OP , Stepp DW . Reduced constrictor reactivity balances impaired vasodilation in the mesenteric circulation of the obese Zucker rat. Am J Physiol Circ Physiol. (2005) ;289: :H2097–H2102. |
[39] | Davidson EP , Coppey LJ , Kleinschmidt TL , et al. Vascular and neural dysfunctions in obese Zucker rats: effect of AVE7688. Exp Diabetes Res. (2009) ;2009: :912327. |
[40] | Lemaster K , Jackson D , Welsh D , et al. Altered Distribution of Adrenergic Constrictor Responses Contributes to Sketetal Muscle. Microcirculation. (2017) ;24: :1–27. |
[41] | Rodriguez-Rodriguez R , Jiménez-Altayó F , Alsina L , et al. Mediterranean tomato-based sofrito protects against vascular alterations in obese Zucker rats by preserving NO bioavailability. Mol Nutr Food Res. (2017) ;61: :1–11. |
[42] | Moral-Sanz J , Menendez C , Moreno L , et al. Pulmonary arterial dysfunction in insulin resistant obese Zucker rats. Respir Res. (2011) ;12: :1–10. |
[43] | Yilmaz B , Usta C . Ellagic acid-induced endothelium-dependent and endothelium-independent vasorelaxation in rat thoracic aortic rings and the underlying mechanism. Phyther Res. (2013) ;27: :285–9. |
[44] | Jia H , Liu JW , Ufur H , et al. The antihypertensive effect of ethyl acetate extract from red raspberry fruit in hypertensive rats. Pharmacogn Mag. (2011) ;7: :19–24. |
[45] | Jones SP , Greer JJM , van Haperen R , et al. Endothelial nitric oxide synthase overexpression attenuates congestive heart failure in mice. Proc Natl Acad Sci. (2003) ;100: :4891–6. |
[46] | Mitchell JA , Ali F , Bailey L , et al. Role of nitric oxide and prostacyclin as vasoactive hormones released by the endothelium. Exp Physiol. (2008) ;93: :141–7. |
[47] | Mollace V , Muscoli C , Masini E , et al. Modulation of prostaglandin biosynthesis by nitric oxide and nitric oxide donors. Pharmacological. (2005) ;57: :217–52. |
[48] | Ranjan V , Xiao ZS , Diamond SL . Constitutive Nos Expression in Cultured Endothelial-Cells Is Elevated by Fluid Shear-Stress. Am J Physiol Circ Physiol. (1995) ;38: :H550–H555. |
[49] | Sena CM , Pereira AM , Seiça R . Endothelial dysfunction - A major mediator of diabetic vascular disease. Biochim Biophys Acta - Mol Basis Dis. (2013) ;1832: :2216–31. |
[50] | Ma H , Johnson SL , Liu W , et al. Evaluation of polyphenol anthocyanin-enriched extracts of blackberry, black raspberry, blueberry, cranberry, red raspberry, and strawberry for free radical scavenging, reactive carbonyl species trapping, anti-glycation, anti-β-amyloid aggregation, and mic. Int J Mol Sci; 19. Epub ahead of print 2018. DOI:10.3390/ijms19020461. |
[51] | Zhou L , Yao GD , Lu LW , et al. Neolignans from Red Raspberry (Rubus idaeus L.) Exhibit Enantioselective Neuroprotective Effects against H 2 O 2 -Induced Oxidative Injury in SH-SY5Y Cells. J Agric Food Chem. (2018) ;66: :11390–7. |
[52] | Dudzinska D , Bednarska K , Boncler M , et al. The influence of Rubus idaeus and Rubus caesius leaf extracts on platelet aggregation in whole blood. Cross-talk of platelets and neutrophils. Platelets. (2016) ;27: :433–9. |
[53] | Seeram NP , Momin RA , Nair MG , et al. Cyclooxygenase inhibitory and antioxidant cyanidin glycosides in cherries and berries. Phytomedicine. (2001) ;8: :362–9. |
[54] | Mulabagal V , Lang GA , Dewitt DL , et al. Anthocyanin content, lipid peroxidation and cyclooxygenase enzyme inhibitory activities of sweet and sour Cherries. J Agric Food Chem. (2009) ;57: :1239–46. |
[55] | Seeran NP , Zhang Y , Nair MG . Inhibition of Proliferation of Human Cancer Cells and Cyclooxygenase Enzymes by Anthocyanidins and Catechins. Nutr Cancer. (2009) ;26: :101–6. |
[56] | Xiao X , Shi D , Liu L , et al. Quercetin suppresses cyclooxygenase-2 expression and angiogenesis through inactivation of P300 signaling. PLoS One; 6. Epub ahead of print 2011. DOI:10.1371/journal.pone.0022934 |
[57] | Karlsson S , Nnberg E , Fjaeraa C , et al. Ellagic acid inhibits lipopolysaccharide-induced expression of enzymes involved in the synthesis of prostaglandin E2 in human monocytes. Br J Nutr. (2010) ;103: :1102–9. |
[58] | Yang Y , Andrews MC , Hu Y , et al. Anthocyanin extract from black rice significantly ameliorates platelet hyperactivity and hypertriglyceridemia in dyslipidemic rats induced by high fat diets. J Agric Food Chem. (2011) ;59: :6759–64. |
[59] | Vichai V , Suyarnsesthakorn C , Pittayakhajonwut D , et al. Positive feedback regulation of COX-2 expression by prostaglandin metabolites. Inflamm Res. (2005) ;54: :163–72. |
[60] | Nakano M , Denda N , Matsumoto M , et al. Interaction between cyclooxygenase (COX)-1- and COX-2-products modulates COX-2 expression in the late phase of acute inflammation. Eur J Pharmacol. (2007) ;559: :210–8. |
[61] | Sánchez A , Contreras C , Martínez P , et al. Enhanced cyclooxygenase 2-mediated vasorelaxation in coronary arteries from insulin-resistant obese Zucker rats. Atherosclerosis. (2010) ;213: :392–9. |
[62] | Caughey GE , Cleland LG , Penglis PS , et al. Roles of Cyclooxygenase (COX)-1 and COX-2 in Prostanoid Production by Human Endothelial Cells: Selective Up-Regulation of Prostacyclin Synthesis by COX-2. J Immunol. (2001) ;167: :2831–8. |