DNA methylation balance is involved in anthocyanin accumulation during Vaccinium corymbosum fruit ripening
Abstract
BACKGROUND:
DNA methylation balance is an important regulatory mechanism for mammalian and plant development. The fruit ripening and anthocyanin accumulation of Vaccinium corymbosum are complex developmental processes that involve numerous physiological, biochemical, and structural alterations.
OBJECTIVE:
This study aimed to investigate the correlation of DNA methylation balance, DNA methylation and demethylation-related gene expression models and anthocyanin accumulation during blueberry fruit ripening.
METHODS:
The anthocyanin contents during V. corymbosum ‘O’Neal’ fruit development were evaluated. The V. corymbosum DNA methylation- and anthocyanin accumulation-related genes were isolated, and their relative expression patterns were detected during flower bud enlargement and fruit development. Moreover, the relative expression patterns of anthocyanin biosynthetic genes and the dynamic changes in the DNA methylation of the promoter sequences of key anthocyanin biosynthetic genes were evaluated.
RESULTS:
The results showed that the DNA methylation level of V. corymbosum fruit was consistent with anthocyanin accumulation during ripening, and the expression levels of anthocyanin biosynthetic and DNA methylation-related genes.
CONCLUSIONS:
During V. corymbosum fruit ripening, anthocyanin accumulation is regulated partially by DNA methylation balance of VcCHS and VcANS promoters.
1Introduction
Epigenetics, first introduced by Conrad Waddington in the early 1940s, refers to the phenomenon of heritable changes in gene function or gene expression that do not change DNA sequences during cell proliferation and ontogenetic development [1–5]. Such effects on cellular and physiological phenotypic traits might be involved in dynamic transcriptional or posttranscriptional regulation mechanisms, including DNA methylation, chromatin remodeling, histone posttranslational modifications, noncoding and coding RNAs [1, 3, 4]. In eukaryotes, DNA methylation primarily refers to the methylation at the fifth carbon of cytosine residues (5mC) and mainly occurs in the CpG island of mammalian genomes, and in the CG, CHG, and CHH motifs (H represents A, T, or C) of plant genomes [6–8]. As an important epigenetic modification, the precise patterns of genomic DNA methylation are essential and conserved for gene regulation, genome defense and maintaining genomic stability [6–8]. In general, most DNA methylated loci are located in the heterochromatin regions, transposable elements, and promoter sequences [3, 32, 44]. Recently, DNA methylation has been reported to be involved in regulating transposon silencing, gene expression, chromosome interactions, plant development, plant responses to biotic and abiotic stimuli, fruit ripening, root nodulation, and other developmental processes [3, 6–10, 37–43].
The establishment and maintenance of DNA methylation in higher plants is generally catalyzed by DNA methyltransferases that are regulated by complex mechanisms. Four families of DNA methyltransferases, DNA methyltransferases (METs), domains rearranged methyltransferases (DRMs), chromomethylases (CMTs), and histone lysine methyltransferases (HKMTs) regulate the methylation of cytosine residues in symmetrical or asymmetrical contexts. Together with DNA demethylases (DMEs or DMLs), histone-modifying enzymes, chromatin remodeling factors and RNA interference machinery, DNA methylation is regulated precisely, which maintains the dynamic balance of genomic methylation levels, and the responses to developmental signals and environmental stimuli [3, 5–8].
Blueberry (Vaccinium spp.) belongs to the Ericaceae family of deciduous or evergreen shrub plants, and contains numerous important cultivated species, such as V. corymbosum (highbush blueberry), V. ashei (rabbiteye blueberry), V. angustifolium (lowbush blueberry) and hybrid varieties from V. corymbosum×V. angustifolium (half-high blueberry) [11]. Currently, blueberry has become the second abundant berry in the world after strawberry, although its commercial cultivation history is no more than 100 years [12]. Because it is a rich source of bioactive compounds in fruit, such as anthocyanins, flavonols, and proanthocyanidins, blueberry has gathered considerable interests. These bioactive molecules not only supply nutrients, but also play important roles in scavenging free radicals and improving immune activities, such as anti-inflammatory, antimutagenic, antimicrobial, anticancer and antiobesity activities, etc. [13–15].
Anthocyanin content is an important trait for blueberry fruit quality, and affects its nutritional and commercial values. The biosynthesis pathway of anthocyanins has been studied extensively, and several enzymes in the biosynthetic pathway, such as chalcone synthase (CHS), flavanone 3′-hydroxylase (F3′H), dihydroflavonol 4-reductase (DFR), anthocyanidin synthase (ANS), and UDP-glucoside: flavonoid glucosyltransferase (UFGT), have been isolated and identified [16–19]. However, the correlation between DNA methylation changes and anthocyanin accumulation in blueberry fruit was not extensively studied before. In this study, V. corymbosum DNA methylation-related and anthocyanin accumulation-related genes were isolated, and their relative expression patterns were detected during flower bud enlargement and fruit development. In addition, the relative expression patterns of key anthocyanin biosynthetic genes and the dynamic changes in DNA methylation in the promoter sequences of VcCHS and VcANS genes were analyzed.
2Materials and methods
2.1Plant materials
6-year-old southern highbush blueberry (V. corymbosum cv. ‘O’Neal’) planted in the orchard at Zhejiang Normal University (119°65′ E and 29°08′ N, Jinhua, Zhejiang Province, China) were used in this experiment. According to Yang et al. [20], 30–100 flower buds or fruits at specific developmental stages (Fig. 1a) were randomly tagged, collected and processed from multiple plants. Excluding the tissues used for physiological analysis, all processed tissues were frozen immediately in liquid nitrogen and stored at –80 °C for further use.
Fig. 1
Developmental stages and anthocyanin contents of V. corymbosum (cv. O’Neal) flowers and fruits. a. The flower and berry developmental stages used in this study. b. The total anthocyanin content in the ovaries and fruits throughout development.
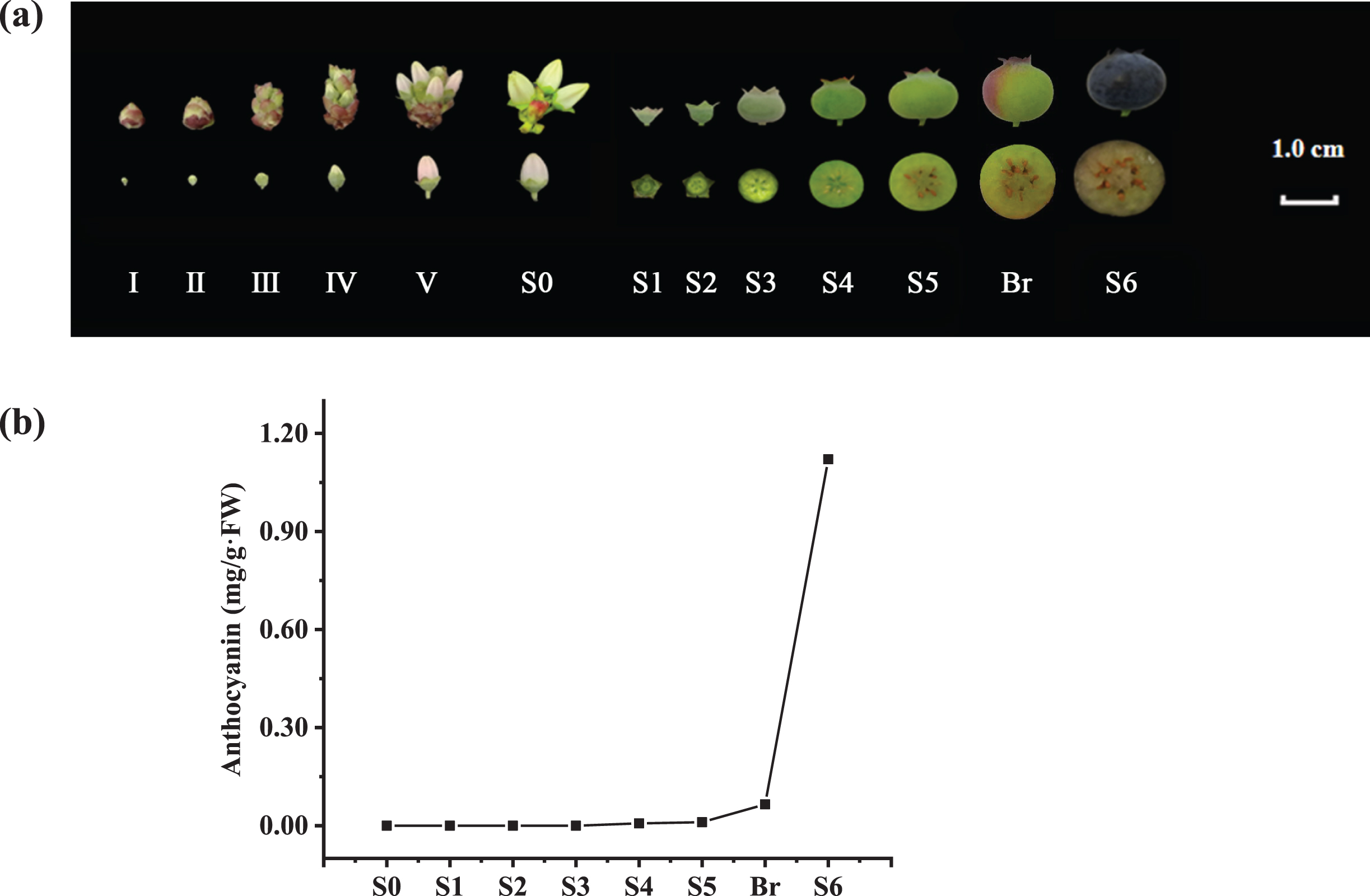
2.2Total anthocyanin content analysis
Total anthocyanin contents were estimated using the pH differential method [17, 18]. Briefly, flower buds and fruits at different developmental stages were extracted with 60% ethanol solution (pH 3.0, including 0.1% (v/v) HCl) at 52 °C for 145 min. Each extract was diluted with 0.05 M potassium chloride buffer (pH 1.0) and 0.1 M sodium acetate buffer (pH 4.5) to attain the same dilution. After stabilization at room temperature for 20 min, the absorbance of each diluted solution was measured at 524 nm and 700 nm, with a distilled solution without extracts used as the blank. The total anthocyanin content, expressed as the amount (mg) of cyanidin-3-glucoside/100 g.FW was calculated using the following formula: C = (A × Mw × V × n)/(ɛ × b × V0) . Of these parameters, A was the difference of [(A524-A700)(pH 1.0)-(A524-A700)(pH 4.5)], Mw was the molecular weight of cyanidin-3-glucoside (449.2 g/mol), V was the total extract volume of sample, n was the fold dilution, ɛ was the molar absorptivity coefficient of cyanidin-3-glucoside [29,600 L/(mol.cm)], b was path length (1 cm), and V0 was the sample volume used for spectrophotometry.
2.3Genomic DNA, total RNA extraction and cDNA synthesis
Genomic DNA from fruits during anthocyanin accumulation (stages S5, Breaker, S6) was extracted using a modified cetyltrimethylammonium bromide (CTAB) method [21], and residual RNA was removed using RNase A (TaKaRa Biotechnology Co., Ltd., Dalian, China). Total RNA extraction, RNA quality analysis and 1st strand cDNA synthesis were performed according to Yang et al. [22].
2.4Isolation and sequence analysis of VcMET1, VcDRM2, VcCMT3 and VcDME1 cDNAs and the promoters of VcCHS and VcANS
The full-length coding sequences (CDSs) of the possible V. corymbosum MET1, DRM2, CMT3, and DME1 genes and the promoter sequences of VcCHS and VcANS genes were searched and downloaded from Genome Database of Vaccinium (https://www.vaccinium.org/). Specific primers were subsequently designed on the basis of the multiple comparative analysis of nucleotide sequences with other plant homologous genes (Table S1). The isolation and sequence analysis of full-length cDNAs and promoter sequences were performed according to Yang et al. [22].
2.5Relative expression patterns of V. corymbosum DNA methylation-, DNA demethylation- and anthocyanin biosynthesis- related genes during flower bud enlargement and fruit development
Based on isolated or reported cDNA sequences, specific primers for real-time quantitative PCR (qPCR) analysis (Table S1) were designed by online Primer3 Input software (version 0.4.0, http://bioinfo.ut.ee/primer3–0.4.0/), and VcGAPDH was used to normalize the amount of cDNAs among samples [23]. The reagents, reaction system, procedure and data analysis were performed according to Bustin et al. [24] and Yang et al. [22].
2.6Bisulfite conversion and sequencing
Extracted genomic DNA was used for bisulfite conversion according to the manual of EZ DNA Methylation Gold Kit (Zymo 5005, Zymo Research Corp., California, USA). Modified DNA was amplified by PCR using Q5 High-Fidelity DNA Polymerase (New England Biolabs Inc., Beijing, China), and the amplification primers were listed in Table S1. The specific amplicons were inserted into pMD19-T vector (TaKaRa Biotechnology Co., Ltd., Dalian, China), and transformed into Escherichia coli DH5α. 10 to 15 positive clones (obtained from 2 independent replicates) were selected for sequencing and sequence analysis.
2.7Statistical analysis
Each determination was conducted in at least three biological repetitions, unless otherwise indicated. The data were represented as the mean value and standard error (mean±SE, n≥3). Statistical significance was evaluated via one-way analysis of variance (ANOVA) followed by Tukey’s post hoc test using SPSS16.0 software.
3Results
3.1Anthocyanin primarily accumulated before and after the Breaker stage in V. corymbosum fruit
Similar to previous studies [15–18], anthocyanin mainly accumulated in the pericarp of ripe blueberry fruits. In this study, the anthocyanin level was too low to be detected during V. corymbosum flower bud enlargement and early fruit development (stages S0–S4). Then, a small amount of anthocyanin (approximately 0.011 mg/g.FW) began to accumulate in the fruit at stage S5, although its pericarp was still light green. Once approximately 1/4 of the fruit exhibited pale red or light purple (stage Breaker), the anthocyanin content was approximately 0.036 mg/g.FW. Subsequently, fruit became dark blue or purple rapidly at stage S6, and the total anthocyanin content increased approximately 24 fold, reaching up to 0.859 mg/g.FW (Fig. 1b).
3.2Structures and characteristics of V. corymbosum DNA methylation- and DNA demethylation-related genes
3 DNA methylation-related cDNAs and 1 DNA demethylation-related cDNA were isolated from V. corymbosum fruit, and were named according to their homologous genes with the highest identity (Table 1). As shown in Table 1, the deduced amino acid sequences of VcMET1, VcDRM2 and VcDME1 had the highest identity (83%, 79% and 69%) with Actinidia chinensis PSS02925.1, PSR87569.1, and PSR92965.1, respectively, while VcCMT3 had the highest identity (69%) with Camellia sinensis XP_028051823.1.
Table 1
Bioinformatic analysis of isolated V. corymbosum DNA methylation and demethylation related sequences
Target gene | GenBank Accession number | Protein length (aa) | Molecular weight (KDa) | Homologous proteins with highest identity |
VcMET1 | KY964443.2 | 1,548 | 174.76 | Actiniclia chinensis PSS02925.1 83% |
VcDRM2 | MF074241.1 | 606 | 68.29 | Actiniclia chinensis PSR87569.1 79% |
VcCMT3 | MF074242.2 | 866 | 97.04 | Camellia sinensis XP_028051823.1 69% |
VcDME1 | MF074243.3 | 1,873 | 460.33 | Actiniclia chinensis PSR92965.1 69% |
Compared with the sequence of VcDME1, the deduced amino acid sequences of VcMET1, VcDRM2 and VcCMT3 were relatively conserved, and consisted of a common conserved site-specific DNA-cytosine methylase (Dcm) domain (Fig. 2). In addition, VcMET1 contained two cytosine-specific DNA methyltransferase replication foci (DNMT1-RFD) domains, and two bromo adjacent homology (BAH) domains. VcDRM2 contained a ubiquitin-associated (UBA) domain, and VcCMT3 contained a BAH domain (Fig. S1). The sequence of VcDME1 was relatively variable, containing only a conserved helix–hairpin–helix domain, a single permuted zf-CXXC (Perm-CXXC) domain and a RRM-DME domain at the 3’ end (Fig. S1).
Fig. 2
MEME generated conserved structures and sequences from isolated V. corymbosum DNA methylation-related proteins.
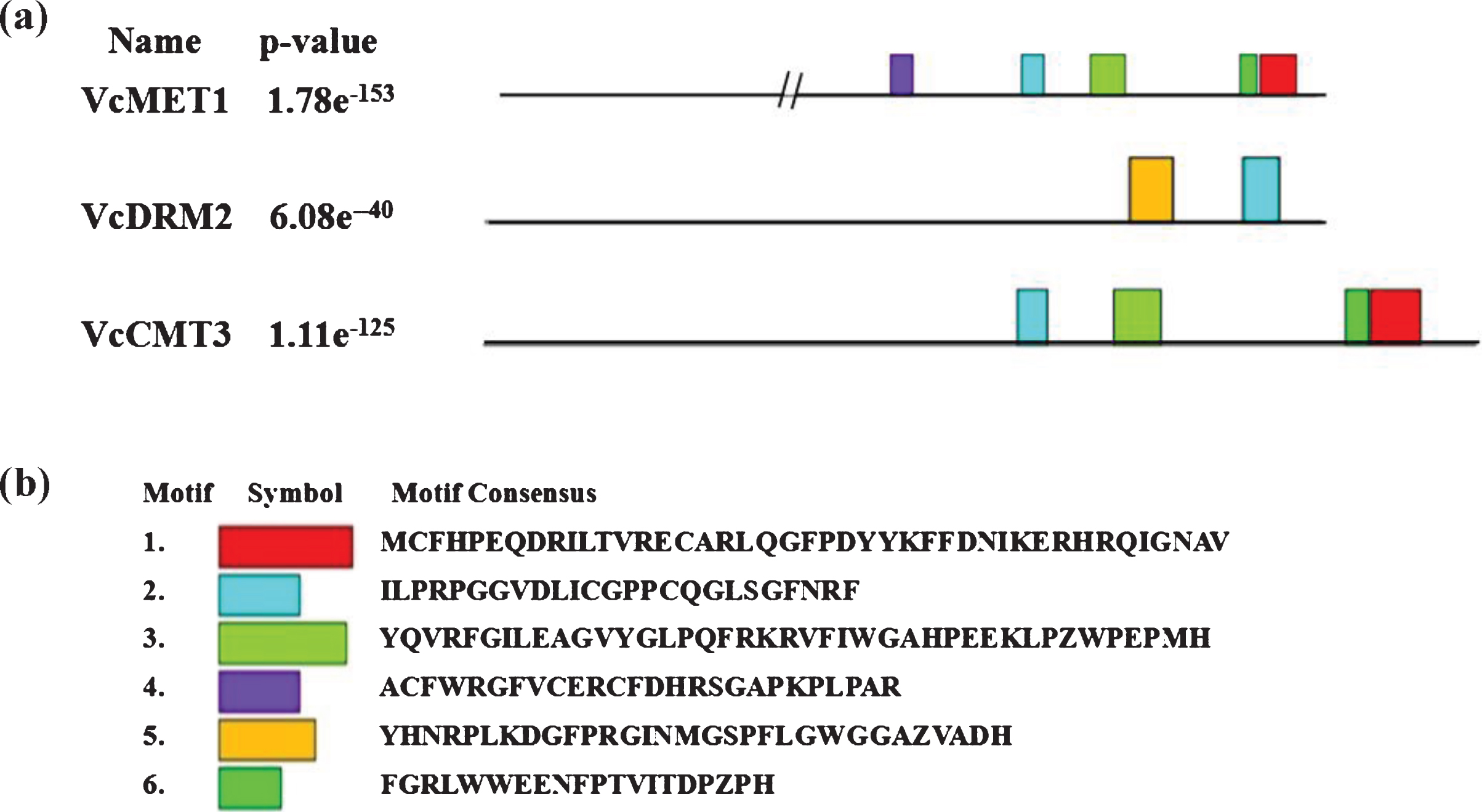
3.3Isolation and characterization of VcCHS and VcANS promoter sequences
CHS is the first enzyme that catalyzes malonyl-CoA and P-coumaroyl-CoA to form anthocyanins and other flavonoids in plants, and ANS is a key enzyme downstream of the anthocyanin biosynthetic pathway [16, 25, 37]. In this study, the isolated VcCHS and VcANS promoters were 1,330 bp and 1,264 bp in length, respectively. The putative regulatory elements in the VcCHS and VcANS promoters (Table 2) were analyzed by the online database PlantCARE (http://bioinformatics.psb.ugent.be/webtools/plantcare/html/). In addition to basal regulatory elements for eukaryotic gene transcription, such as the TATA-box, CAAT-box and other elements, VcCHS promoter contained multiple consensus sequences for Myb protein binding sites, anaerobic induction (ARE), abscisic acid (ABA) responsiveness, light responsiveness, methyl jasmonate (MeJA) responsiveness, auxin responsiveness and core G-box sites. VcANS promoter contained one or more cis-acting regulatory elements for ARE, light responsiveness, ABA responsiveness, MeJA responsiveness, salicylic acid (SA) responsiveness, auxin responsiveness, and Myb protein-binding sites (Table 2). These regulatory elements located in the promoters of VcCHS and VcANS suggested that anthocyanin biosynthesis might be differentially modulated by transcription factors (e.g. Myb), and environmental and physiological factors, such as light, temperature, sugars, and hormones [26, 27].
Table 2
Predicted cis-acting regulatory elements in VcCHS and VcANS promoter region
cis- elements name | cis-element sequence | cis-element position | Function |
VcCHS promoter | |||
Myb | CAACTG | +164, –167, +522, +642, | Myb binding site |
–1,128, +1,149 | |||
ARE | AAACCA | –211, –481, +847, –875, | cis-acting regulatory element essential for the anaerobic induction |
–1,231 | |||
G-box | TACGTG | +255 | light responsiveness |
ABRE | ACGTG | +226, –666 | ABA responsiveness |
TGACG-motif | TGACG | +281 | MeJA-responsiveness |
TGA-element | AACGAC | +772 | auxin responsiveness |
TCT-motif | TCTTAC | +951 | light responsiveness |
TATA-box | TATA | core promoter element around -30 of transcription start | |
CAAT-box | CAAAT, CAAT | common cis-acting element in promoter and enhancer regions | |
VcANS promoter | |||
Myb | CAACCA | +239 | Myb binding site |
ABRE | CACGTG | +281 | ABA responsiveness |
G-box | CACGTG | +281 | light responsiveness |
CGTCA-motif | CGTCA | +371 | MeJA-responsiveness |
Box 4 | ATTAAT | +411, +415 | light responsiveness |
TCA-element | CCATCTTTTT | +527 | SA responsiveness |
TGA-element | AACGAC | –620 | auxin responsiveness |
ARE | AAACCA | –654, +689, –810, +853 | cis-acting regulatory element essential for the anaerobic induction |
TCT-motif | TCTTAC | –831 | light responsiveness |
GA-motif | ATAGATAA | –993 | light responsiveness |
TATA-box | TATA | core promoter element around –30 of transcription start | |
CAAT-box | CAAAT, CAAT | common cis-acting element in promoter and enhancer regions |
3.4The developmental expression patterns of V. corymbosum DNA methylation- and DNA demethylation-related genes
The expression patterns of isolated V. corymbosum DNA methylation- and DNA demethylation-related genes were evaluated throughout flower bud enlargement and fruit development in this study. In addition to the reference gene VcGAPDH, VcMET1 transcripts at anthesis (stage S0) were chosen to normalize other V. corymbosum DNA methylation and demethylation gene expression levels. Interestingly, the highest transcripts of VcMET1, VcDRM2 and VcCMT3 genes were detected at the early stage of blueberry flower bud enlargement (stage I), but then these transcript levels decreased dramatically from stage II and were maintained at relatively lower levels. Specifically, VcMET1 and VcCMT3 genes exhibited similar expression patterns, with a second expression peak at stage S3, and then, the expression levels decreased to an undetectable level from stage S5 until maturity. VcDRM2 transcripts also showed a downward trend throughout flower bud enlargement and fruit development, and the expression abundance of VcDRM2 was much lower than that of VcMET1 and VcCMT3. For VcDME1 gene, its expression abundance decreased gradually from the early stage of flower bud enlargement and then exhibited a fluctuating increasing and decreasing trend during anthesis and late fruit development (Fig. 3).
Fig. 3
The developmental expression patterns of V. corymbosum DNA methylation- and DNA demethylation-related genes throughout flower bud enlargement and fruit development.
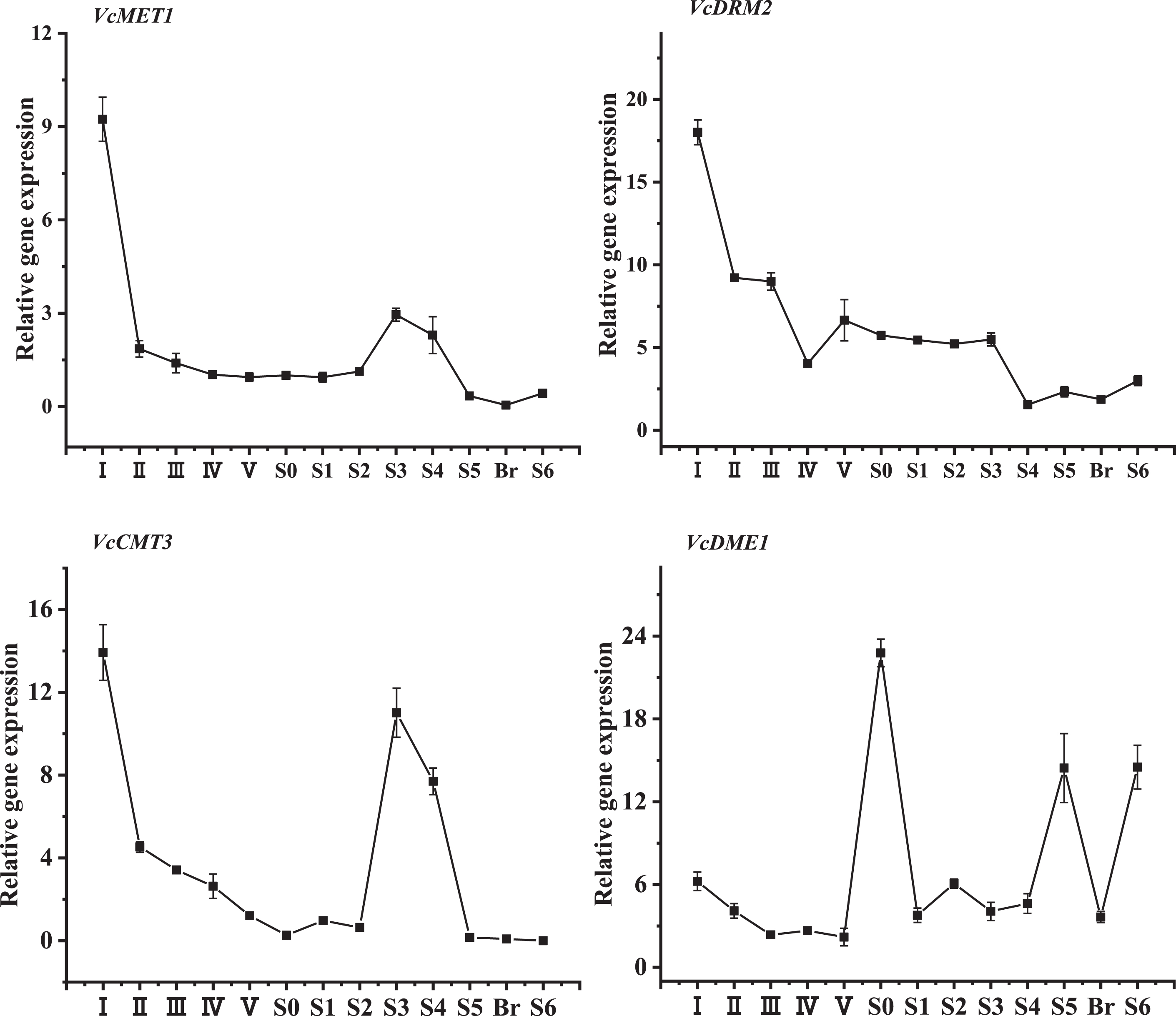
3.5The developmental expression patterns of V. corymbosum anthocyanin biosynthesis-related genes
Because anthocyanin accumulation primarily occurred in mature fruit, the expression patterns of selective anthocyanin biosynthetic genes were evaluated during V. corymbosum ‘O’Neal’ fruit development. Besides the reference gene VcGAPDH, Vc ANS transcripts at anthesis (stage S0) were chosen to normalize other V. corymbosum anthocyanin biosynthesis-related gene expression levels. The expression patterns of VcCHS, VcF3’H, and VcANS genes were somewhat similar, and exhibited a biphasic profile, with two peaks during early and late fruit development (Fig. 4). The mRNA levels of VcCHS and VcANS genes were higher than those of VcF3’H gene, and the highest expression peak was present at the early fruit development and the beginning of anthocyanin accumulation. Interestingly, the transcript levels of VcCHS and VcANS genes decreased sharply at the breaker stage, and then increased again till mature. VcDFR gene also exhibited an expression peak during early fruit development and then were maintained at a relatively lower level until anthocyanin accumulated in fruit, while the expression abundance of Vc UFGT gene maintained a relatvie low level at the early stages of fruit development, but gradually increased following fruit anthocyanin accumulation. In addition, the expression levels of VcF3’H, VcDFR and VcANS were relatively higher during early fruit development (stage S1 or stage S2), while the highest expression levels of VcCHS and VcUFGT genes occurred during the breaker stage or mature stage.
Fig. 4
The developmental expression patterns of V. corymbosum anthocyanin biosynthesis-related genes throughout fruit development.
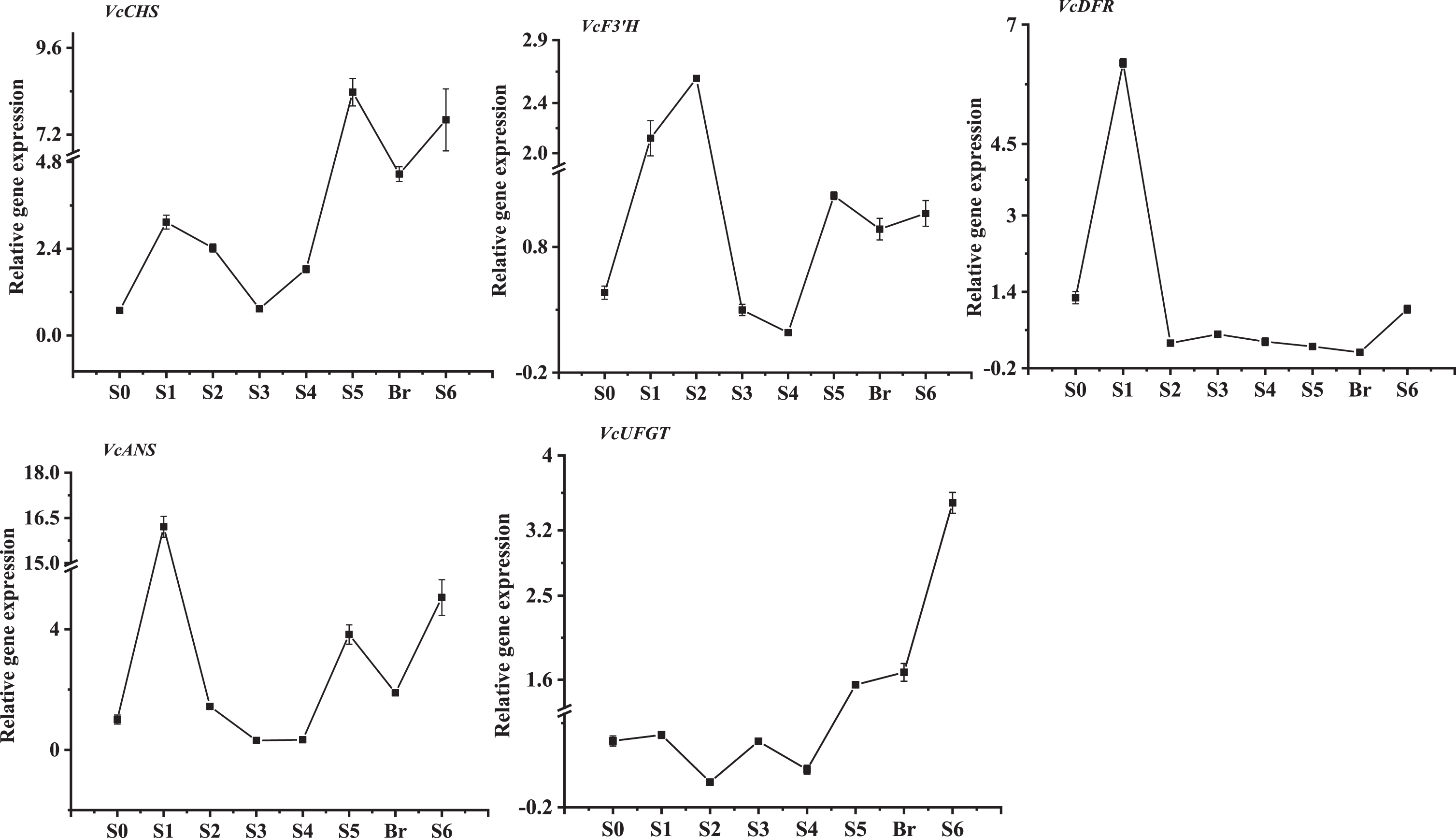
3.6DNA methylation variation in the VcCHS and VcANS promoters during anthocyanin accumulation in V. corymbosum fruit
Purified genomic DNA extracted from V. corymbosum fruits during anthocyanin accumulation stages (stages S5, Breaker and S6) was converted by bisulfite, and then the bisulfite-converted genomic DNA was used as a template to isolate the VcCHS and VcANS promoters. After the removal of partially unreliable bases, 1,309-bp VcCHS and 1,206-bp VcANS promoter sequences were selected for the evaluation of DNA methylation changes.
During the anthocyanin accumulation process, the total variable 5mC of blueberry fruits at stages S5 and S6 were weakly lower than those at the breaker stage in the VcCHS bisulfite sequenced promoter loci (Fig. 5a). 5mC at the CHH motifs (54.05%) was dominant followed by CG motifs (35.14%) and CHG motifs (10.81%) (Fig. 5b). In addition, approximately 50 variable methylated/demethylated cytosine sites (3.82%) were detected in all three stages, and 7 sites were located in the important regulatory elements, especially in TATA box, CTCC site, Myb-binding site and ARE site (Fig. S3).
Fig. 5
Specific DNA methylation status in the VcCHS and VcANS promoters during anthocyanin accumulation. a. Total cytosine methylation values in the VcCHS and VcANS promoters. b. Cytosine methylation values in percentage of CG, CHG and CHH motifs in the VcCHS and VcANS promoters.
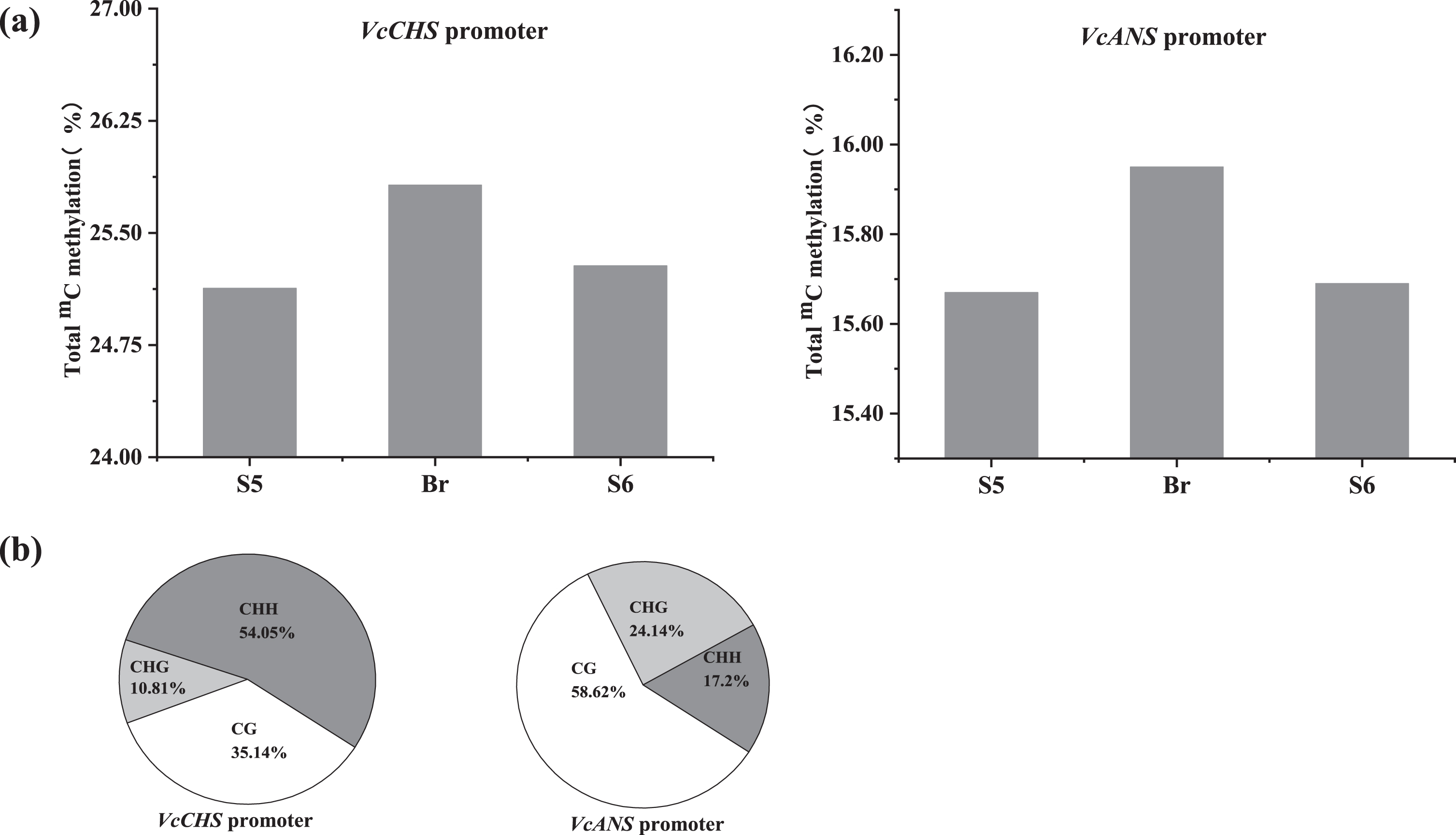
In the VcANS bisulfite sequenced promoter loci, the total variable 5mC in the VcANS promoter at stages S5, breaker and S6 was 25.13%, 25.82% and 25.28%, respectively (Fig. 5a). And the ratio of 5mC at the CHH, CG and CHG motifs was 58.62%, 24.14% and 17.24%, respectively. Similar to the VcCHS promoter, a total of 43 mC variation sites (3.41%) were detected in the VcANS promoter during anthocyanin accumulation, and 4 sites were located in the core regulatory elements, such as CAAT box (enhancer region), box S, and box 4, responsible for light and anaerobic induction (Fig. S3).
4Discussion
4.1V. corymbosum fruit color was positively correlated with the anthocyanin content and anthocyanin biosynthesis-related gene expression
Fruit skin color is an important quality indicator determining its acceptability among blueberry consumer [28]. V. corymbosum fruit is dark blue or purple, which is mainly attributed to the accumulation of abundant anthocyanins in the exocarp before maturation. In fact, immature V. corymbosum fruit is green, at which stages the total anthocyanins content is not detectable. Once V. corymbosum fruit entered veraison and postveraison, the total anthocyanin content could be detected and increased dramatically.
The most selective anthocyanin biosynthetic genes exhibited a biphasic profile, with two peaks during early and late fruit development, while the relative abundance of anthocyanin-specific VcUFGT transcripts gradually increased following fruit anthocyanin accumulation. The latter expression peak of VcCHS, VcF3’H, VcDFR and VcANS genes was unquestionably followed by anthocyanin accumulation in the fruit, and the first expression peak might be involved in the proanthocyanidin biosynthesis, because anthocyanin and proanthocyanidin biosynthesis shared the same upstream biosynthetic enzymes [16, 19, 29]. In our previous studies, the level of total proanthocyanidins peaked at the early stages of fruit development and subsequently declined as the fruit matured (data not shown). Similar results have been found in north highbush blueberry and blackberry fruit [16, 30], and the switch in flavonoid biosynthesis from proanthocyanidins to anthocyanins might be involved in protecting fruit and promoting seed dispersal [16].
4.2Dynamic changes in DNA methylation occurred during V. corymbosum fruit development and fruit ripening
Epigenetic modifications, especially DNA methylation, play very important roles in plant growth and development. The dynamical changes in DNA methylation, is a ‘timing switch’ for specific developmental stages in plants [3, 5, 9]. In sweet orange, DNA methylation gradually increased with a gradual decrease in the expression of DNA demethylase genes during ripening [31]. However, DNA methylation gradually decreased following the upregulation of DNA demethylase expression, and ripening-induced demethylation was abolished in the promoters of several fruit-ripening genes [32]. In this study, the expression of VcMET1, VcDRM2 and VcCMT3 genes was high at the early stages of flower bud enlargement (stages I and II), at the end of the first rapid fruit growth stage (stage S3) and at the beginning of the second rapid fruit growth stage (stage S4). Following the process of fruit ripening and nutrient accumulation, the expression of these DNA methylation maintenance related genes gradually decreased, and even became undetectable. In contrast, VcDME was mainly expressed at anthesis (stage S0) and exhibited a fluctuating increasing and decreasing trend during anthocyanin accumulation and fruit ripening (Fig. 3). These observations suggested that DNA methylation and demethylation worked together to regulate blueberry fruit development and ripening. Recently, the m6A methylation in mRNAs was declined during tomato fruit ripening [33], indicating that not only DNA methylation and demethylation but also other epigenetic mechanisms were involved in fruit growth and development.
4.3DNA methylation changed dynamically in the promoters of VcCHS and VcANS during anthocyanin biosynthesis
DNA methylation is mainly enriched in the heterochromatin regions, transposable elements, and promoter sequences, etc.[3, 32, 44], and DNA methylation level in the promoter regions of special genes could be used for the ‘fine tuning’ of gene expression [5, 9, 32]. In this study, the total variable 5mC levels of VcCHS and VcANS promoters were approximately 25% and 16%, respectively, and weakly increased and then decreased following anthocyanin accumulation and fruit ripening (Fig. 5). This trend was similar to that detected in climacteric fruit, such as tomato, and nonclimacteric fruit such as orange and strawberry [31, 32, 34]. Furthermore, some variable 5mC sites in the VcCHS and VcANS promoters were located in the cis-regulatory elements, such as the CAAT box (enhancer region), box S and box 4, responsible for light and anaerobic induction and gene transcription. In addition to these structural genes, transcription factors, such as Myb and bHLH, were also associated with anthocyanin accumulation regulated by DNA methylation [26, 35, 36, 45].
5Conclusions
The dynamic balance of DNA methylation is an important regulatory mechanism for animal and plant development. Fruit ripening, and anthocyanin accumulation in fruit are complex developmental processes that involve in numerous physiological, biochemical, and structural alterations. In this study, DNA methylation and demethylation were involved in anthocyanin accumulation in V. corymbosum fruit by regulating the DNA methylation levels of the promoters of the anthocyanin biosynthetic genes VcCHS and VcANS. Our results may provide a relatively more complete understanding of the regulation of anthocyanin synthesis and fruit ripening.
Conflict of interest
The authors have no conflict of interest to report.
Author Contributions
Study design, acquisition of data, analysis, and interpretation of data: Lei Yu, Ya Zhou, Yihui Zhang, Wei Liu, Yongqiang Li, Mei Lu, Chaoyang Fan, Nan Shao, Li Yang and Weidong Guo.
Manuscript writing and revising: Lei Yu, Ya Zhou and Li Yang.
Acknowledgments and Funding
This work was supported by the major program for science and technology of Zhejiang province (grant numbers 2018C02007 and 2016C02052-9), and open research subject of Zhejiang engineering and technology research center of specialty plant germplasm innovation and utilization of southern Zhejiang province (grant numbers ZX201906 and ZX201907).
Supplementary material
[1] The supplementary material is available in the electronic version of this article: https://dx.doi.org/10.3233/JBR-200553.
References
[1] | Wassenegger M , Heimes S , Riedel L , Sänger HL . RNA-directed de novo methylation of genomic sequences in plants. Cell. (1994) ;76: (3):567–76. |
[2] | Rodrigues JA , Zilberman D . Evolution and function of genomic imprinting in plants. Genes Dev. (2015) ;29: (24):2517–31. |
[3] | Giovannoni J , Nguyen C , Ampofo B , Zhong SL , Fei ZJ . The epigenome and transcriptional dynamics of fruit ripening. Annu Rev Plant Biol. (2017) ;68: :61–84. |
[4] | Duan CG , Zhu JK , Cao XF . Retrospective and perspective of plant epigenetics in China. J Genet Genomics. (2018) ;45: (11):621–38. |
[5] | Liu RE , Lang ZB . The mechanism and function of active DNA demethylation in plants. J Integr Plant Biol. (2020) ;62: (1):148–59. |
[6] | Pikaard CS , Scheid OM . Epigenetic regulation in plants. Cold Spring Harb Perspect Biol. (2016) ;6: (12):a019315. |
[7] | Varriale A . DNA methylation in plants and its implications in development, hybrid vigour, and evolution. In: Rajewsky N, Jurga S, Barciszewski J (ed). Plant Epigenetics, RNA Technologies. Springer, 2017;263-80. |
[8] | Zhang HM , Lang ZB , Zhu JK . Dynamics and function of DNA methylation in plants. Nat Rev Mol Cell Bio. (2018) ;19: (8):489–506. |
[9] | Gallusci P , Hodgman C , Teyssier E , Seymour GB . DNA methylation and chromatin regulation during fleshy fruit development and ripening. Front Plant Sci. (2016) ;7: :807. |
[10] | Yeunga AWK , Tzvetkovb NT , Zengind G , Wang DD , Xu SW , Mitrovici G , Brncic M , Dall’Acquak S , Pirgozliev V , Kijjoam A , Georgievn MI , Atanasov AG . The berries on the top. J Berry Res. (2019) ;9: :125–39. |
[11] | Retamales JB , Hancock JF (ed) Blueberries (2nd Edition). In Crop Production Science in Horticulture. CABI Publishing, Wallingford. (2018) . |
[12] | Ballington JR , Collection, utilization, and preservation of genetic resources in Vaccinium. HortScience. (2001) ;36: :213–20. |
[13] | Karppinen K , Zoratti L , Nguyenquynh N , Häggman H , Jaakola L . On the developmental and environmental regulation of secondary metabolism in Vaccinium spp. berries. Front Plant Sci. (2016) ;7: :655. |
[14] | Miller K , Feucht W , Schmid M . Bioactive compounds of strawberry and blueberry and their potential health effects based on human intervention studies: a brief overview. Nutrients. (2019) ;11: (7):1510. |
[15] | Spinardi A , Cola G , Gardana CS , Mignani I . Variation of anthocyanin content and profile throughout fruit development and ripening of highbush blueberry cultivars grown at two different altitudes. Front Plant Sci. (2019) ;10: :1045. |
[16] | Zifkin M , Jin A , Ozga JA , Zaharia LI , Schernthaner JP , Gesell A , Abrams SR , Kennedy JA , Constabel CP . Gene expression and metabolite profiling of developing highbush blueberry fruit indicates transcriptional regulation of flavonoid metabolism and activation of abscisic acid metabolism. Plant Physiol. (2012) ;158: (1):200–24. |
[17] | Li LL , Zhang HH , Liu ZS , Cui XY , Zhang T , Li YF , Zhang LY . Comparative transcriptome sequencing and de novo analysis of Vaccinium corymbosum during fruit and color development. BMC Plant Biol. (2016) ;16: (1):223. |
[18] | Lin Y , Wang YH , Li B , Tan H , Li DN , Li L , Liu X , Han JC , Meng XJ . Comparative transcriptome analysis of genes involved in anthocyanin synthesis in blueberry. Plant Physiol Biochem. (2018) ;127: :561–72. |
[19] | Li XB , Jin L , Pan XH , Yang L , Guo WD . Proteins expression and metabolite profile insight into phenolic biosynthesis during highbush blueberry fruit maturation. Food Chem. (2019) ;290: :216–28. |
[20] | Yang L , Cai KL , Huang HY , Zhang YH , Zong Y , Wang SJ , Shi JL , Li XP , Liao FL , Lu M , Guo WD . Comparative analysis of anatomy, gene expression of Vaccinium corymbosum cyclins and cyclin dependent kinases during the flower bud and fruit ontogeny. Sci Horti. (2019) ;251: :252–9. |
[21] | Cheng YJ , Guo WW , Yi HL , Pang XM , Deng XX . An efficient protocol for genomic DNA extraction from Citrus species. Plant Mol Biol Rep. (2003) ;21: :177a–177g. |
[22] | Yang L , Chen MM , Cai KL , Zhang LJ , Zhu YF , Ye Q , Lu M , Liao FL , Chen WR , Guo WD . VcFAS, VcSUN and VcOVATE orchestrated the fruit morphogenesis in southern highbush blueberry during the pre-anthesis and fruit development. Sci Horti. (2018) ;240: :109–15. |
[23] | Die JV , Rowland LJ . Superior cross-species reference genes: a blueberry case study. Plos One. (2013) ;8: (9):e73354. |
[24] | Bustin SA , Benes V , Garson JA , Hellemans J , Huggett J , Kubista M , Mueller R , Nolan T , Pfaffl MW , Shipley GL , Vandesompele J , Wittwer CT . The MIQE guidelines: minimum information for publication of quantitative real-time PCR experiments. Clin Chem. (2009) ;55: (4):611–22. |
[25] | Dutt M , Dhekney SA , Soriano L , Kandel R , Grosser JW . Temporal and spatial control of gene expression in horticultural crops. Hortic Res. (2014) ;1: :14047. |
[26] | Gu KD , Wang CK , Hu DG , Hao YJ . How do anthocyanins paint our horticultural products? Sci Hortic. (2019) ;249: :257–62. |
[27] | Liu HN , Su J , Zhu YF , Yao GF , Allan AC , Ampomah-Dwamena C , Shu Q , Wang KL , Zhang SL , Wu J . The involvement of PybZIPa in light-induced anthocyanin accumulation via the activation of PyUFGT through binding to tandem G-boxes in its promoter. Hortic Res. (2019) ;6: :134. |
[28] | Duarte C , Guerra M , Daniel P , Camelo AL , Yommi A . Quality changes of highbush blueberries fruit stored in CA with different CO2 levels. J Food Sci. (2009) ;74: (4):S154–S159. |
[29] | Constabel CP . Molecular controls of proanthocyanidin synthesis and structure: prospects for genetic engineering in crop plants. J Agric Food Chem. (2018) ;66: (38):9882–8. |
[30] | Chen Q , Yu HW , Tang HR , Wang XR . Identification and expression analysis of genes involved in anthocyanin and proanthocyanidin biosynthesis in the fruit of blackberry. Sci Hortic. (2012) ;141: :61–8. |
[31] | Huang H , Liu R , Niu Q , Tang K , Zhang B , Zhang H , Chen K , Zhu JK , Lang Z . Global increase in DNA methylation during orange fruit development and ripening. Proc Natl Acad Sci USA. (2019) ;116: (4):1430–6. |
[32] | Lang ZB , Wang YH , Tang K , Tang D , Datsenka T , Cheng JF , Zhang YJ , Handa AK , Zhu JK . Critical roles of DNA demethylation in the activation of ripening-induced genes and inhibition of ripening-repressed genes in tomato fruit. Proc Natl Acad Sci USA. (2017) ;114: (22):E4511–E4519. |
[33] | Zhou LL , Tian SP , Qin GZ . RNA methylomes reveal the m6A-mediated regulation of DNA demethylase gene SlDML2 in tomato fruit ripening. Genome Biol. (2019) ;20: :156. |
[34] | Cheng JF , Niu QF , Zhang B , Chen KS , Yang RH , Zhu JK , Zhang YJ , Lang ZB . Downregulation of RdDM during strawberry fruit ripening. Genome Biol. (2018) ;19: :212. |
[35] | Li WF , Ning GX , Mao J , Guo ZG , Zhou Q , Chen BH . Whole-genome DNA methylation patterns and complex associations with gene expression associated with anthocyanin biosynthesis in apple fruit skin. Planta. (2019) ;250: (6):1833–47. |
[36] | Jiang SH , Wang N , Chen M , Zhang R , Sun QG , Xu HF , Zhang ZY , Wang YC , Sui XQ , Wang SF , Fang HC , Zuo WF , Su MY , Zhang J , Fei ZJ , Chen XS . Methylation of MdMYB1 locus mediated by RdDM pathway regulates anthocyanin biosynthesis in apple. Plant Biotechnol. J. (2020) ;18: :1736–48. |
[37] | Zhu YC , Zhang B , Allan AC , Kui LW , Zhao Y , Wang K , Chen KS , Xu CJ . DNA demethylation is involved in the regulation of temperature-dependent anthocyanin accumulation in peach. Plant J. (2020) ;102: (5):965–76. |
[38] | Jia HR , Zhang ZB , Zhang SH , Fu WH , Su LY , Fang JG , Jia HF . Effect of the methylation level on the grape fruit development process. J Agric Food Chem. (2020) ;68: (7):2099–115. |
[39] | Lü P , Yu S , Zhu N , Chen YR , Zhou B , Pan Y , Zhong S . Genome encode analyses reveal the basis of convergent evolution of fleshy fruit ripening. Nature Plants. (2018) ;4: (10):784–91. |
[40] | Xiao K , Chen J , He QM , Wang YX , Shen HL , Sun L . DNA methylation involved in pepper (Capsicum annuum L.) fruit ripening regulation and interacts with phytohormones. J Exp Bot. (2020) ;71: (6):1928–42. |
[41] | Yang Y , Tang K , Datsenka TU , Liu WS , Lv SH , Lang ZB , Wang XG , Gao JH , Wang W , Nie WF , Chu ZQ , Zhang H , Handa AK , Zhu JK , Zhang HM . Critical function of DNA methyltransferase 1 in tomato development and regulation of the DNA methylome and transcriptome. J Integr Plant Biol. (2019) ;61: (12):1224–42. |
[42] | Zuo JH , Wang YX , Zhu BZ , Luo YB , Wang Q , Gao LP . Comparative analysis of DNA methylation reveals specific regulations on ethylene pathway in tomato fruit. Genes. (2018) ;9: (5):266. |
[43] | Li Y , Kumar S , Qian WQ . Active DNA demethylation: mechanism and role in plant development. Plant cell Rep. (2018) ;37: (1):77–85. |
[44] | Deniz O , Frost JM , Branco MR . Regulation of transposable elements by DNA modifications. Nat Rev Genet. (2019) ;20: (7):417–31. |
[45] | Ma CQ , Jing CJ , Chang B , Ya JY , Liang BW , Liu L , Yang YZ , Zhao ZY . The effect of promoter methylation on MdMYB1 expression determines the level of anthocyanin accumulation in skins of two non-red apple cultivars. BMC Plant Bio. (2018) ;18: (1):108. |