Evolution of Acyl-CoA-binding protein gene family in plants provides insights into potential functions of grapevine (Vitis vinifera L.)
Abstract
BACKGROUND:
Grapevine was one of the most important perennial fruit crops worldwide. Acyl-CoA-binding proteins (ACBPs) in eudicots and monocots show conservation in an acyl-CoA-binding domain (ACB domain) which binds acyl-CoA esters.
OBJECTIVE:
The information and data provided in the present study contributes to understand the evolutionary processes and potential functions of this gene family in grapevine growth and development, and responses to abiotic stress.
METHODS:
Using the complete grapevine genome sequences, we investigated the number grapevine ACBP genes, the exon-intron structure, phylogenetic relationships and synteny with the Arabidopsis ACBP gene family. Furthermore, the expression profiles of VvACBP genes based on public microarray data in different tissues, and the expression patterns responding to different exogenous hormones as well as abiotic and biotic stresses were presented. The qRT-PCR was used to verify the microarray data under drought stress treatments. Finally, the leaf relative water content (RWC), leaf chlorophyll content, and enzymatic activities were measured to further examine the tolerance to drought stress in grapevine.
RESULTS:
The six grapevine ACBPs were identified. Their distribution into various groups differed from Arabidopsis and rice. Synteny analysis demonstrated that several VvACBP genes were found in corresponding syntenic blocks of Arabidopsis, suggesting that these genes arose before the divergence of the respective lineages. Sequence alignment and structural annotation provided an overview of variations that might contribute to functional divergence from Arabidopsis ACBPs. Expressional analyses suggested that both conserved and variant biological functions exist in ACBPs across different species. The expression pattern of these genes were similar in the microarray and qRT-PCR analyses. Gene structure organization and expression characteristics of VvACBPs resembled those of their Arabidopsis orthologous, although species-specific
differences also exist. Differential regulation of genes suggested functional diversification among isoforms. The biochemical and physiological data showed the tolerance to drought stress of grapevine.
CONCLUSIONS:
These findings provided insight into evolution of ACBP gene family in plants and a solid foundation for a deeper understanding of the complex molecular responses of grapevine to stress.
1Introduction
Lipids play a vital role in maintaining cell function and mediating responses to stress during plant growth and development. They contribute to the structural basis of cellular membranes, maintaining their integrity and composition, also provide energy for numerous cellular events [1]. In higher plants, two groups of proteins that bind lipids have been characterized: the lipid-transfer proteins [2] and the acyl-CoA-binding proteins (ACBPs) [3, 4]. The ACBPs have been demonstrated to bind various acyl-CoAs and phospholipids with a high specificity and affinity [3, 5– 10], and likely to participate in acyl-CoA/ phospholipid transport in the cytosol between the plasma membrane and the endoplasmic reticulum (ER) [4, 10, 11].
In animals, plants, fungi and protists, except for prokaryotes, Archaea, several plant and animal pathogens, the 10-kDa cytosolic ACBP, ranging between 82 and 92 amino acid residues were highly-conserved [10]. An overall phylogenetic analysis of the 10-kDa prototype ACBP show lineage-specific duplication and purifying selection during evolution from metazoans and non-metazoans [10]. Prototype ACBPs show conservation in structure and function spanning 1500 million year [12], but the large multifunctional proteins with a predicted ACB domain have been identified in many eukaryotes.
Plant 10-kDa ACBPs were first identified in Brassica napus [13] and Arabidopsis thaliana [14]. The overexpression of Arabidopsis ACBP6 (AtACBP6) confers freezing tolerance by upregulating the expression of phospholipase Dδ (PLDδ), which generates phosphatidic acid (PA) from phospholipids. Hence, it is speculated that AtACBP6 may participate in the intracellular binding and trafficking of phosphatidylcholine (PC) during plant lipid metabolism through its PC-binding ability [15]. AtACBP6 was also demonstrated to bind acyl-CoA esters as well as saturated (16:0 and 18:0) and unsaturated (18:1 and 18:2) PC [14– 16]. The overexpression of B. napus 10-kDa ACBP in Arabidopsis have resulted the significant increase of polyunsaturated fatty acids in developing seeds, which indicated that the cytosolic 10-kDa ACBP has an effect on the equilibrium between metabolically active acyl pools (acyl-CoA and phospholipid pools) involved in phosphatidic acid (FA) modifications and triacylglycerol bioassembly in plants. [17].
Another five larger forms of AtACBPs, their molecular weight (MW) ranging from 37.5 to 73.1 kDa were founded in Arabidopsis [3, 4]. AtACBP1 and AtACBP2 are subcellularly localized to the ER and plasma membrane [18, 19], the overexpression of AtACBP1 or AtACBP2 displayed an enhanced tolerance to heavy metals, suggesting their role in phospholipid membrane repair after heavy metal stress [16, 20]. AtACBP1 and AtACBP2 are essential in early embryo development for the embryo lethal observed in the acbp1/acbp2 double mutant [21]. The AtACBP3 is membrane-associated and apoplast-targeted [9, 22]. Recent findings reveal that AtACBP3 binds PC, phosphatidylethanolamine (PE) and acyl-CoA esters in vitro [23], and the overexpression of AtACBP3 accelerated both dark-induced and age-dependent leaf senescence [22]. In contrast, the remaining three AtACBPs (AtACBP4, AtACBP5 and AtACBP6) are cytosolic proteins [15, 24]. The AtACBP4 and AtACBP5 preferentially bind oleoyl-CoA esters in the cytosol, suggesting that these ACBPs are potential candidates for acyl-CoA transport from the chloroplasts to the ER [24].
To our knowledge, the characterized and functional analysis of VvACBP genes in grapevine (Vitis vinifera L.) had not yet been reported. Grapevine was one of the most important perennial fruit crops worldwide and the release of the grapevine genome data now allows a comprehensive genome-wide identification and analysis of VvACBP genes [25]. We also investigated the exon-intron structure, phylogenetic relationships and synteny with the Arabidopsis ACBP gene family. Moreover, the expression profiles of VvACBP genes in different tissues, and the expression patterns responding to different exogenous hormones as well as abiotic and biotic stresses were presented. Finally, we present biochemical and physiological data [the leaf relative water content (RWC), leaf chlorophyll content, and enzymatic activities] regarding drought stress condition responses to further verify the tolerance to drought stress in grapevine. We proposed to provide a solid foundation for a deeper understanding of the complex molecular responses of grapevine to stress.
2Materials and methods
2.1Identification and sequence analysis of ACB proteins in grapevine
To identify ACB proteins, a HMM (Hidden Markov Model) profile of the ACBP domain (PF00887) was first downloaded from Pfam [26] (http://pfam.xfam.org/, verified 8 Dec. 2016). Then, HMMER [27] was used to search the customized database containing the protein sequences in the annotated V. vinifera genome (v.2) which was downloaded from the Grapevine Genome CRIBI Biotech Centre (CRIBI Genomics, http://genomes. cribi.unipd.it/grape/, verified 8 Dec. 2016). The candidate HMMER-selected sequences were further confirmed by Pfam (PF00887) and SMART [28] (Sm00356; http://smart.embl-heidelberg.de/, verified 8 Dec 2016). Proteins with the presence of ACBP domain with confidence (E-value <1.0) were considered as ACB proteins. All identical sequences were checked manually to remove redundant sequences. The same HMMER approach was also used to find other protein domains in the 6 ACB proteins, including ANK (ankyrin repeat domain, PF12796) and kelch (kelch domain, PF01344). The predicted amino acid sequence, molecular weight (kDa), GRAVY, and isoelectric point (pI) of each VvACBP were calculated using the web-based ExPASy programs [29] (http://www.expasy.org/tools/, verified 8 Dec. 2016).
2.2Sequence alignments and phylogenetic analysis
Full-length ACB protein sequences of grapevine (V. vinifera), Arabidopsis (A. thaliana), rice (Oryza. sativa), maize (Zea. mays), strawberry (Fragaria. vesca), soybean (Glycine. max), Polulus. trichocarpa and Physcomitrella. patens were obtained from Phytozome (https://phytozome.jgi.doe.gov/pz/portal.html) and aligned using ClustalX v.2.0 [30]. Phylogenetic trees were constructed using MEGA6.0 [31]. The maximum-likelihood (ML) method was used to construct different trees with the pairwise deletion option. For statistical reliability, bootstrap analysis was performed with 1000 replicates to evaluate the significance of each node. Moreover, sequence logos for the ACBP motifs were produced using the web-based Web Logo software [32] (http://weblogo.berkeley.edu/logo.cgi, verified 8 Dec.2016).
2.3Exon-intron structure and synteny analysis
A diagram of the exon-intron structure in the VvACBP, AtACBP and OsACBP genes was constructed using the web-based software, GSDS2.0 [33] (http://gsds.cbi.pku.edu.cn/index.php). Synteny analysis were conducted using Circos software with the default parameters [34].
2.4Analyses of ACBP gene expression
Robust multiarray average normalized microarray expression data was downloaded from the Plant Expression Database (http://plexdb.org/, verified 8 Dec. 2016). The analysis included four microarray experiments, including response to salt, drought, cold, and oxidative stresses, as well as the application of ABA. The Affymetrix GeneChip 16K V. vinifera (Grapevine) Genome Array was used to evaluate Experiments VV1 [35], 9 [36], 13 [37], and 29 [38]. Normalized transcript expression data of VvACBP genes were analyzed in 54 different tissues of grapevine [39] (GSE36128) using Gene Expression Omnibus datasets (http://www.ncbi.nlm.nih.gov/gds/, verified 8 Dec. 2016). The multiexperiment viewer (MeV) software [40] was used to produce a heat map.
2.5Plant materials and treatments
Grapevine plants (Vitis vinifera, Pinotnoir 40024, the sequenced genotype) were cultivated in growth chamber with 22 °C in a 8/16 h dark/light photoperiod and 70– 80% relative humidity. For the first four weeks, grapevine plants were well watered. Then, water was withheld to impose drought treatment. We just stopped watering the plants. The plants withheld of water were growing in outdoor cultivation shed. Grapevine leaf samples were collected at 0 (control), 2, 4, and 8 days after water was withheld. All the collected samples were frozen in liquid nitrogen immediately and stored at – 80 °C for further analysis. Three biological replicates were used for each treatment or control.
2.6RNA isolation and real-time quantitative RT– PCR analysis
Total RNA was extracted from grapevine leaves using the Plant Total RNA Isolation Kit (Foregene, Chengdu, China) according to the manufacturer’s instructions. The genomic DNA was removed from the sample using DNase I (RNase-free DNase set, Qiagen, Hilden, Germany), and RNA concentration and quality were measured by determining the optical density (OD) ratios at 260 nm and 280 nm with a One DropOD-1000 spectrophotometer (Thermo Scientific, Wilmington, DE). Then, first strand cDNA was synthesized from 1μg total RNA using Prime Script RT Reagent Kit (Takara, Japan) according to the manufacturer’s instructions.
Specific primers for the six grapevine ACBP genes were designed using the IDT Primer Quest tool (http://sg.idtdna.com/Primerquest/Home/Index). The actin gene (VIT_212s0178g00200) was used as a reference gene [41]. The qRT– PCR was conducted using a LightCycler 480 SYBR Green I Master (Roche, Mannheim, Germany) on a Roche 480 Real-Time PCR System (Roche, Mannheim, Germany). The qRT– PCR was performed according to the manufacturer’s protocol, and the total volume of each reaction was 15μl. The PCR amplification conditions were as follows: 95°C 10 min, 40 cycles of 10 s at 95°C, 20 s at 58°C, and 10 s at 72°C. The relative expression levels of the target genes were calculated by the 2–ΔΔCt method.
2.7Measurement of grapevine leaf relative water content (RWC)
Measuring leaf relative water content (RWC) was one of reliable way to get the water status of a leaf without any need for special equipment. Grapevine leaf samples were collected at 0 (control), 2, 4, 6, and 8 days after water was withheld, with two samples at each time point. Weighed the fresh leaves to determine the fresh leaf mass (FM) values for each leaf dehydration stage. Leaf dry mass (DM) was determined after oven drying for one hour at 150 °C. Another sample at each time point was putted into water for one hour. The saturated mass (SM) was estimated. RWC was determined for each stage of leaf dehydration as RWC=(FM – DM)/ (SM – DM) *100% [42]. The experiment was carried out with three biological replicates and three technical replicates per biological replicate.
2.8Measurement of leaf level chlorophyll content
Grapevine leaves Chlorophyll content was measured by ethanol extraction colorimetry. Leaf samples were collected at 0 (control), 2, 4, 6 and 8 days after water was withheld. The fresh samples were shredded. Then we putted them into 95% ethanol with a total volume of 20 mL for 24 hours. The absorbance at 665, 645, and 470 nm was calculated to estimate chlorophyll a and b content [43]. The experiment was carried out with three biological replicates and three technical replicates per biological replicate.
2.9Measurement of enzymatic activities
Antioxidant enzyme [superoxide dismutase (SOD) and peroxidase (POD)] activities were quantified using the relevant protocols according to Zhang [44] SOD activity was assayed by using the photochemical nitroblue tetrazolium (NBT) method. The photo-reduction of NBT was measured at 560 nm. POD activity was measured with guaiacol as the substrate, and the absorbance was measured at 470 nm. The experiment was carried out with three biological replicates and three technical replicates per biological replicate.
2.10Statistics analysis
A student’s t-test (P < 0.01 and P < 0.05) was performed, where appropriate, using the IBM SPSS Statistics v25 software (SPSS, Inc., USA) [45].
3Results and discussion
3.1Identification and classification of grapevine ACBP genes
In this study, we identified six grapevine ACBP genes designated as VvACBP1 to VvACBP6, respectively (Table 1). The ACB domain was located at the N-terminal segment in each VvACBP1/2/3/6, however VvACBP4 and VvACBP5 contain a C-terminal ACB domain. Six VvACBP domains were aligned to generate sequence logos and compared to those in Arabidopsis and rice (Fig. 1). It indicated that all of the ACBP domain of grapevine, Arabidopsis and rice share the same conserved distribution X6-L-X2-L-X3-A-X2-G-X6-P-X9-KW-X2-W-X10-AW homo-domains (Fig. 2). Amino acid mutation sites more focused on central sequence, less variation on both ends. However, differences were observed in the combined sequence logos among Arabidopsis, rice, and grapevine. For example, Tyrosine (Y) was more conserved at position 8 of the in grapevine, while Glutamic acid (E) was more conserved at position 49 in the Arabidopsis, and Leucine (L), Glicine (G) more conserved at position 5, 9 in the rice (Fig. 2).
Table 1
Genome position and annotation reference for VvACBP genes, as given on the Genotype website or at NCBI/Genbank, their deduced polypeptides and predicted subcellular localization
Gene name Locus | tag/gene ID | NCBI accession No | Number of amino acids | Molecular weight | Theoretical pI | Grand average of hydropathicity (GRAVY) | Subcellular localization | Chromosome location |
VvACBP1 | VIT_202s0033g01330 | CBI37468.3 | 91 | 10240.55 | 5.22 | – 0.678 | Golgi_and_Endoplasmic_Reticulum | Chr2 from 16785784 to 16789538 Strand+ |
VvACBP2 | VIT_211s0016g01350 | XP_010656483.2 | 88 | 9886.28 | 6.28 | – 0.659 | Golgi_and_Endoplasmic_Reticulum | Chr11 from 1091492 to 1093482 Strand – |
VvACBP3 | VIT_216s0098g01570 | XP_010662896.1 | 351 | 37859.85 | 4.4 | – 0.404 | Secreted_and_Endoplasmic_Reticulum_and_Membrane | Chr16 from 21733789 to 21737586 Strand+ |
VvACBP4 | VIT_207s0129g00430 | XP_019076864.1 | 478 | 51646.52 | 4.14 | – 0.192 | Extracellular | chr7 from 15677482 to 15682565 Strand+ |
VvACBP5 | VIT_218s0001g09340 | NP_001268019.1 | 289 | 31633.96 | 4.35 | – 0.426 | Extracellular | Chr18 from 7839549 to 7845389 Strand+ |
VvACBP6 | VIT_214s0060g02410 | XP_010659627.1 | 674 | 73683.54 | 5.14 | – 0.416 | Cytoplasm_and_Nucleus | Chr14 from 2034805 to 2046583 Strand – |
Fig. 1
Protein sequence alignment and ACBP domain structure of six predicted Acyl-CoA-binding (ACB) proteins in grapevine. Sequence logos for common ACBP domains. ACBP domains of grapevine (Vitis vinifera L.), rice (Oryza sativa L.) and Arabidopsis
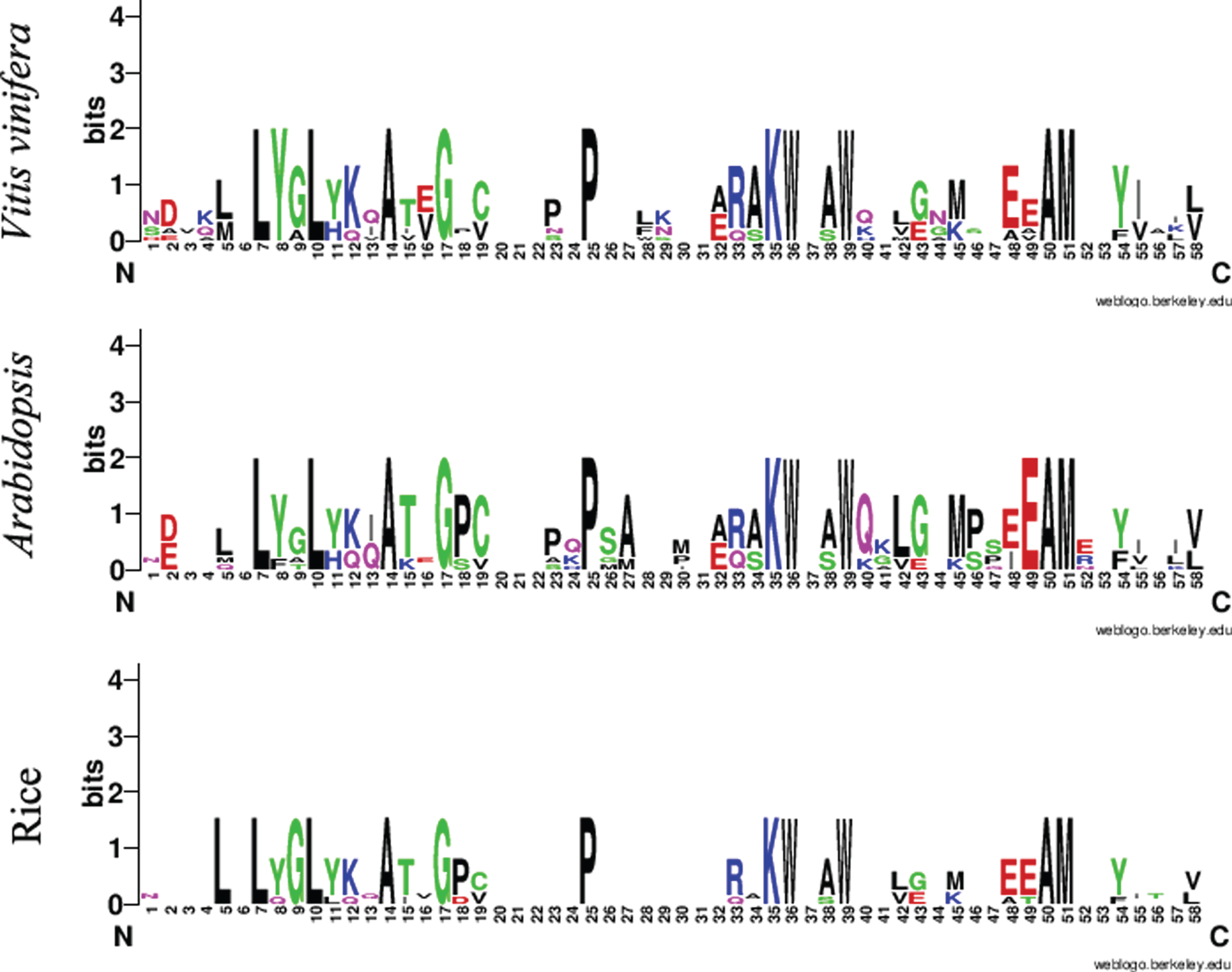
Fig. 2
Comparison in architecture of the grape and Arabidopsis acyl-CoA-binding protein(ACBP) families. The ACB domain, ankyrin repeats, kelch motifs, putative transmembrane region, signal peptide and coiled coil region are shown. Six grape ACBPs are designated as VvACBP1-VvACBP6 (protein size indicated in the brackets). Arabidopsis ACBPs are designated as AtACBP1-AtACBP6.
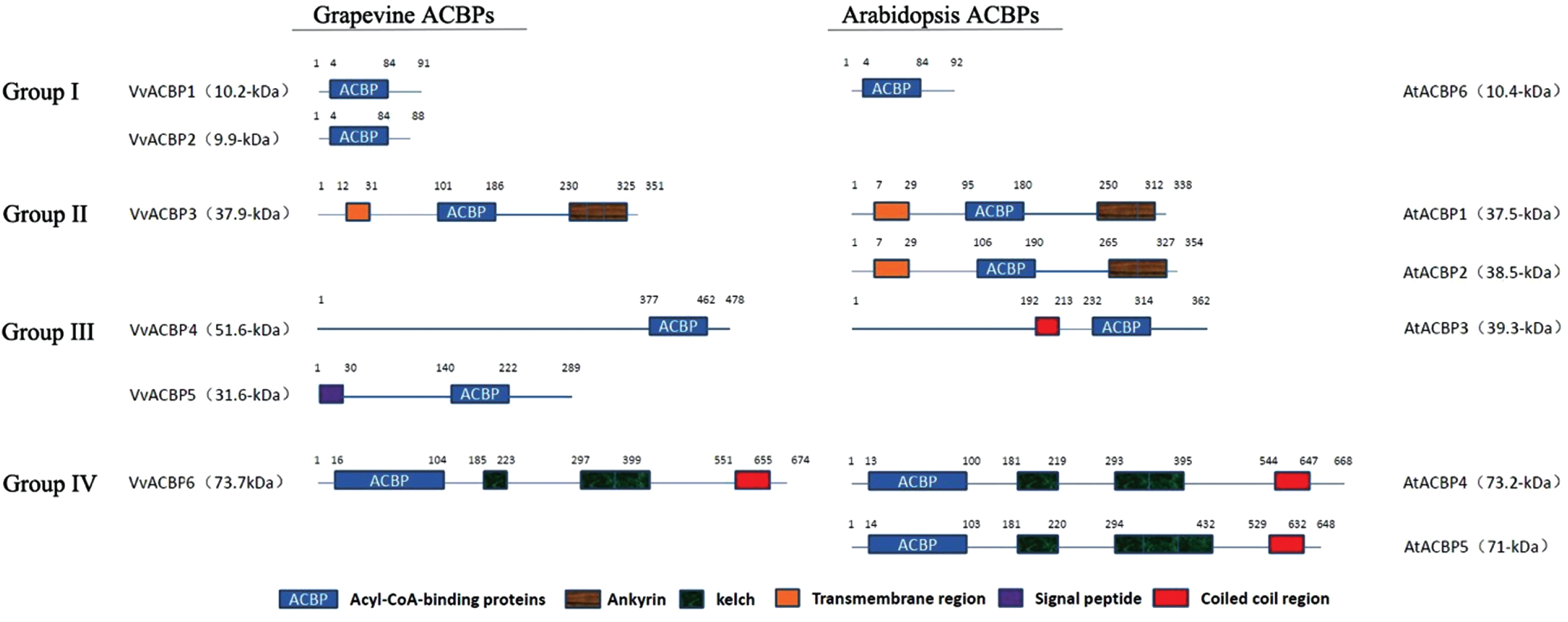
The ACBPs in plants were classified into four groups: Group I, small ACBPs; Group II, ANK-ACBPs; Group III, large ACBPs; and Group IV, Kelch-ACBPs. Two of six grapevine ACBPs belonged to Group I, VvACBP1 and VvACBP2. Group I proteins consisted of an average of 100 amino acid residues and were present in almost every plant species examined [10]. Amino acids of VvACBP1 and VvACBP2 were 88 and 91, the subcellular localizations of them were predicted to the ER and Golgi, respectively. While AtACBP6, OsACBP1, and OsACBP2 belonged to Group I were located at the cytosol. VvACBP3 of Group II was also located at the ER consisted with the ER-targeted Group II members (AtACBP1, AtACBP2 and OsACBP4), which indicated a fact that members of Group II displayed a conservation within subcellular localization [46]. The localization of Group I (VvACBP1, VvACBP2) and Group II (VvACBP3) overlapped at the ER. Given that these grapevine ACBPs belonged to distinct groups, they might have essential and non-overlapping roles at the ER. Seed storage reserved such as TAG was synthesized at the ER and then stored in the form of lipid droplets and protein bodies [47, 48]. ER bodies were ER-derived structures associated with protein storage and resistant to insects or pathogens in Arabidopsis [49]. Expression of the Arabidopsis ER body membrane proteins, Membrane Protein of Endoplasmic Reticulum Body1 (MEB1) and MEB2, in yeast enhanced tolerance to metal stress [50]. These observations indicated that ER bodies were closely related with stress responses.
VvACBP4 and VvACBP5, the Group III VvACBPs, was localized to the extracellular, but the AtACBP3 and OsACBP5 had been detected in the apoplast and periphery of the ER and Golgi [9, 22]. VvACBP6 (Group IV) was localized to the extracellular cytoplasm and nucleus, but the same group members, AtACBP4 and AtACBP5 was located at the cytosol, and the OsACBP6 had an unusual localization in the peroxisomal matrix [46]. We were interested in the potential connection between cellular localization and functionalities of the VvACBP protein and further experimental analyses were being carried out.
All these six genes (VIT_202s0033g01330, VIT_211s0016g01350, VIT_216s0098g01570, VIT_207s0129g00430, VIT_218s0001g09340 and VIT_214s0060g02410) were mapped to the specific grapevine chromosome (2, 11, 16, 7, 18 and 14). Amino acids in length of these ACBP genes ranged from 88 to 674. Their corresponding molecular weight (MW) varied from 9.86 to 73.68 kDa with isoelectric point (pI) values from 4.14 to 6.28, and the grand average hydropathy (GRAVY) values ranged from – 0.192 to – 0.678 (Table 1).
3.2Phylogenetic analysis of VvACBPs
To further examine the evolution of the ACB protein family, the predicted VvACBP amino acid sequences were compared with ACBPs from seven represent species, F. vesca, A.thaliana, G. max, Z. mays, O. sativa, P. trichocarpa and P. patens to conduct a phylogenetic tree. In the maximum-likelihood (ML) phylogenetic tree, the 58 ACB protein genes included four strawberry ACBPs, five rice ACBPs, six grapevine ACBPs, six Arabidopsis ACBPs, nine maize ACBPs, nine moss ACBPs, eight poplar ACBPs, and 11 ACBPs from soybean. Four groups of orthologous were identified (Fig. 3).
Fig. 3
Phylogenetic analysis of grapevine ACB proteins with other plant. The phylogenetic tree was constructed with ACBP domain protein sequences from V. vinifera (VvACBP), Z. mays (ZmACBP), O. sativa (OsACBP), A. thaliana (AtACBP), F. vesca (FvACBP), G. max (GmACBP), P. trichocarpa (PtACBP) and P. patens (PpACBP). They were classified to four groups: I, II, III, IV. VvACB proteins are indicated with red squares and AtACB proteins with green triangles and OsACB proteins with blue diamonds. All accession numbers or locus IDs of the ACBP genes are listed in the phylogenetic tree.
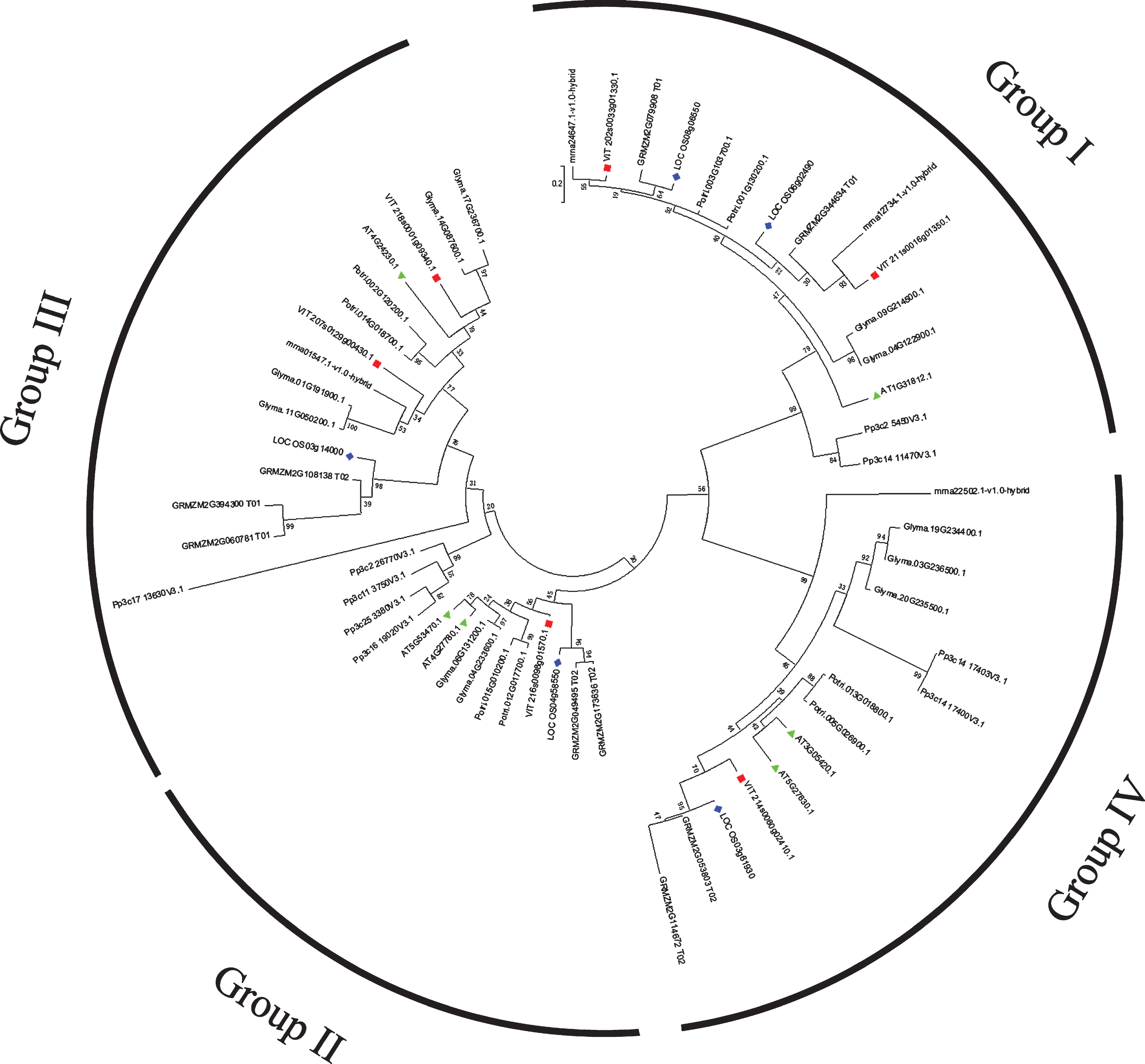
According to the phylogenetic tree, the VvACBP sequences were distributed amongst all groups, VvACBP1 and VvACBP2 belonged to Group I, VvACBP3 possessed ankyrin repeats and belonged to Group II, VvACBP4 and VvACBP5 were designated Group III and kelch motif-containing, VvACBP6 was a Group IV protein. In the clustering analysis of the eight species, Group I ACBPs were conserved from P. patens to higher plants with either one or two members. The apparent absence of group II in F. vesca and P. patens might result from the incomplete genome sequences available (Fig. 3). These results suggested that there were still not stable after the divergence of angiosperms. The presence of multiple groups of ACBPs in early-divergent plant lineages as well as in higher plants, and observations of conservation of four groups among higher plants, supported a previous report that land plants retained multifold ACBP functions across lineages [23]. As the P. patens possessed multiple groups I, II and IV members, we suggested that duplication events within groups I, II and IV occurred before the origin of land plants. Interestingly, the strawberry ACBP family emerged as the smallest which comprising 4 members, while the soybean ACBP family, comprising 11 members, emerged as the largest among all species examined (Fig. 3). A final determination of whether ACBPs evolved from four ancestral genes, however, still required more supportive data.
3.3Gene structure analysis of VvACBPs
The conserved motif analysis of VvACBPs supported the phylogenetic relationship and classification of grapevine ACBPs (Fig. 1). It showed differences of number and type of conserved motif, which revealed different functions of these ACBP genes
All VvACB proteins contained the complete ACBP domains. In Group I, VvACBP1, VvACBP2 and AtACBP6 only consisted a ACBP domain. Group II (VvACBP3, AtACBP1, AtACBP2) and IV (VvACBP6, AtACBP4, AtACBP5) were multi-domain proteins, Group II contained a transmembrane region and a C-terminal ankyrin repeats, which enabled ACBPs to interact with partner proteins [20, 51– 53], Group IV contained two kelch motifs and a coiled coil region at the N-terminus which was different from previous studies [23] (Fig. 2). VvACBP4, VvACBP5 located at Group III contains a C-terminal ACB domain and the majority were accompanied by a signal peptide or a coiled coil region at the N-terminus that dictates subcellular localization.
Intron– exon structures were identified based on the coding sequences and the corresponding genome sequences (Fig. 4). Intron– exon organization of the six VvACBP, six AtACBP,, and five OsACBP genes indicated that VvACBP genes could be classified into four clusters. The exon-rich cluster included VvACBP6 in Group IV containing 18 exons, and the exon-less subgroup Group I, II, and III included the other five grapevine ACBP genes with only 4– 6 introns (Fig. 4). The similar intron rich/poor pattern of ACBP family members was also observed in both monocot (rice) and dicots (Arabidopsis). Genes in Group I (AtACBP6, OsACBP1, OsACBP2, VvACBP1 and VvACBP2) contained 4 exons, genes in Group II (AtACBP1, AtACBP2, OsACBP4, and VvACBP3) all contained 6 exons, and genes in Group IV (AtACBP4, AtACBP5, OsACBP6, and VvACBP6) all contained 14 or more exons. However, the number of exons in Group III genes was uneven, such as AtACBP3 contained 4 exons, OsACBP4 contained 5 exons, while VvACBP4, VvACBP5 contained 3 and 6 exons each.
Fig. 4
Phylogenetic analysis and exon-intron structures of VvACBP, AtACBP and OsACBP genes. Numbers above or below branches in the tree indicate bootstrap values. Exons and introns are indicated by yellow boxes and black horizontal lines, respectively.
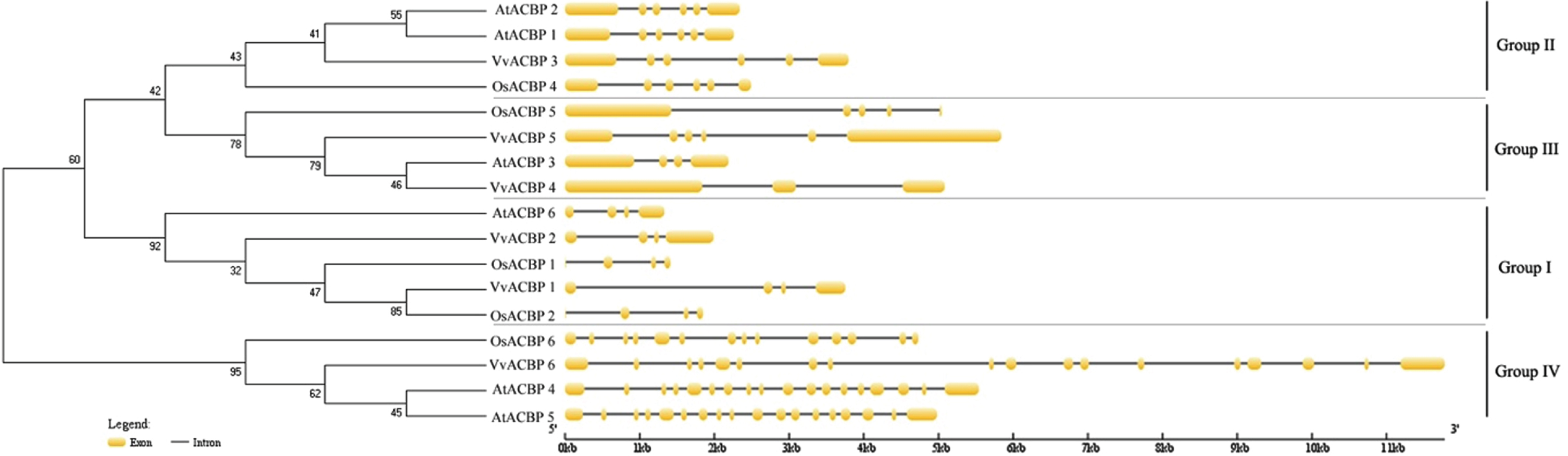
Comparing with the intron distributions of VvACBPs within different groups deduced that the derivative forms of VvACBPs might associate with the products of gene duplications, which were based on intron losses from the earlier multiple intron homologs. Because of high intron gain rates in earlier eukaryotic evolution, Thus, the expansion of ACBP family was dependent on the intron losses from the ancient multiple intron ACBP lineages along gene duplication, which was consistent of the previous studies of high intron gain rates in earlier eukaryotic evolution, reduction of intron number in recent eukaryotic evolution was a common feature [54].
3.4Chromosome locations and synteny analysis of VvACBP and AtACBP genes
Genomic comparison was a convenient and often effective way to transfer knowledge of genome structure and function gained from a well-studied taxon to a species where less information is available [5]. Thus, the predicted function of the VvACBP genes might be suggested by a comparison with their respective orthologous in the model plant Arabidopsis, whose ACBP genes had previously been characterized.
Syntenic groups containing orthologs of two VvACBP genes and three ACBP genes from Arabidopsis were identified (Fig. 5). According to this analysis, three paired VvACBP-AtACBP genes (VvACBP3-AT4G27780, VvACBP6-AT3G05420 and VvACBP6-AT5G27630) were located in genomic regions with synteny between the grapevine and Arabidopsis genomes (Table S1, S2), indicating that these genes may be derived from a common ancestor. Based on this type of comparative genomic analysis, it was possible to deduce potential function of genes to guide future functional studies.
Fig. 5
Synteny analysis of ACBP genes from grape and Arabidopsis. The positions of related VvACBP genes and AtACBP genes are depicted in the grape chromosomes and Arabidopsis chromosomes, respectively. Colored lines connecting two chromosomal regions indicate syntenic regions between grape and Arabidopsis chromosomes.
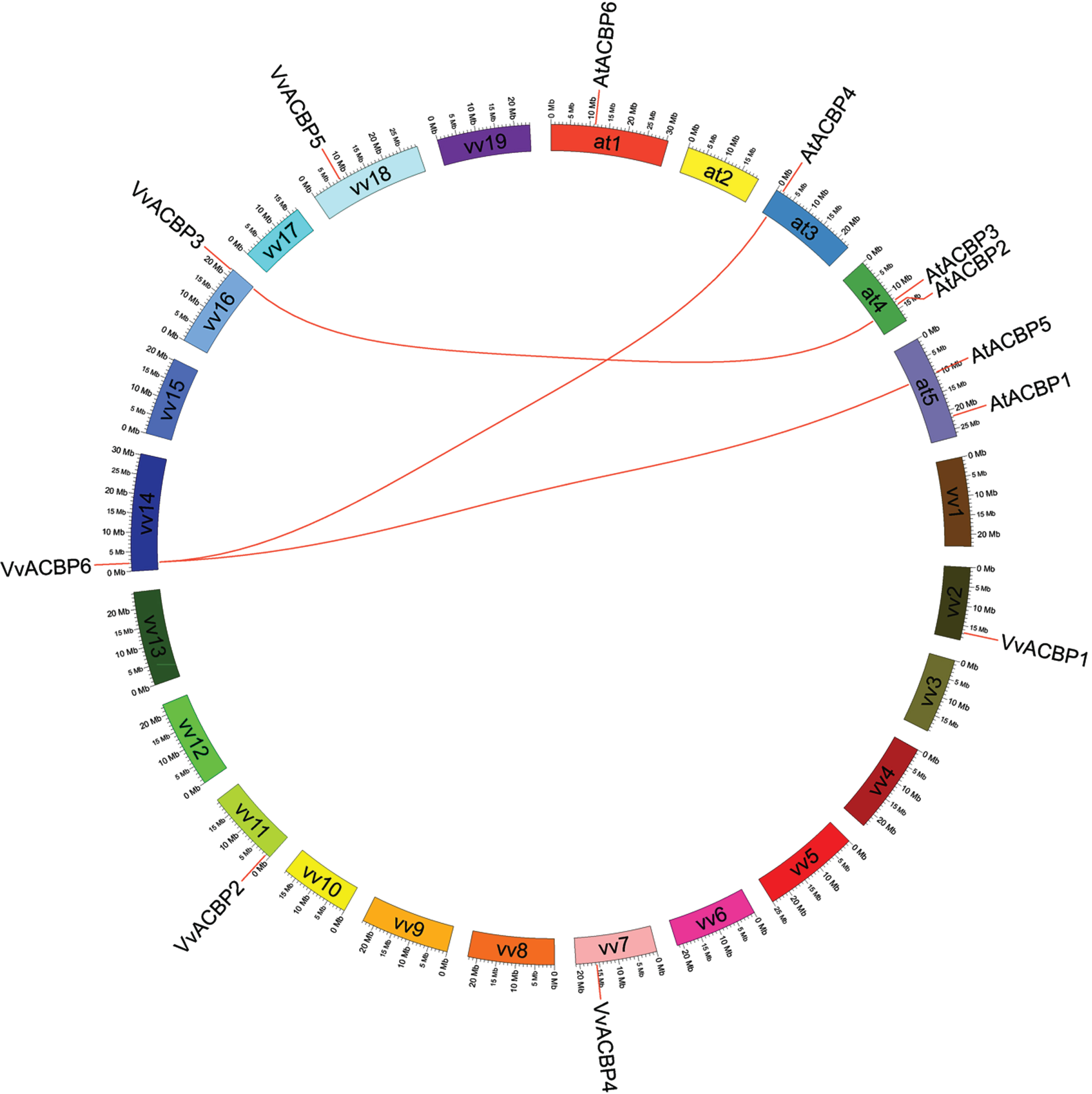
Previous study showed that the expression of AtACBP2::GUS was detected in the guard cells [55] and could be induced by drought stress responses and oxidative stress (H2O2). And their overexpression in transgenic plants had reduced lipid hydroperoxide content following H2O2 treatment and promoted membrane restoration after oxidative stress [20, 52, 56]. For the AtACBP4 and AtACBP5 with kelch motifs have been verified to interact with protein partners via these domains and mediate protein-protein interactions [4, 57, 58]. AtACBP4 also could contribute to drought tolerance by playing a role in cuticle formation [59]. It was possible that the grapevine homologs of these three Arabidopsis protein could be involved in similar functions; further experimental analyses were necessary to confirm this.
3.5Cis-Regulatory elements analysis of VvACBP genes
The Stress-related cis-elements including ABRE (involved in the response to ABA), LTR (involved in response to low temperature), HSE (involved in response to heat and oxidative stress), MBS (involved in drought-inducibility), W-box (fungal elicitor responsive element), Wun-motif (wound-responsive element), ARE (essential for the anaerobic induction), and TC-rich repeats (involved in defense and stress responsiveness), had been extensively characterized for their important roles under stress conditions [60– 63]. Stress-responsive elements were investigated in the promoter regions of VvACBPs to explore the possible responsive mechanisms of grapevine ACBP genes to abiotic stress and detected in the six ACBP gene promoter regions (1500 bp upstream of the translation start site) of VvACBPs. In the promoter regions of six VvACBPs, 42 cis-elements were involved in responding abiotic stresses (Table 2). The number of stress-responsive elements in the selected promoter regions of six VvACBPs ranged from 5 (VvACBP4) to 8 (VvACBP2 and VvACBP5). All the six promoter regions of VvACBPs contained ARE elements and TC-rich repeats (Table 2). An ABRE element was found in four promoter regions of VvACBP1/2/5/6. Four HSE elements and two MBS, W-box elements were located in the promoter regions of four genes and two genes, respectively. Moreover, other stress-responsive cis-elements were detected, including Wun-motif only in gene (VvACBP6), and LTR element in one gene (VvACBP3), respectively. Therefore, most ACBP family members in grapevine could be induced and transcriptionally regulated during different abiotic stresses.
Table 2
The main cis-element analysis of VvACBP genes promoter sequences.
Element name | Numbers of each member contains and their location | |||||
VvACBP1 | VvACBP2 | VvACBP3 | VvACBP4 | VvACBP5 | VvACBP6 | |
ABRE | 2 (656,1088) | 1 (919) | 3 (324,326,327) | 1 (281) | ||
LTR | 1 (1370) | |||||
HSE | 2 (825,1147) | 2 (171,710) | 2 (853,1361) | 2 (166,721) | ||
MBS | 1 (107) | 1 (269) | ||||
W-Box | 1 (219) | 1 (711) | ||||
Wun-motif | 1 (498) | |||||
ARE | 1 (1195) | 2 (572,673) | 3 (204,799,1039) | 2 (641,1230) | 3 (748,753,1437) | 1 (855) |
TC-rich | 1 (883) | 3 (50,365,831) | 1 (607) | 1 (891) | 1 (1300) | 2 (1377,1474) |
Stress-responsive cis-elements ABRE (response to ABA), LTR (response to low temperature), HSE (response to heat and oxidative stress), MBS (drought-inducibility), W-box (fungal elicitor responsive element), Wun-motif (wound-responsive element), ARE (anaerobic induction), and TC-rich repeats (defense and stress responsiveness).
3.6Expression patterns of VvACBP genes in different tissues and organs
There were several similarities in the spatial expression models of VvACBP genes and those from other plant species. The VvACBP1, VvACB2, OsACBP1, OsACBP2 and AtACB6 proteins were closely related and this was also reflected by their presence within the same phylogenetic group I (Fig. 6). AtACBP6 was expressed preferentially in the siliques, pollen grains, microspores, tapetal cells [64], also expressed in the cotyledons, hypocotyl and cotyledonary-staged embryo [65], and developing seeds than in roots and leaves [14], suggesting their overlapping roles in pollen development. While the closely related VvACBP1 was highly expressed in berries, slightly expressed in stamen, inflorescence, seeds and seedlings, VvACBP2 was highly expressed in bud-AB, leaf-S, berryskin-V and berryskin-PHWI.
Fig. 6
Expression profiles of VvACBP genes identified in 54 different grapevine tissues. Intensity of expression is represented in the colored bar at the top of the chart. Fifty-four different tissues were listed in the up. The scale bars represent values from 0 to 0.60191137.

AtACBP1 and AtACBP2, two members of groups II, with highly conserved homologues of 82% identity, and similar expression patterns, were identified to be widely expressed in various organs throughout Arabidopsis development, flowers [6, 7, 18, 20, 52, 55, 66], siliques [6, 7, 18, 20, 52], mature seeds [18, 21, 55, 66, 67], seedlings [55, 66, 67], rosettes [55, 66] and mature plants [6, 7, 20, 52, 55, 66, 68], highly expressed in Arabidopsis embryos. But the results of GUS reporter assays revealed that AtACBP1::GUS was expressed in the trichomes [66] whereas AtACBP2::GUS was detected in the guard cells [55]. These were consistent with their function in heavy metal and drought stress responses, respectively, as trichomes had been previously reported to accumulate lead [Pb(II)] [69, 70], while the guard cells controlled water loss. In addition, their high expression in siliques, particularly in developing and mature seeds, indicated potential roles in seed development, dormancy and germination [18, 21, 55, 66], and the related VvACBP3 is highly expressed in bud-W, berryflesh, berrypericarp, seed-V, berryskin and stem-W.
Group III, AtACBP3 was expressed in flowers, siliques, seedlings, rosettes and mature plants [3, 22, 71]. From GUS assays, AtACBP3 was strongly expressed in the phloem tissue of leaves and stems [71]. AtACBP3 could be involved in phloem-associated long-distance lipid signaling and lipid metabolism [72, 73] for the binding capability with long-distance lipid [9, 22, 74], PC and PE [22]. Observations in the expression of AtACBP3 at the stigma also indicate a potential role related to reproduction [71], and the related VvACBP4, VvACBP5 are highly expressed in bud, inflorescences, stem, berry, richis, seed and root. Expression of VvACBP was detected in grapevine leaves exposed to tunicamycin-induced endoplasmic reticulum (ER) stress as well as cold and heat shock treatments. The transgenic Arabidopsis plants exhibited thicker inflorescences and rosette leaves and disease resistance to Pseudomonas syringae and Colletotrichum higginsianum than those of the control plants, suggesting that VvACBP may also enhance disease resistance in grapevine [75] (Our sequence alignment indicated that it was VvACBP5).
Groups IV, AtACBP4, AtACBP5, the two cytosolic AtACBPs, were expressed in flowers [3, 15, 53, 76], siliques [3, 14, 15, 53, 76], mature plants [3, 14, 15, 24, 53, 76], and seedlings [76]. Recent expression profiles by quantitative RT-PCR also showed that AtACBP4 and AtACBP5 were highly expressed in the inflorescences [64]. Subsequent GUS assays revealed the expression of AtACBP4 in pollen grains, AtACBP5 in microspores and tapetal cells. This was similar to the expression patterns observed for VvACBP6, which was highly expressed nearly in all organs except the leaf-FS, leaf-S and berryskin-R. It therefore seems that members of individual ACBP groups have similar expression signatures, again suggesting that there may be conserved functionality amongst members of the same groups.
3.7Expression profiles and qRT– PCR expression analysis of VvACBP genes following various stress treatments
The microarray data (Table S3) obtained from the Affymetrix Gene Chip 16K V. vinifera Genome Array (Fig. 7) enabled the analysis of the expression of six VvACBP genes. The expression profiles of the six VvACBP had been investigated in response to various experimental treatments, including several abiotic stresses [35, 38, 77, 78].
Fig. 7
Expression profiles of VvACBP genes identified in PLEXdb. The intensity of expression is represented in the colored bar at the top of the chart. Expressed under conditions listed in the middle. The scale bars represent values from 0 to 0.23060082.
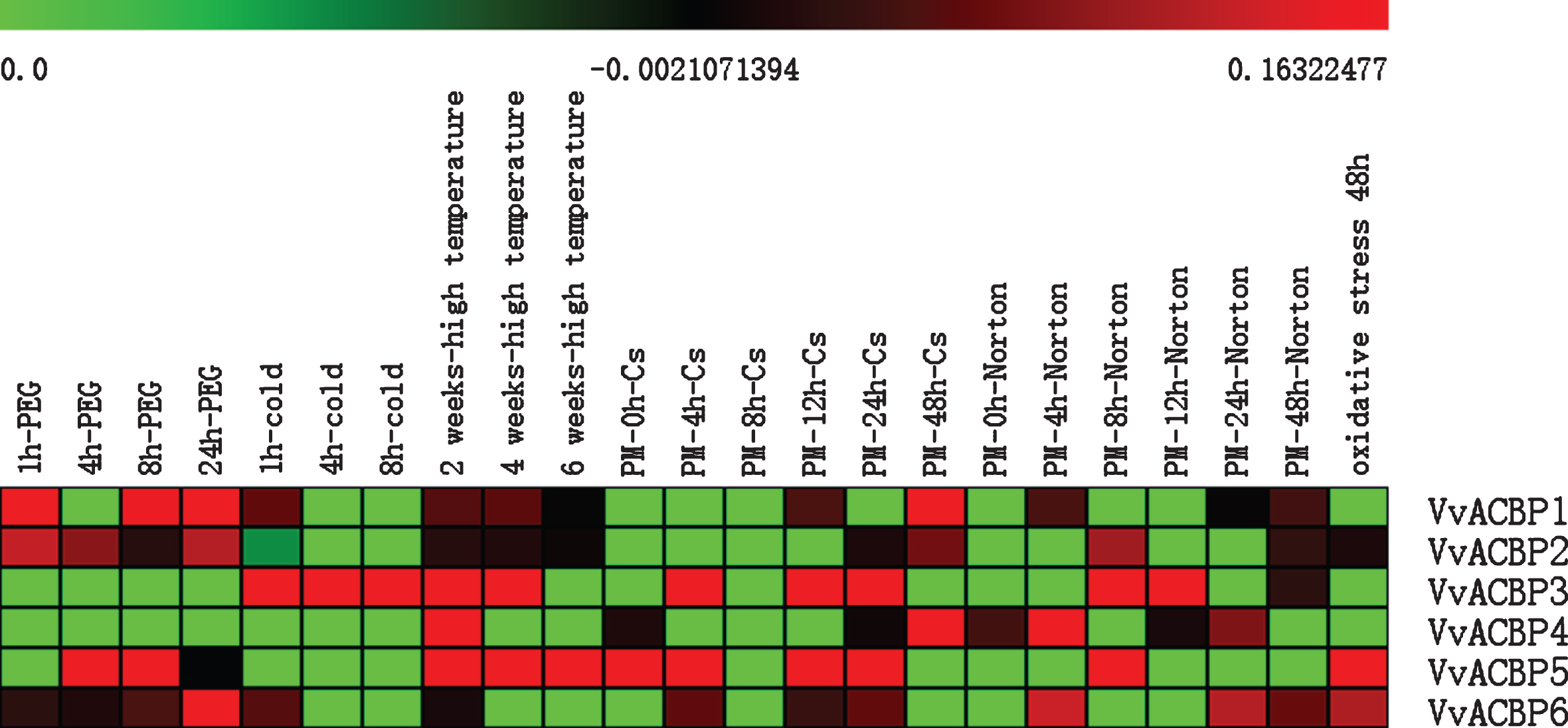
Low temperature stress could cause substantial losses in agriculture. In Arabidopsis, AtACBP1 and AtACBP6 had been reported to be engaged in the modulation of membrane lipids (e.g. PC and PA) in response to freezing stress [15, 79]. The overproduction of AtACBP6 in Arabidopsis dramatically improved freezing tolerance in flowers, up to 30% more intact flowers survived in AtACBP6-overexpressors when compared to the wild type [80]. This finding suggested that AtACBP6 was a promising candidate for the genetic engineering of cold tolerance. VvACBP1 and VvACBP2, the homologous of AtACBP6 genes in Group I exhibited increased expression in response to the cold treatment of 5°C, slightly up-regulated at 1 h and then its expression decreased. The two genes also exhibited continually increased expression in response to high temperature. Remarkably, the expression of VvACBP4 and VvACBP5 in Group III, was obviously up-regulated following the high temperature treatment (Fig. 7). Most notable, VvACBP3, the homologous of AtACBP1 and AtACBP2 in Group II was obviously up-regulated which might correlate with the special cis-element LTR in its promoter region, also, VvACBP3 exhibited an increased expression in response to the high temperature treatment at 2 weeks and 4 weeks then its expression decreased at 6 weeks.
The overexpression of AtACBP2 showed improved drought tolerance, which was proposed that the accumulation of reactive oxygen species (ROS) in the guard cells promoted stomatal closure to reduce water loss [55]. AtACBP3, AtACBP4 and AtACBP6 also could contribute to drought tolerance, which corresponded to significant water loss under drought conditions by playing a role in cuticle formation [59], suggesting the importance of these AtACBPs in cuticular lipid metabolism and drought tolerance [59]. VvACBP1 and VvACBP2 weas in Group I, and VvACBP6 in Group IV, these genes showed their highest level of expression at 24 h after the PEG treatment. Notably, VvACBP1 was slightly down-regulated at 4 h after the PEG treatment and then its expression increased by 8 h and 24 h, and VvACBP5 in Group III was up-regulated at 4 h, 8 h after the salt treatment and then its expression decreased by 24 h (Fig. 7). The overexpression of AtACBP1 and AtACBP2 in transgenic plants reduced lipid hydroperoxide content following oxidative stress (H2O2) and promoted membrane restoration after oxidative stress [20, 52, 56]. In response to oxidative (H2O2) stress, two VvACBP genes, VvACBP5 and VvACBP6, exhibited the 2.6 and 1.5-fold increase in expression, respectively (Fig. 7).
The ACBP genes were reported played key roles in response to biotic stress. AtACBP3 had been reported to participate in plant defense against infections caused by pathogens, and exemplary in this regard [59, 81], which might be attributed to differences in plant-pathogen recognition as well as the plant-defense pathways mediated [82]. VvACBP4 and VvACBP5, the homologous of AtACBP3 genes in Group III of V. vinifera ‘Cabernet sauvignon’ and V. aestivalis ‘Norton’ innoculated with Erysiphe necator conidiospores exhibited continued high expression respectively at the different time periods. A previous study had shown that the overexpression of AtACBP3 promoted the degradation of the autophagy protein ATG8, which was involved in plant innate immunity-associated programmed cell death. Further investigations suggested that the knockout mutants of AtACBP3, AtACBP4 or AtACBP6 showed diminished capability in the generation of signals such as SA and SA glucoside for induction of systemic acquired resistance.
The qRT-PCR were used to further analyze the expression patterns in the six grapevine ACBP genes under drought treatments. Specific primers for the six VvACBPs were listed (Table S4). The qRT-PCR results showed that the five VvACBPs had different expression patterns under drought stresses (Fig. 8). For instance, VvACBP1 were highly down-regulated at the 8-day time point, whereas VvACBP5 were up-regulated at the same time point, significantly. The expression pattern of these two genes were similar in the microarray and qRT-PCR analyses. These analyses provided direct evidence of gene functions in response to drought stress treatments.
Fig. 8
The expression patterns of grapevine ACBP genes under drought stresses. The qRT– PCR expression data of 5 selected VvACBPs subjected to drought stress by withholding water for 0 (control), 2, 4, and 8 d after full water saturation. Values are the means±SE of three replicates for each, and bars with different letters are significantly different at P < 0.05 according to T-test.
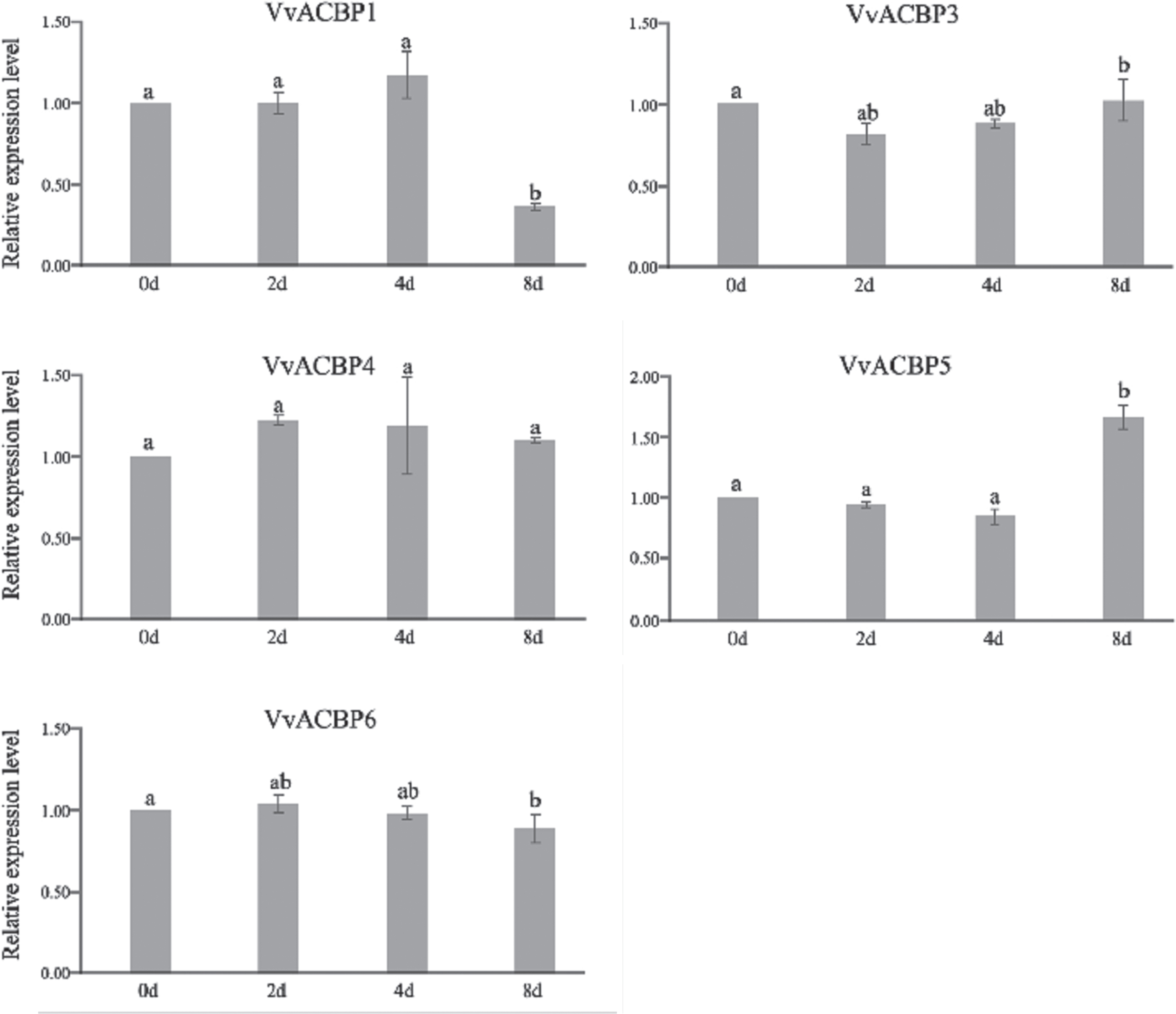
3.8Analysis of biochemical and physiological indexes in grapevine under drought stress
To further verify the tolerance to drought stress in grapevine, we measured relative water content (RWC), leaf chlorophyll content, and enzymatic activities. After 2, 4, 6, and 8 d drought treatment, the leaf RWC was decreased compared with the control (0 d). Remarkably, after 6 d water withheld, the leaf RWC was significantly lower than that in the control stage in grapevine. After 8 d drought treatment, the RWC of leaf was 14.9%, decreased rapidly (Fig. 9A). The result showed the leaves strongly dehydrated after 8 d drought treatment. The leaf water status reflected the tolerance of drought stress in grapevine.
Fig. 9
The biochemical and physiological traits of grapevine under drought stresses. (A) Leaf relative water content (RWC); (B) Leaf Chlorophyll content; (C) POD activity; (D) SOD activity. Values are the means±SE of three replicates for each, and bars with different letters are significantly different at P < 0.05 according to T-test.
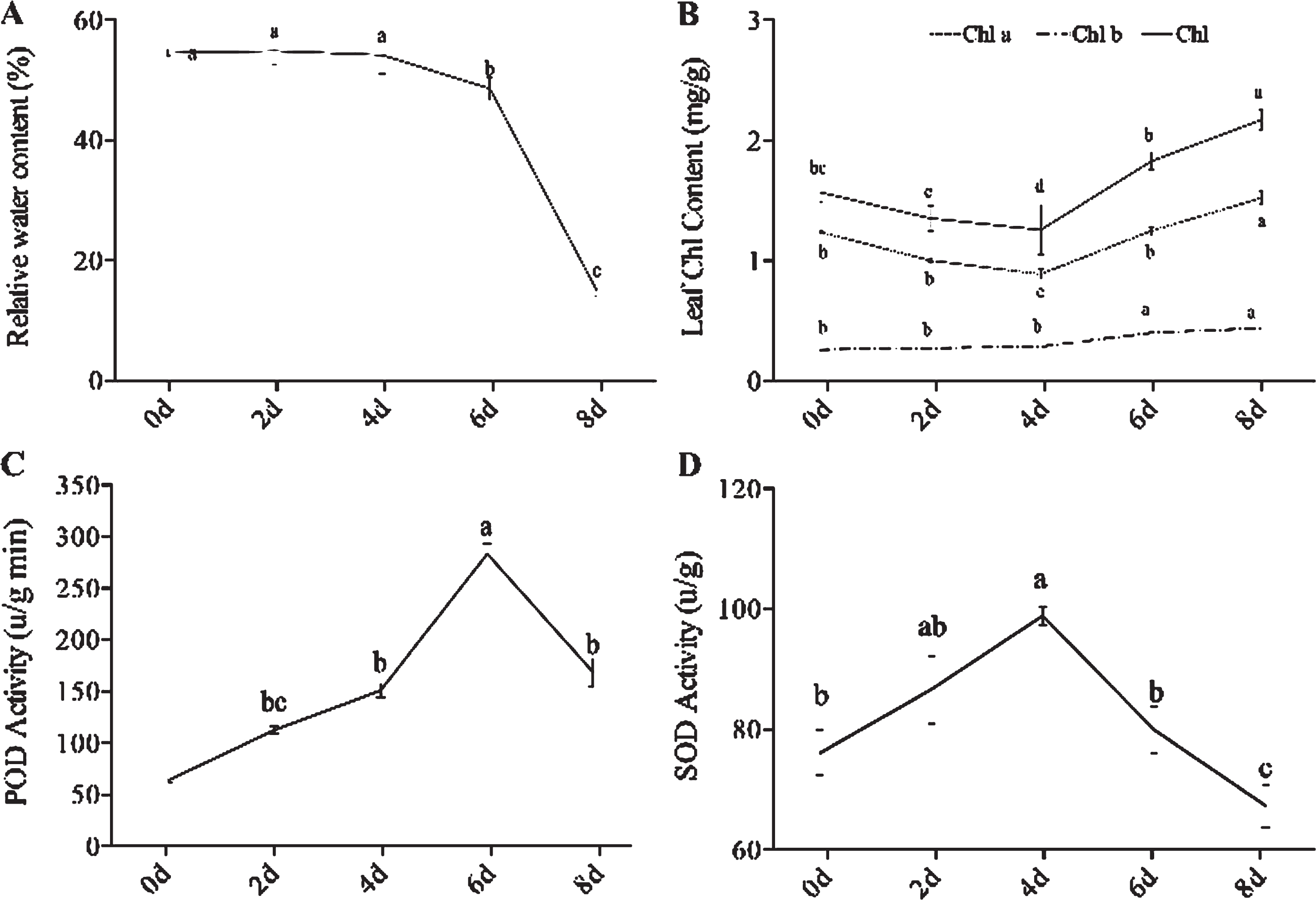
The content of chlorophyll was closely related to the photosynthesis of plants [83]. As Fig. 9B showed the leaf chlorophyll a + b concentration ranged from 1.2 to 2.1 mg/g over the drought treatments. At 4 d drought treatment, the leaf chlorophyll had the lowest content. However, the leaf chlorophyll content increased after 6 d drought treatment. Drought stress damaged the chloroplast structure, the chlorophyll content in grapevine leaf also decreased due to the imbalance of chlorophyll metabolism. With the rapid decrease of the leaf RWC, the chlorophyll content per unit mass of leaf would increase.
Since plants produced crucial antioxidant enzymes in reactive oxygen species (ROS) scavenging under drought stress [84, 85], we measured the activities of two key antioxidant enzymes (POD and SOD) in all drought treatment stages. The two enzymes activity changed obviously over the drought stress exposure. We detected that the POD and SOD activity in grapevine was elevated following drought treatment, while the POD activity peaked at 4 d of 284.7μ/g min following drought stress (Fig. 9C), and the SOD peaked at 6 d following drought stress (Fig. 9D). The plants produced the ROS- scavenging enzymes like POD and SOD during plants exposure to abiotic stress to minimize the cellular injury [86].
4Conclusions
By searching the whole grapevine genome sequence, totally six ACBP genes were identified. Expression characteristics of different VvACBPs resembled those of their homologous genes in Arabidopsis or other plant species. Syntenic and phylogenetic analysis helped to refine the resolution of the relationship between ACBP family members in various plant species and suggested possible functional roles for the grapevine ACBPs. Expression patterns of different VvACBPs varied either under normal or stress conditions, pointing to possible isoform sub-functionalisation. The biochemical and physiological data highlight a good relation among the stress conditions and changes in expression of VvACBPs. When the stress conditions became more severe, in fact, a different trend in expression of the isoforms occurs, so giving a first indication on their possible role in the response to water stress. Given the importance of this gene family in plants, present results may ameliorate further research to better understand the molecular mechanisms of grapevine response to environmental constraints, like various types of abiotic or biotic stress, and to ascribe roles of ACBP machinery in grapevine development and morphological evolution. Future work will focus on functional analysis of the corresponding proteins.
Conflicts of interest
The authors have no conflict of interest to report.
Acknowledgments and Funding
This work was supported by the open funds of the Key Laboratory of Viticulture and Enology, Ministry of Agriculture (KLVE2017.4), China, partly supported by the Earmarked Fund for Modern Agro-industry Technology Research System (CARS-29-yz-5) and the Fund Program for the Scientific Activities of Selected Returned Overseas Professionals in Shanxi Province (zyzz2019001).
Supplementary material
[1] The supplementary material is available in the electronic version of this article: https://dx.doi.org/10.3233/JBR-200528.
References
[1] | Liu F , Zhang X , Lu C , Zeng X , Li Y , Fu D , Wu G . Non-specific lipid transfer proteins in plants: presenting new advances and an integrated functional analysis. Journal of Experimental Botany. 2015:erv313. |
[2] | Kader J-C . Lipid-transfer proteins in plants. Annual Review of Plant Biology. (1996) ;47: (1):627–54. |
[3] | Leung K-C , Li H-Y , Mishra G , Chye M-L . ACBP4 and ACBP5, novel Arabidopsis acyl-CoA-binding proteins with kelch motifs that bind oleoyl-CoA. Plant Molecular Biology. (2005) ;55: (2):297–309. |
[4] | Xiao S , Chye M-L . An Arabidopsis family of six acyl-CoA-binding proteins has three cytosolic members. Plant Physiology and Biochemistry. (2009) ;47: (6):479–84. |
[5] | Rasmussen JT , Rosendal J , Knudsen J . Interaction of acyl-CoA binding protein (ACBP) on processes for which acyl-CoA is a substrate, product or inhibitor. Biochemical Journal. (1993) ;292: (3):907–13. |
[6] | Chye M-L . Arabidopsis cDNA encoding a membrane-associated protein with an acyl-CoA binding domain. Plant Molecular Biology. (1998) ;38: (5):827–38. |
[7] | Chye M-L , Li H-Y , Yung M-H . Single amino acid substitutions at the acyl-CoA-binding domain interrupt 14 [C] palmitoyl-CoA binding of ACBP2, an Arabidopsis acyl-CoA-binding protein with ankyrin repeats. Plant Molecular Biology. (2000) ;44: (6):711–21. |
[8] | Knudsen J , Neergaard TB , Gaigg B , Jensen MV , Hansen JK . Role of acyl-CoA binding protein in acyl-CoA metabolism and acyl-CoA– mediated cell signaling. The Journal of Nutrition. (2000) ;130: (2):294S–8S. |
[9] | Leung K-C , Li H-Y , Xiao S , Tse M-H , Chye M-L . Arabidopsis ACBP3 is an extracellularly targeted acyl-CoA-binding protein. Planta. (2006) ;223: (5):871–81. |
[10] | Burton M , Rose TM , Færgeman NJ , Knudsen J . Evolution of the acyl-CoA binding protein (ACBP). Biochemical Journal. (2005) ;392: (2):299–307. |
[11] | Xiao S , Chye M-L . New roles for acyl-CoA-binding proteins (ACBPs) in plant development, stress responses and lipid metabolism. Progress in Lipid Research. (2011) ;50: (2):141–51. |
[12] | Færgeman NJ , Wadum M , Feddersen S , Burton M , Kragelund BB , Knudsen J . Acyl-CoA binding proteins; structural and functional conservation over 2000 MYA. Molecular and Cellular Biochemistry. (2007) ;299: (1-2):55–65. |
[13] | Hills MJ , Dann R , Lydiate D , Sharpe A . Molecular cloning of a cDNA fromBrassica napus L. for a homologue of acyl-CoA-binding protein. Plant Molecular Biology. (1994) ;25: (5):917–20. |
[14] | Engeseth NJ , Pacovsky RS , Newman T , Ohlrogge JB . Characterization of an Acyl-CoA-Binding Protein from Arabidopsis thaliana. Archives of Biochemistry and Biophysics. (1996) ;331: (1):55–62. |
[15] | Chen Q-F , Xiao S , Chye M-L . Overexpression of the Arabidopsis 10-kilodalton acyl-coenzyme A-binding protein ACBP6 enhances freezing tolerance. Plant Physiology. (2008) ;148: (1):304–15. |
[16] | Xiao S , Gao W , Chen QF , Ramalingam S , Chye ML . Overexpression of membrane-associated acyl-CoA-binding protein ACBP1 enhances lead tolerance in Arabidopsis. The Plant Journal. (2008) ;54: (1):141–51. |
[17] | Yurchenko OP , Nykiforuk CL , Moloney MM , Ståhl U , Banaś A , Stymne S , Weselake RJ : A 10-kDa acyl-CoA-binding protein (ACBP) from Brassica napus enhances acyl exchange between acyl-CoA and phosphatidylcholine. Plant Biotechnology Journal. (2009) ;7: (7):602–10. |
[18] | Chye ML , Huang BQ , Zee SY . Isolation of a gene encoding Arabidopsis membrane-associated acyl-CoA binding protein and immunolocalization of its gene product. The Plant Journal. (1999) ;18: (2):205–14. |
[19] | Li H-Y , Chye M-L . Membrane localization of Arabidopsis acyl-CoA binding protein ACBP2. Plant Molecular Biology. (2003) ;51: (4):483–92. |
[20] | Gao W , Xiao S , Li HY , Tsao SW , Chye ML . Arabidopsis thaliana acyl-CoA-binding protein ACBP2 interacts with heavy-metal-binding farnesylated protein AtFP6. New Phytologist. (2009) ;181: (1):89–102. |
[21] | Chen QF , Xiao S , Qi W , Mishra G , Ma J , Wang M , Chye ML . The Arabidopsis acbp1acbp2 double mutant lacking acyl-CoA-binding proteins ACBP1 and ACBP2 is embryo lethal. New Phytologist. (2010) ;186: (4):843–55. |
[22] | Xiao S , Gao W , Chen Q-F , Chan S-W , Zheng S-X , Ma J , Wang M , Welti R , Chye M-L . Overexpression of Arabidopsis acyl-CoA binding protein ACBP3 promotes starvation-induced and age-dependent leaf senescence. The Plant Cell. (2010) ;22: (5):1463–82. |
[23] | Meng W , Su YC , Saunders RM , Chye ML . The rice acyl-CoA-binding protein gene family: phylogeny, expression and functional analysis. New Phytologist. (2011) ;189: (4):1170–84. |
[24] | Xiao S , Li H-Y , Zhang J-P , Chan S-W , Chye M-L . Arabidopsis acyl-CoA-binding proteins ACBP4 and ACBP5 are subcellularly localized to the cytosol and ACBP4 depletion affects membrane lipid composition. Plant Molecular Biology. (2008) ;68: (6):571–83. |
[25] | Jaillon O , Aury J-M , Noel B , Policriti A , Clepet C , Casagrande A , Choisne N , Aubourg S , Vitulo N , Jubin C . The grapevine genome sequence suggests ancestral hexaploidization in major angiosperm phyla. Nature. (2007) ;449: (7161):463–7. |
[26] | Finn RD , Coggill P , Eberhardt RY , Eddy SR , Mistry J , Mitchell AL , Potter SC , Punta M , Qureshi M , Sangrador-Vegas A . The Pfam protein families database: towards a more sustainable future. Nucleic Acids Research. (2016) ;44: (D1):D279–D85. |
[27] | Finn RD , Clements J , Eddy SR . HMMERweb server: interactive sequence similarity searching.NucleicAcids Research. 2011:gkr367. |
[28] | Letunic I , Doerks T , Bork P . SMART: recent updates, new developments and status in 2015. Nucleic Acids Research. (2015) ;43: (D1):D257–D60. |
[29] | Gasteiger E , Gattiker A , Hoogland C , Ivanyi I , Appel RD , Bairoch A . ExPASy: the proteomics server for in-depth protein knowledge and analysis. Nucleic Acids Research. (2003) ;31: (13):3784–8. |
[30] | Larkin MA , Blackshields G , Brown N , Chenna R , McGettigan PA , McWilliam H , Valentin F , Wallace IM , Wilm A , Lopez R . Clustal W and Clustal X version 2.0. Bioinformatics. (2007) ;23: (21):2947–8. |
[31] | Tamura K , Stecher G , Peterson D , Filipski A , Kumar S . MEGA molecular evolutionary genetics analysis version 6.0. Molecular Biology and Evolution. (2013) ;30: (12):2725–9. |
[32] | Crooks GE , Hon G , Chandonia J-M , Brenner SE . Web Logo: a sequence logo generator. Genome Research. (2004) ;14: (6):1188–90. |
[33] | Rambaldi D , Ciccarelli FD . Fancy Gene: dynamic visualization of gene structures and protein domain architectures on genomic loci. Bioinformatics. (2009) ;25: (17):2281–2. |
[34] | Krzywinski M , Schein J , Birol I , Connors J , Gascoyne R , Horsman D , Jones SJ , Marra MA . Circos: an information aesthetic for comparative genomics. Genome Research. (2009) ;19: (9):1639–45. |
[35] | Coito JL , Rocheta M , Carvalho L , Amâncio S . Microarray-based uncovering reference genes for quantitative real time PCR in grapevine under abiotic stress. BMC Research Notes. (2012) ;5: (1):220. |
[36] | Dash S , Van Hemert J , Hong L , Wise RP , Dickerson JA . PLEXdb: gene expression resources for plants and plant pathogens. Nucleic Acids Research. (2012) ;40: (D1):D1194–D201. |
[37] | Fung RW , Gonzalo M , Fekete C , Kovacs LG , He Y , Marsh E , McIntyre LM , Schachtman DP , Qiu W . Powdery mildew induces defense-oriented reprogramming of the transcriptome in a susceptible but not in a resistant grapevine. Plant Physiology. (2008) ;146: (1):236–49. |
[38] | Carvalho LC , Vilela BJ , Mullineaux PM , Amâncio S . Comparative transcriptomic profiling of Vitis vinifera under high light using a custom-made array and the Affymetrix GeneChip. Molecular Plant. (2011) ;4: (6):1038–51. |
[39] | Fasoli M , Dal Santo S , Zenoni S , Tornielli GB , Farina L , Zamboni A , Porceddu A , Venturini L , Bicego M , Murino V . The grapevine expression atlas reveals a deep transcriptome shift driving the entire plant into a maturation program. The Plant Cell. (2012) ;24: (9):3489–505. |
[40] | Howe E , Holton K , Nair S , Schlauch D , Sinha R , Quackenbush J . Mev: multiexperiment viewer. In: Biomedical Informatics for Cancer Research. Springer; 2010;267-77. |
[41] | Zhu K , Wang X , Liu J , Tang J , Cheng Q , Chen J-G , Cheng Z-MM . The grapevine kinome: annotation, classification and expression patterns in developmental processes and stress responses. Horticulture Research. (2018) ;5: (1):19. |
[42] | Sade N , Galkin E , Moshelion M . Measuring Arabidopsis, Tomato and Barley Leaf Relative Water Content (RWC). Bio-Protocol. (2015) ;5: (8):e1451. |
[43] | Zhang Y , Chen JM , Thomas SC . Retrieving seasonal variation in chlorophyll content of overstory and understory sugar maple leaves from leaf-level hyperspectral data. Canadian Journal of Remote Sensing. (2007) ;33: (5):406–15. |
[44] | Guo T , Zhang G , Zhou M , Wu F , Chen J . Effects of aluminum and cadmium toxicity on growth and antioxidant enzyme activities of two barley genotypes with different Al resistance. Plant and Soil. (2004) ;258: (1):241–8. |
[45] | Dunn P . SPSS survival manual: a step by step guide to data analysis using IBM SPSS. Australian & New Zealand Journal of Public Health. (2013) ;37: (6):597–8. |
[46] | Du Z-Y , Arias T , Meng W , Chye M-L . Plant acyl-CoA-binding proteins: An emerging family involved in plant development and stress responses. Progress in Lipid Research. (2016) ;3: :165–81. |
[47] | Graham IA . Seed storage oil mobilization. Annu Rev Plant Biol. (2008) ;59: :115–42. |
[48] | Herman EM , Larkins BA . Protein storage bodies and vacuoles. The Plant Cell. (1999) ;11: (4):601–13. |
[49] | Hayashi Y , Yamada K , Shimada T , Matsushima R , Nishizawa N , Nishimura M , Hara-Nishimura I . A proteinase-storing body that prepares for cell death or stresses in the epidermal cells of Arabidopsis. Plant and Cell Physiology. (2001) ;42: (9):894–9. |
[50] | Yamada K , Hara-Nishimura I , Nishimura M . Unique defense strategy by the endoplasmic reticulum body in plants. Plant and Cell Physiology. (2011) ;52: (12):2039–49. |
[51] | Li H-Y Chye M-L . Arabidopsis acyl-CoA-binding protein ACBP2 interacts with an ethylene-responsive element-binding protein, AtEBP, via its ankyrin repeats. Plant Molecular Biology. (2004) ;54: (2):233–43. |
[52] | Gao W , Li HY , Xiao S , Chye ML . Acyl-CoA-binding protein 2 binds lysophospholipase 2 and lysoPC to promote tolerance to cadmium-induced oxidative stress in transgenic Arabidopsis. The Plant Journal. (2010) ;62: (6):989–1003. |
[53] | Li H-Y , Xiao S , Chye M-L . Ethylene-and pathogen-inducible Arabidopsis acyl-CoA-binding protein 4 interacts with an ethylene-responsive element binding protein. Journal of Experimental Botany. (2008) ;59: (14):3997–4006. |
[54] | Roy SW , Penny D . Patterns of intron loss and gain in plants: intron loss– dominated evolution and genome-wide comparison of O. sativa and A. thaliana. Molecular Biology and Evolution. (2007) ;24: (1):171–81. |
[55] | Du ZY , Chen MX , Chen QF , Xiao S , Chye ML . Overexpression of Arabidopsis acyl-CoA-binding protein ACBP2 enhances drought tolerance. Plant, Cell & Environment. (2013) ;36: (2):300–14. |
[56] | Du ZY , Chen MX , Chen QF , Gu JD , Chye ML . Expression of Arabidopsis acyl-CoA-binding proteins AtACBP1 and AtACBP4 confers Pb (II) accumulation in Brassica juncea roots. Plant, Cell & Environment. (2015) ;38: (1):101–17. |
[57] | Michaely P , Bennett V . The ANK repeat: a ubiquitous motif involved in macromolecular recognition. Trends in Cell Biology. (1992) ;2: (5):127–9. |
[58] | Adams J , Kelso R , Cooley L . The kelch repeat superfamily of proteins: propellers of cell function. Trends in Cell Biology. (2000) ;10: (1):17–24. |
[59] | Xia Y , Yu K , Gao Q-m , Wilson EV , Navarre D , Kachroo P , Kachroo A . Acyl CoA binding proteins are required for cuticle formation and plant responses to microbes. Lipid Signaling in Plants. 2012;175. |
[60] | Yamaguchi-Shinozaki K , Shinozaki K . Organization of cis-acting regulatory elements in osmotic-and cold-stress-responsive promoters. Trends in Plant Science. (2005) ;10: (2):88–94. |
[61] | Xiang Y , Huang Y , Xiong L . Characterization of stress-responsive CIPK genes in rice for stress tolerance improvement. Plant Physiology. (2007) ;144: (3):1416–28. |
[62] | Kim J-S , Mizoi J , Yoshida T , Fujita Y , Nakajima J , Ohori T , Todaka D , Nakashima K , Hirayama T , Shinozaki K . An ABRE promoter sequence is involved in osmotic stress-responsive expression of the DREB2A gene, which encodes a transcription factor regulating drought-inducible genes in Arabidopsis. Plant and Cell Physiology. (2011) ;52: (12):2136–46. |
[63] | Hernandez-Garcia CM , Finer JJ . Identification and validation of promoters and cis-acting regulatory elements. Plant Science. (2014) ;217: :109–19. |
[64] | Hsiao A-S , Yeung EC , Ye Z-W , Chye M-L . The Arabidopsis cytosolic acyl-CoA-binding proteins play combinatory roles in pollen development. Plant and Cell Physiology. (2015) ;56: (2):322–33. |
[65] | Hsiao A-S , Haslam RP , Michaelson LV , Liao P , Chen Q-F , Sooriyaarachchi S , Mowbray SL , Napier JA , Tanner JA , Chye M-L . Arabidopsis cytosolic acyl-CoA-binding proteins ACBP4, ACBP5 and ACBP6 have overlapping but distinct roles in seed development. Bioscience Reports. (2014) ;34: (6):e00165. |
[66] | Du ZY , Chen MX , Chen QF , Xiao S , Chye ML . Arabidopsis acyl-CoA-binding protein ACBP1 participates in the regulation of seed germination and seedling development. The Plant Journal. (2013) ;74: (2):294–309. |
[67] | Zimmermann P , Hirsch-Hoffmann M , Hennig L , Gruissem W . GENEVESTIGATOR. Arabidopsis microarray database and analysis toolbox. Plant Physiology. (2004) ;136: (1):2621–32. |
[68] | Xue Y , Xiao S , Kim J , Lung S-C , Chen L , Tanner JA , Suh MC , Chye M-L . Arabidopsis membrane-associated acyl-CoA-binding protein ACBP1 is involved in stem cuticle formation. Journal of Experimental Botany. 2014:eru304. |
[69] | Martell EA . Radioactivity of tobacco trichomes and insoluble cigarette smoke particles. Nature. (1974) ;249: (5454):214–17. |
[70] | Lei M , Chen T-B , Huang Z-C , Wang Y-D , Huang Y-Y . Simultaneous compartmentalization of lead and arsenic in co-hyperaccumulator Viola principis H. de Boiss.: An application of SRXRF microprobe. Chemosphere. (2008) ;72: (10):1491–6. |
[71] | Zheng S-X , Xiao S , Chye M-L . The gene encoding Arabidopsis acyl-CoA-binding protein 3 is pathogen inducible and subject to circadian regulation. Journal of Experimental Botany. 2012:ers009. |
[72] | Guelette BS , Benning UF , Hoffmann-Benning S . Identification of lipids and lipid-binding proteins in phloem exudates from Arabidopsis thaliana. Journal of Experimental Botany. (2012) ;63: (10):3603–16. |
[73] | Benning UF , BanitaTamot BSG , Hoffmann-Benning S: New aspects of phloem-mediated long-distance lipid signaling in plants. Lipid Signaling in Plants. 2012;215. |
[74] | Xie LJ , Yu LJ , Chen QF , Wang FZ , Huang L , Xia FN , Zhu TR , Wu JX , Yin J , Liao B . Arabidopsis acyl-CoA-binding protein ACBP3 participates in plant response to hypoxia by modulating very-long-chain fatty acid metabolism. The Plant Journal. (2015) ;81: (1):53–67. |
[75] | Takato H , Shimidzu M , Ashizawa Y , Takei H , Suzuki S . An acyl-CoA-binding protein from grape that is induced through ER stress confers morphological changes and disease resistance in Arabidopsis. Journal of Plant Physiology. (2013) ;170: (6):591–600. |
[76] | Xiao S , Chen Q-F , Chye M-L . Light-regulated Arabidopsis ACBP4 and ACBP5 encode cytosolic acyl-CoA-binding proteins that bind phosphatidylcholine and oleoyl-CoA ester. Plant Physiology and Biochemistry. (2009) ;47: (10):926–33. |
[77] | Cramer GR , Ergül A , Grimplet J , Tillett RL , Tattersall EA , Bohlman MC , Vincent D , Sonderegger J , Evans J , Osborne C . Water and salinity stress in grapevines: early and late changes in transcript and metabolite profiles. Functional & Integrative Genomics. (2007) ;7: (2):111–34. |
[78] | Grimplet J , Deluc LG , Tillett RL , Wheatley MD , Schlauch KA , Cramer GR , Cushman JC . Tissue-specific mRNA expression profiling in grape berry tissues. BMC Genomics. (2007) ;8: (1):187. |
[79] | Du Z-Y , Xiao S , Chen Q-F , Chye M-L . Depletion of the membrane-associated acyl-coenzyme A-binding protein ACBP1 enhances the ability of cold acclimation in Arabidopsis. Plant Physiology. (2010) ;152: (3):1585–97. |
[80] | Liao P , Chen Q-F , Chye M-L . Transgenic Arabidopsis flowers overexpressing acyl-CoA-binding protein ACBP6 are freezing tolerant. Plant and Cell Physiology. 2014:pcu037. |
[81] | Xiao S , Chye M-L . Overexpression of Arabidopsis ACBP3 enhances NPR1-dependent plant resistance to Pseudomonas syringe pv tomato DC3000. Plant Physiology. (2011) ;156: (4):2069–81. |
[82] | Smith C . Tansley Review No. 86 Accumulation of phytoalexins: defence mechanism and stimulus response system. New Phytologist. (1996) ;132: (1):1–45. |
[83] | Agusti S , Enriquez S , Frostchristensen H , Sandjensen K , Duarte CM . Light harvesting among photosynthetic organisms. Functional Ecology. (1994) ;8: (2):273–9. |
[84] | Gill SS , Tuteja N . Reactive oxygen species and antioxidant machinery in abiotic stress tolerance in crop plants. Plant Physiology and Biochemistry. (2010) ;48: (12):909–30. |
[85] | Choudhury FK , Rivero RM , Blumwald E , Mittler R . Reactive oxygen species, abiotic stress and stress combination. Plant Journal. (2017) ;90: (5):856–67. |
[86] | You J , Chan Z . ROS Regulation During Abiotic Stress Responses in Crop Plants. Frontiers in Plant Science. (2015) ;6: :1092–1092. |