GFP transient expression and silencing in Fragaria x ananassa
Abstract
BACKGROUND:
Stable transformation, transient expression, and post-transcriptional gene silencing (PTGS) are powerful methodologies that allow exploration of gene function.
OBJECTIVE:
We aimed to apply these methodologies to strawberry leaves. Methods: the binary vectors pBIN19-sgfp, pBICdsGFP and pBIN61-P19 were transferred into A. tumefaciens EHA105 supervirulent strain by electroporation. The sgfp gene silencing was carried out in stably transformed GFP (green fluorescent protein) F. x ananassa Duch. cultivar ‘Pájaro’ strawberry plants by agroinfiltration. GFP-fluorescence was observed using a stereomicroscope (507 nm).
RESULTS:
We attained a GFP transgenic F. x ananassa plant that expresses the functional protein in all the tissues during a complete and normal life cycle. In planta sgfp transient expression and silencing have also been achieved in F. x ananassa cv. ‘Pájaro’ leaves of wild type and GFP transgenic plants, respectively. Agrobacterium-mediated transient expression was visualized as high intensity green fluorescent spots as early as 7 days post-agroinfiltration (dpa), peaking between 10 and 14 dpa and persisting as long as 24 dpa. A knockdown GFP phenotype was achieved by silencing using a dsGFP hairpin.
CONCLUSION:
This work contributes significantly to the reverse genetics field in strawberry, might help to gain knowledge in the analysis of functional promoters and thereby allow protein expression and silencing of genes. This will help to develop resistant plants expressing plant defense elicitors or silencing pathogen receptors and/or negative regulators of plant defense.
1Introduction
Reverse genetics is a powerful methodology that allows exploration of gene function through modifying a known gene sequence or expression to further analyse its phenotypic effects. For this purpose several approaches have been developed in plants, including stable and transient transformation, mutagenesis, and silencing [1]. Agrobacterium-mediated transformation is the most common methodology for delivering genes of interest in plants [2]. The green fluorescent protein (GFP) of the jellyfish Aequorea victoria has been used as a universal reporter in a broad range of biological systems [3]. However, the use of GFP in higher plants has been limited by aberrant splicing of the corresponding mRNA and by protein insolubility. Therefore, a new synthetic GFP gene sequence (sgfp) with optimal human codons in plant cells was attained, resulting in a more soluble version of the protein GFP [4]. Moreover, a site-directed mutagenesis in the chromophore (the replacement of the serine position 65 with a threonine -S65T-) was also introduced into sgfp to create sgfp(S65T), resulting in enhanced brightness, faster chromophore formation and slower photobleaching [4]. The sgfp(S65T) gene, hereafter in the study referred to as sgfp, became a very useful marker gene for establishing efficient transformation protocols and monitoring promoter or protein functionality in plants. In general, the level of sgfp expression peaks between 2 and 3 days post-agroinfiltration (dpa), and declines rapidly thereafter due to an intrinsic post-transcriptional gene silencing process (PTGS), as described in leaves of Arabidopsis [5], tobacco [6] and potato [7], or as for lettuce and tomato fruits [5], and even strawberry fruits [8].
PTGS is an interesting strategy that has been successfully used in plants for studying gene silencing. One approach is based in the introduction of plasmid constructs encoding a double-stranded RNA (dsRNA) homologous in sequence to the gene of interest [9]. As a result, the target gene transcripts are degraded leading to a knockout or knockdown phenotype [10]. During the evolutionary race between plants and viruses, the latter have evolved proteins that suppress PTGS, thereby allowing plant infection [11–13]. Among them, the protein P19 encoded by the tomato bushy stunt virus (TBSV) is a very effective suppressor that lets the virus evade the host silencing response restoring high levels of the transiently expressed protein [14, 15, 16].
Strawberry, including the cultivated octoploid Fragaria x ananassa Duch. and the wild diploid F. vesca, is considered a plant model system for genetic and molecular studies in the Rosaceae family due to its numerous advantages, such as the availability of the complete genome sequences [17–20]. Particularly for F. x ananassa fruits, reverse genetics has been successfully applied to investigate the function of a target gene using stable transformation, transient expression, and PTGS. New protocols were adjusted using mainly GUS as the marker gene rather than GFP, and beneficial traits such as disease resistance and fruit quality have been improved [8, 21–28]. However, there are few studies on transient expression and silencing in strawberry leaves, which are an interesting tissue to apply reverse genetics techniques using genes encoding pathogen effectors and defense-inducing elicitors. In fact, there is only one study reporting transient expression of yellow fluorescent protein (YFP) in detached leaves of F. vesca [29] and another about virus-induced gene silencing (VIGS) of a F. x ananassa cultivar ‘Benihoppe’ phytoene desaturase (PDS) gene [30]. Therefore, the objectives of the present study were to develop 1) a stable F. x ananassa cv. ‘Pájaro’ GFP transgenic line, 2) an Agrobacterium-mediated methodology for transient expression of sgfp in strawberry leaves, and 3) a PTGS-based tool using a dsGFP hairpin.
2Materials and methods
2.1Plant material
Strawberry plants of F. x ananassa Duch. cv. ‘Pájaro’ were micropropagated at the Strawberry BGA (Banco de Germoplasma Activo) at Universidad Nacional de Tucumán, Argentina. Strawberry plants that were not stably transformed and thus maintain its wild genetic background are hereafter referred to as wild type (WT) plants. Briefly, runner meristems obtained from healthy strawberry WT plants were implanted into initiation medium (IM) containing macroelements according to the N30K formulation [31], microelements and vitamins (Neolab, Argentina) according to MS basal medium [32], 100 mg/L myoinositol, 3% (m/v) sucrose, hormones (Neolab, Argentina): 1 mg/L butyric indol acid (IBA), 0.1 mg/L benzylaminopurine (BAP) and 0.1 mg/L gibberellic acid (GA3), and 2.1.1 g/L PhytagelTM (Sigma-Aldrich, St. Louis, MO, USA). After one month, emerging shoots were transferred into multiplication medium (MM), which differed from IM by using 0.3 mg/L BAP as unique hormone, and replacing Phytagel™ with 6 g/L agar (Sigma-Aldrich, St. Louis, MO, USA). They were transplanted into fresh MM every month and the process was repeated a maximum of five times to minimize the possibility of somaclonal variation. For genetic stable transformation, in vitro plant material that was previously transplanted into fresh MM for five times, was transplanted into a modified MM supplemented with 0.5 mg/L kinetin (Neolab, Argentina) instead of BAP, and maintained for one month prior to assays. Etiolated plants were used to investigate whether a chlorophyll masking phenomenon was taking part in GFP stably transformed in vitro plants. For this assay, one-month-old in vitro-grown plants were maintained in rooting medium, prepared with half strong salts and vitamins according to MS, 100 mg/L myoinositol, 4% (m/v) sucrose and 6 g/L agar (Sigma-Aldrich, St. Louis, MO, USA), and then kept in darkness for one month to eliminate chlorophyll. All meristems, explants and plants grown and kept in vitro were incubated in a growth chamber under controlled conditions in a 16 h light cycle (white fluorescent light, 350 μmol/m2.s) and 24°C±2°C.
For transient expression and silencing assays, three-month-old plants grown in soil were used. To obtain these plants, first, in vitro shoots proliferated in MM were transferred into rooting medium, and after one month these plantlets were transplanted into a humus/perlite soil mixture (2/1, m/m) and kept in a growth chamber under controlled conditions in a 16 h light cycle (white fluorescent light, 350 μmol/m2.s) and 24°C±2°C. For the first week these plants were covered with a transparent bag to maintain 100% relative humidity (RH); then were maintained uncovered for another week, and finally were moved to a greenhouse under semi-controlled conditions in a 16 h natural light cycle, 24°C±4°C with 70% RH.
2.2Plasmids and Agrobacterium tumefaciens
The binary vectors pBIN19-sgfp, pBICdsGFP, and pBIN61-P19 were transferred into A. tumefaciens EHA105 supervirulent strain [33] by electroporation following instructions of the manufacturer (MicroPulser Electroporator, BioRad, USA). pBIN19-sgfp expresses the marker gene sgfp which encodes the modified sGFP protein (hereafter referred as GFP) [4] (Online Resource 1A). pBICdsGFP expresses the self-complementary sgfp gene separated by an intron sequence, codifying for a RNA GFP hairpin (dsGFP) [34] (Online Resource 1B). pBIN61-P19 expresses the orf4 gene from tomato bushy stunt virus (TBSV) that encodes the silencing suppressor P19 [15] (Online Resource 1C). Transformed clones were evaluated by restriction site analysis. Plasmid DNA was extracted from three isolated colonies per construct using PureYield Plasmid Miniprep System (Promega, USA) and digested with the following restriction endonucleases: EcoRI and HindIII for pBIN19-sgfp and pBIN61-P19 constructs, or HindIII and SalI for pBICdsGFP (Thermo Fisher Scientific, USA). The fragments were examined by electrophoresis on a 1% (m/v) agarose gel (Biodynamics SRL, Argentina) and stained with ethidium bromide (Thermo Fisher Scientific, USA) according to [35]. Since the expected bands were visualized for pBIN19-sgfp (1.5 kb and 12 kb), pBIN61-P19 (764 bp, 411 bp, 290 bp and 11.7 kb) and pBICdsGFP (666 bp, 2.3 kb and 11.7 kb) it was confirmed the correct plasmid transference.
2.3Plant stable transformation
F. x ananassa stable transformation was carried out with A. tumefaciens strain EHA105 containing the pBIN19-sgfp plasmid, according to [36] with minor modifications. One cm2-leaf disks were excised from ‘Pájaro-WT’ in vitro plants cultured during one month in the modified MM, placed abaxial side up and cultured on shoot regeneration medium (SRM), a basal MS medium supplemented with 3% (m/v) sucrose, 1 mg/L BAP, 0.1 mg/L 2.4-dichlorfenoxiacetic acid (2.4-D) (Neolab, Argentina), and 2.2 g/L Phytagel™ (Sigma-Aldrich, St. Louis, MO, USA), for four days prior to inoculation. The leaf explants were dipped into 25 ml of EHA105(pBIN19-sgfp) bacterial culture for 15 min at room temperature with gently shaking every 2 min. The bacterial culture grown at OD600 = 2 was diluted with liquid MSO medium (MS basal salts without sugar) at the ratio of 1 : 10 LB broth:liquid MSO. Explants were blotted dry on autoclaved filter paper N°1 (Whatman), placed abaxial side up on SRM, and maintained in darkness at 20°C. After two days, the explants were transferred to selection medium (SM) which was similar to SRM but supplemented with 25 mg/L kanamycin (Kn) (Sigma-Aldrich, St. Louis, MO, USA) and 500 mg/L cefotaxim (Cf) (Klonal Laboratorios, Argentina). They were subcultured to fresh SM every seven days for two times and thereafter every fourteen days. In addition, leaf explants without Agrobacterium inoculation were cultured in SRM (regeneration control) or in SM (selection control). Glass Petri dishes (100×25 mm) with 25 ml of all media were used. After four months, transgenic shoots regenerated from each leaf explant were transferred into selective rooting medium (SRM’), similar to the rooting medium described above but containing 25 mg/L Kn. After one month, Kn-resistant rooted plants were transferred into a sterilized humus/perlite soil mixture (2/1, m/m) and maintained in greenhouse. All the explants used in this study were incubated in a growth chamber under controlled conditions as mentioned in 2.1 sub-section. Treatments were repeated five times with ten explants per treatment (n = 50) and replicated three times independently. Each of the two transgenic lines attained were vegetatively multiplied to provide sufficient plant material for the studies.
2.4Transient expression assays
A. tumefaciens strains EHA105 containing the pBIN19-sgfp, pBICdsGFP and pBIN61-P19 plasmids were agroinfiltrated in ‘Pájaro-WT’ leaves according to an adapted protocol from [27]. Briefly, bacteria were grown in 50 ml LB containing 5 g/L sucrose, 2 mM MgSO4 and 10 mM morpholine ethanesulphonic acid (MES, pH 5.6) (Sigma-Aldrich, St. Louis, MO, USA), 25 mg/L nalidixic acid, 50 mg/L Kn and 20 μmol/L acetosyringone (Neolab, Argentina), at 28°C and 135 rpm. When the cultures reached OD600 = 1 were centrifuged (5.000 rpm, 4°C) and pellets resuspended in the infiltration solution (IS), a MS modified medium with MS salts, 10 mM MES, 20 g/L sucrose, 200 μmol/L acetosyringone (pH 5.6) and incubated at 20°C with 135 rpm until OD600 = 0.8. 500 μl of any bacterial suspension were carefully infiltrated in the abaxial side of leaves with the help of a 3 ml-syringe without needle. Infiltrated areas (2 mm diameter) were slightly marked with blue ink and excess of bacteria was removed with tissue paper (Online Resource 2). The wild type bacterium EHA105 (without plasmid, denoted as EHA105-WT) and the IS were used as controls. Plants were maintained in a growth chamber under controlled conditions as mentioned in 2.1 sub-section, but at 20°C±2°C.
2.5Silencing and suppression of silencing assays
The sgfp gene silencing was carried out in stably transformed GFP F. x ananassa plants by agroinfiltration according to [37]. With a similar technique as for transient expression assays, leaves were infiltrated with a co-culture of A. tumefaciens EHA105(pBIN19-sgfp) + EHA105(pBICdsGFP), from now on denoted as pBIN19-sgfp + pBICdsGFP, for silencing studies. EHA105-WT and the IS were used as controls. The suppression of intrinsic silencing was studied in WT plants by agroinfiltration of a co-culture of EHA105(pBIN19-sgfp) + EHA105(pBIN61-P19), from now on denoted as pBIN19-sgfp + pBIN61-P19. Plants were maintained in a growth chamber under controlled conditions as mentioned in 2.4 sub-section.
2.6Analysis of GFP expression
GFP was observed using a stereomicroscope (Leica MZ16 F, Leica Microsystems, Germany) equipped with an external lamp set with a 484 nm excitation filter. Detection of green fluorescence (507 nm) was indicative of GFP protein expression. Pictures were taken with a digital camera (COOLPIX 4300, Nikon) and saved as.TIF files for further quantification using ImageJ 1.44 software. Fluorescence values were corrected as follows: for GFP transient expression assays, fluorescence (pBIN19 - sgfp) – basal fluorescence (mean between EHA105 - WT and IS) ; for silencing assays, fluorescence (pBIN19 - sgfp +pBICdsGFP) – basal fluorescence (mean between EHA105 - WT and pBICdsGFP) , and for suppression of silencing assays, fluorescence (pBIN19 - sgfp +pBIN61 - P19) – basal fluorescence (mean between EHA105 - WT and dsGFP) . Means±standard errors (SEs) were calculated from fluorescence corrected values and represented in each graph.
2.7Image processing
Each of the digital images was processed using ImageJ 1.44 software (NIH) (https://imagej.nih.gov/ij/), an open source, Java-written image processing program. Digital image files in.TIF format were open in ImageJ, and image settings were adjusted by selecting IMAGE – TYPE – 16-bit; EDIT – INVERT; PROCESS – SUBSTRACT BACKGROUND where “Rolling ball radius” was set in 50.0 pixels and “light background” was selected. In IMAGE – ADJUST – THRESHOLD “B&W” was set in the right window and the bar was dragged to modify the black coloration as faithfully as the green fluorescence in the original picture. Quantification parameters “Area”, “Area fraction” and “Display label” were set in ANALYSE – SET MEASURMENTS and after selecting MEASURE – ANALYSE a Summary table appeared from which “% Area” values were chosen to present the results. This parameter represents the percentage of black-colored area from the total area of the image, given in pixels.
2.8Statistical analysis
For green fluorescence analysis in transient expression and silencing assays one leaf (including the three leaflets) of three plants was infiltrated at two points on each side of the central vein (n = 36). For suppression of sgfp silencing assays one leaf (including the three leaflets) of one plant was infiltrated at two points on each side of the central vein (n = 12). Fluorescence values in transient expression assays in WT plants (Fig. 2), and in silencing assays in GFP stably transformed plants (Fig. 4) were analyzed via Kruskal-Wallis test (non-parametric data). Fluorescence values in suppression of intrinsic sgfp silencing in WT plants were analyzed via ANOVA followed by LSD-Fisher test (parametric data). In all figures significant differences are indicated with different letters (P≤0.05). Statistical analyses were performed using the InfoStat 2013 software [38].
3Results
3.1F x ananassa stable transformation
In vitro propagated F. x ananassa cv. ‘Pájaro’ plants were used as leaf explant donors for further stable genetic transformation assays. Briefly, plants were micropropagated from apical meristems (Online Resource 3B) extracted from runners (Online Resource 3A), after four weeks emerged shoots (Online Resource 3C), which were transferred to MM where proliferated forming clump shoots (Online Resource 3D). These new shoots were transferred to the modified MM supplemented with kinetin, and after one month served as leaf explant donors for stable transformations assays. Eight out of fifty leaf discs transformed with pBIN19-sgfp induced calluses after three months post Agrobacterium-mediated stable transformation (Online Resource 4A), and only two of them finally regenerated shoots. Shoots formed from each callus were considered as independent transformation events. After transferring shoots to SM supplemented with kn 25 μg/ml, two kn-resistant lines were obtained, representing 4% transformation efficiency. Calluses from leaf explants cultivated in SRM (regeneration control) grew faster than the transformed ones (Online Resource 4B) with 60% of efficiency. Whereas non-transformed leaf discs cultured in SM (negative control) did not develop calluses and died after 4 months (Online Resource 4C).
The expression of GFP protein was analysed in planta during the different developmental stages of both transgenic lines. Only one of them presented strong and uniform green fluorescence in all tissues observed; hence, it was chosen for further analysis and studies (Fig. 1). During calluses formation in the leaf tissue of the selected transgenic line, a homogenous and strong green fluorescence was observed in the entire cellular mass (Fig. 1A). Intense fluorescence was observed in proliferating shoots (Fig. 1B) and roots (Fig. 1C) of in vitro plants, but it almost disappeared in totally expanded leaves (Fig. 1D). Transgenic flowers presented normal aspect with its calyx and sepals, the corolla with five white petals, and numerous stamens inserted at the base of the receptacle (Fig. 1E). Under UV light, green fluorescence was visualized in the petals, receptacle and stamens (Fig. 1F). Zoom view of a fluorescent stamen (Fig. 1G). Likewise, the fleshy receptacle tissue of white (Fig. 1H) and red (Fig. 1I) fruits displayed intense fluorescence. The vegetatively multiplicated T0 progeny of transgenic plants grew normally in greenhouse and produced numerous runners (Fig. 1J). Foliar tissue of these plants displayed green fluorescence partially masked by red fluorescence from chlorophyll (Fig. 1K).
Fig.1
Expression of GFP protein from transformed callus to strawberry fructification. A) A transformed callus expressing GFP protein; bar = 3 mm. B) A fluorescent non-expanded leaf, bar = 3 mm; C) fluorescent root, bar = 1 mm and D) expanded non-fluorescent leaf, bar = 3 mm. Normal flower with its calyx, non-expanded petals, and numerous stamens inserted into the receptacle observed under white (E) and UV light (F); bars = 7 mm. G) A fluorescent stamen observed under UV light; bar = 1 mm. View of a longitudinally-cut white (H) and red (I) fruit observed under UV light, bars = 9 mm. J) Vegetative multiplication of stably transformed GFP strawberry plants in greenhouse. K) Foliar tissue from these plants displayed green fluorescence partially masked by red fluorescence from chlorophyll. One representative image of each developmental stadium was used for illustration.
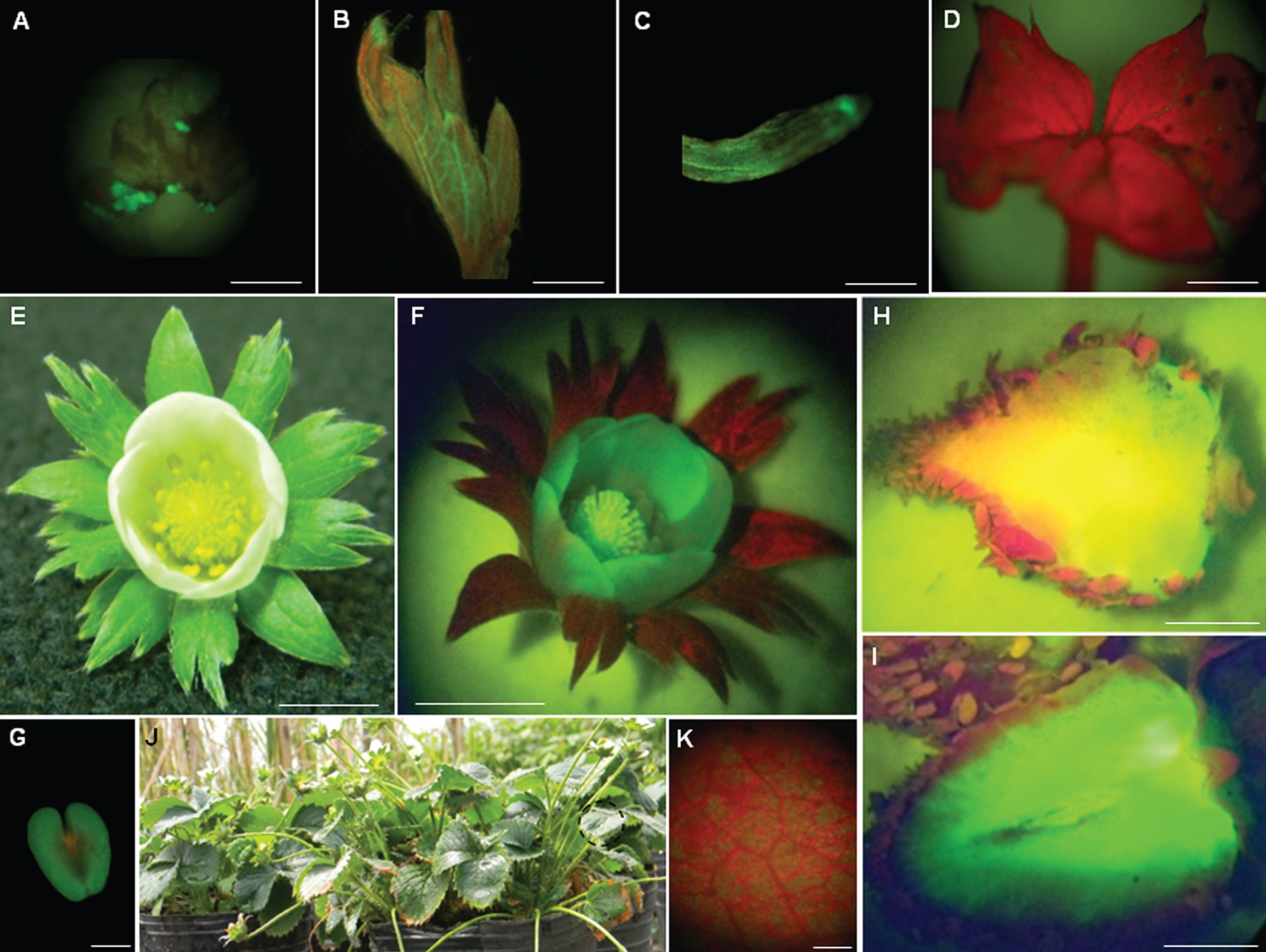
To investigate whether the lack of fluorescence in those totally expanded leaves was due to a masking phenomenon of the red fluorescence emitted by chlorophyll, in vitro-grown plants were kept in darkness until chlorophyll disappeared (Online Resource 5A, C). When they were exposed under UV light, an intense green fluorescence was detected in shoots (Online Resource 5B) and roots (Online Resource 5D). In addition to these observations, the fact that green fluorescence was detected in adult leaves of stably transformed GFP plants grown in soil (Fig. 1K) led us to speculate that a gene silencing process was occurring during plant ontogeny process. This hypothesis was reinforced by the fact that non-fluorescent leaves of in vitro GFP transgenic plants (Online Resource 6A,) could regenerate fluorescent calluses when cultured in SRM (Online Resource 6B, C).
3.2sgfp transient expression in F. x ananassa leaves
In WT plants, green fluorescent spots denoting GFP transient expression were slightly visible for first time 7 days post agroinfiltration (dpa) with pBIN19-sgfp. No damages on foliar tissue were recorded just after agroinfiltration, rejecting any possible initial fluorescence emitted as a mechanical response (Online Resource 2). The level of fluorescence highly increased among 10 and 14 dpa (Fig. 2A). Thereafter, the fluorescence decreased being almost imperceptible 24 dpa. By that time leaves started to get necrotic making difficult further fluorescence observations. On the contrary, no fluorescence was observed after agroinfiltration with the controls EHA105-WT or IS at any time analysed. Evaluation of the green spots allowed estimating quantitatively that the maximum GFP expression level was reached between 10 and 14 dpa (Fig. 2B). From these results we speculated that a silencing mechanism was activated after the peak of sgfp expression which might be the cause of fluorescence decay observed thereafter. To verify this hypothesis, agroinfiltration with the co-culture pBIN19-sgfp+pBIN61-P19 was carried out in WT plants. This procedure caused a significant fluorescence increase in these plants 17 dpa, reaching similar levels as in the transient expressed control pBIN19-sgfp (Fig. 3A, B).
Fig.2
In planta sgfp transient expression in WT F. x ananassa leaves. A) Green fluorescence after agroinfiltration with pBIN19-sgfp at different days post agroinfiltration (DPA). Infiltrations with EHA105-WT or infiltration solution were used as controls. B) Quantification of green fluorescence. Means±SEs are represented from one typical assay (n = 36). Two independent assays were performed with similar results. Analysis of non-parametric variance was performed via Kruskal-Wallis test. Different letters represent statistically significant differences (P≤0.05). Bar = 0.9 mm.
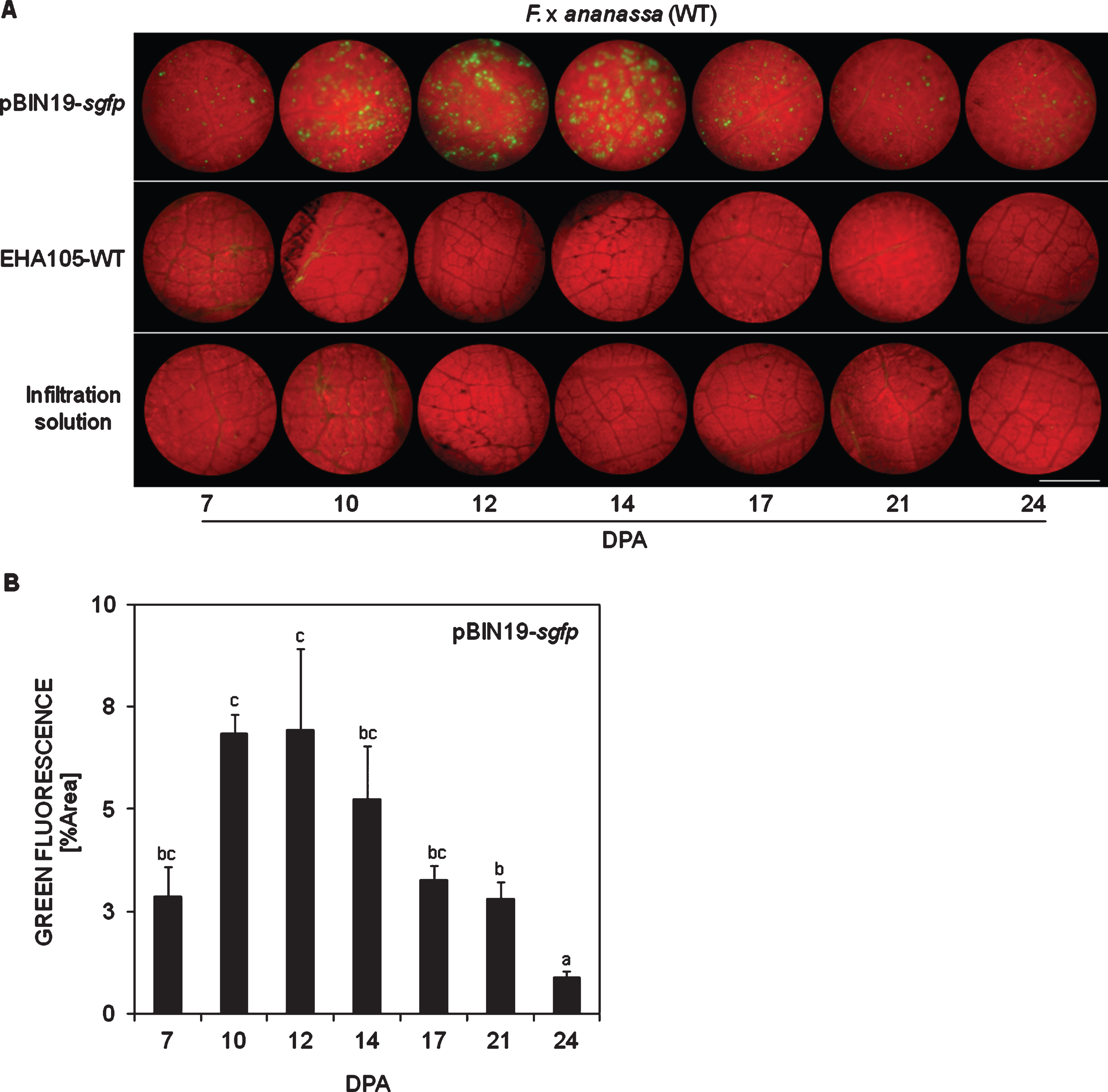
Fig.3
In planta suppression of intrinsic sgfp silencing in WT F. x ananassa leaves. A) Restoring of green fluorescence by the silencing suppressor protein P19 after agroinfiltration with a co-culture of pBIN19-sgfp + pBIN61-P19, compared towards the transient expression positive control pBIN19-sgfp at different days post agroinfiltration (DPA). B) Quantification of green fluorescence. Means±SEs are represented from one assay (n = 12). Analysis of variance (ANOVA) followed by LSD-Fisher test was performed. Different letters represent statistically significant differences (P≤0.10). Bar = 0.9 mm.
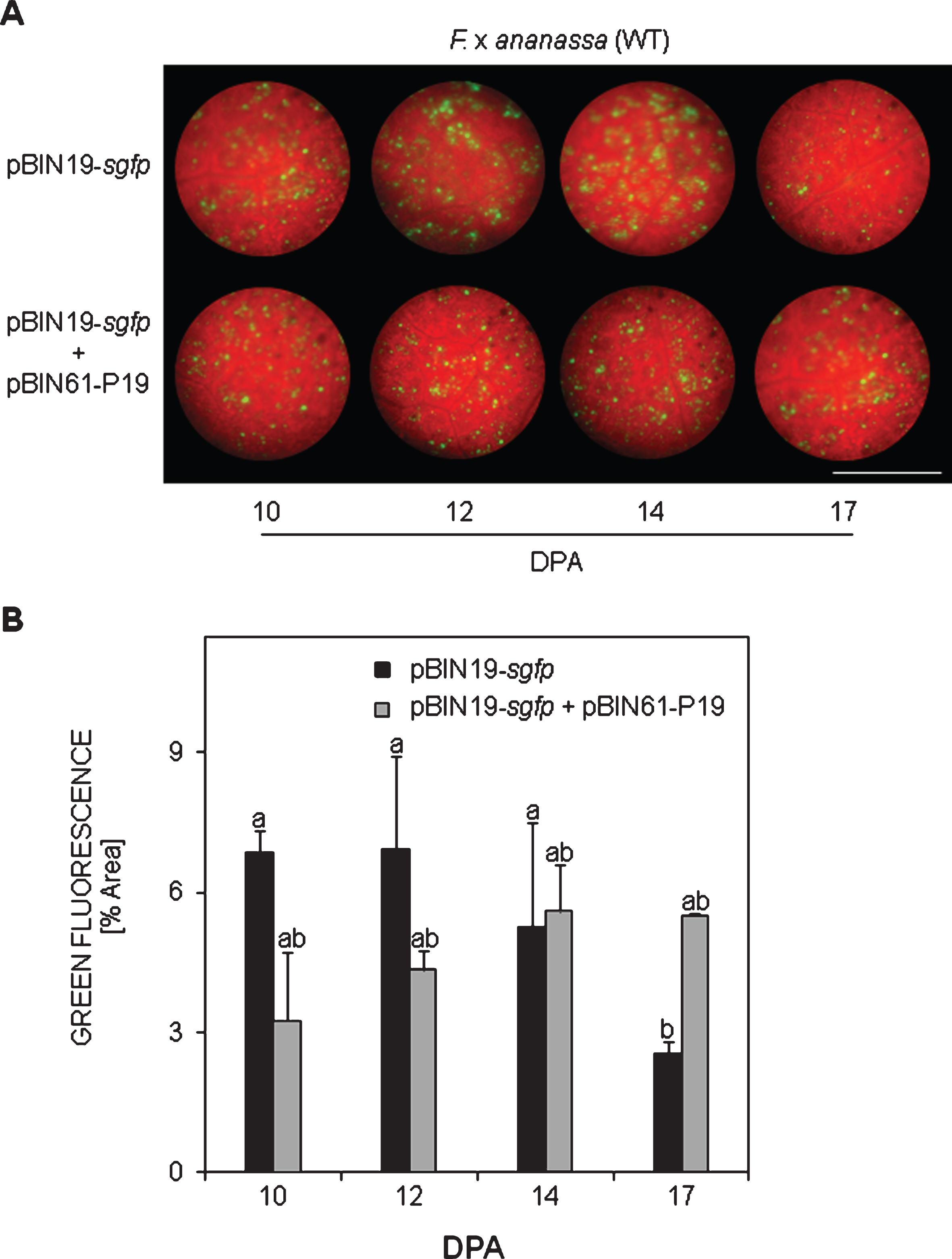
3.3sgfp silencing in transgenic F. x ananassa leaves
With the aim to verify whether the dsGFP hairpin was actually able to silence the sgfp transgene (panels pBICdsGFP and EHA105-WT in Fig. 4A) or not, a similar strategy as in WT plants was carried out but the pBIN19-sgfp construct was co-infiltrated to enhance the fluorescent signal over the green constitutive background. This strategy allowed us to clearly observe the transient expressed GFP fluorescence as brilliant green spots protruding over the green background 10 dpa on, and it was specially observed in veins 12 and 14 dpa (panel pBIN19-sgfp). To confirm whether a silencing mechanism was induced by the hairpin in strawberry leaves, a co-culture of the pBIN19-sgfp + pBICdsGFP was agroinfiltrated. In this case decay in the fluorescence was detected between 12 and 17 dpa (Fig. 4B), mainly in the tissue around veins (see panel pBIN19-sgfp + pBICdsGFP in Fig. 4A), in comparison to the control pBIN19-sgfp.
Fig.4
In planta sgfp silencing in stably transformed GFP F. x ananassa leaves. A) Residual green fluorescence after pBICdsGFP and EHA105-WT (control) agroinfiltrations, and after silencing (pBIN19-sgfp + pBICdsGFP) in comparison to pBIN19-sgfp (control) at different days post agroinfiltration (DPA). B) Quantification of green fluorescence. Means±SEs are represented from one typical assay (n = 36). Two independent assays were performed with similar results. Analysis of non-parametric variance was performed via Kruskal-Wallis test. Different letters represent statistically significant differences (P≤0.05). Bar = 0.9 mm.
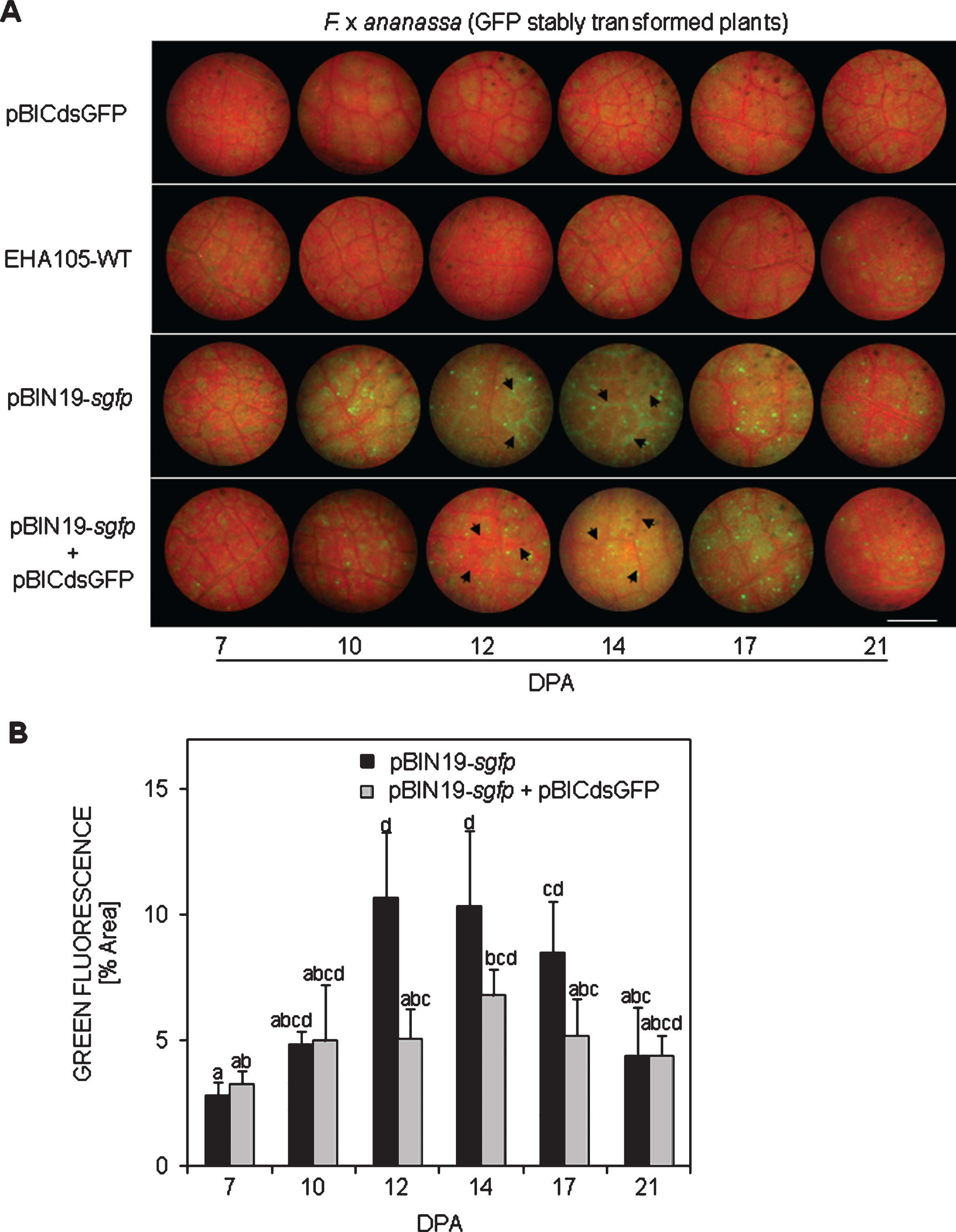
4Discussion
4.1GFP transgenic plant
With the aim to develop in planta transient expression and silencing systems in F. x ananassa cv. ‘Pájaro’ leaves, first a stably transformed GFP strawberry plant was attained. In the present study we report for the first time the generation of a GFP transgenic plant in the octoploid strawberry F. x ananassa cv. ‘Pájaro’ that expresses the protein in all the tissues. Transgenic plants grew normally as denoted by flowers, fruits and runner production. There are few studies reporting stable transformation with sgfp gene in strawberry, three performed in the diploid Fragaria vesca [39, 40, 41], and just one in the octoploid F. x ananassa [42]. An interesting issue observed in this study is that while young and expanding in vitro tissues displayed a strong and homogenous green fluorescence, mature leaves did not, as if chlorophyll masking effect was taking place. Fluorescence disappearance was also reported in transgenic F. vesca plants by [39 and 40], who proposed that green fluorescence could be masked by the intense red fluorescence of chlorophyll, but did not prove it. Here the hypothesis of chlorophyll masking was rapidly discredited since intense green fluorescence was detected in GFP stably transformed in vitro-grown etiolated plants and also in mature leaves of GFP transgenic plants grown in greenhouse. It is widely reported that PTGS is involved in regulating the expression of endogenous and foreign genes along the development of plants through highly specific interactions [43]. According to our observations, it is more likely that a PTGS process occurs during in vitro plant ontogeny of F. x ananassa plants than a chlorophyll masking effect, being active during a specific stage of the in vitro culture and not in undifferentiated cells from regenerating calluses, neither in expanding young tissues nor in mature tissues from plants grown in soil.
4.2sgfp transient expression
In planta sgfp transient expression was observed for first time 7 dpa in WT F. x ananassa leaves. Our finding does not coincide with observations made in numerous plant species in which transient expression of genes in general, and gfp in particular, is detected between 2 and 4 dpa, like reported in leaves of Arabidopsis [5], tobacco [6] and potato [7], or as for lettuce and tomato fruits [5], and even strawberry fruits [8]. The difference in transient expression timing between strawberry fruits and leaves could be due to tissues nature, being earlier and clearly visualized in fleshy and soft strawberry fruits than in foliar tissue. Another possibility is that fluorescent compounds such as polyphenols present in F. x ananassa leaves could interfere with an early low initial green fluorescence. It is noteworthy that the transient expressed GFP protein was observed as bright and diffuse green fluorescent spots in strawberry leaves, rather than a regular green patch as observed in the well-studied tobacco system [6, 44]. The setup of this simple and reproducible protocol allowed long-term and high levels of sgfp expression, and is of great importance since, to our current knowledge, there are no other studies reporting in planta sgfp transient expression in Fragaria x ananassa leaves. Instead, most of the studies available for strawberry are performed in fruits and use GUS despite the drawbacks this marker gene implies [8, 24, 26]. There is only one report about YFP transient expression in leaves of F. vesca but was carried out in detached leaves [27]. The current study might contribute to in vivo continuous monitoring of functional promoters and protein expression in F. x ananassa leaves. For example, defense-inducing elicitors such as the fungal subtilisin-like protein AsES well studied in our laboratory could be transiently expressed in order to evaluate its efficiency to protect against pathogens, similarly as when it is applied exogenously [45, 46, 47]. The results of this study might justify obtaining a pathogen resistant AsES-transgenic strawberry plant.
By the silencing protein suppressor P19 we show that an intrinsic PTGS mechanism could be activated in strawberry leaves and might be the responsible for the rapid decrease of fluorescence after sgfp peak expression 17 dpa. Despite new assays should be carried out to confirm this observation, our preliminary results are in agreement with numerous authors who established that PTGS either contributes to, or is the major cause of the rapid decline in transient expression of genes introduced into Nicotiana benthamiana and A. thaliana [37, 48, 49]. Similarly, PTGS governed the rapid declined of gfp expression in Lima bean (Phaseolus lunatus L. cv. ‘Henderson-Bush’) until undetectable levels 72 hpa [50].
4.3sgpf gene silencing
There are no reports about gene silencing in strawberry leaves. All available studies were carried out in fruits in which genes such as mannose-binding lectin (FaMBL1) involved in unripe and ripe fruit susceptibility to Colletotrichum acutatum [21], glycosyltransferase 1 (FaGT1) involved in anthocyanin synthesis [22], chalcone synthase (CHS) [23], or the transcription factor FcMYB1 [26] have been partially or totally silenced. In the present study no reduction of constitutive fluorescence was detected after the RNA hairpin dsGFP was agroinfiltrated in leaves of GFP stably transformed F. x ananassa plants. Our results disagree with those achieved in N. benthamiana and A. thaliana in which gfp was clearly silenced by the dsGFP hairpin [48, 49]. To confirm that a silencing mechanism was being activated in strawberry leaves we first enhanced the green fluorescence signal by agroinfiltration of pBIN19-sgfp and in this background we evaluated the hairpin capacity to silence the sgfp transgene by monitoring fluorescence decay. This strategy allowed us to detect fluorescence reduction of about 30% and 50% along the time, confirming sgfp silencing. It is likely there is a threshold that determines the levels of sgfp mRNA required for triggering specific degradation of all homologous mRNA in the cytoplasm, as proposed in tobacco by [51]. We can also speculate that there are remaining traduced GFP proteins that are so stable that keep emitting fluorescence although the silencing process might had effectively blocked the transduction of sgfp mRNA. Interestingly, fluorescence decay was observed mainly in the tissue around veins supporting the idea that in strawberry there is a silencing-mediating diffusible signal that moves from the initial infiltration point through veins, as observed in N. benthamiana and A. thaliana [48, 49].
In this study we obtained knockdown GFP phenotypes rather than knockouts; thus, we speculate that the expression of dsGFP hairpin alone is not sufficient to induce a complete silencing of sgfp in stably transformed GFP F. x ananassa leaves. Future studies should be conducted in order to quantify mRNA and siRNA to better understand this phenomenon. It is of great importance to count with an effective silencing method in leaves of the cultivated strawberry because it might be used for silencing genes associated to the synthesis of allergenic compounds, as well as genes related to pathogen receptors [52] and negative regulators of defense genes.
5Conclusion
The present study describes for the first time the attainment of a transgenic F. x ananassa cv. ‘Pájaro’ plant expressing GFP protein in all the tissues during the whole life cycle. In addition, efficient and reproducible systems for the continuous monitoring in planta of sgfp transient expression and silencing are reported for the first time in F. x ananassa leaves. These contributions are of great importance for the reverse genetic field in the Rosaceae family in general and strawberry in particular because they may be further used for the expression and silencing of genes of potential agronomic interest in agronomical important species.
Author contributions
VHC, MEI and RHTG performed the experiments, and collected, analyzed and interpreted the data. VHC, MEI, GRV and JCDR conceived and designed the experiments. VHC and MEI wrote the manuscript. JCDR, APC and VHC revised critically the article. All authors approved the final version of the manuscript.
Conflict of interest
The authors have no conflict of interest to report.
Funding
This work was supported by Agencia Nacional de Promoción Científica y Tecnológica (PICT 2017-0653) and Universidad Nacional de Tucumán (PIUNT 26/D642). VHC was supported by a Postdoctoral Fellow from Consejo Nacional de Investigaciones Científicas y Técnicas (CONICET). MEI and RHTG were supported by a CONICET Doctoral Fellow.
Acknowledgments
We want to thank Dr. Leandro Peña from the Institute of Agricultural Research (IVIA, Spain) for generously providing the A. tumefaciens supervirulent strain EHA105 and pBIN19 construct, and Dr. Sebastián Asurmedi from the Biotechnology Institute (CICVYA-INTA, Argentina) for generously providing the pBICdsGFP and pBIN61-P19 constructs.
Supplementary material
[1] The supplementary material is available in the electronic version of this article: https://dx.doi.org/10.3233/JBR-190447.
References
[1] | Gilchrist E , Haughn G . Reverse genetics techniques: Engineering loss and gain of gene function in plants. Brief Funct. Genomics. (2010) ;9: :103–110. |
[2] | Gelvin SB . Agrobacterium-mediated plant transformation: The biology behind the “gene-jockeying” tool. Microbiol. Mol. Biol. Rev. (2003) ;67: :16–37. |
[3] | Heim R , Cubitt AB , Tsien R . Improved green fluorescence. Nature. (1995) ;373: :663–664. |
[4] | Chiu W , Niwa Y , Zeng W , Hirano T , Kobayashi H , Sheen J . Engineered GFP as a vital reporter in plants. Curr. Biol. (1996) ;6: :325–330. |
[5] | Wroblewski T , Tomczak A , Michelmore R . Optimization of Agrobacterium-mediated transient assays of gene expression in lettuce, tomato and Arabidopsis. Plant Biotechnol. J. (2005) ;3: :259–273. |
[6] | Sparkes IA , Runions J , Kearns A , Hawes C . Rapid, transient expression of fluorescent fusion proteins in tobacco plants and generation of stably transformed plants. Nat. Protoc. (2006) ;1: :2019–2025. |
[7] | Bhaskar PB , Venkateshwaran M , Wu L , Ané JM , Jiang J . Agrobacterium-Mediated Transient Gene Expression and Silencing: A Rapid Tool for Functional Gene Assay in Potato. PLoS One. (2009) ;4: :e5812. |
[8] | Miyawaki K , Fukuoka S , Kadomura Y , Hamaoka H , Mito T , Ohuchi H , Schwab W , Noji S . Establishment of a novel system to elucidate the mechanisms underlying light-induced ripening of strawberry fruit with an Agrobacterium-mediated RNAi technique. Plant Biotechnol. (2012) ;29: :271–277. |
[9] | Broderson P , Voinnet O . The diversity of RNA silencing pathways in plants. Trends Genet. (2006) ;22: :268–280. |
[10] | Filipowicz W , Jaskiewicz L , Kolb FA , Pillai RS . Post-transcriptional gene silencing by siRNAs and miRNAs. Curr. Opin. Struct. Biol. (2005) ;15: :331–341. |
[11] | Anandalakshmi R , Pruss GJ , Ge X , Marathe R , Smith TH , Vance VB . A viral suppressor of gene silencing in plants. Proc. Natl. Acad. Sci. USA. (1998) ;95: :13079–13084. |
[12] | Kasschau KD , Carrington JC . A counter-defensive strategy of plant viruses: Suppression of post-transcriptional gene silencing. Cell. (1998) ;95: :461–470. |
[13] | Voinnet O . RNA silencing as a plant immune system against viruses. Trends Genet. (2001) ;17: :449–459. |
[14] | Kontra L , Csorba T , Tavazza M , Lucioli A , Tavazza R , Moxon S , Tisza V , Medzihradszky A , Turina M , Burgyán J . Distinct Effects of p19 RNA Silencing Suppressor on Small RNA Mediated Pathways in Plants. PLoS Pathog. (2016) ;12: :e1005935. |
[15] | Lakatos L , Szittya G , Silhavy D , Burgyan J . Molecular mechanism of RNA silencing suppression mediated by p19 protein of tombus viruses. EMBO J. (2004) ;23: :876–884. |
[16] | Voinnet O , Rivas S , Mestre P , Baulcombe D . An enhanced transient expression system in plants based on suppression of gene silencing by the p19 protein of tomato bushy stunt virus. Plant J. (2003) ;33: :949–956. |
[17] | Li Y , Pi M , Gao Q , Liu Z , Kang C . Updated annotation of the wild strawberry Fragaria vesca V4 genome. Hor. Res. (2019) ;6: :61. |
[18] | Yang Y , Davis TM . A New Perspective on Polyploid Fragaria (Strawberry) Genome Composition Based on Large-Scale, Multi-Locus Phylogenetic Analysis. Genome Biol. Evol. (2017) ;9: :3433–3448. |
[19] | Edger PP , Smith R , McKain MR , Cooley AM , Vallejo-Marin M , Yuan Y , Bewick AJ , Ji L , Platts AE , Bowman MJ , Childs KL , Washburn JD , Schmitz RJ , Smith GD , Chris Pires J , Puzey JR . Subgenome dominance in an interspecific hybrid synthetic allopolyploid and a 140-year-old naturally established neo-allopolyploid monkeyflower. Plant Cell. (2017) ;29: :2150–2167. |
[20] | Edger PP , Poorten TJ , VanBuren R , Hardigan MA , Colle M , McKain MR , Smith RD , Teresi SJ , Nelson ADL , Man Wai C , Alger EI , Bird KA , Yocca AE , Pumplin N , Ou S , Ben-Zvi G , Brodt A , Baruch K , Swale T , Shiue L , Acharya CB , Cole GS , Mower JP , Childs KL , Jiang N , Lyons E , Freeling M , Puzey JR , Knapp SJ . Origin and evolution of the octoploid strawberry genome. Nature Genet. (2019) ;51: :541–547. |
[21] | Guidarelli M , Zoli L , Orlandini A , Bertolini P , Baraldi E . The Mannose Binding Lectin gene FaMBL1 is involved in the resistance of unripe strawberry fruits to Colletotrichum acutatum . Mol. Plant Pathol. (2014) ;15: :832–840. |
[22] | Griesser M , Hoffmann T , Bellido ML , Rosati C , Fink B , Kurtzer R , Aharoni A , Muñoz-Blanco J , Schwab W . Redirection of flavonoid biosynthesis through the down-regulation of an anthocyanidin glucosyltransferase in ripening strawberry fruit. Plant Physiol. (2008) ;146: :1528–1539. |
[23] | Hoffmann T , Kalinowski G , Schwab W . RNAi-induced silencing of gene expression in strawberry fruit (Fragaria x ananassa) by agroinfiltration: A rapid assay for gene function analysis. Plant J. (2006) ;48: :818–826. |
[24] | Hoffmann T , Kurtzer R , Skowranek K , Kiessling P , Fridman E , Pichersky E , Schwab W . Metabolic engineering in strawberry fruit uncovers a dormant biosynthetic pathway. Metab. Eng. (2011) ;13: :527–531. |
[25] | Lin X , Xiao M , Luo Y , Wang J , Wang H . The effect of RNAi-induced silencing of FaDFR on anthocyanin metabolism in strawberry (Fragaria×ananassa) fruit. Sci. Hortic. (2013) ;160: :123–128. |
[26] | Salvatierra A , Pimentel P , Moya-León MA , Herrera R . Increased accumulation of anthocyanins in Fragaria chiloensis fruits by transient suppression of FcMYB1 gene. Phytochem. (2013) ;90: :25–36. |
[27] | Spolaore S , Trainotti L , Casadoro G . A simple protocol for transient gene expression in ripe fleshy fruit mediated by Agrobacterium . J. ExBot. (2001) ;52: :845–850. |
[28] | Yeh SY , Huang FC , Hoffmann T , Mayershofer M , Schwab W . FaPOD27 functions in the metabolism of polyphenols in strawberry fruit (Fragaria sp.) . Front. Plant Sci. (2014) ;5: :518. |
[29] | Cui MY , Wei W , Gao K , Xie YG , Guo Y , Feng JY . A rapid and efficient Agrobacterium-mediated transient gene expression system for strawberry leaves and the study of disease resistance proteins. Plant Cell Tiss. Organ Cult. (2017) ;131: :233–246. |
[30] | Tian J , Cheng L , Han ZY , Yao YC . Tobacco rattle virus mediated gene silencing in strawberry plants. Plant Cell Tiss. Organ Cult. (2014) ;120: :1131–1138. |
[31] | López-Aranda J , Pliego-Alfaro F , Lopez-Navidad I , Barceló-Muñoz M . Micropropagation of strawberry (Fragaria x ananassa Duch.). Effect of mineral salts, benzyladenine levels and number of subcultures on the in vitro and field behavior of the obtained microplants and the fruiting capacity of their progeny. J. Hort. Sci. (1994) ;69: :625–637. |
[32] | Murashige T , Skoog F . A revised medium for rapid growth and bioassays with tobacco tissue cultures. Physiol. Plant. (1962) ;15: :473–497. |
[33] | Hood EE , Gelvin SB , Melchers S , Hoekema A . New Agrobacterium helper plasmids for gene transfer to plants (EHA105). Trans. Res. (1993) ;2: :208–218. |
[34] | Takeda A , Sugiyama K , Nagano H , Mori M , Kaido M , Mise K , Tsuda S , Okuno T . Identification of a novel RNA silencing suppressor, NSs protein of Tomato spotted wilt virus. FEBS J. (2002) ;532: :75–79. |
[35] | Sambrook J , Fritish E , Maniatis T . (ed.): Molecular Cloning, a Laboratory Manual. Cold Spring, Harbor Laboratory Press, Cold Spring Harbor, New York – (1989) . |
[36] | Vellicce GR , Coll Y , Castagnaro A , Díaz Ricci JC . Transformation of a strawberry cultivar using a modified regeneration medium. Hort. Sci. (2003) ;38: :277–280. |
[37] | Johansen LK , Carrington JC . Silencing on the spot. Induction and suppression of RNA silencing in the Agrobacterium-mediated transient expression system. Plant Physiol. (2001) ;126: :930–938. |
[38] | Di Rienzo JA , Casanoves F , Balzarini MG , Gonzalez L , Tablada M , Robledo CW . InfoStat versión 2013. Grupo InfoStat, FCA, Universidad Nacional de Córdoba, Argentina. http://www.infostat.com.ar;(2013) . |
[39] | Oosumi T , Gruszewski HA , Blischak LA , Baxter AJ , Wadl PA , Shuman JL , Veilleux RE , Shulaev V . High-efficiency transformation of the diploid strawberry (Fragaria vesca) for functional genomics. Planta. (2006) ;223: :1219–1230. |
[40] | Phillip AW . Improved regeneration and Agrobacterium-mediated transformation of wild strawberry (Fragaria vesca L.). MS diss, Virginia Polytechnic Institute and State University;(2005) . |
[41] | Zhang Q , Folta KM , Davis TM . Somatic embryogenesis, tetraploidy, and variant leaf morphology in transgenic diploid strawberry (Fragaria vesca subspecies vesca ‘Hawaii 4’). BMC Plant Biology;201;14: :23. |
[42] | Razavi F , Folta K , Zamariola L , Geelen D , De Keiser E , De Riek J , Van Labeke MC . Genetic transformation of Fragaria with candidate genes involved in drought stress. Acta Hort. (2012) ;961. |
[43] | Baulcombe D . RNA silencing in plants. Nature. (2004) ;431: :356–363. |
[44] | Leuzinger K , Dent M , Hurtado J , Stahnke J , Lai H , Zhou X , Chen Q . Efficient Agroinfiltration of Plants for High-level Transient Expression of Recombinant Proteins. J. Vis. Exp. (2013) ;77: :e50521. |
[45] | Chalfoun NR , Grellet CF , Martínez-Zamora MG , Díaz-Perales A , Castagnaro AP , Díaz-Ricci JC . Purification and Characterization of AsES Protein: A subtilisin secreted by Acremonium strictum is a novel plant defense elicitor. J. Biol. Chem. (2013) ;288: :14098–14113. |
[46] | Hael Conrad V , Abou Mansour E , Díaz Ricci JC , Métraux JP , Serrano M . The novel elicitor AsES triggers a defense response against Botrytis cinerea in Arabidopsis thaliana. Plant Sci. (2015) ;241: :120–127. |
[47] | Hael Conrad V , Perato SM , Arias ME , Martínez Zamora MG , Martos GG , Castagnaro AP , Díaz-Ricci JC , Chalfoun NR . The elicitor protein AsES induces a SAR response accompanied by systemic microbursts and micro-HRs in Fragaria ananassa. Mol. Plant Microbe Interact. (2017) ;31: :46–60. |
[48] | Hamilton A , Voinnet O , Chappell L , Baulcombe D . Two classes of short interfering RNA in RNA silencing. EMBO J. (2002) ;21: :4671–4679. |
[49] | Himber C , Dunoyer P , Moissiard G , Ritzenthaler C , Voinnet O . Transitivity-dependent and independent cell-to-cell movement of RNA silencing. EMBO J. (2003) ;22: :4523–4533. |
[50] | Chiera JM , Lindbo JA , Finer JJ . Quantification and extension of transient GFP expression by the co-introduction of a suppressor of silencing. Trans. Res. (2008) ;17: :1143–1154. |
[51] | Palauqui JC , Elmayan T , Pollien JM , Vaucheret H . Systemic acquired silencing: Transgene-specific post-transcriptional silencing is transmitted by grafting from silenced stocks to non-silenced scions. EMBO J. (1997) ;16: :4738–4745. |
[52] | Nekrasov V , Wang C , Win J , Lanz C , Weigel D , Kamoun S . Rapid generation of a transgene-free powdery mildew resistant tomato by genome deletion. Sci. Rep. (2017) ;7: :482. |