Metabolic and physiologic profile during the fruit ripening of three blueberries highbush (Vaccinium corymbosum) cultivars
Abstract
BACKGROUND:
Blueberry was introduced as a crop in Argentina about 30 years ago. Its harvesting period ranges from September to December, during the northern hemisphere (its main export destination) fall season, which makes it a profitable commercial crop. As most part of the production is exported fresh, the understanding of biochemical aspects connected with fruit firmness is crucial to improve marketable conditions.
OBJECTIVE:
The main purpose of this work is to explore the metabolic and physiologic changes in three highbush blueberry cultivars during maturation and the possible association with their contrasting firmness features.
METHODS:
Vaccinium corymbosum cv. ‘Emerald’, ‘Snowchaser’ and ‘O’Neal’, in order of decreasing firmness, were collected at green and ripe stages. Metabolites were analyzed by gas chromatography-mass spectrometry (GC-MS) and HPLC. Total phenolic compounds, pectin methyl esterase (PME) and β-galactosidase activities were quantified by colorimetric assays.
RESULTS:
Multivariate analysis of metabolites differentiated fruit regarding their maturation state in the first place. Malic, citric and phosphoric acids, asparagine (Asn) and mannitol were more abundant in green fruits. Conversely, mature fruits were distinguished by their higher content of citrulline and turanose. Other compounds were responsible for the differentiation between varieties: histidine (His), valine (Val), arginine (Arg), methionine (Met) and sucrose where high in ripe Snowchaser, while green and ripe Emerald had more tryptophane (Trp), glycine (Gly), phenylalanine (Phe), Trp, Gly and glucose. An interesting finding is that Emerald, the firmer variety, had less xylose content at both stages, possibly owing to a minor degree of cell wall degradation. Fold change of PME and β-galactosidase activity from green to ripe fruit demonstrated a divergent tendency in Emerald and Snowchaser compared to O’Neal. A correlation study strongly and positively connected firmness with citric acid and phenylalanine (Phe) content, while xylose, leucine (Leu) and shikimic acid were negatively related to this attribute.
CONCLUSIONS:
This study suggests that changes in the content of a few metabolite and activities of cell wall modifying enzymes during maturation period could be correlated with the observed difference in firmness of the blueberries studied. These findings may yield clues for improvements in fertilization protocols as well as to serve to the guided development of new varieties based on biochemical quality traits.
1Introduction
Vaccinium corymbosum (blueberry) belongs to the Ericaceae family and is native from eastern of North America. Regarding their growth size they can be classified in highbush (V. corymbosum), lowbush (V. angustifolium) or rabbiteye (V. virgatum) [1]. Highbush blueberries comprise Southern and Northern varieties, with low and high chilling requirement (number of hours at temperatures below 7°C between 200 and 400), respectively. Southern highbush cultivars are thus well adapted to the mild climate of the Mediterranean region of Argentina. The cultivated area of blueberries has expanded rapidly since it was initially introduced in the mid 1990 decade. Indeed, acreage has grown six times in the last ten years [https://inta.gob.ar/sites/default/files/script-tmp-cadena_arandano.pdf].
Most of the Argentinean berries production is exported to the northern hemisphere, during the boreal fall season (September to December), when it might reach competitive prices due to lack of local production. Given the relevance of this crop, many efforts have been directed to improve fruit attributes which may have influence quality, transport and marketable conditions. One critical trait is firmness, since a soft fruit is perceived as over-ripen and may impact negatively on the consumer’s choice. Loss of firmness is caused by several factors, such as turgor, cell membranes damage, dehydration and cell wall dynamics [2–5]. At the same time, these components are affected by climate, soil composition and varieties, among other aspects, causing inconsistent results in terms of fertilization, pre- and postharvest treatments [6, 7]. In Argentine, as in many other countries, calcium fertilization is a current practice, both by soil or foliar application [8–10], with fluctuating results. Research in this field has been focused on the measure of several parameters related to cell wall metabolism after diverse calcium treatments, like composition and methylation degree of pectin, activities of enzymes involved in cell wall synthesis and degradation and/or calcium levels associated with wall components [9, 11, 12]. However, there are not many studies intended to characterize different varieties under the same edaphic and fertilization conditions that help to understand the intrinsic features of each cultivar that could be relevant for their general field and postharvest behavior.
In this study, a metabolomic approach has been considered to evaluate three blueberry varieties broadly cultivated in the eastern region of Argentina at two developmental stages. They were selected according to their differences in firmness attributes and were characterized based on their metabolite content and physiological features. They all are southern highbush cultivars, successfully adapted to mild winters in this region of South America. ‘Emerald’ (U.S. Plant Patent 12165 P2) fruit is large, firm, exhibits a medium blue color, with good flavor and is very productive. ‘Snowchaser’ (U.S. Plant Patent 19503 P3) is an early ripening variety, exhibits a medium sized, good flavored fruit with a light blue color. ‘O’Neal’ is an early, public variety, with medium size fruit and very sweet (http://www.fallcreeknursery.com/commercial-fruit-growers/varieties). The activity of two enzymes linked to cell wall metabolism was also measured and the analysis were conducted to advance in the understanding of metabolites/activities that could be considered as a biochemical signature in terms of fruit quality. After these studies some basic differences were evident between developmental stages and varieties. Furthermore, the abundance of some metabolites and relative variation of cell wall modifying enzymes could be correlated with firmness. This knowledge may help to outline fertilization programs adapted to local climate and soils. Likewise, it can assist agronomists with low-cost, reliable, methods for screening and selection of varieties.
2Methods
2.1Fruit sampling
Blueberries from ‘Emerald’, ‘Snowchaser’ and ‘O’Neal’ cultivars were collected at local orchards in Concordia (Entre Ríos, Argentina) during the morning, in two consecutive seasons, 2015 and 2016. Mature bushes used for field experiments were located in commercial fields. The plants were grown on raised pine bark rows with a plant density of 3333 plants/ha. Overhead sprinklers were used for frost protection. Standard agro-technical procedures including winter pruning, fertirrigation, pest, diseases and weed control were performed during the growing season. Flower phenology was monitored in each cultivar to determine the progression of fruit maturity. The sampling dates in each cultivar were at 27 days after full bloom (DAFB) and 88 DAFB, corresponding to initial green fruit (25% of the final size) and ripe fruit (full blue fruit), respectively. Snowchaser blooms in June and harvest season last from September to November. Emerald blooms in July and harvest season spans from October to December, while O’Neal blooms in late July and is harvested from November to December. Thirty berries were collected from five different plants of each variety. After manual collection, epicarp and pulp (meso and endocarp) were separated and frozen at –80°C until analysis, except for texture determinations that were performed immediately after harvest. All the subsequent determinations were performed on the pulp. At least three biological replicates were performed for metabolite measures. Each consisted of a pool with the same weight of tissue from two different fruits.
2.2Texture analysis
Compression measure was performed with a TA.TX Plus Texture Analyser (Stable Micro Systems Ltd, UK), according to the following conditions: load: 5 kg; cylindrical plunger diameter: 75 mm; compression force at 10% of axial deformation; speed: 1 mm s−1. Fifteen determinations were done for each sample.
2.3Metabolite purification, derivatization and analysis
Samples were prepared as described by Perotti et al. [13]. Briefly, 300 mg of frozen tissue from 6 different fruit (2 fruit per pool, 3 biological replicates) was powdered in a mortar with liquid nitrogen. After transfer to glass tubes, 4.2 mL of cold methanol and 75μg of ribitol (as internal standard) were added, to allow the relative quantification of metabolites. Extracts were incubated at 70°C for 15 min. Afterwards, 1.5 mL of chloroform were added, followed by incubation at 37°C for 5 min. Finally, after adding 3 mL of water, extracts were centrifuged for 15 min at 2200×g and 4°C. The polar phase (450μL) was dried in a vacuum centrifuge (CentriVap, Labconco) until complete evaporation. For derivatization, 30μL of 20 mg/mL methoxyamine in pyridine were added. Tubes were shaken and incubated at 37°C for 90 min. Finally, 45μL of N-methyl-N-trimethylsylil-trifluoroacetamide (MSTFA) were added to each tube and incubated at 37°C for 30 min. Chromatographic runs were performed by injecting 2μL of derivatized sample in a 30 m long, 0.25 mm thick VSF GC/MS capillary column using an automatic system (Varian Inc.) coupled to a ThermoQuest mass spectrometer. Data were collected and analyzed using the Lab Solution software (Shimadzu). Spectra obtained were analyzed by comparing individual peak areas for each metabolite relative to that of ribitol, the internal standard. Data were revised using the online software Mass Spectra & Retention Time Index (MSRI) (The Comprehensive System Biology Project - CSB, http://www.csbdb.de/index.html) from the Golm Metabolomic Institute (Germany) to confirm the identity of the compounds.
2.4Amino acid extraction, derivatization and analysis
Amino acids were extracted from 0.2 g of tissue of 6 different fruit (2 fruit per pool, 3 biological replicates) that were powdered in a mortar with liquid nitrogen. After homogenization with 1.5 mL of HCl 0.1M, samples were centrifuged and supernatants were deproteinized with TCA (10% final concentration) as described in [14]. Derivatization was performed according to [15]. Samples were centrifuged, and supernatants were dried with 50μL of a methanol: water: triethylamine (2:2:1) mixture. Subsequently, 20μL of a derivatizing mix of methanol: water: triethylamine: phenyl isothiocyanate (7:1:1:1) were added to each sample. After incubation at room temperature for 20 min, samples were filtered, resuspended in 500μL of mobile phase and injected in a 250 mm×4.6 mm i.d. 5μm Lunatrademark C18 reversed phase column (Phenomenex, Torrance, CA, USA) at 40°C and a flow rate of 1.0 mL/min following the protocol described in [16].
2.5Total protein extraction and determination of protein concentration
Total protein extraction was carried out by grinding 0.6 g of tissue with liquid nitrogen and 1.5 mL of extraction buffer (1 M NaCl, 12.5 mM citric acid, 50 mM Na2HPO4 and PMSF 1:100 v/v, pH 6.5). Homogenates were shaken for 1.5 h at 4°C, centrifuged at 10.000×g for 20 min, and the recovered supernatants were maintained at 4°C for protein and enzyme activities measures. Protein concentration was determined using Bradford protein assay method [17], using Bio-Rad protein assay reagent and bovine serum albumin as standard.
2.6Pectin methyl esterase (PME) and β-galactosidase (β-gal) activity assays
PME activity was measured in berries using the pectoplate technique on a total protein and fresh weight basis [18]. Each well contained 0.65 ug of total protein. After 16 hs of incubation at 37°C, plates were stained with 0.05% ruthenium red. After destain, the area of red haloes resulting from de-methylesterification of pectin was registered in cm2. One PME activity unit (U) is defined as the area of red halo/hour. β-galactosidase activity was assayed in the same enzyme extracts as PME and was determined by measuring the hydrolysis rate of 4-nitrophenyl β-D-galactopyranoside (pNPG). Reaction mixtures were composed by 100μL of extract, 500μL of 0.1 M HAc-NaAc buffer pH 4.5, 400μL of BSA 0.1% and 400μL of 13 mM pNPG substrate. After 25 min at 37°C, 300μL of reaction mixture aliquots were taken and reactions were stopped by the addition of 450μL of 0.2 M sodium carbonate. The amount of p-nitrophenol was measured at 415 nm using a molar absorption coefficient of 18000 M–1 cm–1. One unit of β- galactosidase was defined as the amount of extract used to release 1 nmol of p-nitrophenol/minute at 37°C [19]. Three biological replicates were analyzed, and measurements were made in triplicate for both enzymes.
2.7Total phenolics determination
Total phenolic content was assayed employing the Folin-Ciocalteu reagent [20]. Fifteen milligrams of endocarp were extracted using 2 mL of buffer (80 % methanol and 1 % HCl) at room temperature for two hours in an orbital shaker. The mixture was centrifuged at 2500×g for 5 min and the supernatant was recovered. The pellets were re-extracted by repeating the previous steps, the supernatants were mixed and used for phenolic content determination. Combined supernatants (150μL) were mixed with 100μL of Folin-Ciocalteu reagent at room temperature for 3 min. 500μL of sodium bicarbonate (20%) were added and the reaction was allowed to stand for 120 min at room temperature. Absorbance was measured at 730 nm and results were expressed as gallic acid equivalents/g fresh weight. Determinations were made in triplicate.
2.8Statistical procedures
Data presented was evaluated using t-Student test and analysis of variance (ANOVA). Significant differences were calculated by the Bonferroni and Holm-Sidak test using the Sigma Stat Package (p < 0.05). In cases where the normality test (Shapiro-Wilk) failed, Kruskal-Wallis ANOVA on Ranks was carried out and Dunn’s method was used for all pairwise multiple comparison.
3Results and discussion
3.1Firmness and total phenolic content of ripe blueberries
Since fruit from this three varieties were known to have dissimilar firmness [21, 22], assays were conducted to ascertain the value of this parameter. Thus, considering the classification in soft (<1.6 N), medium (1.61–1.80 N) and firm (>1.81 N) categories [23], O’Neal would be a soft variety, Emerald definitely firm and Snowchaser firm, although very close to the medium firmness range.
Total phenolic content (TPC) in the pulp of blueberry samples was determined according to the Folin-Ciocalteu colorimetric method in fully mature endocarps (Table 1). The TPC was similar in Snowchaser and Emerald, and significantly lower in O’Neal. Phenolic compounds, that comprise flavonoids, anthocyanins and tannins, were found to change in composition and accumulation degree in response to several growth conditions, such as season, temperature, location or light incidence [24–26]. These compounds are related to plant defense and possess antioxidant functions and, although usually concentrated in the epicarp, they are also present in fruit pulp [25, 27]. In addition, the nutraceutical value of these substances for human health has been highlighted [28, 29]. One important fact about TPC, related to the purpose of this study, is that an inhibitory effect of phenolic compounds on PME activity has been described [30].
Table 1
Firmness and total phenolic content (TPC). Values represent means±SDs. Different letters indicate statistically significant differences. Data were tested using ANOVA. Significant differences were calculated by the Bonferroni and Holm-Sidak test using the Sigma Stat Package (p < 0.05). In cases where the normality test (Shapiro-Wilk) failed, Kruskal-Wallis ANOVA on Ranks was carried out and Dunn’s method was used for all pairwise multiple comparison
Variety | Firmness (N) | TPC (μg gallic acid/mg fw) |
---|---|---|
Emerald | 3.05±0.43 a | 0.36±0.03 a |
Snowchaser | 1.91±0.32 b | 0.39±0.03 a |
O’Neal | 1.53±0.23 c | 0.19±0.01 b |
Total free amino acid (FAA) levels, calculated from values acquired after HPLC analysis on a fresh weight base, did not display variation between green and mature fruit of any variety (Fig. 1). This result is mainly a consequence of the fact that concentrations of the two main contributors to total FAA content, proline (Pro) and tryptophan (Trp) did not show statistically significant differences between stages in any variety. Emerald green fruit contained a significantly higher FAA amount compared to O’Neal at the same stage, while Snowchaser was not significantly different from either of the two other varieties at any stage (Fig. 1). The amino acids that contributed the most to total FAA content were: glutamate (Glu) (12.0 %), Pro (46.4 %) and Trp (15 %) for O’Neal green fruit, Pro (33.6 %), tyrosine (Tyr) (10.9 %) and Trp (16.4 %) for O’Neal ripe fruit, hydroxyproline (OHPro) (9.4 %), Pro (44.1 %) and Trp (14.6 %) for Emerald green fruit, Pro (47.4 %) and Trp (13.2 %) for Emerald ripe fruit, Pro (47.7 %) and Trp (9.9 %) for Snowchaser green fruit, and Pro (45.1 %), valine (Val) (12.5 %) and Trp (12.2%) for Snowchaser ripe fruit (see Supplementary Table 1).
Fig. 1
Total free amino acid (FAA) content at the green and ripe stages for each variety. Quantification data are presented as means±standard deviation of three biological replicates. Lower case letters indicate statistically significant differences between ripening stage in a variety. Significant differences between varieties are represented by capital letters at green stage, and bold capital letters at ripe stage. Data was tested using ANOVA and t-Student test. All pairwise multiple comparisons were performed by the Bonferroni and Holm-Sidak method using the Sigma Stat Package (p < 0.05).
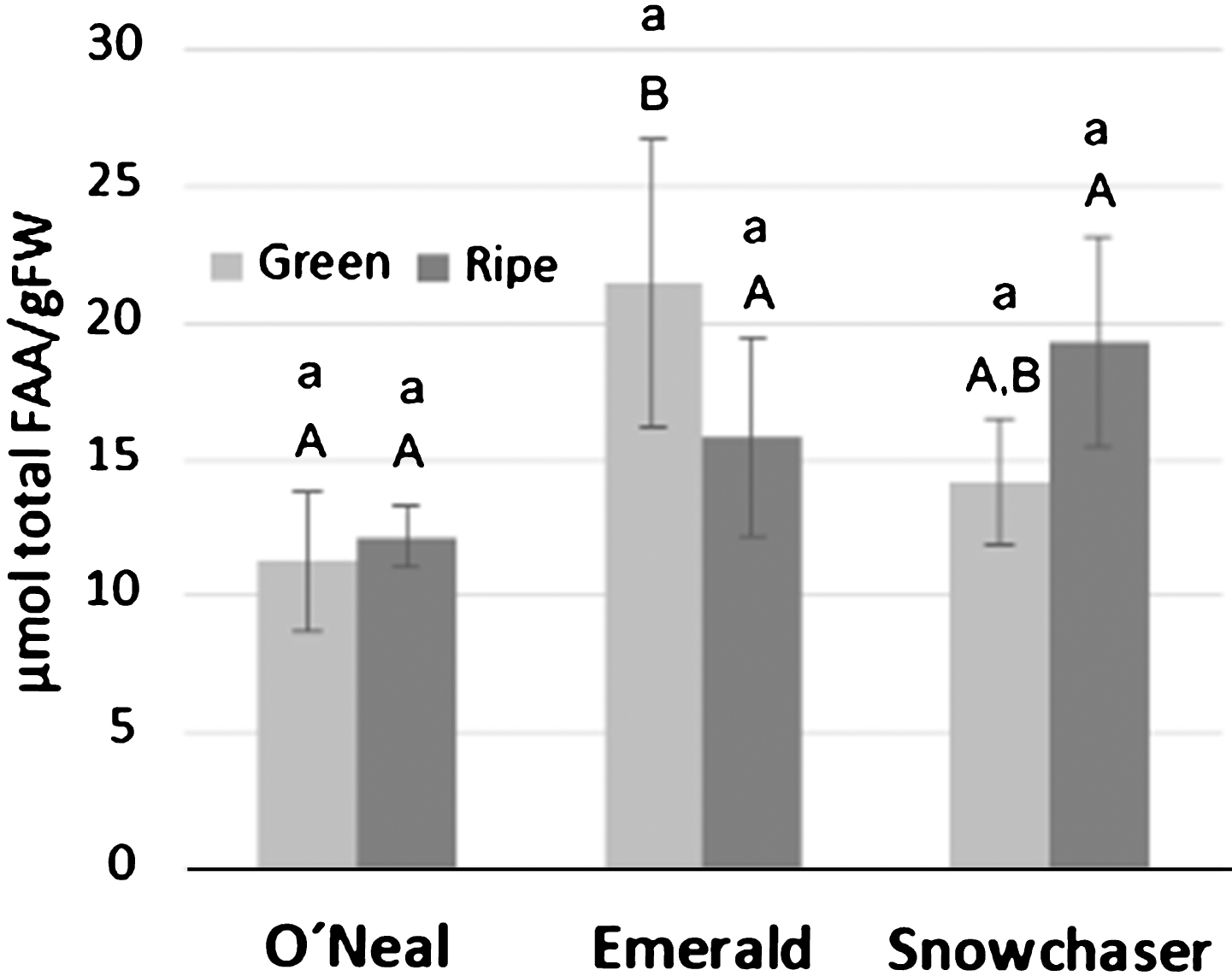
A few amino acids (Fig. 2) presented a particularly high abundance in some of the stages or varieties, in relation to others. Those whose abundance was higher than 40 % of the total concentration in a particular variety and stage were: Glu in O’Neal green fruit, aspartic acid (Asp) and glutamine (Gln) in Emerald green fruit, citrulline (Cit) in Emerald ripe fruit, Asn and threonine (Thr) in Snowchaser green fruit, Val and His in Snowchaser ripe fruit (Fig. 2, boxes). Gln relative content decreased with ripening in O’Neal and Emerald, Glu decreased in O’Neal, His increased in O’Neal and Snowchaser but decreased in Emerald, Cit increased in O’Neal and Emerald but was barely detected in Snowchaser and Thr increased in Emerald and decreased in Snowchaser (Fig. 2, asterisks). The amount of each amino acid in μmol/g FW is given in Supplementary Table 2.
Fig. 2
Amino acid contribution in each variety and ripening stage. The amino acids whose levels were higher than 40% are enclosed in a box, those with statistically significant difference in concentration between both maturation stages are indicated with asterisks (see the text for more details).
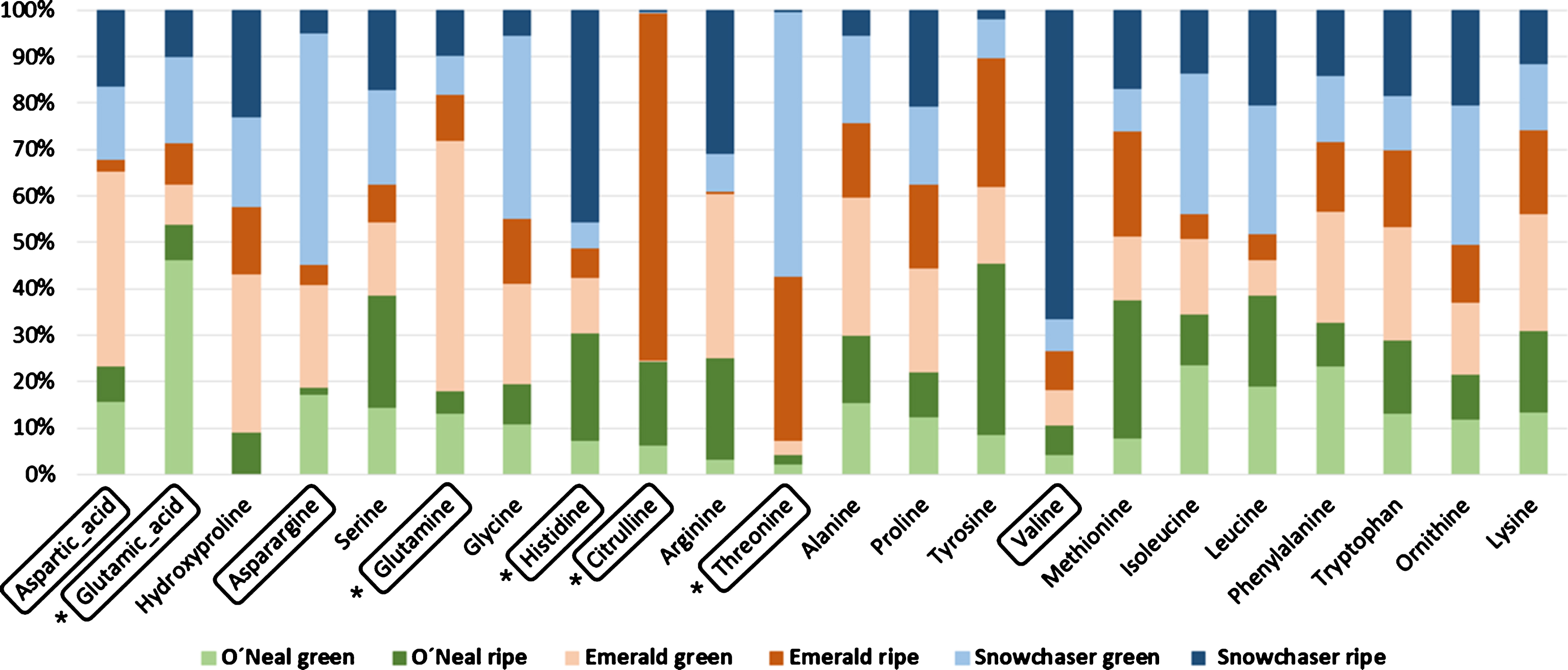
FAA participate in several metabolic processes such as salinity response, protein turnover or nitrogen metabolism and transport (especially for Glu, Gln and asparagine). Levels reported here were within the same range of those informed for strawberries and tomatoes [31, 32] although in the latter total FAA content was lower in green than in ripe fruit. In the blueberries analyzed here, total protein (in μg/g FW) was significantly lower in Emerald at the green stage in comparison with other varieties (data not shown), suggesting that protein turnover in green fruit is more relevant in this variety. The measure of enzymatic activities such as glutamate synthase, glutamine and asparagine synthetase could give information about the events linked to variation of these amino acids. High FAA, especially for essential amino acids, may also indicate a better nutritional quality, though this is not relevant in green fruit. A deeper analysis about the possible implications of these amino acids on berries metabolism is considered in the context of other metabolite changes in section 3.2.
3.2Metabolites in green and mature fruit: Sugars, sugar alcohols, amino acids, organic acids
The use of a metabolomic approach, aimed at comparing the natural variance of plants or to study the incidence of maturation state, environment or other aspects in the plant metabolic content has been intense in the last years [33–35]. Hence, by means of GC-MS and HPLC chromatographic techniques, changes in 40 metabolites of the varieties under study were identified in green and mature pulp of fruit (see relative values of each metabolite in Supplementary Table 3).
After data normalization, and multivariate statistical analysis, three principal components were able to explain 82.1 % of the total variance (Fig. 3). Principal component 1 (40.5 %) was able to distinguish green from ripe fruit. The three varieties display higher levels of phosphoric acid (PA), galactose (Gal), melibiose (Mel), mannitol, malic and citric acids, Asn, Asp, OHPro, Gln, Gly, Pro and isoleucine (Ile) at the green stage.
Fig. 3
Principal component (PC) analysis of metabolites from ripe and green fruit of the varieties under study. The percentage of variance explained by each component is indicated between parentheses (A: PC1 + PC2, B: PC1 + PC3). Metabolites that contribute the most to each component are indicated (the contribution data of all the variables is shown in supplementary table 4). Arg: arginine; Asn: asparagine; Asp: aspartic acid; Cit: citrulline; CQA: caffeoyl quinic acid; Fru: fructose; Gal: galactose; Gln: glutamine; Gly: glycine; His: histidine; Ile: isoleucine; Leu: leucine; Lys: lysine; Mel: melibiose; Met: methionine; MPG: mono palmitoyl glycerol; OHPro: hydroxy Proline; PA: phosphoric acid; Phe: phenylalanine; Pro: proline; Raf: raffinose; Ser: serine; Suc: sucrose; Thr: threonine; Trp: tryptophan; Tur: turanose; Val: valine; Xyl: xylose.
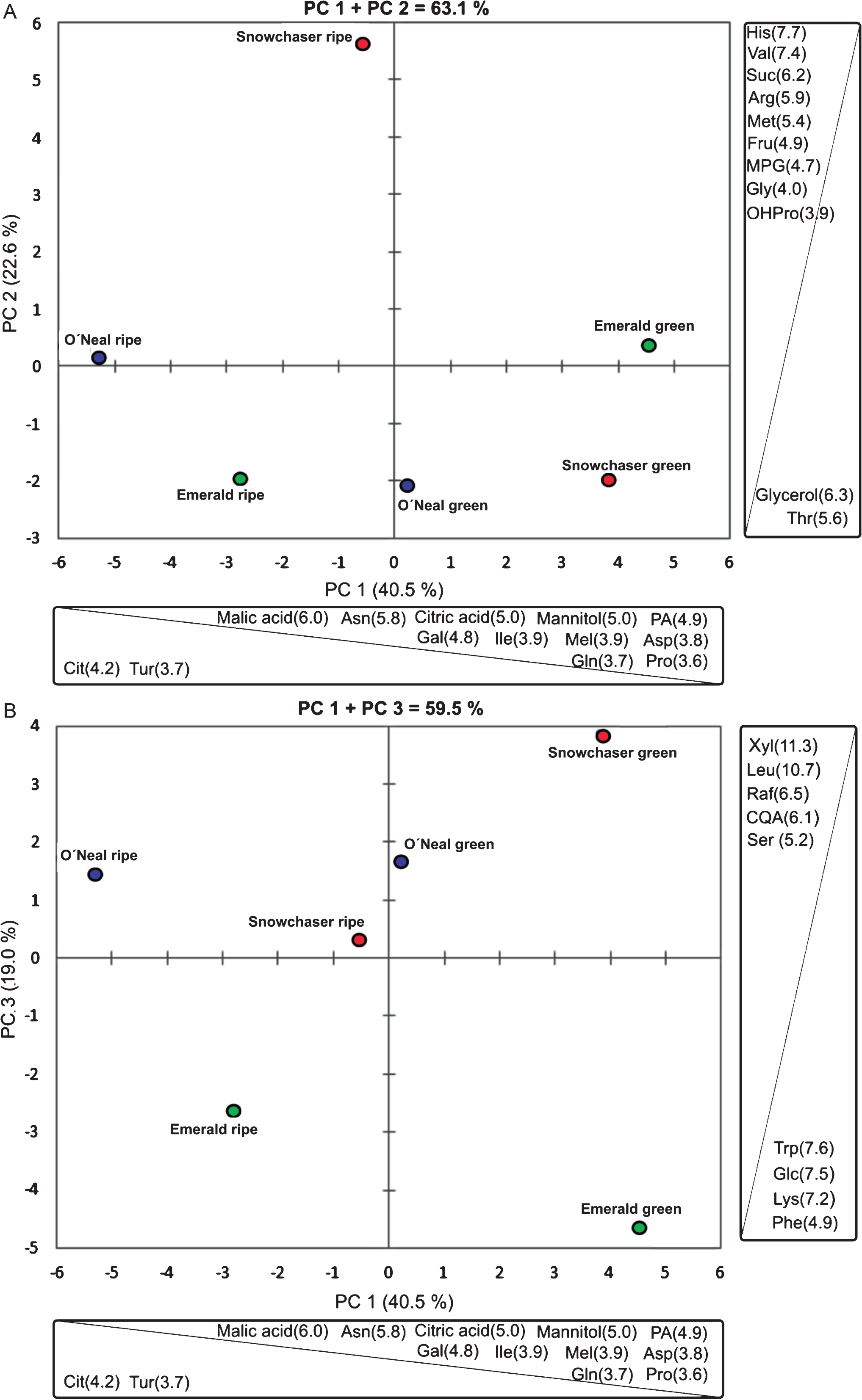
What this means for blueberry physiology is a multifaceted question since, as previously mentioned, FAA are directly and indirectly involved in a number of biological processes. For instance, Pro, Arg, Met and Glu are important in the regulation of plant responses to several environmental signals related to abiotic or biotic stress [36, 37]. Others may have effects on fruit taste: Glu is responsible for the delicious (umami) taste but also has taste-enhancing properties [38, 39]. His, Gly, lysine (Lys) and alanine (Ala) are highly correlated with sweetness, as Val, Phe and Tyr are with bitterness [40]. Levels of most of the amino acid detected (see Supplementary Table 2) were near or above their taste threshold [41]. Asn and Gln are involved in nitrogen transport and storage, being important links between nitrogen and carbon metabolism. Additionally, essential amino acids (not synthesized by mammals, e.g. methionine, cysteine, leucine, Lys, Ile, Val and aromatic amino acids) are appreciated by their nutritional value. Leu and Ile are precursors of branched chain fatty acids, which increase membranes fluidity. Pro, besides its role as osmoprotectant, is a component of an important family of glycosylated cell wall proteins, along with OHPro [42]. Galactose is also a major component of these glycoproteins, followed by arabinose and xylose [43]. From PCA analysis it is noticeable that in green fruit, several free amino acids with diverse functions are abundant, suggesting that metabolic processes such as nitrogen transport and storage, protein turnover and cell wall dynamic are very active at this stage.
As expected, malic and citric acid levels are more elevated in green fruit. These organic acids have several roles in plant metabolism, as cytosolic pH regulators or as biosynthetic precursors, and are also key contributors to taste in the mature fruit. Their level, controlled by degradation, synthesis and transport, fluctuate with growth conditions, climate, maturation stage and variety [44]. Citric acid is also associated with iron metabolism and availability. Mannitol and other polyalcohols are relevant osmolytes that protect tissues from dehydration and help to maintain turgor pressure [45]. Mannitol is also linked to boron transport from source to sink tissues through the phloem [46, 47], which may be very active during the green stage. Melibiose (D-galactopyranosyl-alpha-1-6-D-glucopyranose), along with raffinose and stachyose, is part of the raffinose family oligosaccharides (RFOs), metabolites known to accumulate under different environmental stresses [48]. Galactose is also associated with RFOs, with ascorbic acid synthesis (via galacturonic acid) and is a constituent of pectin side-chains.
Ripe Emerald and O’Neal were connected by having higher citrulline (Cit) and turanose content with respect to Snowchaser. Citrulline was first discovered in watermelon (Citrullus lanatus) and, although it is more abundant in cucurbitaceous plants, it is an ubiquitous non-proteinogenic amino acid [49]. Studies carried out in watermelon also showed increasing levels during fruit development [50]. Cit was not detected in Snowchaser at either maturity stage. Cit is also related with Arg metabolism and, indirectly, with putrescine synthesis. Moreover, it is a potent hydroxyl radical scavenger; it accumulates after drought stress, for which it has been ascribed a role as an osmoprotectant; and a role as a long- distance nitrogen transporter has also been suggested [49]. Turanose (3-O-α- D-glucopyranosyl-fructose) is an analog of sucrose with roles as a signaling molecule [51], able to activate regulatory MAPK pathways independently from sucrose, and to greatly accelerate fruit ripening in strawberries [52].
Notably, ripe Snowchaser fruit was separated from all other samples by principal component 2 (22.6%, Fig. 3 A), showing elevated contents of monopalmitoylglycerol (MPG), fructose, His, sucrose, Arg, Val and Met. Meanwhile, glycerol and Thr were abundant in ripe Emerald, green Snowchaser and green O’Neal. An increased content of MPG is produced by the action of lipases on diacylglycerols and may be indicative of a reorganization of certain cellular membranes components. Sugars in general, besides being a source of energy in heterotrophic tissues, have been connected to cold stress and osmoprotection or as ROS scavengers and protein stabilizers [53]. Sucrose is linked to a plethora of biological functions, and its signaling roles have been thoroughly reviewed [54, 55]. Higher fructose levels contribute to a sweeter fruit, since its sweetening power is higher than that of sucrose. As mentioned before, His is also associated with sweet taste, as Val is with bitterness.
A further distinction was hinted by principal component 3 (19.0 %, Fig. 3 B), since green and ripe Emerald fruit formed a detached group characterized by higher contents of Phe, Trp, Lys and glucose, while Snowchaser and O’Neal, held more serine (Ser), raffinose, caffeoyl-quinic acid (CQA), xylose and Leu levels at both maturity stages.
Phenylalanine, as the other two aromatic amino acids, is synthesized in plants through the shikimate pathway and is precursor of anthocyanins, flavonoids, lignin and other relevant phenolic compounds. Trp is a precursor of auxin, melatonin, serotonin and niacin, having crucial hormonal and nutritional functions [56, 57]. It also forms indole acetic (IAA) or jasmonic acid conjugates that thwart IAA hormonal responses [58]. CQA, esters between quinic and caffeic acid, are part of a group of compounds also known as chlorogenic acids, molecules with antioxidant properties [59]. Xylose has been identified as one the most abundant non-cellulosic sugars in blueberry primary cell walls [60] and dicots in general [61]. It may arise from xyloglucan depolymerization, which has a backbone of 1,4 β- linked glucose residues, as cellulose, but also holds short chains of xylose and galactose. This glycan cross-links with cellulose, strengthening the wall, suggesting that its integrity may be relevant during softening [62].
3.2.1Summary of metabolite differences found between maturation stages and varieties
Analytical inspection of the differential metabolite abundance in blueberries made it possible to indicate in the first place, a clear distinction between ripe and green fruit supported by the abundance of organic acids in green fruit, which decrease later on during development. Other compounds, like galactose, Pro and OHPro, suggest dynamic changes in cell wall metabolism, possibly related with cell expansion in this period. Secondly, a few metabolites allowed further distinction between varieties: some of them linked with taste, aroma and nutritional quality (sugars, free amino acids), while other were related with cell wall, hormone and antioxidant metabolisms (xylose, Trp, Phe and CQA). Thus, ripe Snowchaser was characterized by high His, Val, Arg, Met and sucrose; while green and ripe Emerald had more Trp, Gly, Phe and glucose.
An interesting finding is that Emerald had less free xylose content at both stages, which could indicate a lesser degree of cell wall depolymerization than the other varieties, a fact that may contribute to the higher measured firmness.
3.3Cell wall modifying enzymes
As mentioned previously, events such as loss of turgor, degree of pectin methylation, solubilization, branching and depolymerization, do not follow the same pattern in different species, or even in particular varieties from the same species. The preceding section showed that variation in some metabolites levels point to a differential change in cell wall metabolism depending on the variety and maturity stage. In order to increase our understanding of these processes, the activities of two enzymes crucially involved in cell wall metabolism were measured. PME catalyzes the demethylation of pectin (polygalacturonic acid) rendering a more porous cell wall in which the action of other pectinolytic enzymes, such as polygalacturonases, pectate lyases and β-galactosidases, is enabled [63]. At the same time, PME activity is necessary to generate sites for calcium bridges (free carboxylates), which turn the cell wall mechanically more resistant and less susceptible to hydrolysis [64]. β- galactosidases (β-gal) are present in plants as a family of glycosyl hydrolases that fulfill diverse roles: although mainly implicated in cell wall metabolism, they are also able to modify glycoproteins and glycolipids [65]. Neutral sugars (galactose, xylose, arabinose) could help to anchor pectins to the cell wall, thus as a consequence of β-gal activity on xyloglucans and rhamnogalacturonan I, it becomes softer and free galactose levels increase [66, 67].
A significant lower PME activity was detected in Emerald mature fruit in contrast with the other two varieties, which did not show substantial differences between them (Fig. 4 A and C). Additionally, a remarkable divergence was exhibited by green fruits, where O’Neal presented a higher PME activity than the other varieties, followed by Snowchaser and then by Emerald. Furthermore, PME activity increased in Emerald and Snowchaser, whereas in O’Neal, it decreased in ripe versus green fruit. On the other hand, when β-gal activity was measured (Fig. 4 B), Emerald green fruit showed the highest activity, with no significant difference between O’Neal and Snowchaser. Considering the ripe stage, O’Neal had the highest β-gal activity while the level in the other varieties did not vary considerably. With the intention to better understand the possible consequences on cell wall integrity, the fold change between both activities at ripe versus green stage was calculated (Fig. 5). It was evident that Emerald and Snowchaser, both firmer varieties, had the same tendency: higher PME and lower β-gal activity at ripe versus green stage, while the opposite was observed in the less firm, O’Neal. However, the fold change was substantially higher in Emerald (9.35) relative to Snowchaser (0.42) for PME, while for β-gal, levels decreased from green to ripe in Emerald (−0.76) and Snowchaser (−0.20) at a comparable degree.
Fig. 4
Activity of cell wall related enzymes in green versus ripe fruit. A. Pectin methyl esterase (PME) activity in the three varieties under study. B. β-galactosidase (β-gal) activity in the three varieties under study. C. Representative images of pectoplate experiment. Quantification data are presented as means±standard deviation of three biological replicates, independent replicates were carried out for each sample. Lower case letters indicate statistically significant differences between ripening stage in a variety. Significant differences between varieties are represented by capital letters at green stage, and bold capital letters at ripe stage. Data was tested using ANOVA and t-Student test. All pairwise multiple comparisons were performed by the Bonferroni and Holm-Sidak method using the Sigma Stat Package (p < 0.05).
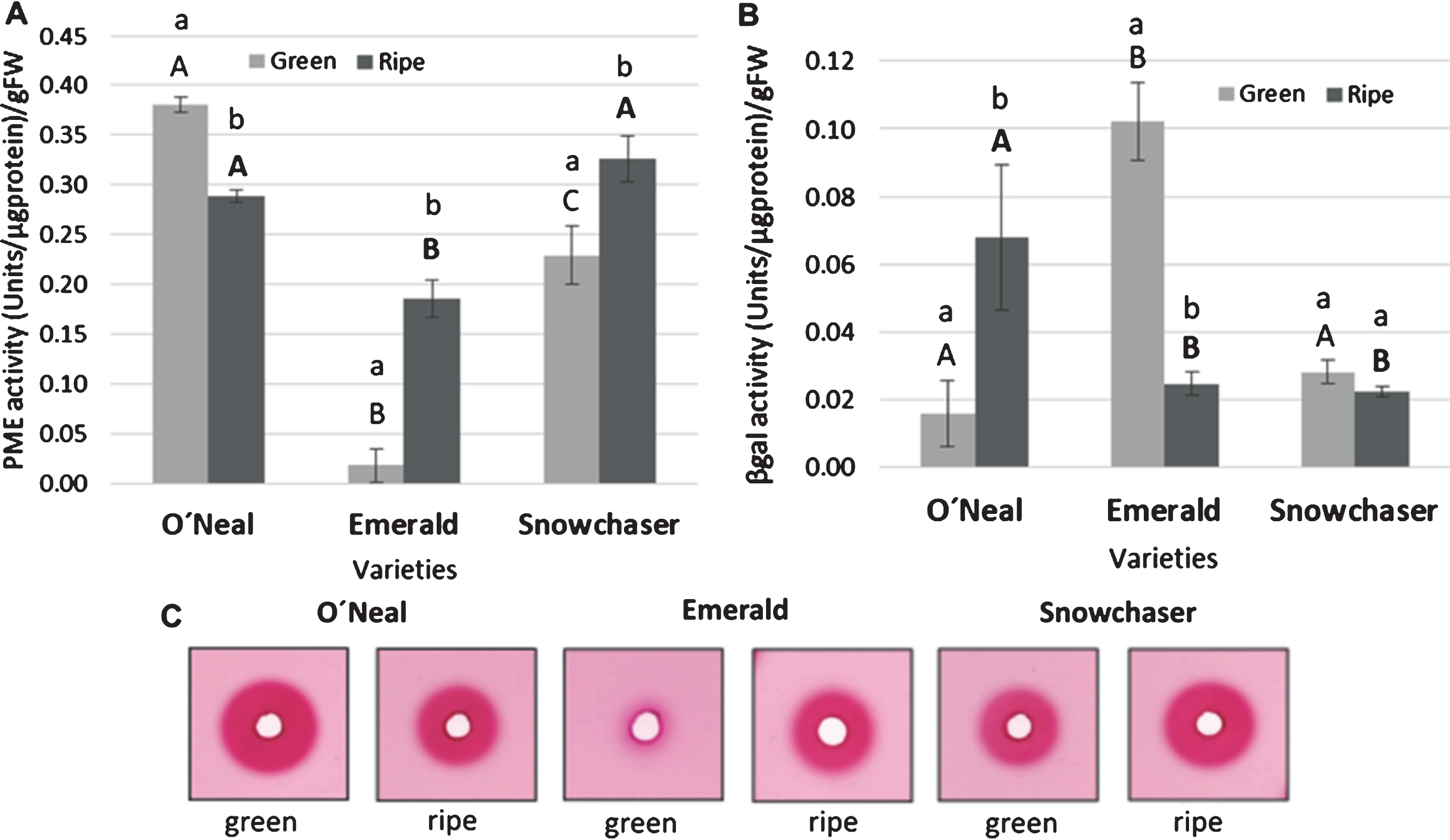
Fig. 5
Enzyme activity fold change from green to ripe fruit. Fold change values for pectin methyl esterase (PME) and β-galactosidase (β-gal) were calculated as: (ripe value - green value)/green value.
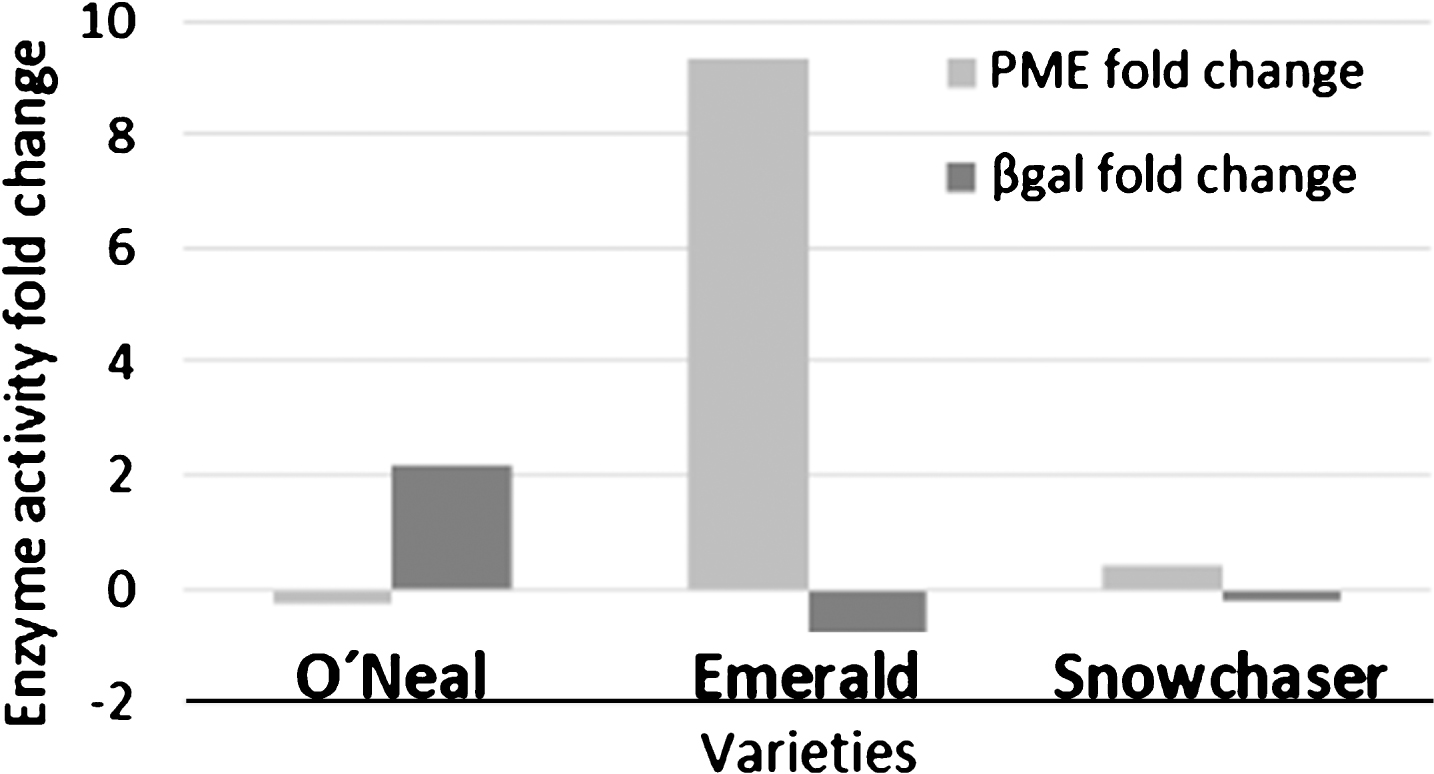
A study indicates that pectin solubilization in berries is observed at early maturation stages and that ripening is associated more with modifications in the hemicellulose-cellulose matrix than with pectin depolymerization [60]. Several factors might influence pectin solubility, it is thought that a high methylation degree, association with calcium and/or cross-linking with other cell wall components, turns it less soluble and difficult to extract [66, 67]. In fact, loss of neutral sugars from side chains of rhamnogalacturonans increased pectin solubilization in kiwi fruit [68]. Thus, one process that could promote its solubilization is demethylation conducted by PME. Above mentioned results are in agreement with more active pectin de-methylation in green O’Neal, that continue to be high in ripe stage. The important point here is that β-gal activity is higher in the less firm variety at ripe stage, suggesting that a combination of a more soluble pectin in presence of this high level of a hydrolytic enzyme could be in part the cause of a reduced firmness. On the contrary, for Snowchaser and Emerald, pectin demethylation appears to increase during ripening but in concert with a decrease in β-gal activity, more evident for Emerald, the firmer one. A similar observation, but with another hydrolytic enzyme, polygalacturonase, was reported with grape berries [69]. However, it is worth to mention that both activities might result from the contribution of distinct isoforms known to be present and differentially expressed during development [65, 70]. Thus, which isoform is responsible for each activity needs further research. The measure of other enzymatic activities, such as polygalacturonases, pectate lyases, as well as the calcium content in cell wall is necessary to gain information about the metabolic process that is actually taking place.
3.4Firmness related metabolites
With the aim of gaining insight into the molecular processes that could be related more specifically with fruit firmness, a correlation analysis between this factor, primary metabolites content, TPC, β-gal and PME activities was performed. The correlation coefficient allowed to ascertain the linkage between these parameters, independently of the variety. A total of 903 pairs were analyzed, from which, in mature fruit, 195 resulted in significant correlation coefficients (p-value < 0.05, Fig. 6 A). Citric acid (0.94), Phe (0.75), xylose (−0.83), Leu (−0.74) and shikimic acid (−0.73) demonstrated strong and significant positive and negative correlation with firmness, respectively (Fig. 5 A). Other variables showed correlations that, although not statistically significant, were strong, as for mannitol (0.77) and β-gal (−0.75).
Fig. 6
Correlation matrix between primary metabolites and firmness in mature fruit (A) or green fruit (B). Circles represent Spearman’s correlation coefficient value for a pair of metabolites. Positive correlations are displayed in light grey and negative correlations in dark grey. Color intensity and size of the circle are proportional to the correlation coefficients. Significant correlations are indicated with a star inside the corresponding circle (p-value < 0.5).
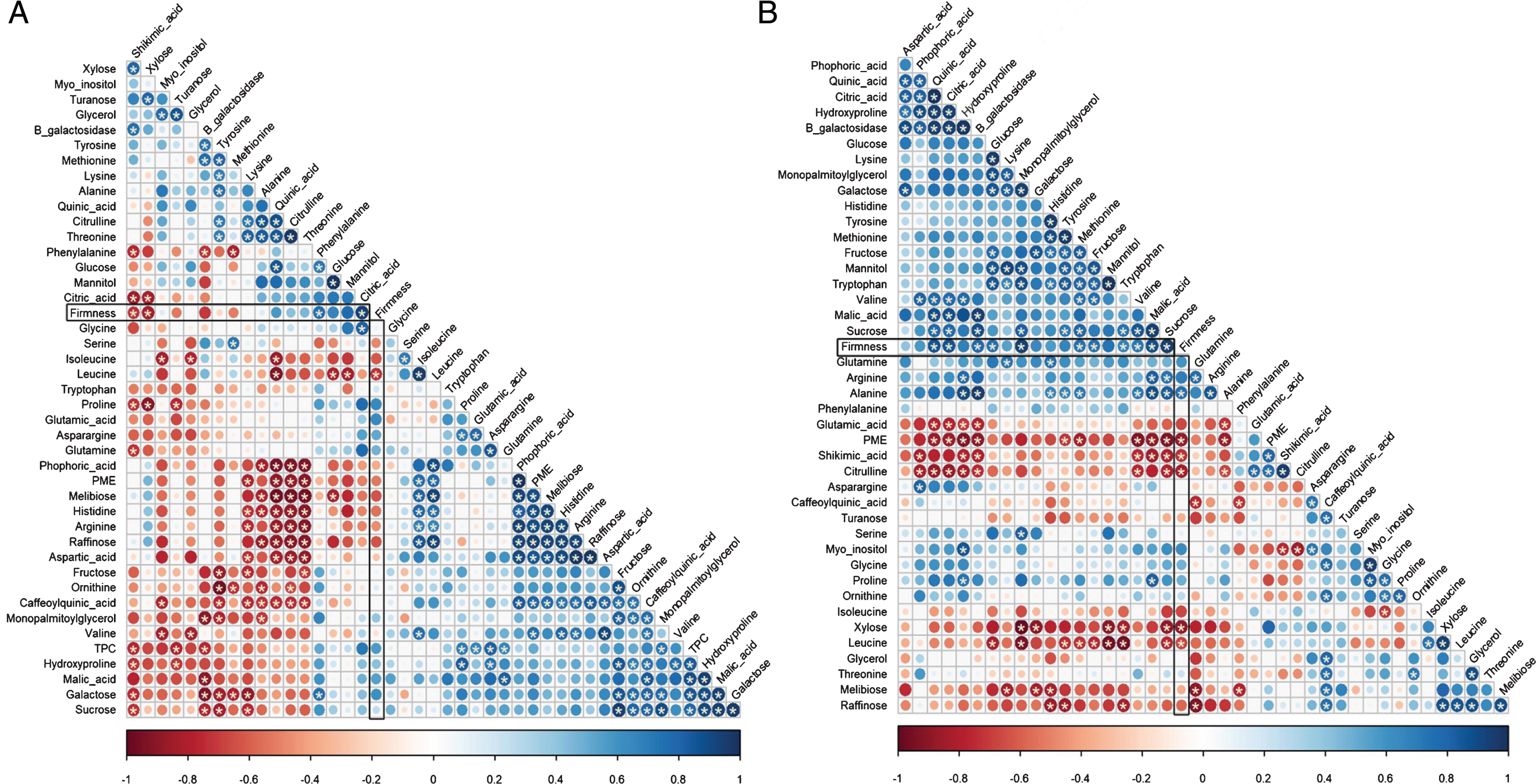
As some metabolic changes that are known to be related to firmness in ripe fruit take place early in developmental stages [71, 72], the same analysis was carried out in green fruit, in an attempt to relate them with observed firmness later on in the ripe stage (Fig. 6 B). In this case, 185 pairs resulted in significant correlation coefficients (p-value < 0.05). The following metabolites displayed a significant and strong correlation with firmness: sucrose (0.96), malic acid (0.89), MPG (0.94), quinic acid (0.86), citric acid (0.86), β-gal (0.79), glucose (0.76), Met (0.75), fructose (0.74), Thr (0.68), Val (0.73), Ala (0.76), Leu (−0.68), PME (−0.87), shikimic acid (−0.73), xylose (−0.89) and citrulline (−0.84).
In plants, a biological marker has been defined as a characteristic that is objectively evaluated as predictor of plant performance [73]. In this sense, a gene, transcript, metabolite or an enzyme activity, are subsets of these biomarkers that could assist to different purposes, for instance, as diagnostic or breeding tools. One way of validating a biomarker is to find out the correlation degree between the marker and the trait under study. In this work, such a correlation analysis, performed between metabolites and enzymes levels in green and ripe fruit with firmness observed at harvest date, helped us to infer that some metabolites can be used as markers of firmness. Indeed, high levels of citric acid and Phe in ripe fruit, or sucrose, citric acid, malic acid, MPG, quinic acid or β-gal activity in green stage, are strongly associated with high firmness. Conversely, a high content of xylose, Leu and shikimic acid in ripe fruit, or large amounts of xylose, PME activity, Cit and shikimic acid in green stage, indicate reduced firmness.
Clarifying the mechanisms by which each of these components affect fruit firmness need extra research. However, it is possible to deduce some clues about them. In tomato, malic acid content was highly correlated with firmness and shelf-life [74], possibly by promoting a decrease in water loss by transpiration [75]. In the present work, as in [12], the combination of high PME and β-gal activities in ripe fruit could indicate cell wall solubilization and degradation, the last statement reinforced by high xylose content at both maturity stages in the less firm variety. Regarding shikimic acid and Leu, precursors for phenylpropanoids and branched fatty acids, respectively, the mechanistic relationships to reduced firmness are not clear at this time.
4Conclusion
Preliminary studies in blueberries, in the first place, helped to characterize changes between green and ripe fruit, and in second place, made it possible to find out some specific differences between varieties. Another relevant outcome is that these findings give some clues to further investigate the potential mechanisms involved in the preservation of firmness in blueberries. Future studies of other development stages and the associated proteome in each variety, as well as the activity of additional pectinolytic enzymes (like polygalacturonases and pectate lyases), their activity modulators (i.e. PME and polygalacturonases inhibitors) and calcium levels will probably contribute to a more complete understanding of changes during fruit growth. A strong correlation between firmness and some metabolites or enzymes related with organic acids, carbohydrate, cell wall, cellular membranes and protein metabolisms could be determined. Furthering our knowledge on how these changes are associated with fruit quality in general, and with firmness in particular, will enhance our understanding about suitable markers or standards useful for future comparison between fertilization treatments, variety selection and local breeding programs.
Conflict of interest
The authors have no conflict of interest to report.
Acknowledgments
This work was supported by grants from by the Agencia Nacional de Promoción Científica y Tecnológica to KEJT (PICT 2016-0091) and FEP (PICT 2015-1074). FEP and KEJT are members of the investigator Career from the Consejo Nacional de Investigaciones Científicas y Técnicas (CONICET), MLM is a Doctoral Fellow of CONICET. FB, MFR and DV are members of the Instituto Nacional de Tecnología Agropecuaria (INTA).
Supplementary material
[1] The supplementary material is available in the electronic version of this article: 10.3233/JBR-180309.
References
[1] | Prodorntti D , Pertot I , Giongo L , Gessler C. Highbush Blueberry: Cultivation, Protection, Breeding and Biotechnology. J Plant Sci Biotechology. (2007) ;1: :44–56. |
[2] | Brummell DA , Dal Cin V , Crisosto CH , Labavitch JM . Cell wall metabolism during maturation, ripening and senescence of peach fruit. J Exp Bot. (2004) ;55: (405):2029–39. |
[3] | Vicente AR , Manganaris GA , Sozzi GO , Crisosto CH . Nutritional Quality of Fruits and Vegetables. In: Postharvest Handling. (2009) . pp. 57–106. |
[4] | Zoccatelli G , Zenoni S , Savoi S , Dal Santo S , Tononi P , Zandona V , . Skin pectin metabolism during the postharvest dehydration of berries from three distinct grapevine cultivars. Aust J Grape Wine Res. (2013) ;19: (2):171–9. |
[5] | Paniagua AC , East AR , Hindmarsh JP , Heyes JA . Moisture loss is the major cause of firmness change during postharvest storage of blueberry. Postharvest Biol Technol. (2013) ;79: :13–9. |
[6] | Basiouny FM , Woods F. Effect of chelated calcium on shelf-life and quality of blueberry fruits (Vaccinium ashei Reade). Proc Fla State Hort Soc. (1992) ;105: :75–8. |
[7] | Berkheimer EJ , Hanson SF . Effect of Soil Calcium Applications on Blueberry Yield and Quality. Small Fruits Rev Food Prod Press Haworth Press Inc. (2004) ;3: (1):133–9. |
[8] | Flores DA , Vázquez ME , Mildemberg JC , Beltrano J. Efecto de la fertilizatión cálcica sobre el rendimiento y tamaño de fruto en arándano alto del sur (Vaccinium corymbosum L.). Hortic Argentina. (2014) ;33: (81):31–6. |
[9] | Angeletti P , Castagnasso H , Miceli E , Terminiello L , Concellón A , Chaves A , . Effect of preharvest calcium applications on postharvest quality, softening and cell wall degradation of two blueberry (Vaccinium corymbosum) varieties. Postharvest Biol Technol. (2010) ;58: (2):98–103. |
[10] | Stückrath R , Quevedo R , De La Fuente L , Hernández A , Sepúlveda V. Effect of foliar application of calcium on the quality of blueberry fruits. J Plant Nutr. (2008) ;31: (7):1299–312. |
[11] | Tamada T . Stages of Rabbiteye and Highbush blueberry fruit developmente and the associated changes in mineral elements. Acta Hortic. (2002) ;574: :129–37. |
[12] | Balic I , Ejsmentewicz T , Sanhueza D , Silva C , Peredo T , Olmedo P , . Biochemical and physiological study of the firmness of table grape berries. Postharvest Biol Technol. (2014) ;93: :15–23. |
[13] | Perotti VE , Del Vecchio HA , Sansevich A , Meier G , Bello F , Cocco M , . Proteomic, metabalomic, and biochemical analysis of heat treated Valencia oranges during storage. Postharvest Biol Technol. (2011) ;62: (2):97–114. |
[14] | Perez-Palacios T , Barroso MA , Ruiz J , Antequera T. A rapid and accurate extraction procedure for analysing free amino acids in meat samples by GC-MS. Int J Anal Chem. (2015) ;2015. |
[15] | Che KU Dahlan-Dauc, Abdul Manan Mat Jais , Zuraini Ahmad , Abdah Md Akim and Aisha Adam . Amino and fatty acid compositions in Haruan traditional extract (HTE). Clin Exp Pharmacol Physiol. (2007) ;34: :198–204. |
[16] | Fish WW . A Reliable Methodology for Quantitative Extraction of Fruit and Vegetable Physiological Amino Acids and Their Subsequent Analysis with Commonly Available HPLC Systems. Food Nutr Sci. (2012) ;3: :863–71. |
[17] | Bradford MM . A rapid and sensitive method for the for the quantitation of microgram quantities of protein utilizing the principle of protein dye-binding. Anal Biochem. (1976) ;72: :248–54. |
[18] | Lionetti V . PECTOPLATE: The simultaneous phenotyping of pectin methylesterases, pectinases, and oligogalacturonides in plants during biotic stresses. Front Plant Sci. (2015) ;6. |
[19] | Pressey R . beta-Galactosidases in Ripening Tomatoes. Plant Physiol. (1983) ;71: (1):132–5. |
[20] | Velioglu YS , Mazza G , Gao L , Oomah BD . Antioxidant Activity and Total Phenolics in Selected Fruits, Vegetables, and Grain Products. J Agric Food Chem. (1998) ;46: (10):4113–7. |
[21] | Lyrene PM . “Emerald” southern highbush blueberry. Hort Science. (2008) ;43: :1606–7. |
[22] | Zapata LM , Malleret AD , Quinteros CF , Lesa CE , Vuarant CO , Rivadeneira MF , Gerard JA . Estudio sobre cambios de la firmeza de bayas de arándanos durante su maduración. Ciencia, Docencia y Tecnol. (2010) ;XXI: (41):159–71. |
[23] | Moggia C , Graell J , Lara I , González G , Lobos GA . Firmness at Harvest Impacts Postharvest Fruit Softening and Internal Browning Development in Mechanically Damaged and Non-damaged Highbush Blueberries (Vaccinium corymbosum L.). Front Plant Sci. (2017) ;8. |
[24] | Karppinen K , Zoratti L , Nguyenquynh N , Häggman H , Jaakola L. On the Developmental and Environmental Regulation of Secondary Metabolism in Vaccinium spp. Berries. Front Plant Sci. (2016) ;7. |
[25] | Lee S , Jung ES , Do SG , Jung GY , Song G , Song JM , . Correlation between species-specific metabolite profiles and bioactivities of blueberries (Vaccinium spp.). J Agric Food Chem. (2014) ;62: (9):2126–33. |
[26] | Howard LR , Clark JR , Brownmiller C. Antioxidant capacity and phenolic content in blueberries as affected by genotype and growing season. J Sci Food Agric. (2003) ;83: (12):1238–47. |
[27] | Vilanova M , Rodríguez I , Canosa P , Otero I , Gamero E , Moreno D , . Variability in chemical composition of Vitis vinifera cv Mencía from different geographic areas and vintages in Ribeira Sacra (NW Spain). Food Chem. (2015) ;169: :187–96. |
[28] | Manganaris GA , Goulas V , Vicente AR , Terry LA . Berry antioxidants: Small fruits providing large benefits. Journal of the Science of Food and Agriculture. (2014) ;94: :825–33. |
[29] | Yi W , Fischer J , Krewer G , Akoh CC . Phenolic compounds from blueberries can inhibit colon cancer cell proliferation and induce apoptosis. J Agric Food Chem. (2005) ;53: (18):7320–9. |
[30] | Whitaker JR , Voragen AGJ , Wong DWS . Hand book of food enzymology. Marcel Dekker, New York, NY, USA. (2003) ;993–1018. |
[31] | Keutgen AJ , Pawelzik E. Modifications of strawberry fruit antioxidant pools and fruit quality under NaCl stress. In: Journal of Agricultural and Food Chemistry. (2007) . pp. 4066–72. |
[32] | Boggio SB , Palatnik JF , Heldt HW , Valle EM . Changes in amino acid composition and nitrogen metabolizing enzymes in ripening fruits of Lycopersicon esculentum Mill. Plant Sci. (2000) ;159: (1):125–33. |
[33] | Lisec J , Schauer N , Kopka J , Willmitzer L , Fernie AR . Gas chromatography mass spectrometry-based metabolite profiling in plants. Nat Protoc. (2006) ;1: (1):387–96. |
[34] | Moreno AS , Perotti VE , Margarit E , Bello F , Vázquez DE , Podestá FE , Tripodi KEJ . Metabolic profiling and quality assessment during the postharvest of two tangor varieties subjected to heat treatments. Postharvest Biol Technol. (2018) ;142: :10–8. |
[35] | Perotti VE , Moreno AS , Trípodi KEJ , Meier G , Bello F , Cocco M , . Proteomic and metabolomic profiling of Valencia orange fruit after natural frost exposure. Physiol Plant. (2015) ;153: (3):337–54. |
[36] | Ashraf M , Harris PJC . Potential biochemical indicators of salinity tolerance in plants. Plant Sci. (2004) ;166: (1):3–16. |
[37] | Galili G , Hofgen R. Metabolic engineering of amino acids and storage proteins in plants. Metab Eng. (2002) ;4: (1):3–11. |
[38] | Lindemann B . Receptors and transduction in taste. Nature. (2001) ;413: :219–25. |
[39] | Keutgen AJ , Pawelzik E. Quality and nutritional value of strawberry fruit under long term salt stress. Food Chem. (2008) ;107: (4):1413–20. |
[40] | Belitz HD , Grosch W , Schieberle P. Belitz, H-D , Grosch W , Schieberle P. In: Lehrbuch der Lebensmittelchemie: 12 Aminosauren (5th ed) Berlin: Springer Verlag. (2001) . pp. 9–33. |
[41] | Kato H , Rhue M , Nishimura T. Role of free amino acids and peptides in food taste. In: Flavor Chemistry. (1989) . pp. 158–75. |
[42] | Kavi Kishor PB , Hima Kumari P , Sunita MSL , Sreenivasulu N. Role of proline in cell wall synthesis and plant development and its implications in plant ontogeny. Front Plant Sci. (2015) ;6: :544. |
[43] | Hijazi M , Roujol D , Nguyen-Kim H , Del Rocio Cisneros Castillo L , Saland E , Jamet E , . Arabinogalactan protein 31 (AGP31), a putative network-forming protein in Arabidopsis thaliana cell walls? Ann Bot. (2014) ;114: (6):1087–97. |
[44] | Etienne A , Génard M , Lobit P , Mbeguié-A-Mbéguié D , Bugaud C. What controls fleshy fruit acidity? A review of malate and citrate accumulation in fruit cells. Journal of Experimental Botany. (2013) ;64: :1451–69. |
[45] | Conde A , Regalado A , Rodrigues D , Costa JM , Blumwald E , Chaves MM , . Polyols in grape berry: Transport and metabolic adjustments as a physiological strategy for water-deficit stress tolerance in grapevine. J Exp Bot. (2015) ;66: (3):889–906. |
[46] | Hu H , Penn SG , Lebrilla CB , Brown PH . Isolation and Characterization of Soluble Boron Complexes in Higher Plants (The Mechanism of Phloem Mobility of Boron). Plant Physiol. (1997) ;113: (2):649–55. |
[47] | Liakopoulos G , Stavrianakou S , Filippou M , Fasseas C , Tsadilas C , Drossopoulos I , . Boron remobilization at low boron supply in olive (Olea europaea) in relation to leaf and phloem mannitol concentrations. Tree Physiol. (2005) ;25: (2):157–65. |
[48] | Krasensky J , Jonak C. Drought, salt, and temperature stress-induced metabolic rearrangements and regulatory networks. Journal of Experimental Botany. (2012) ;63: :1593–608. |
[49] | Joshi V , Fernie AR . Citrulline metabolism in plants. Amino Acids. (2017) ;49: :1543–59. |
[50] | Akashi K , Mifune Y , Morita K , Ishitsuka S , Tsujimoto H , Ishihara T. Spatial accumulation pattern of citrulline and other nutrients in immature and mature watermelon fruits. J Sci Food Agric. (2017) ;97: (2):479–87. |
[51] | Sinha AK , Hofmann MG , Romer U , Kockenberger W , Elling L , Roitsch T. Metabolizable and non-metabolizable sugars activate different signal transduction pathways in tomato. Plant Physiol. (2002) ;128: (4):1480–9. |
[52] | Jia H , Wang Y , Sun M , Li B , Han Y , Zhao Y , . Sucrose functions as a signal involved in the regulation of strawberry fruit development and ripening. New Phytol. (2013) ;198: (2):453–65. |
[53] | Tarkowski LP , Van den Ende W . Cold tolerance triggered by soluble sugars: A multifaceted countermeasure. Front Plant Sci. (2015) ;6. |
[54] | Lastdrager J , Hanson J , Smeekens S. Sugar signals and the control of plant growth and development. J Exp Bot. (2014) ;65: (3):799–807. |
[55] | Ruan Y-L . Sucrose Metabolism: Gateway to Diverse Carbon Use and Sugar Signaling. Annu Rev Plant Biol. (2014) ;65: (1):33–67. |
[56] | Back K , Tan DX , Reiter RJ . Melatonin biosynthesis in plants: Multiple pathways catalyze tryptophan to melatonin in the cytoplasm or chloroplasts. Journal of Pineal Research. (2016) ; 61: :426–37. |
[57] | Zhao Y . Auxin biosynthesis: A simple two-step pathway converts tryptophan to indole-3-Acetic acid in plants. In: Molecular Plant. (2012) . pp. 334–8. |
[58] | Staswick P . Plant hormone conjugation: A signal decision. Plant Signal Behav. (2009) ;4: (8):757–9. |
[59] | Clifford MN . Chlorogenic acids and other cinnamates - Nature, occurrence, dietary burden, absorption and metabolism. Journal of the Science of Food and Agriculture. (2000) ;80: :1033–43. |
[60] | Vicente AR , Saladie M , Rose JKC , Labavitch JM . The linkage between cell wall metabolism and fruit softening: Looking to the future. Journal of the Science of Food and Agriculture. (2007) ;87: :1435–48. |
[61] | Rose JKC . Temporal Sequence of Cell Wall Disassembly in Rapidly Ripening Melon Fruit. Plant Physiol. (1998) ;117: (2):345–61. |
[62] | Rose JKC , Braam J , Fry SC , Nishitani K. The XTH family of enzymes involved in xyloglucan endotransglucosylation and endohydrolysis: Current perspectives and a new unifying nomenclature. Plant and Cell Physiology. (2002) ;43: :1421–35. |
[63] | Brummell DA , Harpster MH . Cell wall metabolism in fruit softening and quality and its manipulation in transgenic plants. Plant Molecular Biology. (2001) ;47: :311–40. |
[64] | Hocking B , Tyerman SD , Burton RA , Gilliham M. Fruit Calcium: Transport and Physiology. Front Plant Sci. (2016) ;7. |
[65] | Chandrasekar B , van der Hoorn RAL. Beta galactosidases in Arabidopsis and tomato-a mini review. Biochem Soc Trans. (2016) ;44: (1):150–8. |
[66] | Popper ZA , Fry SC . Widespread occurrence of a covalent linkage between xyloglucan and acidic polysaccharides in suspension-cultured angiosperm cells. Ann Bot. (2005) ;96: (1):91–9. |
[67] | Zykwinska AW . Evidence for In Vitro Binding of Pectin Side Chains to Cellulose. PLANT Physiol. (2005) ;139: (1):397–407. |
[68] | Redgwell R , Melton L , Brasch D. Cell Wall Dissolution in Ripening Kiwifruit (Actinidia deliciosa) Solubilization of the Pectic Polymers. Plant Physiol. (1992) ;98: (1):71–81. |
[69] | Balic I , Vizoso P , Nilo-Poyanco R , Sanhueza D , Olmedo P , Sepulveda P , . Transcriptome analysis during ripening of table grape berry cv. Thompson Seedless. PLoS One. (2018) ;13: (1). |
[70] | Jolie RP , Duvetter T , Van Loey AM , Hendrickx ME . Pectin methylesterase and its proteinaceous inhibitor: A review. Carbohydrate Research. (2010) ;345: :2583–95. |
[71] | Brummell D . Primary cell wall metabolism during fruit ripening. New Zeal J For Sci. (2006) ;36: (1):99–111. |
[72] | Ng JKT , Schröder R , Brummell DA , Sutherland PW , Hallett IC , Smith BG , . Lower cell wall pectin solubilisation and galactose loss during early fruit development in apple (Malus x domestica) cultivar “Scifresh” are associated with slower softening rate. J Plant Physiol. (2015) ;176: :129–37. |
[73] | Fernandez O , Urrutia M , Bernillon S , Giauffret C , Tardieu F , Le Gouis J , . Fortune telling: Metabolic markers of plant performance. Metabolomics. (2016) ;12: (158):1–14. |
[74] | Lopez MG , Zanor MI , Pratta GR , Stegmayer G , Boggio SB , Conte M , . Metabolic analyses of interspecific tomato recombinant inbred lines for fruit quality improvement. Metabolomics. (2015) ;11: (5):1416–31. |
[75] | Centeno DC , Osorio S , Nunes-Nesi A , Bertolo ALF , Carneiro RT , Araujo WL , . Malate Plays a Crucial Role in Starch Metabolism, Ripening, and Soluble Solid Content of Tomato Fruit and Affects Postharvest Softening. Plant Cell. (2011) ;23: (1):162–84. |