In vitro screening and evaluation of phenolic antioxidant-linked anti-hyperglycemic functions of rabbit-eye blueberry (Vaccinium ashei) cultivars
Abstract
BACKGROUND:
Higher consumption of phenolic-antioxidant rich berries have been associated with lower risks of chronic diseases, such as type 2 diabetes. In order to target for diet-based therapy it is important to screen and evaluate the right cultivars of berries with optimum phenolic antioxidants and associated anti-hyperglycemic functions.
OBJECTIVE:
Therefore, the primary objective of this study was to screen different rabbit-eye blueberry cultivars extracted in water and ethanol for their phenolic antioxidant-linked anti-diabetic properties using in vitro assays. Further impact of cultivars, growing seasons, and locations on targeted human health relevant bioactive profiles were also evaluated.
METHODS:
Water and ethanol extracts of five rabbit-eye blueberry cultivars from two different locations and from two different growing seasons were evaluated for phenolic acids profile, total soluble phenolic content, total antioxidant activity, α-amylase, α-glucosidase, and angiotensin I-converting enzyme (ACE) inhibitory activities using model in vitro assays.
RESULTS:
Significant variations in phenolic antioxidant-linked anti-hyperglycemic functions were observed due to differences in genotypes, growing seasons, and locations. High phenolic antioxidant-linked anti-hyperglycemic functions were observed in Tiffblue, Brightwell, and Primier rabbit-eye blueberry cultivars and indicated anti-diabetic potential.
CONCLUSION:
Genotype×environment interactions are most critical factors which determined in vitro anti-diabetic relevant functionalities of blueberry bioactives.
1Introduction
Blueberries are rich source of dietary fiber, essential micronutrients, and phenolic antioxidants with diverse human health relevant biological activities and functions [1, 2]. Most specifically, blueberries have been known to offer a natural and abundant source of hydrophilic antioxidants such as anthocyanin and other phenolic acids [3]. These natural phenolic antioxidants from plant-based foods are becoming important targets for safe and cost-effective dietary support therapy for oxidative stress-linked non-communicable chronic diseases (NCDs), like type 2 diabetes, cardiovascular disease, and cancer [4–6]. Due to such rich bioactive profile and associated health benefits, blueberries (fresh or frozen) have been highly sought healthy food choice amongconsumers.
The market opportunity of blueberry is rapidly expanding from its geographic origin of America with 10% of the total United States highbush blueberry being exported each year [7]. In the United States, total production of blueberry whether cultivated or wild, in 2014 was around 667.6 million pounds with estimated market value of $824.9 million [8]. Blueberry industry is projecting 25% increase in North American production in next three years with 50% increase in per capita blueberry consumption [7]. There are three different major types of blueberry grown in the United States such as highbush, lowbush, and rabbit-eye with many cultivated varieties from each type being developed in the last few decades for different regions and climatic conditions. Among these blueberry types, hexaploid rabbit-eye blueberries (Vaccinium ashei) are native to the Southeastern United States with lower chilling requirement. Rabbit-eye blueberries are also most adaptable, productive, and resilient against biotic stresses (insects and pathogens), especially under the warmer climate [9]. Due to this wider adaptability and higher resilience against biotic and abiotic stresses, rabbit-eye blueberry cultivation is expanding globally with increased production in South America, China and Australia[10–12].
Blueberry antioxidant has shown protective functions against chronic oxidative stress associated with NCDs, such as type 2 diabetes, and cardiovascular diseases [13, 14]. Recent studies indicated that phenolic antioxidants from berries also influence glucose and lipid metabolism [14, 15]. Phenolic acids and their metabolites from plant-based foods potentially influence digestion, absorption, and metabolism of dietary carbohydrates such as starch and sucrose and therefore control postprandial blood glucose level [15]. For maintaining glucose homeostasis, α-glucosidase and α-amylase are extremely important enzymes that regulate the rate in which starch are digested and subsequently absorbed as glucose in the intestine [15]. The main function of pancreatic α-amylase is by catalyzing hydrolysis of 1,4-glucosidic bonds, resulting in the release of maltose [15]. Similarly, α-glucosidase, an enzyme found in the small intestine, hydrolyzes the 1,4-linked glucose residues in order to further digest carbohydrates to release glucose [16], before immediate absorption by the intestinal cells. By inhibiting these enzymes, carbohydrate digestion can be slowed in its passage in the intestinal tract resulting in delayed glucose absorption [17]. Previous studies have indicated that berries with highest anthocyanin content, such as blueberry and black currant, exhibited far better inhibition of α-glucosidase than strawberries and red raspberry extracts [18–20]. Inhibition of α-amylase and α-glucosidase have proven effective against early onset of type 2 diabetes and associated complications [19, 20]. But pharmaceutical drugs such as Acarbose with inhibitory activities against these enzymes can cause unwanted side effects including flatulence, abdominal discomfort, and diarrhea due to high levels of α-amylase inhibitory activity [21]. Therefore, finding natural plant-based food sources such as berries with moderate α-amylase and high α-glucosidase inhibitory activities are gaining increasing attention for safe and cost-effective dietary management of early stages type2 diabetes.
Although berries and berry phenolics have shown high baseline values of α-amylase and α-glucosidase inhibitory activities both in previous in vitro and in vivo studies [14, 15, 18, 19], but these phenolic antioxidant-linked anti-hyperglycemic functions associated with anti-diabetic potential vary widely among different genotypes, climate conditions, harvesting methods, maturity, storage conditions, and processing system [22–24]. While phenolic antioxidant-linked anti-hyperglycemic properties of blueberry is understood well [24], more studies are needed to compare the impact of cultivars, growing seasons, and growing locations on these human health relevant properties. Therefore, the major aim of this study was to screen and evaluate impact of genotypes, growing seasons, and locations on the phenolic antioxidant-linked anti-hyperglycemic functions of rabbit-eye blueberry using rapid in vitro assay models.
2Materials and methods
2.1Materials
A total of five rabbit-eye blueberry cultivars; Tifblue, Climax, Pimier, Brightwell, and Baldwin were obtained from the Auburn University (grown in two locations 245 miles apart: Dothan, AL 36302 and Cullman, AL 35055 for 2009, and 2010). Both Dothan and Cullman have a humid sub-tropical climate with average annual rainfall of 143–146 cm with slightly cooler average night temperature in Cullman. Dothan has fine-loamy soil, while Cullman has sandstone mixed with alluvial soil. Due to disease infestation no fruits of Baldwin blueberry cultivars were collected from Dothan in both years. Frozen sample (4 months after harvest) of blueberry fruits were shipped to the Department of Food Science, University of Massachusetts, Amherst, MA 01002 in ice and then biochemical analysis was carried out in 2010 and 2011 for the respective collection years. Porcine pancreatic a-amylase (EC 3.2.1.1), baker’s yeast glucosidase (EC 3.2.1.20), 2, 2-diphenyl-1-picrylhydrazyl (DPPH), and 3,5-Dinitrosalicylic acid (DNS) were purchased from Sigma Chemical Co. (St. Louis, MO). All other chemicals and solvents were purchased from Sigma Chemical Co. (St. Louis, MO) and of analytical grade.
2.2Preparation of blueberry extracts
Well ripened and frozen blueberry fruits from five cultivars: Tifblue, Climax, Pimier, Brightwell, and Baldwin were selected. Upon even thawing, 40 g of whole blueberry fruits were added to 100 mL of either distilled water to reflect physiological relevance and water-based foods and beverages or 12% ethanol to reflect typical alcoholic beverage levels and homogenized for 5 minutes using a Waring laboratory blender (Winsted, CN) set on HIGH. Two replications were used for each extraction. The homogenate was then collected after two time centrifugation at 10,000 g for 20 min. The supernatant was then transferred into 50 mL Eppendorf tubes and refrigerated for all in vitro assays.
2.3Phenolic acid profiles (HPLC)
The blueberry sample (0.4 g/mL) extracts (2 mL) were filtered through a 0.2 μm filter. A volume of 5 μL of sample was injected using an Agilent ALS 1100 autosampler into an Agilent 1100 series HPLC (Agilent Technologies, Palo Alto, CA) equipped with a DAD 1100 diode array detector. The solvents used for gradient elution were (A) 10 mM phosphoric acid (pH 2.5) and (B) 100% methanol. The methanol concentration was increased to 60% for the first 8 min and to 100% over the next 7 min, then decreased to 0% for the next 3 min and was maintained for the next 7 min (total run time, 25 min). The analytical column used was Agilent Zorbax SB-C18, 250 - 4.6 mm i.d., with packing material of 5 μm particle size at a flow rate of 1 mL/min at room temperature. During each run the absorbance was recorded at 306 nm and 333 nm and the chromatogram was integrated using Agilent Chem station enhanced integrator. Pure standards of chlorogenic acid, protocatechuic acid, gallic acid, ellagic acid and quercetin in 100% methanol were used to calibrate the standard curves and retention times.
2.4Total soluble phenolics assay
The total soluble phenolic content was determined using Folin–Ciocalteu method, modified by Shetty et al. [25]. Blueberry sample (0.4 g/mL) extract (0.5 mL) and 0.5 mL of distilled water were transferred into a test tube and mixed with 1 mL of 95% ethanol, 5 mL of distilled water, 0.5 mL of 50% (vol/vol) Folin–Ciocalteu, and 1 mL of 5% Na2CO3 and the reaction mixture allowed to stand for 60 min. The absorbance was read at 725 nm and results were expressed as mg of gallic acid equivalent per gram of sample in fresh weight (FW) basis.
2.5Antioxidant activity by 2, 2-diphenyl-1-picrylhydrazyl radical (DPPH) inhibition assay
The DPPH scavenging activity was determined by an assay method modified from Pinto et al. [26]. 1.25 mL of 60 μM DPPH in 95% ethanol was added to 250 μL of each sample extract (0.4 g/mL), with the decrease in the absorbance monitored after 5 min at 517 nm. The absorbance of a control (distilled water instead of sample extract) was also recorded after 5 min at the same wavelength. The percentage of inhibition was then calculated by the following equation:
2.6a-Amylase inhibition assay
The α-amylase inhibitory activity was determined using an assay method modified from the Worthington Enzyme Manual [26]. A total of 500 μL of each blueberry sample extract (0.4 g/mL) and 500 μL of 0.02 M sodium phosphate buffer (pH 6.9 with 0.006 M NaCl) containing α-amylase solution (0.5 mg/mL) were incubated at 25°C for 10 min. After pre-incubation, 500 μL of a 1% starch solution in 0.02 M sodium phosphate buffer (pH 6.9 with 0.006 M NaCl) was added to each tube and then incubated at 25°C for 10 min. Next, 1.0 mL of dinitrosalicylic acid color reagent was added. The test tubes were then incubated in a boiling water bath for 10 min and absorbance was measured at 540 nm after cooling and dilution. Additionally, a 1:2 and 1:5 dilution of the sample extract was performed to investigate potential dose dependence. The a-amylase inhibitory activity was calculated according to the equation below:
2.7a-Glucosidase inhibition assay
The assay method is modified from the Worthington Enzyme Manual for a-glucosidase inhibitory activity [26]. A volume of 50 μL of blueberry sample extract (0.4 g/mL) diluted with 50 μL of 0.1 M potassium phosphate buffer (pH 6.9) and 100 μL of 0.1 M potassium phosphate buffer (pH 6.9) containing glucosidase solution (1.0 U/mL) was incubated in 96-well plates at 25°C for 10 min. After pre-incubation, 50 μL of 5 mM p-nitrophenyl- a-D-glucopyranoside solution in 0.1 M potassium phosphate buffer (pH 6.9) was added to each well at timed intervals. The reaction mixtures were incubated at 25°C for 5 min. Before and after incubation, absorbance readings (A405 extract) were recorded at 405 nm by a microplate reader (Thermomax; Molecular Devices Co., Sunnyvale, CA) and compared to a control which had 50 μL of buffer solution in place of the extract (A405 control). The a-glucosidase inhibitory activity was expressed as a percentage of inhibition and calculated by the equation described in 2.5 section.
2.8Angiotensin I-converting enzyme (ACE) inhibition assay
ACE inhibitory activity was determined using an assay method modified by Kwon et al. [27]. A volume of 50 μL of blueberry sample extract (0.4 g/mL) was incubated with 200 μL of 0.1 M NaCl-borate buffer (0.3 M NaCl, pH 8.3) containing 2 mU of ACE solution at 25°C for 10 min. After pre-incubation, 100 μL of a 5.0 mM substrate (hippuryl-histidyl-leucine) solution was added to the reaction mixture. Test solutions were incubated at 37°C for 1 h. Sample blanks (buffer in place of enzyme and substrate), a control (distilled water instead of sample extract) and a blank (buffer instead of sample extract and enzyme) were also included. The reaction was stopped with 150 μL of 0.5 N HCl. The hippuric acid formed was detected by the High Performance Liquid Chromatography (HPLC) method [27]. The percentage of inhibition was calculated considering the area of the hippuric acid peak according to the equation below:
2.9Statistical analysis
Two extractions were performed for each sample and all in vitro assays were replicated twice (n = 12). Results were calculated using Microsoft Excel XP and expressed as a mean with±standard deviation. All data were analyzed using multivariate analysis (SAS, 9.4 version) and least significant differences were calculated using Tukey’s test (p < 0.05).
3Results and discussion
3.1HPLC analysis of phenolic acids profile
Phenolic acids profile provides significant information on potential biological functions and human health relevant properties in plant-based foods including berries [28]. The major phenolic acids found in this study were catechin, gallic acid, protocatechuic acid, and quercetin (Table 1). Similar composition of phenolic acids were observed in previous studies with blueberry cultivars [19, 24, 29]. Protocatechuic acid was not detected in rabbit-eye blueberry cultivars such as Brightwell and Baldwin. Therefore phenolic acids composition of blueberries significantly varies with the choice of cultivars. Among phenolic acids catechin was most prominent phenolics followed by quercetin and protocatechuic acids detected in this study. Ethanol extracts (12%) of blueberry fruits had significantly higher phenolic acids profile when compared with the water extracts of same blueberry cultivar. Similar effect of extraction solvents on phenolic acids profile of blueberry was observed in a previous study [30]. Overall, higher concentrations of phenolic acids were also observed in rabbit-eye blueberry fruits grown in year 1. Therefore, growing and other environmental conditions also have significant impacts on phenolic acids profile of blueberries. This study suggests that blueberry has rich phenolic acid profile with significant biological activities and functions, including human health relevant properties.
Table 1
Phenolic acid content (ug/ mL) of water (A) and ethanol (B) extracts of five blueberry cultivars from two different locations (Cullman and Dothan) and two growing seasons
A | Catechin | Gallic acid | Protocatechuic acid | Quercetin | ||||
Year 1 | Year 2 | Year 1 | Year 2 | Year 1 | Year 2 | Year 1 | Year 2 | |
Tiffblue | ||||||||
Cullman | 0.326 | 0.301 | 0.014 | 0.012 | 0.145 | 0.121 | 0.108 | 0.112 |
Dothan | 0.282 | 0.245 | 0.006 | 0.011 | 0.132 | 0.118 | 0.097 | 0.084 |
Climax | ||||||||
Cullman | 0.345 | 0.285 | 0.011 | 0.009 | 0.099 | 0.086 | 0.102 | 0.091 |
Dothan | 0.302 | 0.295 | 0.007 | 0.005 | 0.084 | 0.080 | 0.094 | 0.080 |
Primier | ||||||||
Cullman | 0.304 | 0.280 | 0.004 | 0.006 | 0.103 | 0.094 | 0.106 | 0.108 |
Dothan | 0.271 | 0.245 | 0.004 | 0.003 | 0.085 | 0.080 | 0.092 | 0.091 |
Brightwell | ||||||||
Cullman | 0.452 | 0.356 | 0.013 | 0.009 | n.d | n.d | 0.129 | 0.121 |
Dothan | 0.325 | 0.218 | 0.011 | 0.010 | n.d | n.d | 0.118 | 0.108 |
Baldwin | ||||||||
Cullman | 0.314 | 0.285 | 0.016 | 0.014 | n.d | n.d | 0.112 | 0.100 |
B | Catechin | Gallic acid | Protocatechuic acid | Quercetin | ||||
Year 1 | Year 2 | Year 1 | Year 2 | Year 1 | Year 2 | Year 1 | Year 2 | |
Tiffblue | ||||||||
Cullman | 0.385 | 0.364 | 0.003 | 0.002 | 0.176 | 0.165 | 0.121 | 0.114 |
Dothan | 0.370 | 0.312 | 0.004 | 0.003 | 0.168 | 0.158 | 0.103 | 0.105 |
Climax | ||||||||
Cullman | 0.345 | 0.301 | 0.005 | 0.003 | 0.152 | 0.160 | 0.110 | 0.099 |
Dothan | 0.322 | 0.289 | 0.002 | 0.004 | 0.143 | 0.137 | 0.085 | 0.086 |
Primier | ||||||||
Cullman | 0.296 | 0.245 | 0.005 | 0.003 | 0.145 | 0.124 | 0.098 | 0.097 |
Dothan | 0.262 | 0.231 | 0.004 | 0.005 | 0.131 | 0.126 | 0.086 | 0.084 |
Brightwell | ||||||||
Cullman | 0.421 | 0.413 | 0.016 | 0.012 | n.d | n.d | 0.129 | 0.131 |
Dothan | 0.398 | 0.367 | 0.017 | 0.009 | n.d | n.d | 0.110 | 0.105 |
Baldwin | ||||||||
Cullman | 0.365 | 0.359 | 0.006 | 0.005 | n.d | n.d | 0.134 | 0.113 |
3.2Total soluble phenolic content
Total soluble phenolic content of five rabbit-eye blueberry cultivars was determined using Folin-Ciocalteu method [25]. Significant variations in total soluble phenolic content was observed among five rabbit-eye blueberry cultivars, between two locations, two growing seasons, and cultivar×location×growing season interactions (p < 0.05) (Fig. 1A, B). Significantly higher total soluble phenolic content was also observed in ethanol extracts (12%) of rabbit-eye blueberry when compared with water extracts of the same cultivar. Among rabbit-eye blueberry cultivars higher total soluble phenolic content was observed in Tiffblue (0.8–1.3 mg/g F.W.) followed by Brightwell (0.6–1.3 mg/g F.W.) and Primier (0.48–1.19 mg/g F.W.). In all rabbit-eye blueberry cultivars, significantly higher total soluble phenolic content was also observed in year 1 (first growing season) when compared with year 2 (second growing season). In both locations (Cullman and Dothan), average monthly temperature was lower in year 1, while average precipitation before harvest was higher in year 1. Variations in temperature and precipitation may have contributed to the differences in baseline value of total soluble phenolic content of rabbit-eye blueberry cultivars in two different growing seasons. These results suggest that environmental conditions influencing phenotypic factors may have significant impact on phenolic content of rabbit-eye blueberry cultivars. Howard et al. [31] also reported significant impact of genotypes and growing seasons on phenolic content of 13 highbush blueberry cultivars. Similarly in another study, Connor et al. [32] observed significant variations in phenolic content, anthocyanin content, and antioxidant capacity among different highbush blueberry genotypes in three different locations and in two different growing seasons. Similar to the impact of growing seasons, different locations (Dothan and Cullman) also had significant impact on total soluble phenolic content of rabbit-eye blueberry cultivars. Water extracts of rabbit-eye blueberry grown in Cullman had significantly higher total soluble phenolic content when compared with the same cultivars grown in Dothan. In general, Dothan has slightly warmer climate than Cullman and that might have contributed to the lower level of phenolic content of rabbit-eye blueberry fruits from this location. These results suggest that genotype and environmental interaction is critical in modulating the level of phenolic content in blueberry cultivars. Similar impacts of genotype×environment interaction on phenolic bioactives have been reported in other berry cultivars [33].
Fig.1
Total soluble phenolic content (mg/g F.W.) of water (A) and ethanol (B) extracts of five different rabbit-eye blueberry cultivars grown in two locations (Dothan & Cullman) and in two growing seasons. Different capital letter represents significant differences between cultivar×location×growing season interactions at p < 0.05 probability level.
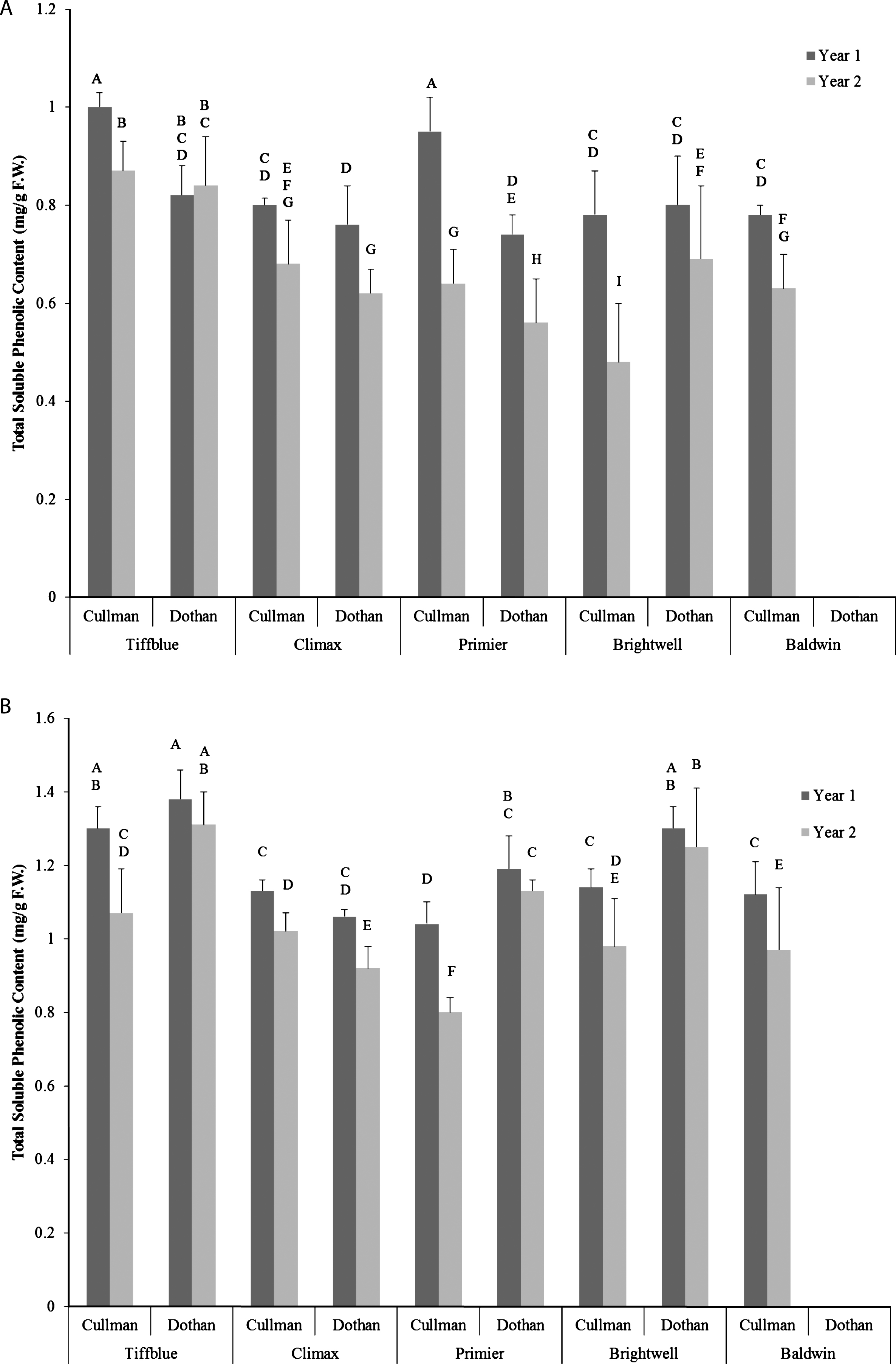
3.3Total antioxidant activity by DPPH radical scavenging assay
Blueberries are rich source of natural antioxidants and many health benefits of blueberry have been attributed to this characteristic [34]. Therefore to understand the potential role of rabbit-eye blueberry cultivars to counter oxidative stress-induced human diseases, antioxidant activity was determined using DPPH free radical scavenging assay. Similar to the total soluble phenolic content significant variations in total antioxidant activity was observed among cultivars, between two growing seasons, two locations, and cultivar×location×growing season interactions (p < 0.05) (Fig. 2A, B). Like total soluble phenolic content, higher total antioxidant was also observed in Tiffblue (66–82% DPPH inhibition) and Brightwell (65–81% DPPH inhibition) rabbit-eye blueberry cultivars. Overall, higher total antioxidant activity was also observed in rabbit-eye blueberries from year 1 when compared with year 2, with only exception being Tiffblue grown in Dothan. Similar findings of genotypes and growing seasons on antioxidant activity of blueberry cultivars have been reported previously [31, 32]. Ethanol extracts (12%) of rabbit-eye blueberry fruits had significantly higher total antioxidant activity when compared with the water extracts of same cultivars. Antioxidant activity of blueberry cultivars also positively correlated with total soluble phenolic content and therefore indicated a phenolic-linked antioxidant function in these rabbit-eye blueberry cultivars (Table 2). Overall, all five rabbit-eye blueberry cultivars had moderate to high antioxidant activity. Similar levels of DPPH radical scavenging activity was reported in blueberries in previous studies [24]. Hyperglycemia has been reported to cause increased production of reactive oxygen species through glucose auto-oxidation and non-enzymatic glycation process [35]. Therefore dietary therapy to manage early stages of type 2 diabetes linked to hyperglycemia needs to include antioxidant enriched foods and food ingredients to counter chronic oxidative and inflammatory states commonly experienced in diabetic condition [36]. Thisin vitro screening study suggests that rabbit-eye blueberry cultivars such as Tiffblue and Brightwell can be targeted for further clinical or epidemiological studies to develop and advance improved dietary strategies to manage oxidative stress commonly associated with NCDs including early stages type 2 diabetes that is associated with hyperglycemia.
Fig.2
Total antioxidant activity (DPPH % inhibition) of water (A) and ethanol (B) extracts of five different rabbit-eye blueberry cultivars grown in two locations (Dothan & Cullman) and in two growing seasons. Different capital letter represents significant differences between cultivar×location×growing season interactions at p < 0.05 probability level.
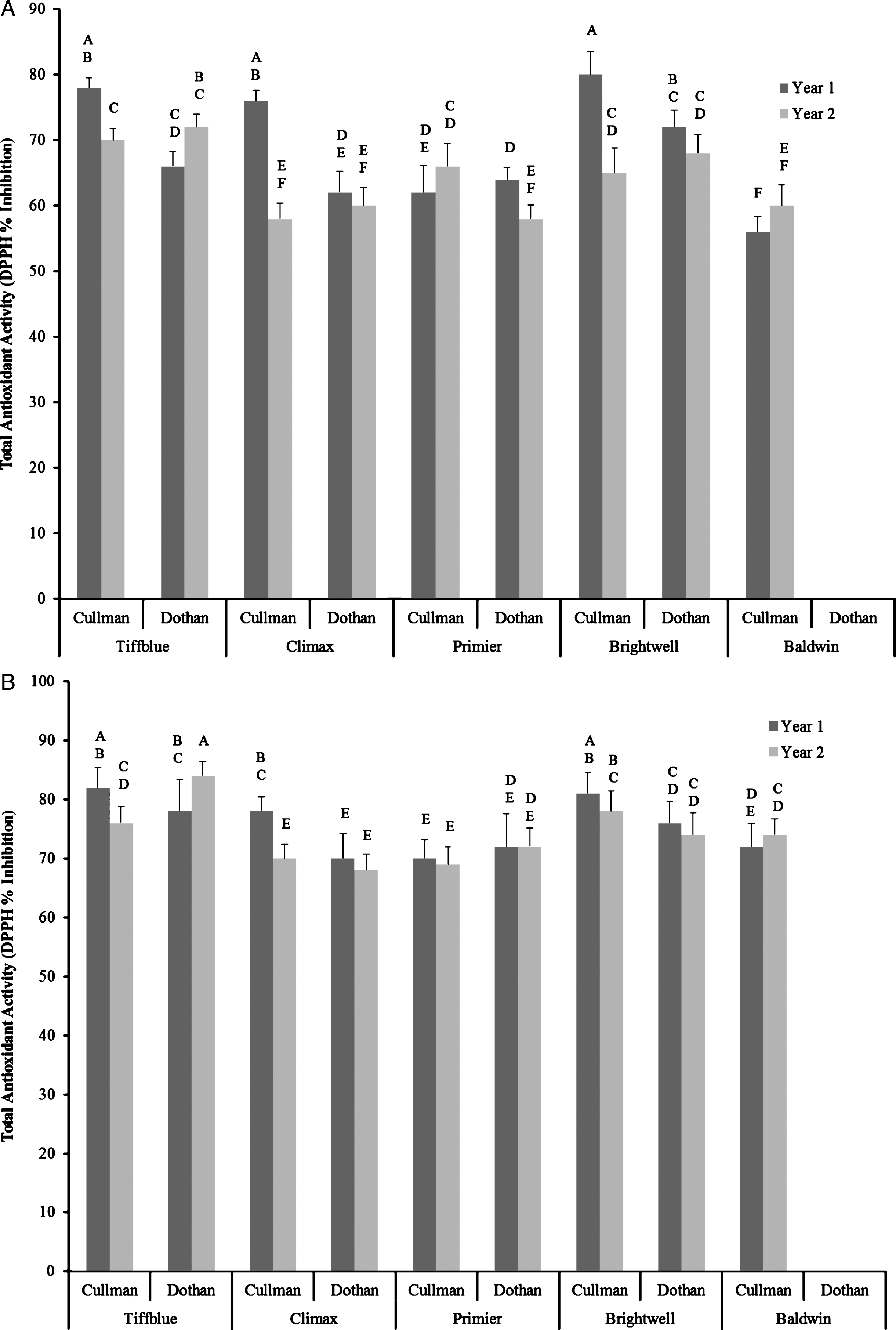
Table 2
Correlation coefficient (r) between phenolic content, antioxidant activity, α-amylase, and α-glucosidase enzyme inhibitory activity of water (WT) and 12% ethanol (ET) extracts of five rabbit-eye blueberry cultivars from two different locations (Dothan and Cullman) and from two growing seasons
WT | DPPH | α-amylase | α-glucosidase | ||
Without Dilution | ½ Dilution | Without Dilution | ½ Dilution | ||
TSP | 0.91 | 0.87 | 0.88 | 0.89 | 0.93 |
DPPH | 0.94 | 0.93 | 0.96 | 0.97 | |
α-amylase | 0.90 | 0.98 | 0.95 | ||
(Without Dilution) | |||||
α-amylase | 0.93 | 0.92 | |||
(1/2 dilution) | |||||
α-glucosidase | 0.97 | ||||
(Without Dilution) | |||||
ET | DPPH | α-amylase | α-glucosidase | ||
Without Dilution | ½ Dilution | Without Dilution | ½ Dilution | ||
TSP | 0.95 | 0.92 | 0.82 | 0.92 | 0.86 |
DPPH | 0.98 | 0.90 | 0.97 | 0.95 | |
α-amylase | 0.89 | 0.98 | 0.95 | ||
(Without Dilution) | |||||
α-amylase | 0.93 | 0.93 | |||
(1/2 dilution) | |||||
α-glucosidase | 0.97 | ||||
(Without Dilution) |
3.4α-Amylase and α-glucosidase inhibitory activities
Cooperation between α-glucosidase and α-amylase is crucial in the control of the postprandial hyperglycemic state in diabetic individuals [16]. Inhibiting α-glucosidase can result in a delayed breakdown of starch and absorption of glucose in the small intestine, which aids in the controlling of the postprandial increase in glucose. Alpha-amylase plays a similar initial role in controlling the rate at which starches are digested into shorter chain length rather than how they are broken and absorbed in the final step as glucose. Therefore, to understand the potential anti-hyperglycemic functions of rabbit-eye blueberry cultivars for α-glucosidase and α-amylase inhibitory activities was determined using in vitro assay models.
Overall, significantly high α-amylase and α-glucosidase inhibitory activity was observed in all undiluted water and ethanol extracts of rabbit-eye blueberry cultivars, irrespective of the growing seasons, locations, and extraction methods. Due to high baseline value (98–100% inhibition) of α-amylase and α-glucosidase inhibitory activity (78–96%) in undiluted water and ethanol extracts, no significant differences was observed among cultivars, growing seasons, and locations and therefore data of undiluted sample was not presented. When diluted in half significant variations in α-amylase inhibitory activity among rabbit-eye blueberry cultivars, locations, growing seasons, and cultivar×location×growing season interactions were observed (Fig. 3A, B). Similar to total soluble phenolic content and antioxidant activity, significantly higher α-amylase inhibitory activity was observed in Tiffblue and Brightwell rabbit-eye blueberry cultivars. Rabbit-eye blueberry cultivars grown in year 1 and in Cullman location was also resulted in higher α-amylase inhibitory activity when compared with rabbit-eye blueberries grown in year 2 and in Dothan locations. This result indicates that α-amylase inhibitory activity of rabbit-eye blueberry cultivars has positive correlations with total soluble phenolic content and total antioxidant activities (Table 2). Therefore, the α-amylase inhibitory activity of rabbit-eye blueberry cultivars may bedependent of phenolic antioxidant profiles and has significant relevance to potentially improve glucose metabolism when consumed as a diet and provides strong biochemical rationale for further clinical studies. Similar levels of α-amylase inhibitory activity in berries were reported in previous studies [19, 20, 24, 26].
Fig.3
Alpha-amylase inhibitory activity (%) of half-diluted water (A) and ethanol (B) extracts of five different rabbit-eye blueberry cultivars grown in two locations (Dothan & Cullman) and in two growing seasons. Different capital letter represents significant differences between cultivar×location×growing season interactions at p < 0.05 probability level.
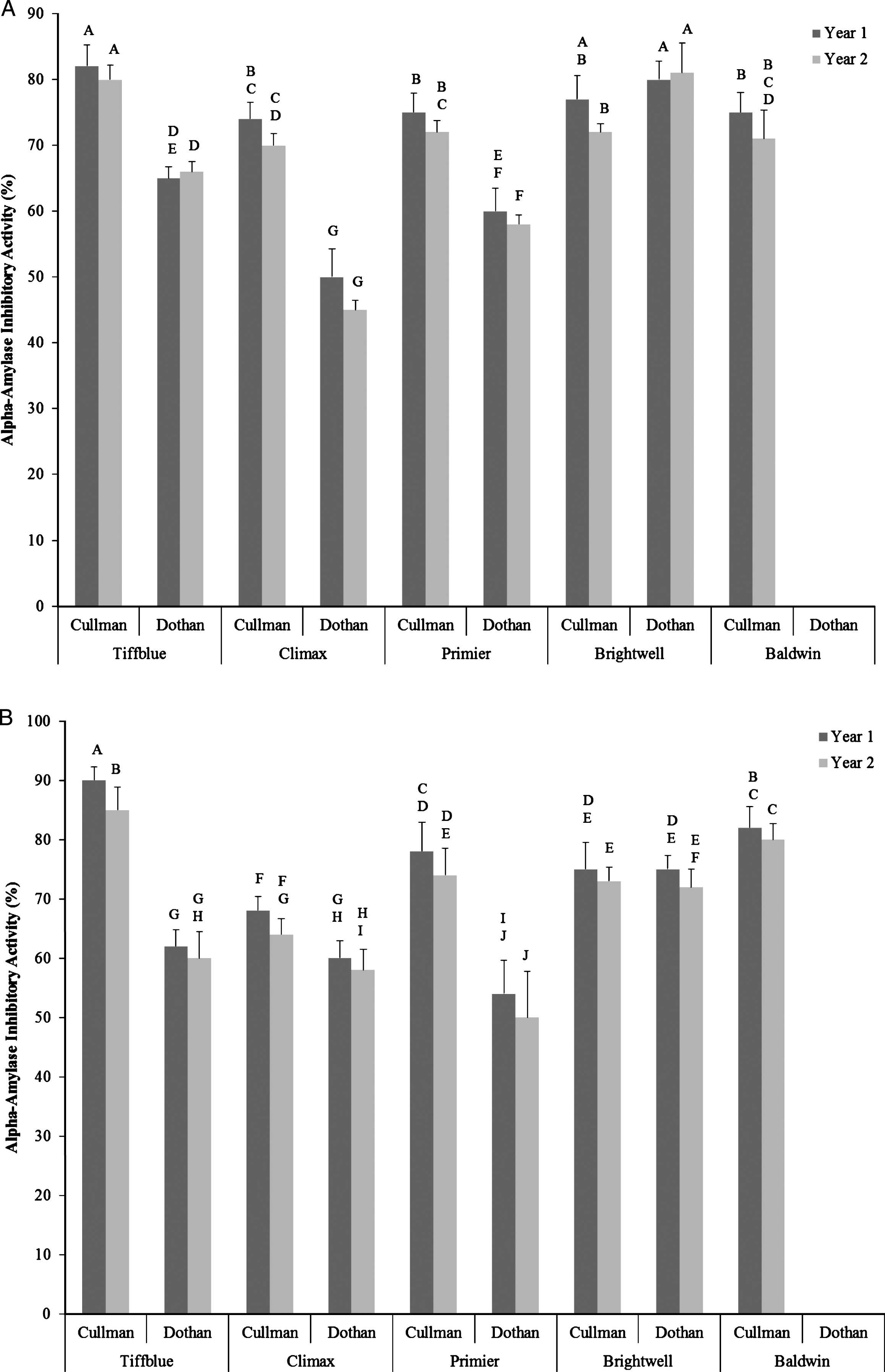
Like α-amylase inhibitory activity significant variations in α–glucosidase inhibitory activities among rabbit-eye blueberry cultivars, between growing seasons, locations, and cultivar×location×growing season interactions were also observed when blueberry extracts was diluted in half (Fig. 4A, B). Higher α–glucosidase inhibitory activity was observed in Brightwell (82%) and Climax (80%) blueberry cultivars, even in half-diluted sample of ethanol extracts (12%). In general, similar to the total soluble phenolic content and total antioxidant activity, higher α–glucosidase inhibitory was also observed in rabbit-eye blueberry cultivars grown in year 1 and in Cullman when compared with blueberry fruits of same cultivars from year 2 and from Dothan location. Similar level of α–glucosidase inhibitory activities has been reported in previous studies [19, 20, 24, 26]. This in vitro result suggests that rabbit-eye blueberry cultivars like Brightwell and Climax with high α–glucosidase and α–amylase inhibitory activity are potentially good dietary food choice and have relevance for slowing down the rapid breakdown and absorption of carbohydrates and subsequent reduction of blood glucose levels and can provide the biochemical rationale for further in vivo studies. This can shape further well designed in vivo studies interfaced with clinical studies. The overall basis and the novelty of this study was to screen important cultivars and to find the significant impact of genotype×environment interactions on α–glucosidase inhibitory activities of rabbit-eye blueberry cultivars using rapid in vitro models for screening. Therefore to optimize phenolic antioxidant-linked anti-hyperglycemic functions, it is important to adopt suitable growing conditions along with the selection of right rabbit-eye blueberry cultivars. The findings of this in vitro study suggests that rabbit-eye blueberry cultivars have significant promise for designing functional food and functional ingredients and can be targeted for dietary therapy of type 2 diabetes based on further in vivo studies.
Fig.4
Alpha-glucosidase inhibitory activity (%) of half-diluted water (A) and ethanol (B) extracts of five different rabbit-eye blueberry cultivars grown in two locations (Dothan & Cullman) and in two growing seasons. Different capital letter represents significant differences between cultivar×location×growing season interactions at p < 0.05 probability level.
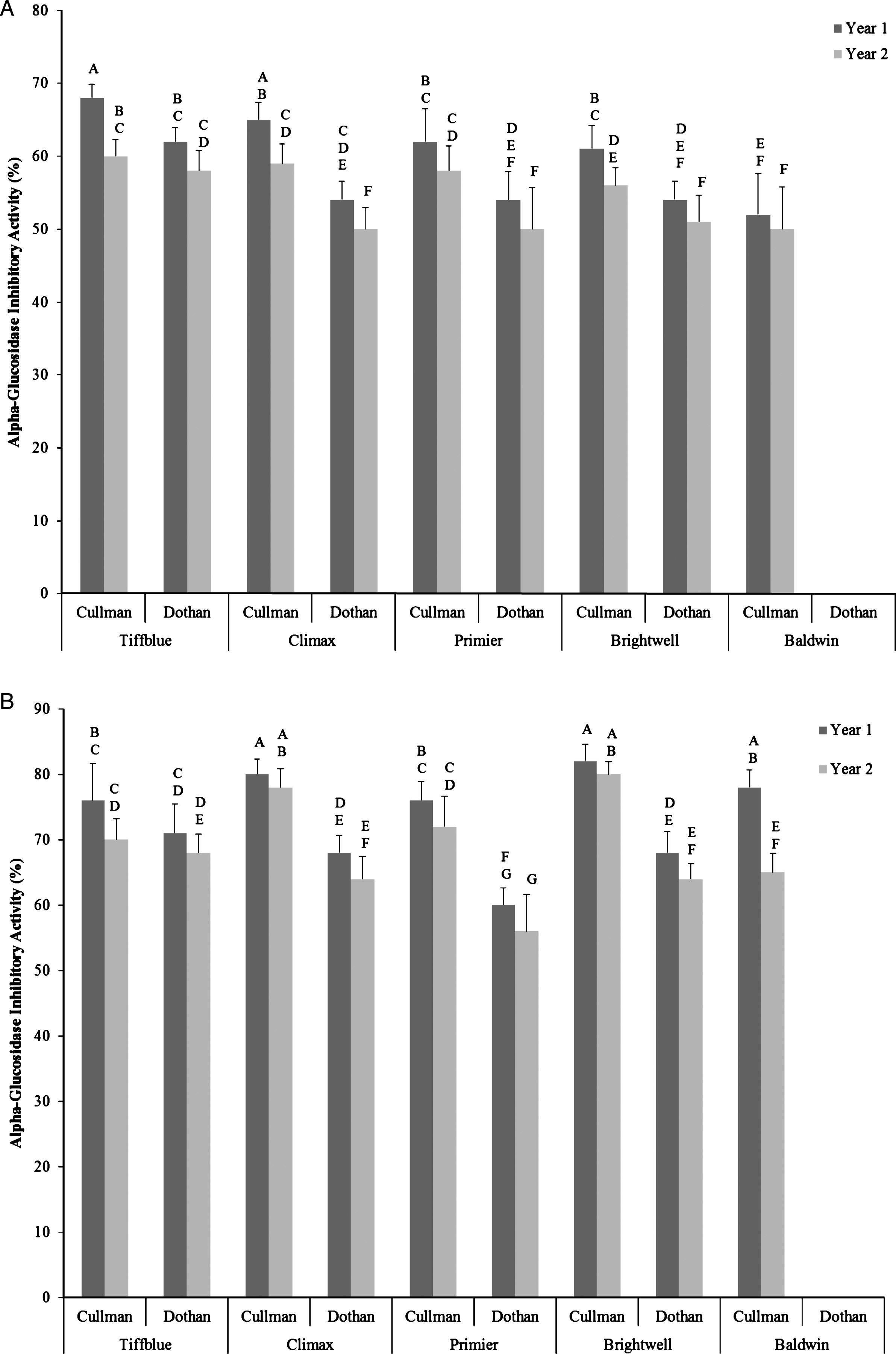
3.5ACE inhibitory activity
The angiotensin I-converting enzyme (ACE) converts angiotensin II from angiotensin I [37, 38]. Once angiotensin II is produced it can form a vasoconstrictor in the pancreas, which functions by constricting arteries and veins causing an increase in blood pressure. By inhibiting this vasoconstrictor it is possible to improve blood flow and insulin secretion through beta cells [38, 39]. Therefore, to determine the potentials of rabbit-eye blueberry cultivars to manage hypertension associated with type 2 diabetes, in vitro ACE inhibitory activity was determined. No ACE inhibitory activity was observed in all rabbit-eye blueberry cultivars evaluated in this study. Previous studies with blueberry and other berries also did not report any ACE inhibitory activities [19, 20, 26].
4Conclusions
Plant-based foods rich in phenolic antioxidants such as berries are excellent choice to be utilized in developing functional foods and functional ingredients to prevent and manage oxidative stress-linked diseases such as type 2 diabetes, CVD, and cancer. Such dietary strategy involving plant-based foods are safe and cost-effective as many pharmaceutical drugs have long term consequences and health care costs are also rising rapidly worldwide. But before utilizing in dietary strategies or in expensive clinical studies, it is important to screen and determine appropriate cultivars and suitable growing conditions for obtaining the optimum levels of human health relevant functional properties in plant foods. In this in vitro study, high phenolic-antioxidant-linked anti-hyperglycemic functions linked to anti-diabetic potential were found in five rabbit-eye blueberry cultivars grown in Alabama. The most important finding of this study is that cultivars, growing seasons, and locations have significant impact on phenolic antioxidant profiles and type 2 diabetes associated anti-hyperglycemic properties in rabbit-eye blueberry cultivars. Rabbit-eye blueberry cultivars such as Tiffblue, Brightwell, and Primier had significantly higher phenolic antioxidant-linked anti-hyperglycemic properties and can provide the biochemical rationale for further clinical studies targeting dietary therapy for early stages type 2 diabetes. This in vitro study also provides scientific rationale to screen large and diverse range of plant-based foods and ingredients and to select appropriate growing environment for optimizing human health relevant bioactive profiles and functions.
Conflict of interest
None to report.
References
[1] | Szajdek A , Borowska EJ . Bioactive compounds and health-promoting properties of berry fruits: A review. Plant Foods Hum Nutr. (2008) ;63: (4):147–56. |
[2] | Hariram Nile S , Won Park S . Edible berries: Bioactive components and their effect on human health (2014) ;30: (2):134–44. |
[3] | Zafra-Stone S , Yasmin T , Bagchi M , Chatterjee A , Vinson JA , Bagchi D . Berry anthocyanins as novel antioxidants in human health and disease prevention. Mol Nutr Food Res. (2007) ;51: (6):675–83. |
[4] | Van den Ende W , Peshev D , De Gara L . Disease prevention by natural antioxidants and prebiotics acting as ROS scavengers in the gastrointestinal tract. Trends in Food Science & Technology. (2011) ;22: :689–97. |
[5] | Alvarez-Suarez JM , Giampieri F , Tulipani S , Casoli T , Di Stefano G , González-Paramás AM , Santos-Buelga C , Busco F , Quiles JL , Cordero MD , Bompadre S . One-month strawberry-rich anthocyanin supplementation ameliorates cardiovascular risk, oxidative stress markers and platelet activation in humans. The Journal of Nutritional Biochemistry. (2014) ;25: (3):289–94. |
[6] | Tulipani S , Alvarez-Suarez JM , Busco F , Bompadre S , Quiles JL , Mezzetti B , Battino M . Strawberry consumption improves plasma antioxidant status and erythrocyte resistance to oxidative haemolysis in humans. Food Chemistry. (2011) ;128: (1):180–6. |
[7] | Blueberry Production Projected to Surpass 1.4 Billion Lb. in 2016 - U.S. Highbush Blueberry Council. (2016) http://www.blueberrycouncil.org/blueberry-production-projected-to-surpass-1-4-billion-lb-in-2016/ |
[8] | Blueberries | Agricultural Marketing Resource Center (2015) . http://www.agmrc.org/commodities-products/fruits/blueberries/ |
[9] | Scherm H , Krewer G . Blueberry production in georgia. Small Fruits Rev. (2003) ;2: (4):83–91. |
[10] | Lohachoompol V , Mulholland M , Srzednicki G , Craske J . Determination of anthocyanins in various cultivars of highbush and rabbiteye blueberries. Food Chem. (2008) ;111: (1):249–254. |
[11] | Li C , Feng J , Huang W-Y , An X-T . Composition of polyphenols and antioxidant activity of rabbiteye blueberry (Vaccinium ashei) in nanjing. J Agric Food Chem. (2013) ;61: (3):523–31. |
[12] | Su M-S , Silva JL . Antioxidant activity, anthocyanins, and phenolics of rabbiteye blueberry (Vaccinium ashei) by-products as affected by fermentation. Food Chem. (2006) ;97: (3):447–51. |
[13] | Shi M , Loftus H , McAinch AJ , Su XQ . Blueberry as a source of bioactive compounds for the treatment of obesity, type 2 diabetes and chronic inflammation. Journal of Functional Foods. (2017) ;30: :16–29. |
[14] | Heinonen IM , Meyer AS , Frankel EN . Antioxidant activity of berry phenolics on human low-density lipoprotein and liposome oxidation. J Agric Food Chem. (1998) ;46: (10):4107–12. |
[15] | Hanhineva K , Törrönen R , Bondia-pons I , Pekkinen J . Impact of dietary polyphenols on carbohydrate metabolism (2010) ;1365–402. |
[16] | Tadera K , Minami Y , Takamatsu K , Matsuoka T . Inhibition of ALPHA-glucosidase and ALPHA-Amylase by flavonoids. J Nutr Sci Vitaminol. (2006) ;52: (2):149–53. |
[17] | Krentz AJ , Bailey CJ . Oral antidiabetic agents. Drugs. (2005) ;65: (3):385–411. |
[18] | McDougall GJ , Stewart D . The inhibitory effects of berry polyphenols on digestive enzymes. Biofactors. (2005) ;23: (4):189–95. |
[19] | Cheplick S , Kwon Y-I , Bhowmik P , Shetty K . Phenolic-linked variation in strawberry cultivars for potential dietary management of hyperglycemia and related complications of hypertension. Bioresour Technol. (2010) ;101: (1):404–13. |
[20] | Pinto MDS , Kwon YI , Apostolidis E , Lajolo FM , Genovese MI , Shetty K . Functionality of bioactive compounds in Brazilian strawberry (Fragaria x ananassa Duch.) cultivars: Evaluation of hyperglycemia and hypertension potential using in vitro models. J Agric Food Chem. (2008) ;56: (12):4386–92. |
[21] | Cheng AYY , Fantus IG . Oral antihyperglycemic therapy for type 2 diabetes mellitus. CMAJ [Internet]. Canadian Medical Association. (2005) ;172: (2):213–26. |
[22] | Wang SY , Camp MJ , Ehlenfeldt MK . Antioxidant capacity and α-glucosidase inhibitory activity in peel and flesh of blueberry (Vaccinium s) cultivars. Food Chem. (2012) ;132: (4):1759–68. |
[23] | Prior RL , Cao G , Martin A , Sofic E , Mcewen J , Brien CO , et al. Antioxidant capacity as influenced by total phenolic and anthocyanin content, maturity, and variety of Vaccinium Species. J Agric Food Chem. (1998) ;8561: (98):2686–93. |
[24] | Cheplick S , Sarkar D , Bhowmik P , Shetty K . Phenolic bioactives from developmental stages of highbush blueberry (Vaccinium corymbosum) for hyperglycemia management using in vitro models. Can J Plant Sci. Agricultural Institute of Canada; (2015) ;95: (4):653–62. |
[25] | Shetty K , Curtis OF , Levin RE , Witkowsky R , Ang W . Prevention of vitrification aßociated with in vitro shoot culture of oregano (Origanum vulgare) by pseudomonas spp. J Plant Physiol. Urban & Fischer. (1995) ;147: (3):447–51. |
[26] | Da Silva Pinto M , Kwon Y-I , Apostolidis E , Lajolo FM , Genovese MI , Sshetty K . Evaluation of red currants (Ribes rubrum L.), black currants (Ribes Nigrum L.), red and green gooseberries (Ribes uva-crispa) for potential management of type 2 diabetes and hypertension using in vitro models. J Food Biochem. (2010) ;34: (3):639–60. |
[27] | Kwon YI , Vattem DA , Shetty K . Evaluation of clonal herbs of Lamiaceae species for management of diabetes and hypertension. Asia Pac J Clin Nutr. (2006) ;15: (1):107–18. |
[28] | Riso P , Klimis-Zacas D , Del Bo’ C , Martini D , Campolo J , Vendrame S , et al. Effect of a wild blueberry (Vaccinium angustifolium) drink intervention on markers of oxidative stress, inflammation and endothelial function in humans with cardiovascular risk factors. Eur J Nutr. Springer-Verlag. (2013) ;52: (3):949–61. |
[29] | Vattem DA , Ghaedian R , Shetty K . Enhancing health benefits of berries through phenolic antioxidant enrichment: Focus on cranberry. Asia Pac J Clin Nutr. (2005) ;14: (2):120–30. |
[30] | Huang W , Zhang H , Liu W , Li C . Survey of antioxidant capacity and phenolic composition of blueberry, blackberry, and strawberry in Nanjing. J Zhejiang Univ Sci B. (2012) ;13: (2):94–102. |
[31] | Howard LR , Clark JR , Brownmiller C . Antioxidant capacity and phenolic content in blueberries as affected by genotype and growing season. J Sci Food Agric. (2003) ;83: (12):1238–47. |
[32] | Connor AM , Luby JJ , Tong CBS , Finn CE , Hancock JF . Genotypic and environmental variation in antioxidant activity, total phenolic content, and anthocyanin content among blueberry cultivars. J Am Soc Hortic Sci. (2002) ;127: (1):89–97. |
[33] | Connor AM , Finn CE , Alspach PA . Genotypic and environmental variation in antioxidant activity and total phenolic content among blackberry and hybridberry cultivars. J Am Soc Hortic Sci. (2005) ;130: (4):527–33. |
[34] | Zheng W , Wang SY . Oxygen radical absorbing capacity of phenolics in blueberries, cranberries, chokeberries, and lingonberries. J Agric Food Chem. (2003) ;51: (2):502–9. |
[35] | Da Silva Pinto M , Kwon YI , Apostolidis E , Lajolo FM , Genovese MI , Shetty K . Potential of Ginkgo biloba L. leaves in the management of hyperglycemia and hypertension using in vitro models. Bioresour Technol. (2009) ;100: (24):6599–609. |
[36] | Marrazzo G , Barbagallo I , Galvano F , Malaguarnera M , Gazzolo D , Frigiola A , et al. Role of dietary and endogenous antioxidants in diabetes. Crit Rev Food Sci Nutr. (2014) ;54: (12):1599–616. |
[37] | Ferrannini E , Cushman WC . Diabetes and hypertension: The bad companions. Lancet. (2012) ;380: (9841):601–10. |
[38] | van Vark LC , Bertrand M , Akkerhuis KM , Brugts JJ , Fox K , Mourad J-J , et al. Angiotensin-converting enzyme inhibitors reduce mortality in hypertension: A meta-analysis of randomized clinical trials of renin–angiotensin–aldosterone system inhibitors involving 158 998 patients. Eur Heart J. (2012) ;33: (16):2088–97. |
[39] | Shouk R , Abdou A , Shetty K , Sarkar D , Eid AH . Mechanisms underlying the antihypertensive effects of garlic bioactives. Nutrition Research. (2014) ;34: :106–15. |