Effect of thoracic expansion restriction on scapulothoracic and glenohumeral joint motion during shoulder external rotation
Abstract
BACKGROUND:
Shoulder external rotation in the throwing motion involves movement of the scapulothoracic and glenohumeral joints, thoracic spine, and the thorax. Restriction of thoracic expansion may decrease scapulothoracic joint motion and compensate by excessive glenohumeral joint motion. However, it is unclear how restricting the expansion of the thorax alters shoulder motion.
OBJECTIVE:
To elucidate changes in scapulothoracic and glenohumeral joint movements caused by restricted thoracic expansion.
METHODS:
Kinematic data were obtained using an electromagnetic tracking device (Liberty; Polhemus), from 18 male participants, during shoulder external rotation in the sitting position with and without restriction of thoracic expansion. The displacements from the start position to the maximum external rotation position were compared, and Pearson’s correlation coefficient was calculated.
RESULTS:
A significant difference was observed in the scapulothoracic posterior tilt angle (
CONCLUSIONS:
Restriction of thoracic expansion decreased scapulothoracic motion and increased glenohumeral motion. Thus, a decrease in thoracic expansion may change scapulothoracic and glenohumeral movements, which may be a risk factor for throwing injuries.
1.Introduction
Throwing injuries of the shoulder are caused by repeated throwing motions in overhead sports such as baseball [1, 2, 3]. Most baseball players with such injuries complain of symptoms from the late cocking phase to the acceleration phase [4]. The late cocking phase, which begins after stride foot contact on the ground, involves trunk rotation and shoulder external rotation. The acceleration phase begins after the shoulder joint has reached its maximum external rotation (MER) position [5]. After MER, the movement of the shoulder joint changes abruptly from external to internal rotation, resulting in a large internal rotation torque and forward shear force on the shoulder [6]. Chronic application of mechanical stress due to repetitive throwing motions result in throwing injuries of the shoulder. Therefore, in order to prevent these injuries, it is necessary to analyze the shoulder joint motion from the late cocking phase to the early acceleration phase, particularly focusing on the MER.
The shoulder joint motion involves the glenohumeral and the scapulothoracic joints. Numerous studies have shown that the coordinated functioning of these joints is important for the prevention of shoulder injuries [7, 8]. In MER, the scapulothoracic joint motions are carried out by external rotation, upward rotation, and posterior tilt in the horizontal, frontal, and sagittal planes, respectively [9]. These three-axes scapulothoracic joint motions can be measured using electromagnetic tracking devices such as the Polhemus Liberty system (Polhemus, Inc., Colchester, VT, USA). According to the Liberty User’s manual, the accuracy of this device is reported to be 0.8 mm and 0.15
The scapula is in contact with the posterior wall of the thorax and moves along this wall when performing movement of the scapulothoracic joint. Therefore, the scapulothoracic joint movement is determined by changes in the configuration of the thorax. A study analyzing the shoulder flexion angle, scapulothoracic posterior tilt angle, and glenohumeral flexion angle during upper limb elevation with limited thoracic expansion movement stated that the shoulder flexion and scapulothoracic posterior tilt angles decreased and the mechanical stress on the glenohumeral joint relatively increased [15]. Thus, restriction of thoracic expansion motion might decrease scapulothoracic joint motion and produce excessive glenohumeral joint motion for compensation.
However, to the best of our knowledge, none of the studies used Liberty to investigate how restriction of thoracic expansion causes kinematic changes in the scapulothoracic and glenohumeral joint motions during shoulder external rotation. The purpose of this study was to investigate this effect, to help prevent the occurrence of throwing injuries, using the electromagnetic tracking device ’Liberty’. Our hypotheses were as follows: 1) restricting thoracic expansion would decrease scapulothoracic external rotation, upward rotation, and posterior tilt angles and increase glenohumeral external rotation angle at MER compared with the same movement without restriction, and 2) there would be a positive correlation between the decrease in scapulothoracic joint angles and the increase in glenohumeral external rotation angle.
2.Materials and methods
2.1Study design and setting
This cross-sectional study examined the kinematic changes in the scapulothoracic and glenohumeral joints during shoulder external rotation with and without restricted thoracic expansion using an electromagnetic device, Liberty. The study was conducted in a laboratory, and healthy males were recruited. All dependent variables of scapulothoracic internal rotation angle, upward rotation angle, posterior tilt angle, and glenohumeral external rotation angle were compared in each participant.
2.2Participants
Eighteen right-handed healthy males (mean
The sample size was calculated using G*power 3.1.9.4 software (Heinrich-Heine-University Düsseldorf, Düsseldorf, Germany). The effect size, mean power, and alpha error were set to 0.25, 0.80, and 0.05, respectively. The analysis revealed that at least 15 participants would make an acceptable sample size.
This study was approved by the Ethical Committee for Epidemiology of Hiroshima University (E-2177), and written informed consent was obtained from all participants in accordance with the Declaration of Helsinki.
2.3Procedures
The participants performed shoulder external rotation in two conditions: with and without restriction of the thorax by taping. Participants sat on a chair with the hip and knee joints flexed at 90
Figure 1.
Participants sat on the chair with their hip and knee joint flexed at 90
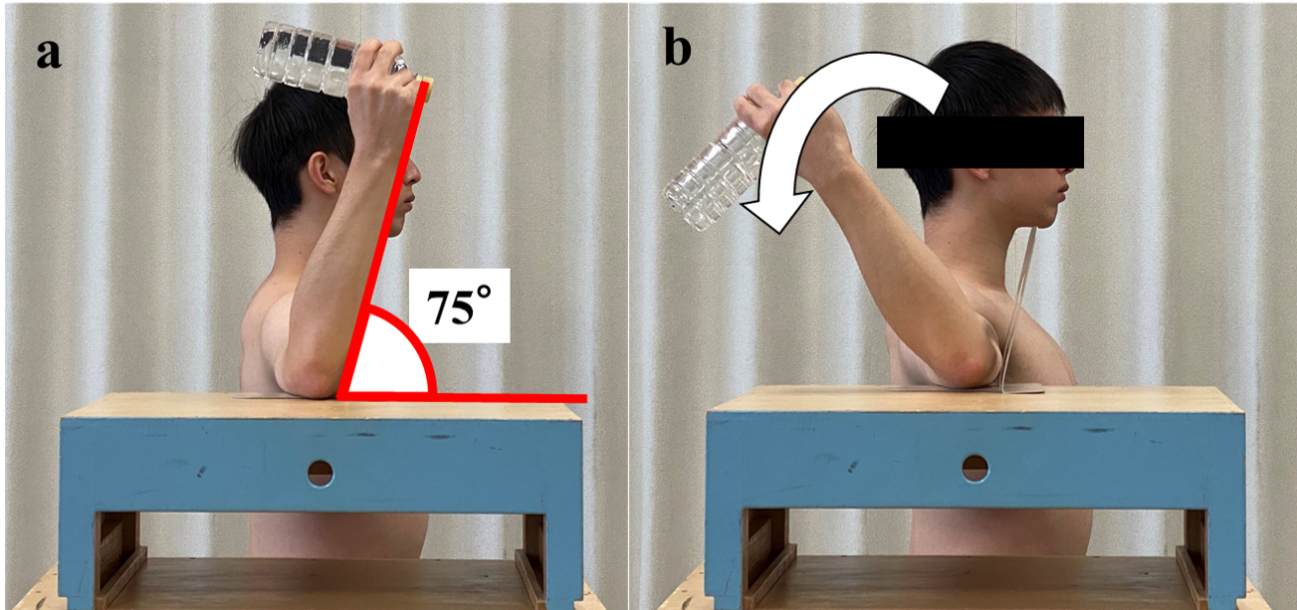
Figure 2.
(a) Without restriction, (b) With restriction. One round of non-elastic tape was applied to the thorax in the maximal expiratory state without applying tension. In addition, an elastic was applied over the non-stretch tape in the same manner for reinforcement. The tape was applied at the 5th and 10th rib positions.
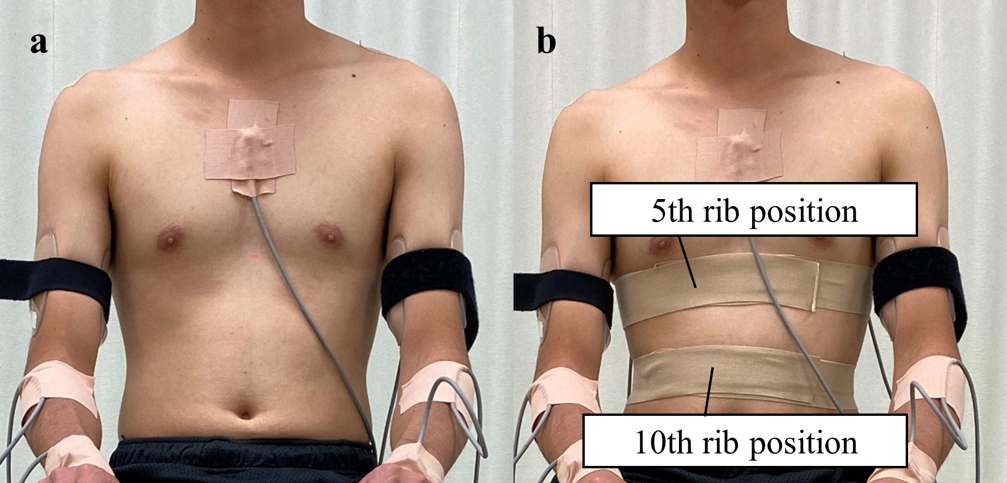
In each condition, the participants performed shoulder external rotation while grasping a 500 mL plastic water bottle with the right hand [17]. The electronic metronome was set to 60 beats per minute and the shoulder external rotation from the starting position (75
2.4Measurement of kinematic data
Kinematic data were measured using an electromagnetic tracking device, Liberty
Figure 3.
SN: sternal notch, XP: xiphoid process, ASIS: anterior superior iliac spine, PS: pubic symphysis, AA: acromial angle, SP: medial border of the scapular spine, IA: inferior angle, HH: humeral head, ME: medial epicondyle, LE: lateral epicondyle.
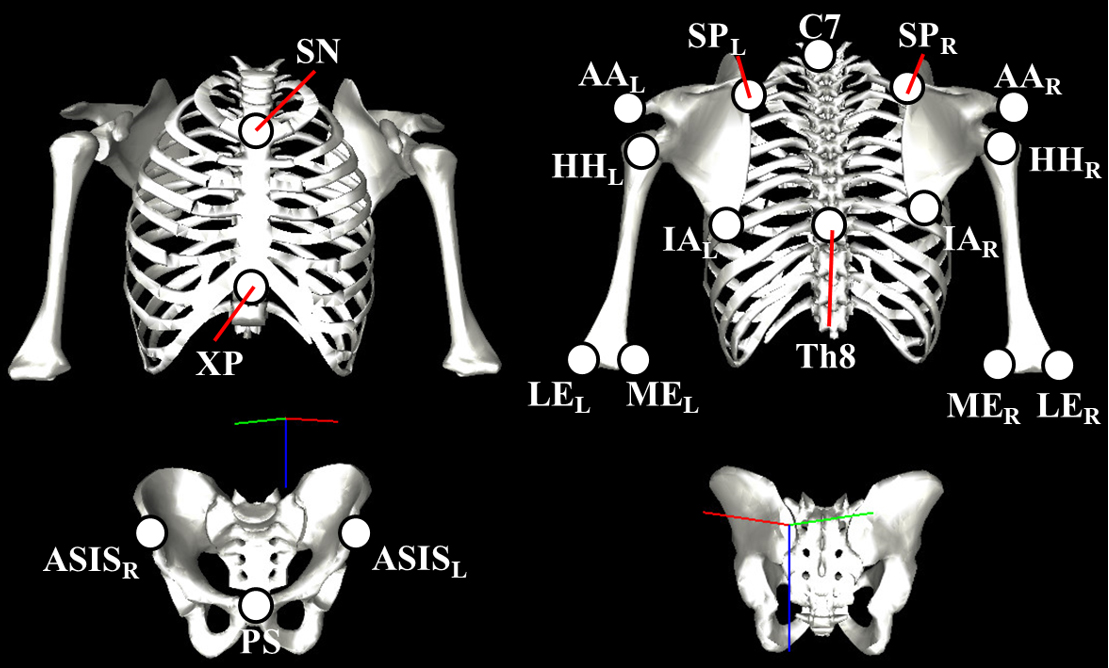
2.5Data analysis
The joint kinematic data were calculated and represented by the Euler angles based on relative orientations of the thorax, scapula, and humerus. The motion of the scapula relative to that of the thorax (scapulothoracic joint) was classified as internal (+) or external (-) rotation, downward (+) or upward (-) rotation, and posterior (+) or anterior (-) tilt (Fig. 4). The motion of the humerus relative to the scapula (glenohumeral joint) was classified as horizontal adduction (+) or abduction (-), elevation (-), and internal (+) or external (-) rotation (Fig. 4).
Figure 4.
ST: scapulothoracic joint, GH: glenohumeral joint.
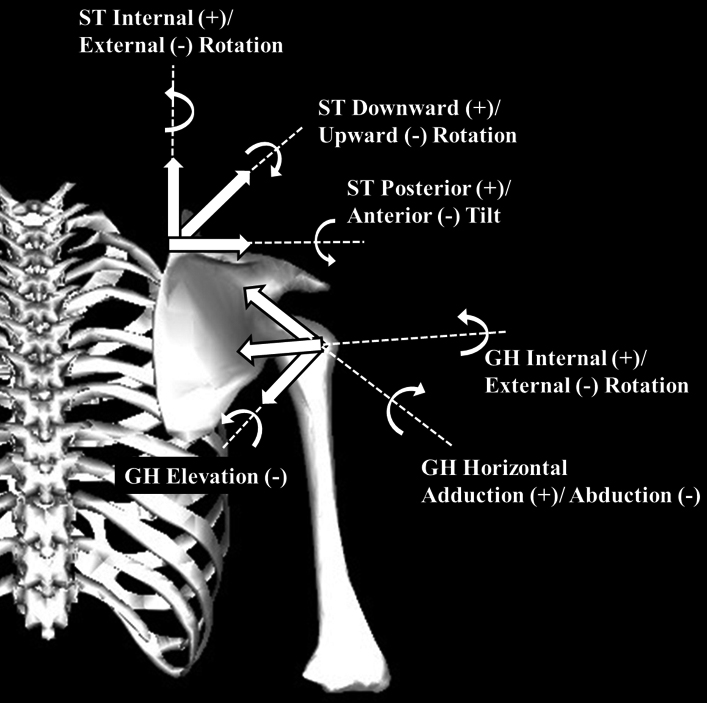
The internal rotation, upward rotation, and posterior tilt angles of the scapulothoracic joint, and the external rotation angle of the glenohumeral joint were measured at two time points: 75
2.6Statistical analysis
IBM SPSS Statistics version 27.0 for Windows (IBM Corp., Armonk, NY, USA) was used for all statistical analyses. The Shapiro-Wilk test was used to check the normality of the data. Since normality could not be obtained for the data, a Wilcoxon signed-rank test was used to compare the difference in thoracic expansion and kinematic data in the two conditions. Effect size (
3.Results
3.1Difference in thoracic expansion
Table 1 shows the results of the difference in thorax expansion. With restriction, a significant decrease was observed in both the 5th and the 10th rib positions, compared to conditions without restriction (5th rib position:
Table 1
Difference in thoracic expansion
Values | Without restriction | With restriction | Effect size | |||||
Mean | 95% CI | Mean | 95% CI | |||||
Lower | Upper | Lower | Upper | |||||
5th rib position (cm) | 5.1 | 4.4 | 5.8 | 2.1 | 1.6 | 2.5 | 0.93 | |
10th rib position (cm) | 5.6 | 4.5 | 6.6 | 1.9 | 1.5 | 2.3 | 0.91 |
SD: standard deviation, CI: confidence interval. *Significant difference between with and without restriction (
Figure 5.
The difference between the displacement with and without restriction was calculated. Spearman’s rank correlation coefficient was used to determine the relationships. A positive displacement of ST posterior tilt indicates posterior tilt and a negative indicates an anterior tilt. On the other hand, positive displacement of GH internal rotation indicates internal rotation, while negative displacement indicates external rotation. A significant positive correlation was observed (
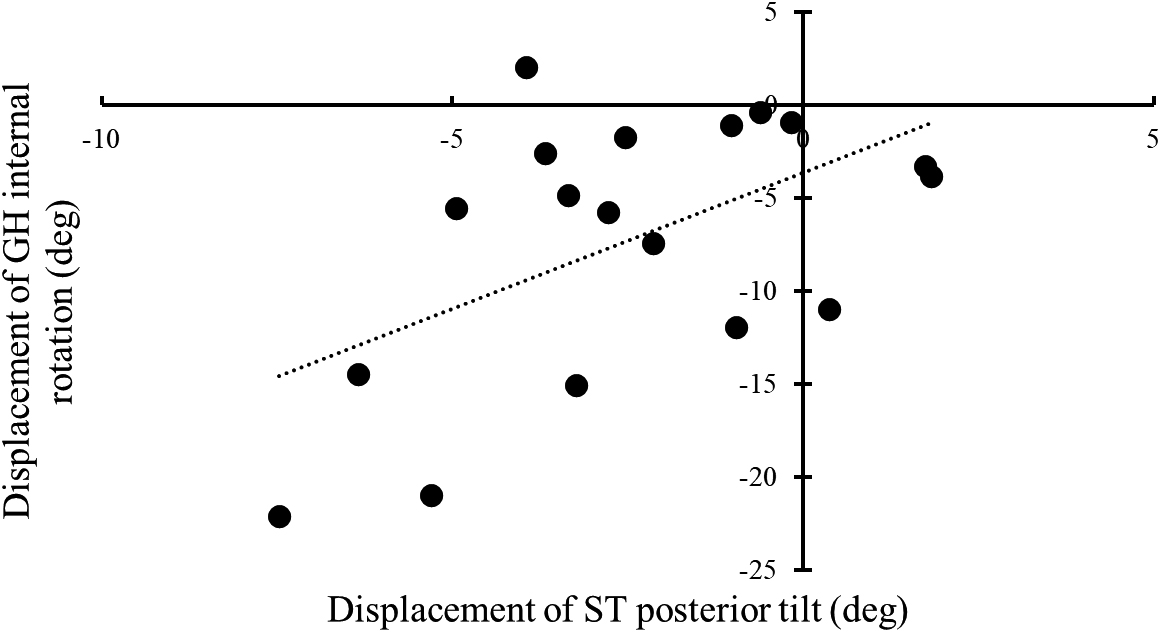
3.2Kinematic data
Table 2 shows the results of the kinematic data of the scapulothoracic and glenohumeral joints between the two conditions. Significant differences were observed in scapulothoracic posterior tilt (
Table 2
Kinematic data of scapulothoracic and glenohumeral motions with and without restriction
Values | Without restriction | With restriction | ||||||||||||||
---|---|---|---|---|---|---|---|---|---|---|---|---|---|---|---|---|
Mean | 95% CI | Mean | 95% CI | Effect size | ||||||||||||
Lower | Upper | Lower | Upper | |||||||||||||
MER (deg) | 103.4 | 97. | 4 | 109. | 4 | 106.1 | 100. | 7 | 111. | 5 | 0. | 162 | 0. | 37 | ||
ST Internal Rotation (deg) | ||||||||||||||||
ER 75 | 15.0 | 10. | 2 | 19. | 8 | 16.5 | 11. | 0 | 21. | 9 | 0. | 241 | 0. | 31 | ||
MER | 7.6 | 2. | 0 | 13. | 2 | 9.4 | 4. | 0 | 14. | 8 | 0. | 106 | 0. | 42 | ||
Displacement | 4 | 4 | 3 | 9 | 0. | 627 | 0. | 13 | ||||||||
ST Downward Rotation (deg) | ||||||||||||||||
ER 75 | 7 | 6 | 8 | 4 | 0. | 485 | 0. | 19 | ||||||||
MER | 7 | 8 | 1 | 0 | 0. | 805 | 0. | 07 | ||||||||
Displacement | 1 | 2 | 9 | 1 | 0. | 820 | 0. | 04 | ||||||||
ST Posterior Tilt (deg) | ||||||||||||||||
ER 75 | 13.2 | 7. | 7 | 18. | 7 | 9.6 | 2. | 7 | 16. | 5 | 0. | 217 | 0. | 33 | ||
MER | 21.4 | 15. | 5 | 27. | 2 | 15.5 | 7. | 5 | 23. | 4 | 0. | 047* | 0. | 50 | ||
Displacement | 8.2 | 6. | 1 | 10. | 3 | 5.8 | 3. | 6 | 8. | 1 | 0. | 001* | 0. | 74 | ||
GH Internal Rotation (deg) | ||||||||||||||||
ER 75 | 0 | 0 | 7 | 5 | 0. | 073 | 0. | 46 | ||||||||
MER | 0 | 0 | 0 | 6 | 0. | 005* | 0. | 67 | ||||||||
Displacement | 5 | 4 | 3 | 2 | 0. | 006* | 0. | 66 |
SD: standard deviation, CI: confidence interval, MER: maximum external rotation, ST: scapulothoracic joint, GH: glenohumeral joint, ER 75
Figure 5 shows the relationship between the difference in displacement of the scapulothoracic and the glenohumeral joints and the presence of restriction. A significant positive correlation existed between the posterior tilt of the scapulothoracic joint and the internal rotation of the glenohumeral joint with a correlation coefficient of 0.54 (
4.Discussion
This study aimed to clarify the effect of restricted thoracic expansion on scapulothoracic and glenohumeral joint motions during shoulder external rotation. It was revealed that restriction of thoracic expansion decreased the scapulothoracic posterior tilt angle and increased the glenohumeral external rotation angle in MER. However, no significant change was observed at MER angle with or without restriction of thoracic expansion. Therefore, increase in glenohumeral external rotation is a compensatory movement for the decrease in scapulothoracic posterior tilt associated with restricted thoracic expansion.
As reported previously, the present study also observed restricted thoracic expansion during maximal expiration and maximal inspiration. Thoracic expansion occurs by rotational movements around the costovertebral joints that occur antero-posteriorly in the upper thorax and medio-laterally in the lower thorax [23]. In the middle of the thorax, the axis of the costovertebral joint is at 45
The scapulothoracic posterior tilt angle in the restricted condition in MER was significantly lower than that in the unrestricted condition. Previous studies showed that changes in the configuration of the thorax were a factor in the restriction of the scapulothoracic joint. The thoracic extension motion is considered to be the cause of this change in thoracic configuration. Backward rotational movement of the ribs by the costovertebral joint is caused by extension of the thoracic spine, especially the lower thoracic spine [25]. As the thorax expands, it is elevated superiorly and anteriorly, and its posterior wall tilts backward. In this study, taping the thorax could have possibly decreased the posterior tilt of the scapulothoracic joint by limiting these movements.
The most important finding of this study was that there was no significant difference in MER angle between conditions, despite the restricted scapulothoracic joint motion. A previous study reported that a decrease in scapulothoracic joint motion resulted in a decrease in MER angle [17]. However, in the present study, MER angle did not change with restriction. This was because the excessive glenohumeral external rotation compensated for the decreased motion of the scapulothoracic joint, as shown in the results of the moderate correlation between displacement of the scapulothoracic posterior tilt and displacement of the glenohumeral internal rotation. It is obvious that the coordinated movement of the scapulothoracic and glenohumeral joints is essential for shoulder movement. It has been reported that malfunctioning of one of the joints directly affected the other joint [26]. Thoracic expansion was reduced and scapulothoracic joint motion was limited. However, it is possible that the glenohumeral movement was increased more to prevent the decrease in shoulder external rotation. These results indicate that MER angle was not altered by limitation of thoracic extension, but ST posterior tilt was increased and GH external rotation was decreased.
One throwing injury caused by excessive glenohumeral external rotation is shoulder internal impingement [11]. This is characterized by contact between the greater tuberosity of the humerus and the glenoid fossa during the late cocking phase and early acceleration phase, when the shoulder exhibits abduction and external rotation, and most athletes with internal impingement complain of pain in the posterior shoulder joint [27, 28]. Previous studies have shown that the narrowing of the distance between the glenoid fossa and the humeral head due to restricted scapulothoracic motion and excessive glenohumeral motion is a factor in the development of shoulder internal impingement [29]. These findings suggest that restriction of thoracic expansion is a risk factor for the development of shoulder internal impingement, as it may cause narrowing of the shoulder joint distance due to excessive glenohumeral external rotation. Improving scapulothoracic joint function by strengthening the middle and lower trapezius is used for rehabilitation for throwing injuries [30]. However, the findings of the present study indicate that thoracic expansion are essential for improving scapulothoracic joint function and that thoracic exercises should also be focused on the rehabilitation of throwing injuries. Future research including people with restricted thoracic expansion is warranted to clarify the causal relationship with shoulder internal impingement.
This study had several limitations. First, the restriction of thoracic expansion was done manually by the same examiner by taping. Therefore, skin movement could possibly have influenced the measurements. Second, the participants were young healthy adult males. The elevation motion in patients with shoulder joint disease may differ from that in healthy individuals. Moreover, the static alignment in overhead athletes may be adapted to competition-specific movements. Thus, there is a need to investigate patients with shoulder joint diseases and overhead athletes in the future. Finally, this study was conducted in the sitting position, so it is not clear whether the results would be similar during actual pitching motion. For detailed clarification of the effect of restricted thoracic expansion on throwing motion, future studies should investigate scapulothoracic and glenohumeral joints motion during actual throwing motion.
5.Conclusion
This study investigated the effects of thoracic expansion restriction on scapulothoracic and glenohumeral joint motions during shoulder external rotation in a sitting position assuming MER. Restriction of thoracic expansion motion decreased the scapulothoracic posterior tilt and increased the glenohumeral external rotation, suggesting that restriction of thoracic expansion increases mechanical stress on the glenohumeral joint. The results indicate that it is necessary to focus on thoracic expansion exercises as the foundation for interventions to improve scapulothoracic joint motion, such as scapular mobilization and periscapular muscle exercises. Emphasis on thoracic extension movement during shoulder joint exercises may reduce the stress on the glenohumeral joint and contribute to the prevention of pitching disorders.
Acknowledgments
The authors would like to thank everyone who participated in this study.
Conflict of interest
The authors have no conflict of interest relevant to this article.
Funding
This research did not receive any specific grant from funding agencies in the public, commercial, or not-for-profit sectors.
References
[1] | Erickson BJ, Chalmers PN, Axe MJ, Romeo AA. Exceeding pitch count recommendations in little league baseball increases the chance of requiring tommy john surgery as a professional baseball pitcher. Orthop J Sports Med. (2017) ; 5: (3). doi: 10.1177/2325967117695085. |
[2] | Norton R, Honstad C, Joshi R, Silvis M, Chinchilli V, Dhawan A. Risk factors for elbow and shoulder injuries in adolescent baseball players: a systematic review. Am J Sports Med. (2019) ; 47: (4): 982-990. doi: 10.1177/0363546518760573. |
[3] | Fleisig GS, Andrews JR, Cutter GR, Weber A, Loftice J, McMichael C, et al. Risk of serious injury for young baseball pitchers: a 10-year prospective study. Am J Sports Med. (2011) ; 39: (2): 253-257. doi: 10.1177/0363546510384224. |
[4] | Freehill MT, Mannava S, Higgins LD, Lädermann A, Stone AV. Thrower’s exostosis of the shoulder: a systematic review with a novel classification. Orthop J Sports Med. (2020) ; 8: (7). doi: 10.1177/2325967120932101. |
[5] | Jobe FW, Tibone JE, Perry J, Moynes D. An EMG analysis of the shoulder in throwing and pitching. A preliminary report. Am J Sports Med. (1983) ; 11: (1): 3-5. doi: 10.1177/036354658301100102. |
[6] | Fleisig GS, Andrews JR, Dillman CJ, Escamilla RF. Kinetics of baseball pitching with implications about injury mechanisms. Am J Sports Med. (1995) ; 23: (2): 233-239. doi: 10.1177/036354659502300218. |
[7] | Kolz CW, Sulkar HJ, Aliaj K, Tashjian RZ, Chalmers PN, Qiu Y, et al. Age-related differences in humerothoracic, scapulothoracic, and glenohumeral kinematics during elevation and rotation motions. J Biomech. (2021) ; 117: . doi: 10.1016/j.jbiomech.2021.110266. |
[8] | Kibler WB, Thomas SJ. Pathomechanics of the throwing shoulder. Sports Med Arthrosc Rev. (2012) ; 20: (1): 22-29. doi: 10.1097/JSA.0b013e3182432cf2. |
[9] | Karduna AR, McClure PW, Michener LA, Sennett B. Dynamic measurements of three-dimensional scapular kinematics: a validation study. J Biomech Eng. (2001) ; 123: (2): 184-190. doi: 10.1115/1.1351892. |
[10] | Miyashita K, Kobayashi H, Koshida S, Urabe Y. Glenohumeral, scapular, and thoracic angles at maximum shoulder external rotation in throwing. Am J Sports Med. (2010) ; 38: (2): 363-368. doi: 10.1177/0363546509347542. |
[11] | Walch G, Boileau P, Noel E, Donell ST. Impingement of the deep surface of the supraspinatus tendon on the posterosuperior glenoid rim: An arthroscopic study. J Shoulder Elbow Surg. (1992) ; 1: (5): 238-245. doi: 10.1016/S1058-2746(09)80065-7. |
[12] | Takahashi M, Iwamoto K, Monma M, Mutsuzaki H, Mizukami M. The area of impingement in the throwing versus nonthrowing shoulder of collegiate baseball players: an MRI study of the simulated late-cocking phase of throwing. Orthop J Sports Med. (2021) ; 9: (3). doi: 10.1177/2325967121992133. |
[13] | Ludewig PM, Reynolds JF. The association of scapular kinematics and glenohumeral joint pathologies. J Orthop Sports Phys Ther. (2009) ; 39: (2): 90-104. doi: 10.2519/jospt.2009.2808. |
[14] | Kibler WB, Ludewig PM, McClure PW, Michener LA, Bak K, Sciascia AD. Clinical implications of scapular dyskinesis in shoulder injury: the 2013 consensus statement from the ’Scapular Summit’. Br J Sports Med. (2013) ; 47: (14): 877-885. doi: 10.1136/bjsports-2013-092425. |
[15] | Okamune R, Miyashita K, Koyama T, Tani Y, Ota K, Emon Y, et al. A three-dimensional motion analysis of anterior upper limb elevation with thoracic motion restriction. Rigakuryoho Kagaku (2020) ; 35: (2): 217-222. doi: 10.1589/rika.35.217. |
[16] | Konda S, Yanai T, Sakurai S. Scapular rotation to attain the peak shoulder external rotation in tennis serve. Med Sci Sports Exerc. (2010) ; 42: (9): 1745-1753. doi: 10.1249/MSS.0b013e3181d64103. |
[17] | Suzuki Y, Muraki T, Sekiguchi Y, Ishikawa H, Yaguchi H, Suzuki Y, et al. Influence of thoracic posture on scapulothoracic and glenohumeral motions during eccentric shoulder external rotation. Gait Posture. (2019) ; 67: : 207-212. doi: 10.1016/j.gaitpost.2018.10.022. |
[18] | Okamune R, Miyashita K, Tani Y, Ota K, Emon Y, Koyama T, et al. A comparison of movements of the thoracic cage when inspiring with thoracic expansion restrictions at different levels. Rigakuryoho Kagaku (2019) ; 34: (1): 21-25. doi: 10.1589/rika.34.21. |
[19] | Kono Y, Sakamitsu T, Sasadai J, Yamamoto M, Okamoto K, Ushio K, et al. The comparison of biomechanics of kayak paddling between para-kayakers and elite able-bodied kayakers: a pilot study. Sport Sci Health. (2021) ; 17: (4): 947-951. doi: 10.1007/s11332-021-00757-4. |
[20] | Wu G, van der Helm FC, Veeger HE, Makhsous M, Van Roy P, Anglin C, et al. ISB recommendation on definitions of joint coordinate systems of various joints for the reporting of human joint motion-Part II: shoulder, elbow, wrist and hand. J Biomech. (2005) ; 38: (5): 981-992. doi: 10.1016/j.jbiomech.2004.05.042. |
[21] | Veeger HE. The position of the rotation center of the glenohumeral joint. J Biomech. (2000) ; 33: (12): 1711-1715. doi: 10.1016/s0021-9290(00)00141-x. |
[22] | Cohen J. Statistical power analysis for behavioral sciences. 2nd ed., Hillsdale: Lawrence Erlbaum Associates, (1988) . |
[23] | Bastir M, García-Martínez D, Torres-Tamayo N, Sanchis-Gimeno JA, O’Higgins P, Utrilla C, et al. In vivo 3D analysis of thoracic kinematics: changes in size and shape during breathing and their implications for respiratory function in recent humans and fossil hominins. Anat Rec (Hoboken). (2017) ; 300: (2): 255-264. doi: 10.1002/ar.23503. |
[24] | Beyer B, Sholukha V, Dugailly PM, Rooze M, Moiseev F, Feipel V, et al. In vivo thorax 3D modelling from costovertebral joint complex kinematics. Clin Biomech (Bristol, Avon). (2014) ; 29: (4): 434-438. doi: 10.1016/j.clinbiomech.2014.01.007. |
[25] | Masharawi Y, Rothschild B, Dar G, Peleg S, Robinson D, Been E, et al. Facet orientation in the thoracolumbar spine: three-dimensional anatomic and biomechanical analysis. Spine. (2004) ; 29: (16): 1755-1763. doi: 10.1097/01.brs.0000134575.04084.ef. |
[26] | Paine R, Voight ML. The role of the scapula. Int J Sports Phys Ther. (2013) ; 8: (5): 617-629. |
[27] | Bolia IK, Collon K, Bogdanov J, Lan R, Petrigliano FA. Management options for shoulder impingement syndrome in athletes: insights and future directions. Open Access J Sports Med. (2021) ; 12: : 43-53. doi: 10.2147/OAJSM.S281100. |
[28] | Corpus KT, Camp CL, Dines DM, Altchek DW, Dines JS. Evaluation and treatment of internal impingement of the shoulder in overhead athletes. World J Orthop. (2016) ; 7: (12): 776-784. doi: 10.5312/wjo.v7.i12.776. |
[29] | Mihata T, Jun BJ, Bui CN, Hwang J, McGarry MH, Kinoshita M, et al. Effect of scapular orientation on shoulder internal impingement in a cadaveric model of the cocking phase of throwing. J Bone Joint Surg Am. (2012) ; 94: (17): 1576-1583. doi: 10.2106/JBJS.J.01972. |
[30] | Mayes M, Salesky M, Lansdown DA. Throwing injury prevention strategies with a whole kinetic chain-focused approach. Curr Rev Musculoskelet Med. (2022) ; 1-12. doi: 10.1007/s12178-022-09744-9. |