Digital Biomarkers for the Assessment of Non-Cognitive Symptoms in Patients with Dementia with Lewy Bodies: A Systematic Review
Abstract
Background:
Portable digital health technologies (DHTs) could help evaluate non-cognitive symptoms, but evidence to support their use in patients with dementia with Lewy bodies (DLB) is uncertain.
Objective:
1) To describe portable or wearable DHTs used to obtain digital biomarkers in patients with DLB, 2) to assess the digital biomarkers’ ability to evaluate non-cognitive symptoms, and 3) to assess the feasibility of applying digital biomarkers in patients with DLB.
Methods:
We systematically searched databases MEDLINE, Embase, and Web of Science from inception through February 28, 2023. Studies assessing digital biomarkers obtained by portable or wearable DHTs and related to non-cognitive symptoms were eligible if including patients with DLB. The quality of studies was assessed using a modified check list based on the NIH Quality assessment tool for Observational Cohort and Cross-sectional Studies. A narrative synthesis of data was carried out.
Results:
We screened 4,295 records and included 20 studies. Seventeen different DHTs were identified for assessment of most non-cognitive symptoms related to DLB. No thorough validation of digital biomarkers for measurement of non-cognitive symptoms in DLB was reported. Studies did not report on aspects of feasibility in a systematic way.
Conclusions:
Knowledge about feasibility and validity of individual digital biomarkers remains extremely limited. Study heterogeneity is a barrier for establishing a broad evidence base for application of digital biomarkers in DLB. Researchers should conform to recommended standards for systematic evaluation of digital biomarkers.
INTRODUCTION
Dementia with Lewy bodies (DLB) is second only to Alzheimer’s disease (AD) in terms of the prevalence of neurodegenerative causes of dementia [1], with the first diagnostic criteria being proposed in 1996 [2, 3]. Aside from cognitive impairment, patients with DLB often suffer from various non-cognitive symptoms such as visual hallucinations, REM sleep behavior disorder (RBD), and parkinsonism [4] either preceding the diagnosis of dementia or emerging at later stages of the disease course [5, 6]. While cognitive symptoms are defining for dementia, non-cognitive symptoms are of equal importance for the specific diagnosis of DLB [4], and they significantly impact patients’ quality of life [7, 8]. Moreover, some non-cognitive symptoms may be manageable such as parkinsonism and autonomic dysfunction (see Table 1). However, it is difficult to evaluate the presence and severity of non-cognitive symptoms in patients with DLB as cognitive deficits may compromise reliable symptom description. Also, high interobserver variability for assessment scales of non-cognitive symptoms such as motor examination using the UPDRS (Unified Parkinson’s Disease Rating Scale) part III has been reported [9]. Thus, there is a need for objective and precise tools to improve the ability to detect non-cognitive symptoms and to assess their severity. At the same time, growing demands on the efficiency of health care systems require implementation of low-cost, data-driven tools in the clinical assessment and decision-making to ensure maximal resource and capacity utilization and quality of future patient care [10].
Table 1
Non-cognitive symptoms in Dementia with Lewy bodies
Symptom | Description |
Parkinsonism | Disorder of movement characterized by bradykinesia (slowness of movement), tremor and rigidity. Problems initiating movements, disordered gait and postural instability may also be present. |
Fluctuations | Fluctuations in cognitive function and especially attention. Patients may “zone out”, become unresponsive or inattentive for seconds, minutes or hours. |
Visual hallucinations and visual illusory perceptions | May include outright visual hallucinations of persons, family members, animals etc. or less well-formed ones such as a passing shadow/figure in the peripheral visual field. Visual illusory perceptions (sometimes referred to as pareidolias) could be a sweater lying on a bed being perceived as a cat, a jacket hung in a dark corner as a figure, a face in the leaves of a tree. |
Rapid eye movement (REM) sleep disorder | Disorder of the REM phase of sleep leading to the patient acting out dreams due to loss of atonia. |
Hyposmia/anosmia | Reduced or complete loss of sense of smell |
Autonomic dysfunction | e.g., erectile dysfunction, constipation, orthostatic hypotension |
Digital biomarkers, which are biomarkers collected from digital health technologies (DHTs, i.e., “A system that uses computing platforms, connectivity, software, and sensors for healthcare and related uses”) [11], show potential in closing these gaps. Indeed, the research field of digital biomarkers is rapidly expanding with an increasing number of available DHTs measuring biological and physiological data [12]. This may be done in an unobtrusive manner such as with smartwatches, which can monitor numerous parameters including limb movement, heart rate, and whereabouts with automatic upload and analysis of data. Portable, or wearable DHTs could become valuable tools due to their convenience, however, evidence to support their use in the assessment of patients with DLB is uncertain. Indeed, application of DHTs in a population with dementia may pose a challenge, thus evaluation of feasibility is of special importance, when considering new digital biomarkers for future clinical practice.
Several use-cases for DHTs in the management of patients with DLB could be envisioned, e.g., as diagnostic and prognostic biomarkers, the latter missing almost entirely from the current biomarker landscape. Moreover, accurate measures of symptoms to track treatment effects and even as trial outcomes are also highly relevant applications.
This review seeks to identify and synthesize current knowledge on digital biomarkers applied for the assessment of non-cognitive symptoms in patients with DLB. Specifically, the objectives were: 1) to identify wearable or portable DHTs used to obtain digital biomarkers in patients with DLB, 2) to assess the ability of these biomarkers to monitor and evaluate non-cognitive symptoms in DLB, and 3) to assess the feasibility of applying digital biomarkers in patients with DLB. To our knowledge, no systematic review exists on this topic.
METHODS
A study protocol for this review was uploaded to the PROSPERO database (ID: CRD42023400196). Reporting on this systematic review was conducted according to the PRISMA 2020 Statement [13].
Eligibility criteria
Original observational or interventional studies published in peer-reviewed international journals were considered eligible if they met all inclusion criteria and none of the exclusion criteria:
Inclusion criteria
- Reporting on adult humans (age > 17 years) meeting diagnostic criteria for DLB (McKeith, 3rd and 4th edition [4, 14]).
- Reporting on digital biomarkers (according to reported definition [11]), which assessed non-cognitive symptoms in DLB and were obtained by wearable or portable DHTs (as evaluated by the reviewers).
- Full text available in English language.
Exclusion criteria
- Studies including < 5 patients with DLB.
- Studies only reporting on patients with dementia of mixed types (e.g., DLB and vascular dementia) or Parkinson’s disease dementia (PDD).
- Studies reporting on digital biomarkers primarily reflecting cognitive features of symptoms (as evaluated by the reviewers), e.g., number of spoken words per minute during cognitive tests.
Information sources and search strategy
We performed searches in MEDLINE and Embase using the Ovid-platform, and in Web of Science from inception to February 28, 2023. References cited in the included studies were also examined for eligibility. Search strategies and results are available in the Supplementary Material.
Selection process
Screening for eligibility was executed by two independent reviewers (NS, MG) using the web based systematic review tool Covidence®. A senior researcher (KF) was consulted in cases where consensus could not be reached between NS and MG. Papers with duplicate data but additional relevant data (e.g., data from different comparators) were included and designated accordingly. Otherwise, the paper based on the most complete dataset was included in the review.
Data collection process
Data was independently extracted by two reviewers (NS, MG) using a modified data extraction sheet in Microsoft Excel®. Any discrepancies upon comparison were resolved by the two reviewers. KF was consulted in cases of no consensus between NS and MG.
Data items
The following data items were extracted from the included reports: a) study design and purpose, b) eligibility criteria for included participants, c) number of participants, d) age and sex of participants, e) diagnosis of participants, f) cognitive scores of participants (e.g., Mini-Mental State Examination (MMSE) scores, or The Montreal Cognitive Assessment (MoCA) scores), g) symptoms assessed, h) DHT hardware and methods including period of measurement for obtainment of digital biomarker, i) DHT software, j) digital biomarker metrics, k) statistical analysis methods, l) reported values of the digital biomarkers measured, m) comparator, n) comparisons with validated symptom assessment (e.g., validated clinical rating scales or ancillary investigations), o) data loss/quality, p) safety assessments (e.g., adverse events), q) methods of assessment of feasibility (e.g., questionnaires, or drop-out), r) funding, s) reported conflicts of interests for study authors.
When data collected from patients with DLB were not reported for these participants specifically, biomarker values and comparisons with validated symptom assessment were not extracted, however, remaining data items were still extracted to identify relevant DHTs and to assess the feasibility of applying digital biomarkers in DLB. Biomarker values for comparators were extracted as a further measure of the ability of the biomarker to measure non-cognitive symptoms in patients with DLB.
Assessments of study quality
Two reviewers (NS, MG) carried out independent, qualitative assessments of the methodology of included studies using a modified check list (see Supplementary Material) adopting seven criteria from the National Institute of Health (NIH) Quality Assessment Tool for Observational Cohort and Cross-Sectional Studies [15]. Not all criteria were adopted as they were to be applied on studies of heterogenous design, and we did not investigate any exposures. Any discrepancies upon comparison were resolved by the two reviewers, and KF was consulted in cases of disagreement. Studies without extracted biomarker values for patients with DLB were not assessed.
Data synthesis
A narrative data synthesis was carried out. No assessment of meta-bias or strength of the body of evidence was made in this review.
RESULTS
The study selection process is illustrated in the PRISMA flow-chart in Fig. 1. We identified 4295 unique citations through database searches. After screening of title and abstract, 67 papers were retrieved for full text assessment of eligibility, resulting in 20 included studies. Of note, one study [16] did meet inclusion criteria but was excluded due to the results already being included in the study by Kazui et al. [17].
Fig. 1
PRISMA flow diagram of the screening process.
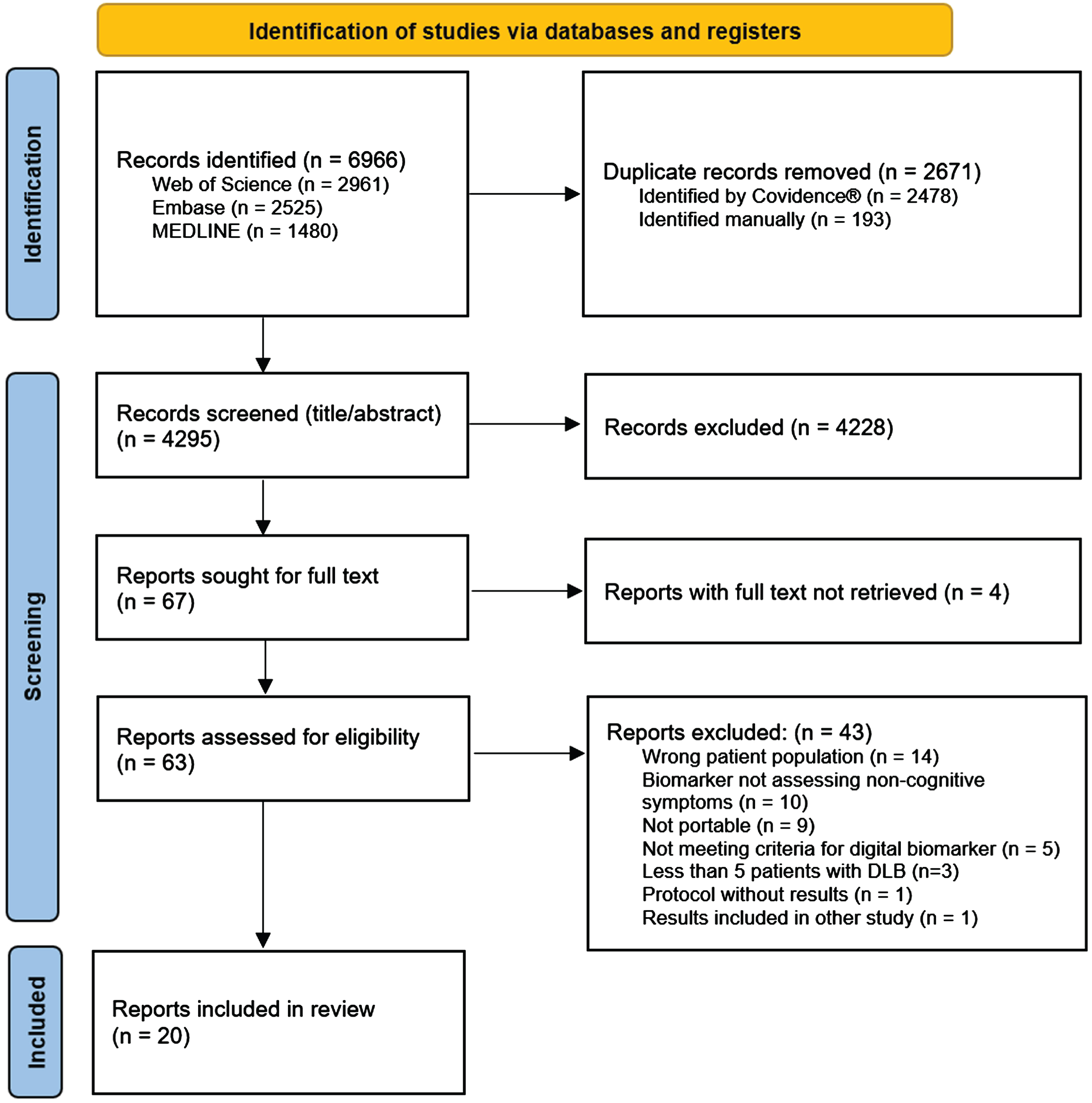
Study presentation
Table 2 gives the characteristics of the included studies. The DHTs applied in the included studies assessed the following groups of symptoms: fine motor skills (n = 4), sleep (n = 4), gait and balance (n = 3), dysautonomia (n = 3), neuropsychiatric symptoms (n = 2), physical activity and rest (n = 2), speech (n = 2), color vision impairment (n = 1), and hypomimia (n = 1). Four studies investigated interventions (pharmacological [17, 18], or non-pharmacological [19, 20]) where DHTs were used to assess outcomes, whereas the remaining studies had observational, cross-sectional designs. Four studies [18, 19, 21, 22] did not provide any subgroup analysis of DLB patients but were still included to identify relevant DHTs and assess the feasibility of applying digital biomarkers in DLB. Most studies were carried out in an outpatient setting, whereas one study investigated the application of DHTs in a hospital setting [20], and one study investigated patients in a long-term care facility [19].
Table 2
Characteristics of included studies
Fine motor skills | ||||
Reference | Study design | Study population | Digital health technology | Paradigm and digital biomarkers |
Bailon et al., 2010 [27] | Cross-sectional | DLB (n = 11), MCI (n = 18), AD (n = 23), controls (n = 52) Outpatient setting | Response button and laptop with Vision180© -software | Participants performed rapid index finger tapping with the preferred hand for measurement of tapping frequency. |
Kragh et al., 2018 [28] | Cross-sectional | DLB (n = 13), AD (n = 17), FTD (n = 19), healthy, aged controls (n = 15) Outpatient setting | Force transducer (Mini40) and laptop | Four simple motor tasks consisting of lifting the device or tapping with either finger, foot, or alternating hand were performed by participants. Both sides were assessed to obtain duration, frequency, interval, and force of tapping. For the lifting task, grip force magnitude, variability, and device movement were measured. |
Wang et al., 2022 [18]* | Randomized controlled trial | Participants with DLB or PDD (n = 238) Groups aggregated according to intervention, not clinical diagnosis (i.e., placebo or various dopaminergic drug doses) Outpatient setting | iPad using the Lilly trial app | Participants were asked to complete daily alternating index and middle finger tapping assessments on the device before, during, and after intervention. Assessments were conducted during a 16-weeks period to measure the total amount of taps per day. |
Yamada et al., 2022 [31]# | Cross-sectional | DLB (n = 27), AD (n = 47), healthy, aged controls (n = 49) Outpatient setting | Tablet with digital pen (Wacom Cintiq Pro 16) | Five commonly used drawing tasks (e.g., clock drawing) were performed with the device for measurement of drawing speed, speed variability and speed smoothness. Pressure variability and pressure smoothness were also assessed. |
Sleep | ||||
Reference | Study design | Study population | Digital health technology | Paradigm and digital biomarkers |
Fukuda et al., 2022 [20] | Interventional, non-randomized | Patients with DLB hospitalized for treatment of severe BPSD in a dementia-care unit (n = 18) | Pressure sensor (Nemuri SCAN) and a monitor | The device was placed under the participant’s mattress during their stay in the hospital to guide the nursing staff in care planning. Measurements included sleep duration, time spent awake, time between bedtime and sleep, times getting out of bed and sleep efficiency. |
Kazui et al., 2017 [17] | Interventional, non-randomized | DLB (n = 16), healthy, aged controls (n = 24) Outpatient setting | Inertial measurement unit (Actiwatch-L) | Participants wore the device for a full week on the non-dominant wrist, and patients were assessed again after taking donepezil for 14 weeks. All participants were monitored continuously to evaluate activity counts per minute, time in bed, total sleep time, sleep latency, snooze time, wake time after sleep onset, and sleep efficiency. |
Moyle et al., 2018 [19]* | Cluster-randomized controlled trial | DLB, AD, VaD, FTD or unspecified dementia (daytime analysis, n = 175, and nighttime analysis, n = 280) Groups aggregated according to intervention, not clinical diagnosis (i.e., usual care, sessions with regular plush toy, or sessions with PARO) Participants residing at Long Term Care facilities | Inertial measurement unit (SenseWear Professional 8.0) | Participants wore the device for 24 hours on the non-dominant triceps once before, twice during, and once after the 10-week intervention period. They were monitored continuously to evaluate time awake, time lying down, and time sleeping for day- and nighttime, respectively. |
Wang et al., 2022 [18]* | Randomized controlled trial | Participants with DLB or PDD (n = 160) Groups aggregated according to intervention, not clinical diagnosis (i.e., placebo or various dopaminergic drug doses) Outpatient setting | Inertial measurement unit (AX3-axivity) | Participants wore the device for two weeks on the non-dominant wrist once before, once during, and once after the 12-week intervention period. They were monitored continuously to evaluate time spent asleep during day and night, respectively. |
Gait and balance | ||||
Reference | Study design | Study population | Digital health technology | Paradigm and digital biomarkers |
Mc Ardle et al., 2019 [32]# | Cross-sectional | DLB (n = 28), PDD (n = 14), AD (n = 32) Outpatient setting | Inertial measurement unit (AX3 Axivity) | The device was fixed to the lumbar region, while participants performed six 10-meter walks in a lab setting for measurement of a total of 14 gait characteristics including means, variabilities, and asymmetries of step time, swing time, stance time, step velocity, and step length. |
Mc Ardle et al., 2021 [34]# | Cross-sectional | DLB (n = 28), AD (n = 32), healthy, aged controls (n = 25) Outpatient setting | Inertial measurement unit (AX3 Axivity) | The device was fixed to the lumbar region, while participants performed six 10-meter walks in a lab setting before wearing the device continuously for a week in a free-living environment. A total of 14 gait characteristics were measured including means, variabilities, and asymmetries of step time, swing time, stance time, step velocity, and step length. |
Mc Ardle et al., 2021 [35]# | Cross-sectional | DLB (n = 26), PDD (n = 13), AD (n = 31), healthy, aged controls (n = 27) Outpatient setting | Inertial measurement unit (AX3 Axivity) | The device was fixed to the lumbar region, while participants maintained an upright position for two minutes while having their balance assessed by means of acceleration magnitude and rate of change in both anteroposterior, mediolateral, and composite directions. |
Dysautonomia | ||||
Reference | Study design | Study population | Digital health technology | Paradigm and digital biomarkers |
Kim et al., 2015 [23] | Cross-sectional | DLB (n = 22), MCI (n = 24), SMI (n = 14), AD (n = 37), healthy, aged controls (n = 25) Outpatient setting | Sphygmomanometer (Mobil-O-Graph) | The device measured participants’ blood pressure every 15 minutes during day, and every 30 minutes during night for assessment of nighttime systolic blood pressure and change of mean blood pressure from day to night (dipping). |
Negami et al., 2013 [24] | Cross-sectional | DLB (n = 20), AD (n = 20) Outpatient setting | Heart rate variability: Acceleration plethysmograph and monitor Sympathetic skin response: surface electrodes (not specified) | Patients placed fingertip into the plethysmograph for two minutes to monitor and assess heart rate variability by low to high frequency power ratio (LF/HF) and the coefficient of variation of the A-A-intervals. Electrodes were placed on the hand for measurements of the sympathetic skin response by wave amplitude after electrical stimulation of the median nerve. |
Dysautonomia | ||||
Reference | Study design | Study population | Digital health technology | Paradigm and digital biomarkers |
Oka et al., 2020 [25] | Cross-sectional | DLB (n = 24), PD (n = 75) Outpatient setting | Blood pressure monitor (not specified) | The device measured participants’ blood pressure every 30 minutes during day and every 60 minutes during night for assessment of their nocturnal fall in systolic blood pressure (dipping). |
Neuropsychiatric symptoms | ||||
Reference | Study design | Study population | Digital health technology | Paradigm and digital biomarkers |
Moyle et al., 2018 [19]* | Cluster-randomized controlled trial | DLB, AD, VaD, FTD or unspecified dementia (daytime analysis, n = 175, and nighttime analysis, n = 280) Groups aggregated according to intervention, not clinical diagnosis (i.e., usual care, sessions with regular plush toy, or sessions with PARO) Participants residing at Long Term Care facilities | Inertial measurement unit (SenseWear Professional 8.0) | Participants wore the device for 24 hours on the non-dominant triceps once before, twice during, and once after the 10-week intervention period. They were monitored continuously to evaluate agitation in daytime and in nighttime by means of step count, and time with elevated physical activity level. |
Nagels et al., 2006 [21] | Cross-sectional | DLB (n = 16), AD (n = 65), FTD (n = 9), mixed dementia (n = 20) Outpatient setting | Inertial measurement unit (Octagonal basic motionlogger) | Participants wore the device fixed to the non-dominant wrist for 48 hours of continuous monitoring to assess agitation by times crossing a certain intensity threshold, time above this threshold, and movement intensity. |
Suzuki et al., 2017 [30] | Cross-sectional | DLB (n = 8), healthy controls (n = 9) Outpatient setting | Eye tracker (EMR-8) | Participants wore the eye tracker mounted on the head for video recording of their eyes, while they described 10 images. Their utterances were classified as correct or pareidolic, and video recordings prior to utterances were analyzed for saccadic movement frequency, saccadic angular velocity, and pupil diameter power change at 0-0.46 Hz after Fast Fourier transformation. |
Physical activity and rest | ||||
Reference | Study design | Study population | Digital health technology | Paradigm and digital biomarkers |
Mc Ardle et al., 2019 [33]# | Cross-sectional | DLB (n = 30), PDD (n = 16), AD (n = 36), healthy, aged controls (n = 26) Outpatient setting | Inertial measurement unit (AX3 Axivity) | Participants wore the device on their lower back for a full week of continuous monitoring in a free-living environment. Activity was measured by walk time, steps per day, bouts per day, time walking per day, mean bout duration, short/long bout ratio, and bout length variability. |
Wang et al., 2022 [18]* | Randomized controlled trial | Participants with DLB or PDD (n = 160) Groups aggregated according to intervention, not clinical diagnosis (i.e., placebo or various dopaminergic drug doses) Outpatient setting | Inertial measurement unit (AX3 Axivity) | Participants wore the device for two weeks on the non-dominant wrist once before, once during, and once after the 12-week intervention. They were monitored continuously to evaluate activity by daytime walking and average acceleration. |
Speech | ||||
Reference | Study design | Study population | Digital health technology | Paradigm and digital biomarkers |
Shellikeri et al., 2022 [29] | Cross-sectional | DLB (n = 23), PDD (n = 37) Outpatient setting | A microphone Software: Speech activity detector (LDC Hmm) and a pitch tracker for Praat | Audio recordings of participants describing a picture were analyzed for acoustic elements of speech, namely fundamental frequency range, and syllables per second. |
Yamada et al., 2022 [36]# | Cross-sectional | DLB (n = 27), AD (n = 45), cognitive normal controls (n = 49) | iPad Air 2 Software: Voice activity detector (IBM Watson), and audio processing libraries for Python (Librosa, Signal_Analysis) | Audio recordings of participants completing five speech tasks (e.g., picture description) on the tablet were analyzed for acoustic elements of speech, namely jitter (tone frequency variation), shimmer (tone amplitude variation). Additionally, phoneme rate was measured but only in picture description. |
Color vision impairment or hypomimia | ||||
Reference | Study design | Study population | Digital health technology | Paradigm and digital biomarkers |
Unger et al., 2019 [26] | Cross-sectional | DLB (n = 62), Pro-DLB (n = 25), AD (n = 24) Outpatient setting | Online version of the Farnsworth D-15 color vision test (Hardware not reported) | Participants were asked to arrange 15 colored tiles into an ordered color spectrum to examine the presence, severity, and type of color vision impairment. |
Jiang et al., 2022 [22] | Cross-sectional | non-AD dementia (including DLB among other etiologies) (n = 45), AD (n = 74), MCI-AD (n = 61), non-AD-MCI (e.g., due to CVD, alcohol abuse, or trauma) (n = 19) Outpatient setting | iPad Air 9.7 running iOS 10 or higher using the visMET-app | Participants’ faces were videorecorded while they passively observed first 20 images with 2-5 objects, then an altered set of the same images. Analyses of the facial expressions of participants were performed to determine the likelihoods of each of seven emotions being expressed. |
DLB, dementia with Lewy bodies; MCI, mild cognitive impairment; AD, Alzheimer’s disease; FTD, frontotemporal dementia; PDD, Parkinson’s disease dementia; BPSD, behavioral and psychological symptoms of dementia; VaD, vascular dementia; SMI, subjective memory impairment; PD, Parkinson’s disease; CVD, cardiovascular disease; Pro-DLB, MCI with REM sleep behavior disorder or parkinsonism but not meeting criteria for PD. *Record appears more than once in table. Several studies provided results on more than one digital biomarker and may occur under different symptom grouping accordingly. #Reporting on participants who are also included in other records by same lead author.
The number of patients with DLB in the included studies ranged from five to 62 with a median of 23. Additionally, one study [18] reported on measurements of 238 patients with Lewy body dementias (DLB or PDD). For participants with DLB, the mean and median age ranged from 66 to 80 years and mean and median MMSE score ranged from 16 to 26.5 reflecting a broad range of disease severity. In five studies, participants with DLB had a mean MMSE score below 22 [17, 23–26], whereas in 10 studies a mean score of 22 or greater was reported [27–36]. Comparisons with healthy controls was reported in 10 studies [17, 23, 27, 28, 30, 31, 33–36], and/or with patients with AD in 12 studies [21, 23, 24, 26–28, 31–36].
Digital health technologies
The various DHTs applied in the included studies are illustrated in Fig. 2, and their application is described in Table 2. Fifteen studies [17, 19–23, 27, 28, 30–36] reported sufficient details on DHT hardware for identification of the specific DHT, and 15 studies [17–19, 21, 22, 26–30, 32–36] reported on applied software, i.e., described algorithms or named computer programs used for data processing. A total of 17 different DHTs were applied in the included studies, but only one DHT (AX3 Axivity) was reported across multiple studies [18, 32–35]. An inertial measurement unit (IMU) was the most widely used type of DHT in the included studies with eight papers reporting biomarkers derived from IMUs [17–19, 21, 32–35]. An IMU, such as an actigraph, measures movement using accelerometry, and was applied in studies, which measured characteristics of balance, gait, agitation, and sleep utilizing varying hardware, software, and assessment paradigms. Indeed, most types of DHTs were reported to give rise to several digital biomarkers and have the potential to assess multiple symptoms.
Fig. 2
Graphical representation of applied DHTs. A graphical representation of the DHTs applied for symptom assessment in the included studies. An illustration might represent multiple DHTs if various assessments are described in the adjacent text. Some types of DHTs were applied across multiple studies as illustrated by the numbers in parentheses. DHT, digital health technology; PPG, photoplethysmograph. Created with BioRender.com.
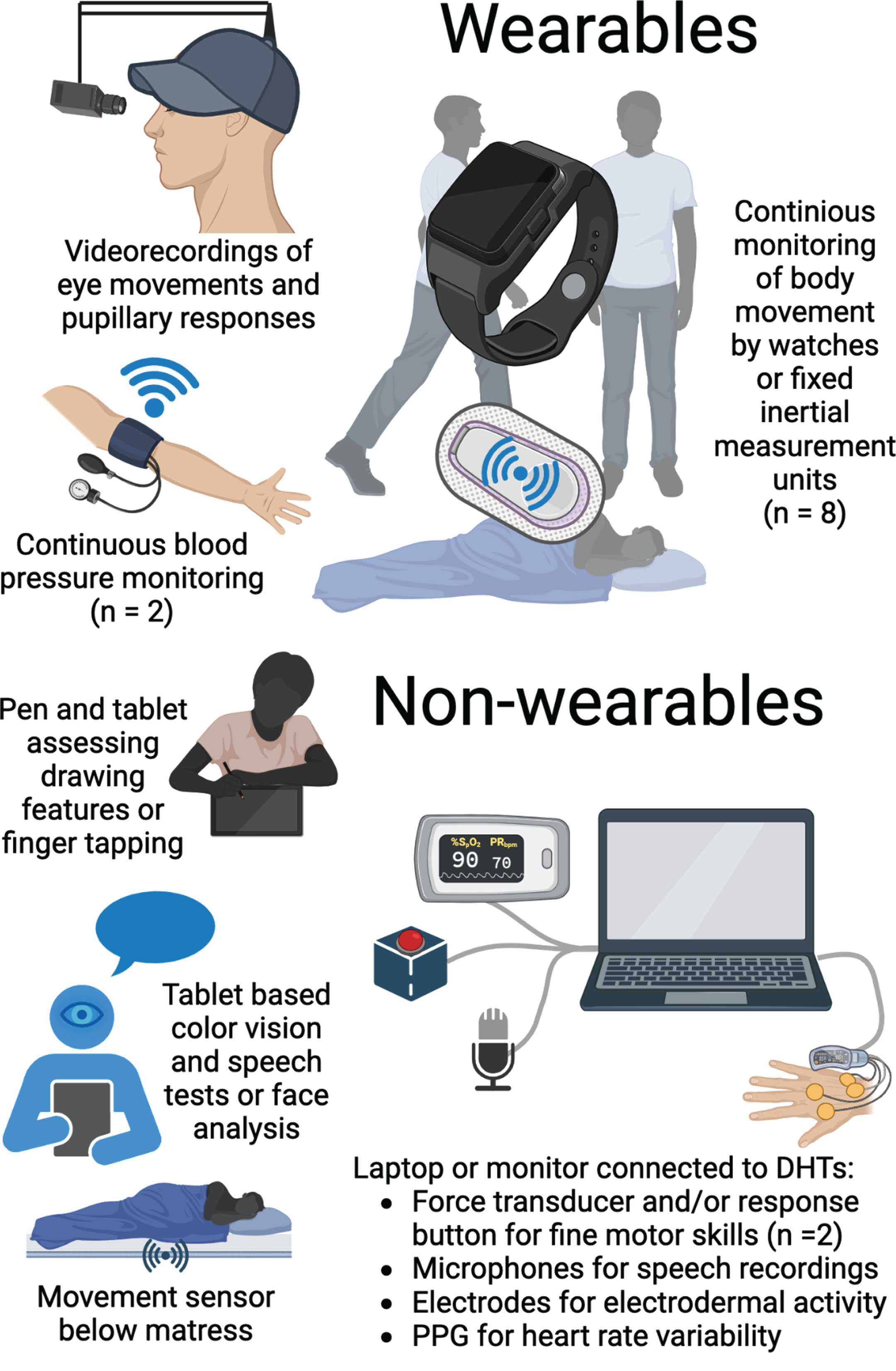
Digital biomarkers and corresponding symptoms
A full list of the investigated digital biomarkers derived from DHTs is given in Table 2. We divided symptoms assessed into nine different types as illustrated by the following examples: Biomarkers such as those representing characteristics of drawing (e.g., pressure applied by a digital pen) and other small movements (e.g., frequency of finger or alternating hand tapping) were grouped under fine motor skills. Sleep was assessed by means of e.g., sleep duration and efficiency. Biomarkers related to autonomic function (e.g., change of mean blood pressure from day to night) were grouped under dysautonomia, whereas biomarkers grouped under neuropsychiatric symptoms group include those reported by the authors as measures of e.g., agitation or pareidolias. Biomarkers reported as characteristics of gait (e.g., asymmetry of step time, or step length) or balance (e.g., acceleration in mediolateral direction) were similar in nature and grouped together accordingly. Biomarkers representing movement on a more general level (e.g., average acceleration per day, steps per day, and bout length variability) were grouped under physical activity and rest. Speech was assessed through, e.g., fundamental frequency range, and phoneme rate, while biomarkers grouped under color vision impairment was reported as such by the authors. Hypomimia was assessed using the likelihoods of different facial emotions expressed including neutral.
Discriminatory power of digital biomarkers
Seven studies [17, 28, 31, 33–36] comparing DLB to controls found group differences on at least one biomarker (Table 3). Assessments of fine motor skills, sleep, and gait or balance resulted in the highest number of different digital biomarker values between these two groups. In seven studies comparing DLB with AD [24, 26, 28, 31, 32, 34, 36], group differences in biomarker values were reported. These were mainly from assessments of fine motor skills, gait, dysautonomia, and color vision impairment (Table 3). In studies comparing DLB with both controls and AD, more biomarkers differed between DLB and controls than between DLB and AD, and DLB differed more significantly from controls than AD [27, 28, 31, 34–36] on the same biomarkers. Of note, four digital biomarkers differed between DLB and PDD (in assessment of gait, balance, and physical activity/rest) [32, 33, 35], one between DLB and Parkinson’s disease (PD, dysautonomia) [25], and one between DLB and frontotemporal dementia (fine motor skills) [28].
Table 3
Main findings from included studies
Fine motor skills | |||
Reference | Main results regarding DLB | Comparisons with validatedsymptom assessment | Feasibility aspects |
Bailon et al., 2010 [27] | No significant differences between groups were reported. | None reported | Not reported |
Kragh et al., 2018 [28] | DLB versus controls: multiple differences in alternating hand tapping, two in lifting, and one in foot tapping. DLB versus AD: inter-tap interval on the non-dominant side in alternating hand tapping differed. DLB versus FTD: inter-peak interval on the dominant side in foot tapping differed. | Motor task score coefficients of variation (range 0.26–0.34) were lower than those of clinical rating (UPDRS, UHDRS, finger tapping, tremor of hands, alternating pronation/supination of hand) for the DLB-group (range 0.49-1.58). | Reported as feasible in patients with dementia in general since AD-patients were able to perform well on the motor tasks despite having the most severe cognitive impairment. |
Wang et al., 2022 [18]* | No subgroup analysis for patients with DLB was reported. | None reported. | Compliance (daily test completion) dropped from 80% to 60% of participants during the study period. |
Yamada et al., 2022 [31]# | DLB versus controls: higher pressure variability, and reduced drawing speed and smoothness in DLB. DLB versus AD: reduced drawing speed and smoothness in DLB. | None reported | Not reported |
Sleep | |||
Reference | Main results regarding DLB | Comparisons with validated symptom assessment | Feasibility aspects |
Fukuda et al., 2022 [20] | Three main patterns of sleeping problems were identified. Digital sleep assessments sometimes differed from staff observations and helped guide in appropriate care planning. | None reported | For several patients, sufficient data (i.e., more than two weeks including the entire first week) could not be obtained. Reasons counting device malfunctioning, patient not sleeping in bed, and patient removing the device from under the bed. |
Kazui et al., 2017 [17] | Compared to controls, patients with DLB had a higher activity count during sleep, spent more time in bed, and slept for longer. Additionally, wake time after sleep onset, and activity counts per minute were reduced after taking donepezil. | The reduction in wake time after sleep onset was strongly correlated to the reduction in the Neuropsychiatric Inventory delusions score (rs = 0.613, p = 0.012). Associations with the Mayo Fluctuation Questionnaire and other Neuropsychiatric Inventory-scores including sleep disturbances were not significant. | In total, four nights of potential data was not obtained due to patients removing the device. |
Moyle et al., 2018 [19]* | No subgroup analysis for patients with DLB was reported. | None reported | After application of a wear-time criteria of 10 + hours, 42% of all participants were included in the daytime analysis (2 with DLB), and 67% in the nighttime analysis (5 with DLB). Issues included participants removing the device and having difficulties wearing it. The device regularly produced unreliable recordings (as evaluated by the authors), causing a substantial loss of data (not quantified). |
Wang et al., 2022 [18]* | No subgroup analysis for patients with DLB was reported. | None reported | Compliance with actigraphy was defined as 23 + hours per day of watch-wearing with > 90% of all participants achieving these requirements in each trial period. |
Gait and balance | |||
Reference | Main results regarding DLB | Comparisons with validated symptom assessment | Feasibility aspects |
Mc Ardle et al., 2019 [32]# | DLB versus PDD: less asymmetry of swing- and stance time in DLB. DLB versus AD: greater variability for step length and step velocity in DLB. No differences remained significant after multiple comparison adjustments. | Gait metrics derived from an instrumented walkway are reported for reference purposes. DLB differed from AD on one and not from controls on any of these metrics. | Six of the 80 enrolled participants were excluded from analysis due to technical issues with the device (not syncing, not turned on, or wrong trial time recorded). |
Mc Ardle et al., 2021 [34]# | Lab-based paradigm: DLB versus controls: Patients had greater variability for step length, step-, swing- and stance time and step velocity as well as more time in the step and swing phase with greater asymmetry for swing time. DLB versus AD: greater variability for step length and step velocity in DLB. Free-living paradigm: DLB versus controls: Patients walked slower, had shorter steps and greater variability of pace. DLB versus AD: no significant differences. A few differences became significant when excluding bouts of duration longer than 10 seconds. | None reported | Lab-based paradigm: Six of the 95 enrolled participants were excluded from analysis due to technical issues with the device (not syncing, not turned on, or wrong trial time recorded). Free-living paradigm: Four of the 95 enrolled participants were excluded from analysis due to missing data. Issues were problems with data upload, unwillingness to wear sensor, and monitor loss. Five participants had < 7 but > 3 days of useful data due to discomfort (n = 1), hospitalization (n = 1), and inadequate data quality (not specified) (n = 3). |
Mc Ardle et al., 2021 [35]# | DLB versus controls: Patients showed greater postural instability in all directions. DLB versus PDD: Less mediolateral instability in DLB. No differences remained significant after multiple comparison adjustments. | No significant associations with the Activities-specific Balance Confidence scale, or the UPDRS-III (motor examination). | Thirteen of the 110 enrolled participants were unable to complete balance assessments. |
Dysautonomia | |||
Reference | Main results regarding DLB | Comparisons with validated symptom assessment | Feasibility aspects |
Kim et al., 2015 [23] | No significant group differences, but nighttime blood pressure dipping showed a tendency towards lower values in groups with more cognitive impairment. | No significant associations. Decreased nighttime blood pressure dipping tended to correlate with spontaneous features of parkinsonism (not specified). | Not reported |
Negami et al., 2013 [24] | Patients with DLB had decreased amplitude of the sympathetic skin response as well as decreased LF/HF-ratio on the heart rate variability assessment when compared to patients with AD. | None reported | Not reported |
Oka et al., 2020 [25] | Patients with DLB were more likely than those with PD to be classified as non-dippers because their nocturnal blood pressure dropped less or even rose. | No significant associations. Prevalence of dysautonomia by means of hypotension, orthostatic (head-up-tilt-table test) or postprandial (oral glucose tolerance test), was higher in the DLB-group. Also, their supine BP (head-up-tilt-table test) was higher. | Not reported |
Neuropsychiatric symptoms | |||
Reference | Main results regarding DLB | Comparisons with validated symptom assessment | Feasibility aspects |
Moyle et al., 2018 [19]* | No subgroup analysis for patients with DLB was reported. | None reported | After application of a wear-time criteria of 10 + hours, 42% of all participants were included in the daytime analysis (2 with DLB), and 67% in the nighttime analysis (5 with DLB). Issues included participants removing the device and having difficulties wearing it. The device regularly produced unreliable recordings (as evaluated by the authors), causing substantial data loss (not quantified). |
Nagels et al., 2006 [21] | No subgroup analysis for patients with DLB was reported. | None reported | Not reported |
Suzuki et al., 2017 [30] | Pupil diameter power change differed between correct and pareidolic utterances in the DLB-group. No differences compared to controls. | None reported | Not reported |
Physical activity and rest | |||
Reference | Main results regarding DLB | Comparisons with validated symptom assessment | Feasibility aspects |
Mc Ardle et al., 2019 [33]# | DLB versus controls: DLB were less active and had lower bout length variability. DLB versus PDD: DLB had relatively more long than short bouts. | Activities-specific Balance Confidence scale score was moderately strongly correlated with bouts per day (r = 0.509, p = 0.004), and walking duration (r = 0.412, p = 0.024), and weakly correlated with Steps per day (r = 0.373, p = 0.042). Additionally, UPDRS-III-score showed weak correlations with bouts per day (r=–0.385, p = 0.039), and walking time (r=–0.382, p = 0.041). | Four of the 112 enrolled participants were excluded from analysis due to missing data. Issues were problems with data upload, unwillingness to wear sensor, and monitor loss. Additionally, five participants had < 7 but > 3 days of useful data due to discomfort (n = 1), hospitalization (n = 1), and inadequate data quality (not specified) (n = 3). |
Wang et al., 2022 [18] | No subgroup analysis for patients with DLB was reported. | None reported | Compliance with actigraphy was defined as 23 + hours per day of watch-wearing with > 90% of all participants achieving these requirements in each trial period. |
Speech | |||
Reference | Main results regarding DLB | Comparisons with validated symptom assessment | Feasibility aspects |
Shellikeri et al., 2022 [29] | No significant differences between groups were reported. | None reported | Not reported |
Yamada et al., 2022 [36]# | DLB versus controls: Patients had more jitter in one speech task, and a lower phoneme rate. DLB versus AD: more jitter in one speech task in DLB. | None reported | Of eight participants not completing every speech task, three patients had DLB, and they completed four tasks each. Issues were verbal refusals or failure to follow instructions. |
Color vision impairment or hypomimia | |||
Reference | Main results regarding DLB | Comparisons with validated symptom assessment | Feasibility aspects |
Unger et al., 2019 [26] | DLB versus AD: higher prevalence and severity of color vision impairment in DLB DLB versus pro-DLB: higher prevalence of color vision impairment in DLB (p = 0.05) | No significant associations were found between presence of color vision impairment and visual hallucinations, probable RBD, or autonomic dysfunction. | Not reported |
Jiang et al., 2022 [22] | No subgroup analysis for patients with DLB was reported. | None reported | Not reported |
Main results include only significant results if not otherwise stated. Due to space limitations, insignificant results are not always presented, but all investigated biomarkers are mentioned in Table 2. For comparisons of digital biomarkers with validated symptom assessments, only results specific to the DLB-group were reported. DLB, dementia with Lewy bodies; AD, Alzheimer’s disease; FTD, frontotemporal dementia; PDD, Parkinson’s disease dementia; UPDRS, Unified Parkinson’s disease rating scale; UHDRS, Unified Huntington’s disease rating scale; PD, Parkinson’s disease. *Record appears more than once in table. Several studies provided results on more than one digital biomarker and may occur under different symptom grouping accordingly. #Reporting on participants who are also included in other records by same lead author.
Comparisons with validated symptom assessment methods
Eight studies [17, 23, 25, 26, 28, 32, 33, 35] compared results from digital biomarkers in patients with DLB to validated symptom assessment methods (Table 3). One study [33] found that digital biomarkers assessing physical activity showed moderately strong, positive correlations with self-reported ability to maintain balance as measured with the Activities-specific Balance Confidence (ABC) scale. In the same study, weak correlations were found between digital biomarkers assessing physical activity and motor symptoms according to clinical evaluation using the UPDRS-III. One digital biomarker assessing sleep was significantly correlated to delusional behavior as assessed with the Neuropsychiatric Inventory (NPI) [17]. In contrast, Kazui et al., who reported these findings, also reported scores from assessments of sleep disturbances using the NPI to be reduced after taking donepezil, but changes of the digital biomarkers which was applied in the trial were not significantly associated to these changes. Of interest, Kragh et al. reported that the variability of certain digital biomarkers assessing fine motor skills (e.g., frequency of finger tapping, and frequency of alternating pronation/supination of hand) were lower than the variability of results from corresponding clinical assessments [28]. Consequently, the authors suggest that digital biomarkers could be more sensitive to small changes that would go undetected by regular clinical examination.
Aspects of feasibility
Data on feasibility of DHTs in the studied patient population was sparse and not collected systematically. Most studies reported pooled results on the whole study population, whereby specific data on aspects of feasibility in patients with DLB could not be extracted (Table 3). In general, data was obtained from assessments of most of the included participants in almost all studies.
A single study, by Negami et al., explicitly reported on adverse events, and participants in this study did not experience adverse events in relation to measurement of symptoms of dysautonomia using automated blood pressure monitoring [24]. We found no studies purposely reporting on participant experience by e.g., questionnaires. However, five studies made mention of user related issues regarding usability of DHTs applied for continuous monitoring (i.e., discomfort [33], unwillingness to wear the DHT [34], difficulties wearing the DHT [19], or participants removing the DHT [17, 19, 20]) but did not provide additional details. Aside from these user related issues, difficulties in the obtainment of useful data were mainly reported as related to technical problems (e.g., device malfunctioning [20], data upload issues [33, 34]), or poor data quality. Specifically, two studies raised concerns about the reliability of DHTs concerning data quality [19, 33], while the remaining did not report on this matter at all. One study [33] quantified the amount of data evaluated as being of inadequate quality, but details from data quality assessments were not reported in any studies. Regarding the two randomized controlled trials using DHTs to assess the effects of interventions, no drop-out were reported to be related to the DHT in one study [19], and reasons for drop-out or non-compliance were not reported on in the other study [18].
Two studies considered feasibility of the applied DHT to be limited based on the reported amount of data loss due to user related issues or low-quality data. Moyle et al. [19] reported almost half of participants not wearing the device for at least 10 of the 12 planned hours, which the authors required for inclusion in the analysis, and Fukuda et al. [20] report unreliable data from the DHT in addition to the DHT not being able to monitor participants due to their behavior. Of note, Wang et al. [18] reported a drop from 80% to 60% of participants adhering to daily finger tapping test completions during the 16-week study period but the authors did not consider this indicative of limited feasibility of applying the digital biomarker for assessment of the effect of the pharmacological intervention.
Results from assessments of study quality
The results of the study methodology assessments are presented in Fig. 3. Four studies were not assessed since no biomarker values for patients with DLB were extracted [18, 19, 21, 22]. In general, studies performed well on providing a clearly stated research question/objective, applying uniform eligibility criteria for all participants, and implementing clearly defined outcomes. In contrast, most studies failed to provide sample size justifications, blind outcome assessors from participant diagnosis, and account for key potential confounders in the statistical analyses. Half of the studies clearly specified the study population.
Fig. 3
Quality assessments of included studies. Results from application of the modified check list for assessment of study methodology. Each column represents a criterion from the check list. Studies marked with YES did meet the criterion, whereas studies marked with NO did not. The applied check list is available in the Supplementary Material.
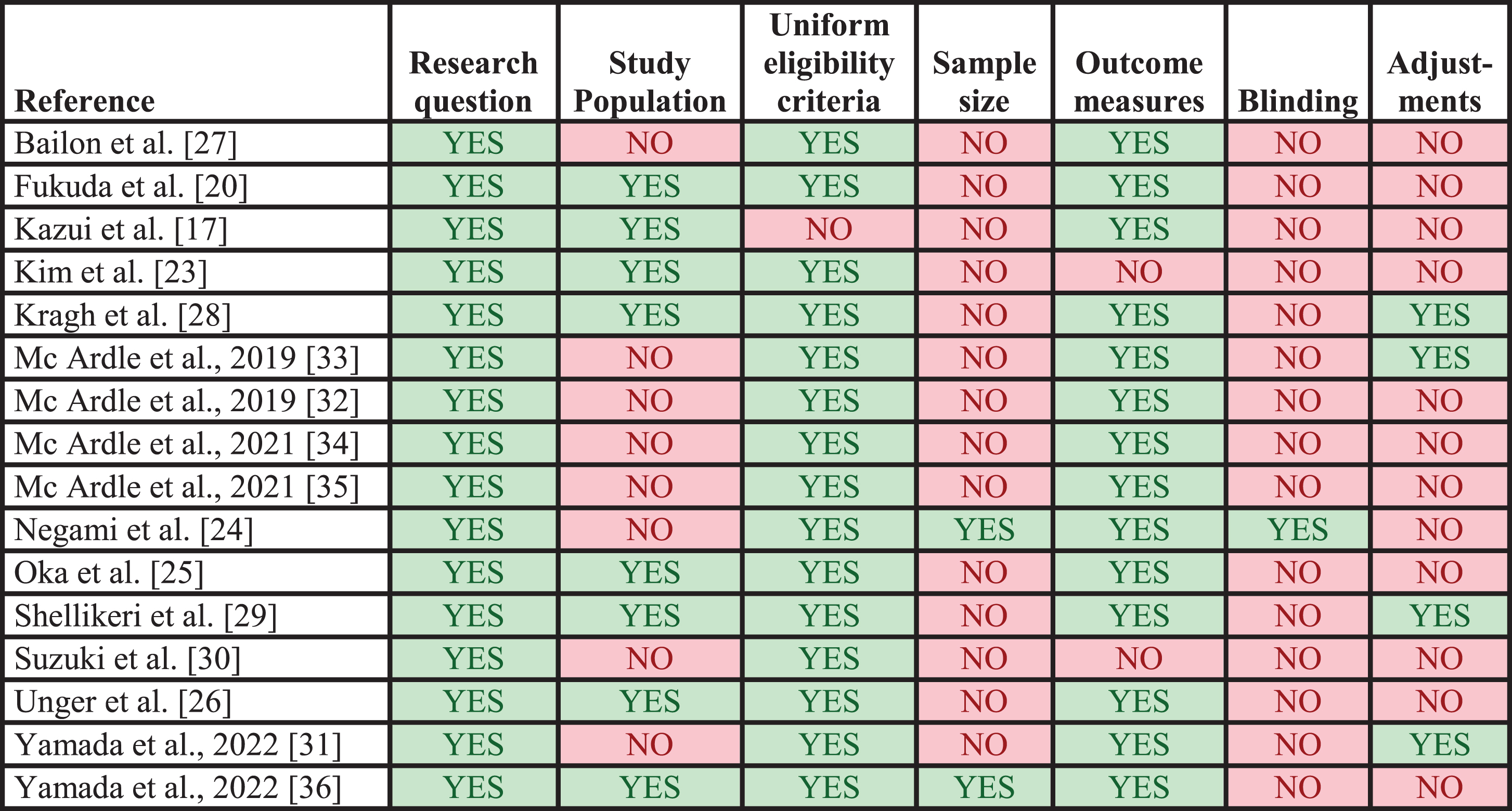
DISCUSSION
We conducted a systematic review on digital biomarkers for the assessment of non-cognitive symptoms in patients with DLB. A wide variety of different DHTs were used across studies mostly assessing motor symptoms. Digital biomarkers assessing physical activity and fine motor skills showed reasonable comparability when compared with other methods for assessment of similar symptoms in patients with DLB, but such comparisons were seldom reported on. Issues related to feasibility were due to technical problems or participants not being compliant to continuous monitoring. However, feasibility was not reported on in a systematic way, and not in patients with DLB, specifically.
So far, data on digital biomarkers related to non-cognitive symptoms in DLB is limited. Reviews show that research in this area extends further in AD [37, 38] and especially in PD [39–43], which however share some symptoms with DLB [44, 45]. In AD and PD, comparable (and sometimes identical) DHTs have been used for assessments of similar symptoms as those in the included studies, e.g., IMUs for assessment of gait [46, 47], sleep [48, 49] and activity [50, 51], and dysautonomia assessed using photoplethysmography [52] or blood pressure monitors [53]. Our findings align with those in AD and PD. Specifically, a vast assortment of DHTs and paradigms have been used, which is a barrier to establish a broad evidence base for specific DHTs that show promise and in terms of comparing DHTs which has been done in relatively few studies [54–57]. We identified no studies directly comparing portable or wearable DHTs in DLB, but one study [32] showed that a wearable IMU used for assessment of gait was superior to an instrumented walkway in terms of providing significant biomarker differences between DLB and other diagnostic groups. Although two studies [17, 20] reported on identical outcomes (i.e., sleep duration and sleep efficiency) in DLB, populations and settings of the studies differed noticeably (i.e., admission in a dementia care unit versus home-based monitoring) thus limiting the value of comparing the DHTs applied. Future research in digital biomarkers in DLB might benefit from the efforts that have been made to compare different DHTs by using a numbered scale reflecting their level of readiness for clinical use in PD [58], although we did not examine thisaspect.
Most common types of symptoms in DLB were investigated, however considering core clinical features of DLB, we did not identify DHTs assessing fluctuations or REM sleep behavior disorder although application of IMUs in measurements of patients with PD have shown high specificity for RBD [59]. Moreover, certain symptoms considered as supportive clinical features of DLB such as constipation or hyposmia [4] were not assessed by any of the included studies. Neither have such symptoms been assessed in patients with PD or AD [37, 39, 40]. Existing wearable DHTs and digital biomarkers might however be used such as a smart belt for assessment of gastrointestinal symptoms [39], and a vocal biomarker for detection of hyposmia [60].
In general, most studies did not report comparisons of digital biomarkers with other methods of symptom assessments in patients with DLB specifically, or they reported comparisons that were not evidently suitable for evaluation of the digital biomarkers’ ability to measure symptoms (e.g., correlations between parkinsonian symptoms and decreased fall in nighttime blood pressure [23], and NPI delusions score and features of sleep [17]). In this regard, evidence to proper evaluate digital biomarkers’ ability to capture non-cognitive symptoms in DLB is lacking. This illustrates a need for a more standardized approach in the validation of digital biomarkers for symptom assessment in DLB. Indeed, the lack of standardization has been raised as a general concern regarding development of novel digital biomarkers, and a checklist to ensure proper validation have been provided for the design of future clinical trials [61]. Ideally, new biomarkers should be validated against current gold standards for symptom assessment.
As all but two studies were cross-sectional, limited evidence is available on the longitudinal application of the investigated digital biomarkers in DLB (i.e., sensitivity to symptom progression) advising the need for prospective study designs in future assessment of the most promising DHT’s. It has been suggested that test-retest repeatability and sensitivity to symptom progression is a valuable and important approach for validating biomarkers [40], and future studies could incorporate these aspects to elucidate this further.
Uncertainty remains as to whether most of the applied DHTs can be considered feasible for use in patients with DLB as data are not reported for key aspects such as adverse events, user experiences, and data quality assessments. Feasibility of applying digital biomarkers in PD [42, 43] or cognitive impairment [62] have been reviewed with similar findings. Of interest, our findings on amounts of data lost due to human factors using wearables matched those of similar studies in patients with PD and were lower than in studies on stroke-patients [43]. This suggests that DHTs could be applied in assessments of patients with DLB on the same terms as in the mentioned patients without dementia, which might make portable and wearable DHTs preferable to demanding methods for obtainment of biomarkers in patient with dementia.
The relevance of specific information on feasibility remains as minor issues such as discomfort or subjective preferences in relation to device usage is of importance to patients, and such information might help in ensuring data collection. Indeed, it has been possible to identify barriers for using DHTs by people with dementia and addressing these barriers have resulted in higher patient satisfaction and a reduction in data lost due to human factors as reported in [43, 51, 63]. One approach to ensure systematic data collection of aspects of feasibility is to include patient reported outcome measures (PROMs) specifically on the use of DHTs for symptom assessment. Such PROMs have been assessed in studies including patients with PD and AD using questionnaires and interviews [51, 64], and similar assessments could be applied in future studies including patients with DLB.
None of the identified studies specifically aimed to investigate DHTs in early versus late disease stages. Between studies, there were differences on mean cognitive performance but there were no tendencies to be detected in terms of which DHTs were applied nor for measures of diagnostic accuracy. However, there were indications of reduced feasibility in studies reporting on populations with more severe behavioral disturbances. It is likely that different DHTs are relevant in early compared to late disease stages, but more studies are needed to further elucidate this.
We recognize that the present review is not without limitations. For instance, there is a possibility of publication bias, since studies on DHTs resulting in adverse events or without significant, positive outcomes might not have been submitted, an aspect we did not investigate by means of meta-analysis, which was not pre-planned. Another limitation is that we included studies not reporting results for patients with DLB explicitly, which might have skewed our findings. Finally, studies not published in English language were not considered due to lack of resources, which might limit retrieval of relevant studies. Despite these shortcomings, this review offers a useful summary of the field and provides important recommendations for future research to attend to. Strengths of this review included a well-planned, rigorous approach and our broad search strategy encompassing several databases, ensuring adequate capture of relevant literature.
Research on digital biomarkers from wearable or portable DHTs in patients with DLB is still in its very infancy in the assessment of the non-cognitive symptoms. Most non-cognitive symptoms have been assessed, but the field is very diverse, without standardized protocols, and thus no broad evidence base exists for individual, digital biomarkers. Currently, no fully validated methods for assessments of non-cognitive symptoms in DLB by wearable or portable DHTs exist and means of feasibility were not formally assessed in the included studies. Thus, there is a need for more knowledge about feasibility and validity of symptom assessment before conclusions can be made concerning the use of digital biomarkers in DLB. Future studies should focus on closing these gaps before digital biomarkers may enter routine clinical practice, ideally by systematically applying outcomes to evaluate feasibility and validity. Our findings support proposed recommendations for future research in wearable DHTs emphasizing the importance of standardization of evaluation of digital biomarkers [42].
AUTHOR CONTRIBUTIONS
Nikolai Sulkjær Sjælland (Conceptualization; Data curation; Formal analysis; Investigation; Methodology; Project administration; Visualization; Writing – original draft); Mathias Holsey Gramkow (Conceptualization; Investigation; Methodology; Writing – review & editing); Steen Gregers Hasselbalch (Conceptualization; Methodology; Writing – review & editing); Kristian Steen Frederiksen (Conceptualization; Methodology; Supervision; Writing – review & editing).
ACKNOWLEDGMENTS
We thank information specialist Trine Kæstel at Rigshospitalet Medical Library for her help with developing search strings.
FUNDING
The study was supported by a grant from the Lundbeck Foundation.
CONFLICTS OF INTEREST
The authors have no conflict of interest to report.
DATA AVAILABILITY
The data supporting the findings of this study are available on request from the corresponding author. The data are not publicly available due to privacy or ethical restrictions.
SUPPLEMENTARY MATERIAL
[1] The supplementary material is available in the electronic version of this article: https://dx.doi.org/10.3233/JAD-240327.
REFERENCES
[1] | McKeith I , Mintzer J , Aarsland D , Burn D , Chiu H , Cohen-Mansfield J , Dickson D , Dubois B , Duda JE , Feldman H , Gauthier S , Halliday G , Lawlor B , Lippa C , Lopez OL , Carlos MacHado J , O’Brien J , Playfer J , Reid W ((2004) ) Dementia with Lewy bodies. Lancet Neurol 3: , 19–28. |
[2] | McKeith IG , Galasko D , Kosaka K , Perry EK , Dickson DW , Hansen LA , Salmon DP , Lowe J , Mirra SS , Byrne EJ , Lennox G , Quinn NP , Edwardson JA , Ince PG , Bergeron C , Burns A , Miller BL , Lovestone S , Collerton D , Jansen ENH , Ballard C , De Vos RAI , Wilcock GK , Jellinger KA , Perry RH ((1996) ) Consensus guidelines for the clinical and pathologic diagnosis of dementia with Lewy bodies (DLB). Neurology 47: , 1113–1124. |
[3] | Mueller C , Ballard C , Corbett A , Aarsland D ((2017) ) Historical landmarks in dementia with Lewy bodies. Lancet Neurol 16: , 348. |
[4] | McKeith IG , Boeve BF , Dickson DW , Halliday G , Taylor J-P , Weintraub D , Aarsland D , Galvin J , Attems J , Ballard CG , Bayston A , Beach TG , Blanc F , Bohnen N , Bonanni L , Bras J , Brundin P , Burn D , Chen-Plotkin A , Duda JE , El-Agnaf O , Feldman H , Ferman TJ , ffytche D , Fujishiro H , Galasko D , Goldman JG , Gomperts SN , Graff-Radford NR , Honig LS , Iranzo A , Kantarci K , Kaufer D , Kukull W , Lee VMY , Leverenz JB , Lewis S , Lippa C , Lunde A , Masellis M , Masliah E , McLean P , Mollenhauer B , Montine TJ , Moreno E , Mori E , Murray M , O’Brien JT , Orimo S , Postuma RB , Ramaswamy S , Ross OA , Salmon DP , Singleton A , Taylor A , Thomas A , Tiraboschi P , Toledo JB , Trojanowski JQ , Tsuang D , Walker Z , Yamada M , Kosaka K ((2017) ) Diagnosis and management of dementia with Lewy bodies. Neurology 89: , 88–100. |
[5] | Fujishiro H , Iseki E , Nakamura S , Kasanuki K , Chiba Y , Ota K , Murayama N , Sato K ((2013) ) Dementia with Lewy bodies: Early diagnostic challenges. Psychogeriatrics 13: , 128–138. |
[6] | Fujishiro H , Nakamura S , Sato K , Iseki E ((2015) ) Prodromal dementia with Lewy bodies. Geriatr Gerontol Int 15: , 817–826. |
[7] | Van De Beek M , Van Steenoven I , Ramakers IHGB , Aalten P , Koek HL , Olde Rikkert MGM , Manniën J , Papma JM , De Jong FJ , Lemstra AW , Van Der Flier WM ((2019) ) Trajectories and determinants of quality of life in dementia with Lewy bodies and Alzheimer’s disease. J Alzheimers Dis 70: , 387–395. |
[8] | Allan L , McKeith I , Ballard C , Kenny RA ((2006) ) The prevalence of autonomic symptoms in dementia and their association with physical activity, activities of daily living and quality of life. Dement Geriatr Cogn Disord 22: , 230–237. |
[9] | Post B , Merkus MP , de Bie RMA , de Haan RJ , Speelman JD ((2005) ) Unified Parkinson’s disease rating scale motor examination: Are ratings of nurses, residents in neurology, and movement disorders specialists interchangeable? Mov Disord 20: , 1577–1584. |
[10] | Waldemar G ((2023) ) Data-driven care for patients with neurodegenerative disorders. Nat Rev Neurol 19: , 447–448. |
[11] | Vasudevan S , Saha A , Tarver ME , Patel B ((2022) ) Digital biomarkers: Convergence of digital health technologies and biomarkers. NPJ Digit Med 5: , 36. |
[12] | Inan OT , Tenaerts P , Prindiville SA , Reynolds HR , Dizon DS , Cooper-Arnold K , Turakhia M , Pletcher MJ , Preston KL , Krumholz HM , Marlin BM , Mandl KD , Klasnja P , Spring B , Iturriaga E , Campo R , Desvigne-Nickens P , Rosenberg Y , Steinhubl SR , Califf RM ((2020) ) Digitizing clinical trials. NPJ Digital Med 3: , 101. |
[13] | Page MJ , McKenzie JE , Bossuyt PM , Boutron I , Hoffmann TC , Mulrow CD , Shamseer L , Tetzlaff JM , Akl EA , Brennan SE , Chou R , Glanville J , Grimshaw JM , Hróbjartsson A , Lalu MM , Li T , Loder EW , Mayo-Wilson E , McDonald S , McGuinness LA , Stewart LA , Thomas J , Tricco AC , Welch VA , Whiting P , Moher D ((2021) ) The PRISMA 2020 statement: An updated guideline for reporting systematic reviews. BMJ 372: , n71. |
[14] | McKeith IG , Dickson DW , Lowe J , Emre M , O’Brien JT , Feldman H , Cummings J , Duda JE , Lippa C , Perry EK , Aarsland D , Arai H , Ballard CG , Boeve B , Burn DJ , Costa D , Del Ser T , Dubois B , Galasko D , Gauthier S , Goetz CG , Gomez-Tortosa E , Halliday G , Hansen LA , Hardy J , Iwatsubo T , Kalaria RN , Kaufer D , Kenny RA , Korczyn A , Kosaka K , Lee VMY , Lees A , Litvan I , Londos E , Lopez OL , Minoshima S , Mizuno Y , Molina JA , Mukaetova-Ladinska EB , Pasquier F , Perry RH , Schulz JB , Trojanowski JQ , Yamada M ((2005) ) Diagnosis and management of dementia with Lewy bodies. Neurology 65: , 1863–1872. |
[15] | Study Quality Assessment Tools | NHLBI, NIH, https://www.nhlbi.nih.gov/health-topics/study-quality-assessment-tools |
[16] | Kanemoto H , Kazui H , Adachi H , Yoshiyama K , Wada T , Nomura KT , Shimosegawa E , Ikeda M ((2020) ) Thalamic pulvinar metabolism, sleep disturbances, and hallucinations in dementia with Lewy bodies: Positron emission tomography and actigraphy study. Int J Geriatr Psychiatry 35: , 934–943. |
[17] | Kazui H , Adachi H , Kanemoto H , Yoshiyama K , Wada T , Tokumasu Nomura K , Tanaka T , Ikeda M ((2017) ) Effects of donepezil on sleep disturbances in patients with dementia with Lewy bodies: An open-label study with actigraphy. Psychiatry Res 251: , 312–318. |
[18] | Wang J , Battioui C , McCarthy A , Dang X , Zhang H , Man A , Zou J , Kyle J , Munsie L , Pugh M , Biglan K ((2022) ) Evaluating the use of digital biomarkers to test treatment effects on cognition and movement in patients with Lewy body dementia. J Parkinsons Dis 12: , 1991–2004. |
[19] | Moyle W , Jones C , Murfield J , Thalib L , Beattie E , Shum D , O’Dwyer S , Mervin MC , Draper B ((2018) ) Effect of a robotic seal on the motor activity and sleep patterns of older people with dementia, as measured by wearable technology: A cluster-randomised controlled trial. Maturitas 110: , 10–17. |
[20] | Fukuda C , Higami Y , Shigenobu K , Kanemoto H , Yamakawa M ((2022) ) Using a non-wearable actigraphy in nursing care for dementia with Lewy bodies. Am J Alzheimers Dis Other Demen 37: , doi:10.1177/15333175221082747. |
[21] | Nagels G , Engelborghs S , Vloeberghs E , Van Dam D , Pickut BA , De Deyn PP ((2006) ) Actigraphic measurement of agitated behaviour in dementia. Int J Geriatr Psychiatry 21: , 388–393. |
[22] | Jiang Z , Seyedi S , Haque RU , Pongos AL , Vickers KL , Manzanares CM , Lah JJ , Levey AI , Clifford GD ((2022) ) Automated analysis of facial emotions in subjects with cognitive impairment. PLoS One 17: , e0262527. |
[23] | Kim JS , Park HE , Oh YS , Song IU , Yang DW , Park JW , Lee KS ((2015) ) 123I-MIBG myocardial scintigraphy and neurocirculatory abnormalities in patients with dementia with Lewy bodies and Alzheimer’s disease. J Neurol Sci 357: , 173–177. |
[24] | Negami M , Maruta T , Takeda C , Adachi Y , Yoshikawa H ((2013) ) Sympathetic skin response and heart rate variability as diagnostic tools for the differential diagnosis of Lewy body dementia and Alzheimer’s disease: A diagnostic test study. BMJ Open 3: , e001796. |
[25] | Oka H , Umehara T , Nakahara A , Matsuno H ((2020) ) Comparisons of cardiovascular dysautonomia and cognitive impairment between de novo Parkinson’s disease and de novo dementia with Lewy bodies. BMC Neurol 20: , 350. |
[26] | Unger RH , Flanigan PM , Khosravi M , Leverenz JB , Tousi B ((2019) ) Clinical and imaging characteristics associated with color vision impairment in Lewy body disease. J Alzheimers Dis 72: , 1233–1240. |
[27] | Bailon O , Roussel M , Boucart M , Krystkowiak P , Godefroy O ((2010) ) Psychomotor slowing in mild cognitive impairment, Alzheimer’s disease and Lewy body dementia: Mechanisms and diagnostic value. Dement Geriatr Cogn Disord 29: , 388–396. |
[28] | Jeppesen Kragh F , Bruun M , Budtz-Jørgensen E , Hjermind LE , Schubert R , Reilmann R , Nielsen JE , Hasselbalch SG ((2018) ) Quantitative measurements of motor function in Alzheimer’s disease, frontotemporal dementia, and dementia with Lewy bodies: A proof-of-concept study. Dement Geriatr Cogn Disord 46: , 168–179. |
[29] | Shellikeri S , Cho S , Cousins KAQ , Liberman M , Howard E , Balganorth Y , Weintraub D , Spindler M , Deik A , Lee EB , Trojanowski JQ , Irwin D , Wolk D , Grossman M , Nevler N ((2022) ) Natural speech markers of Alzheimer’s disease co-pathology in Lewy body dementias. Parkinsonism Relat Disord 102: , 94–100. |
[30] | Suzuki Y , Hirayama K , Shimomura T , Uchiyama M , Fujii H , Mori E , Nishio Y , Iizuka O , Inoue R , Otsuki M , Sakai S ((2017) ) Changes in pupil diameter are correlated with the occurrence of pareidolias in patients with dementia with Lewy bodies. Neuroreport 28: , 187–192. |
[31] | Yamada Y , Kobayashi M , Shinkawa K , Nemoto M , Ota M , Nemoto K , Arai T ((2022) ) Characteristics of drawing process differentiate Alzheimer’s disease and dementia with Lewy bodies. J Alzheimers Dis 90: , 693–704. |
[32] | Mc Ardle R , Del Din S , Galna B , Thomas A , Rochester L ((2020) ) Differentiating dementia disease subtypes with gait analysis: Feasibility of wearable sensors? Gait Posture 76: , 372–376. |
[33] | Mc Ardle R , Del Din S , Donaghy P , Galna B , Thomas A , Rochester L ((2020) ) Factors that influence habitual activity in mild cognitive impairment and dementia. Gerontology 66: , 197–208. |
[34] | Mc Ardle R , Del Din S , Donaghy P , Galna B , Thomas AJ , Rochester L ((2021) ) The impact of environment on gait assessment: Considerations from real-world gait analysis in dementia subtypes. Sensors 21: , 813. |
[35] | Mc Ardle R , Pratt S , Buckley C , Del Din S , Galna B , Thomas A , Rochester L , Alcock L ((2021) ) Balance impairments as differential markers of dementia disease subtype. Front Bioeng Biotechnol 9: , 639337. |
[36] | Yamada Y , Shinkawa K , Nemoto M , Ota M , Nemoto K , Arai T ((2022) ) Speech and language characteristics differentiate Alzheimer’s disease and dementia with Lewy bodies. Alzheimers Dement (Amst) 14,: , e12364. |
[37] | Cote AC , Phelps RJ , Kabiri NS , Bhangu JS , Thomas K ((2021) ) Evaluation of wearable technology in dementia: A systematic review and meta-analysis. Front Med (Lausanne) 7: , 501104. |
[38] | Husebo BS , Heintz HL , Berge LI , Owoyemi P , Rahman AT , Vahia IV ((2020) ) Sensing technology to facilitate behavioral and psychological symptoms and to monitor treatment response in people with dementia: A systematic review. Front Pharmacol 10: , 482618. |
[39] | van Wamelen DJ , Sringean J , Trivedi D , Carroll CB , Schrag AE , Odin P , Antonini A , Bloem BR , Bhidayasiri R , Chaudhuri KR ((2021) ) Digital health technology for non-motor symptoms in people with Parkinson’s disease: Futile or future? Parkinsonism Relat Disord 89: , 186–194. |
[40] | Morgan C , Rolinski M , McNaney R , Jones B , Rochester L , Maetzler W , Craddock I , Whone AL ((2020) ) Systematic review looking at the use of technology to measure free-living symptom and activity outcomes in Parkinson’s disease in the home or a home-like environment. J Parkinsons Dis 10: , 429–454. |
[41] | Godinho C , Domingos J , Cunha G , Santos AT , Fernandes RM , Abreu D , Gonçalves N , Matthews H , Isaacs T , Duffen J , Al-Jawad A , Larsen F , Serrano A , Weber P , Thoms A , Sollinger S , Graessner H , Maetzler W , Ferreira JJ ((2016) ) A systematic review of the characteristics and validity of monitoring technologies to assess Parkinson’s disease. J Neuroeng Rehabil 13,: , 24. |
[42] | Del Din S , Godfrey A , Mazzà C , Lord S , Rochester L ((2016) ) Free-living monitoring of Parkinson’s disease: Lessons from the field. Mov Disord 31: , 1293–1313. |
[43] | Johansson D , Malmgren K , Alt Murphy M ((2018) ) Wearable sensors for clinical applications in epilepsy, Parkinson’s disease, and stroke: A mixed-methods systematic review. J Neurol 265: , 1740–1752. |
[44] | Aldridge GM , Birnschein A , Denburg NL , Narayanan NS ((2018) ) Parkinson’s disease dementia and dementia with Lewy bodies have similar neuropsychological profiles. Front Neurol 9: , 123. |
[45] | Walker L , Stefanis L , Attems J ((2019) ) Clinical and neuropathological differences between Parkinson’s disease, Parkinson’s disease dementia and dementia with Lewy bodies - current issues and future directions. J Neurochem 150: , 467–474. |
[46] | Ellis RJ , Ng YS , Zhu S , Tan DM , Anderson B , Schlaug G , Wang Y ((2015) ) A validated smartphone-based assessment of gait and gait variability in Parkinson’s disease. PLoS One 10: , e0141694. |
[47] | Hsu YL , Chung PC , Wang WH , Pai MC , Wang CY , Lin CW , Wu HL , Wang JS ((2014) ) Gait and balance analysis for patients with Alzheimer’s disease using an inertial-sensor-based wearable instrument. IEEE J Biomed Health Inform 18: , 1822–1830. |
[48] | Aharon-Peretz J , Masiah A , Pillar T , Epstein R , Tzischinsky O , Lavie P ((1991) ) Sleep-wake cycles in multi-infarct dementia and dementia of the Alzheimer type. Neurology 41: , 1616–1619. |
[49] | Stavitsky K , Saurman JL , McNamara P , Cronin-Golomb A ((2010) ) Sleep in Parkinson’s disease: A comparison of actigraphy and subjective measures. Parkinsonism Relat Disord 16: , 280–283. |
[50] | Kirste T , Hoffmeyer A , Koldrack P , Bauer A , Schubert S , Schröder S , Teipel S ((2014) ) Detecting the effect of Alzheimer’s disease on everyday motion behavior. J Alzheimers Dis 38: , 121–132. |
[51] | Brem AK , Kuruppu S , de Boer C , Muurling M , Diaz-Ponce A , Gove D , Curcic J , Pilotto A , Ng WF , Cummins N , Malzbender K , Nies VJM , Erdemli G , Graeber J , Narayan VA , Rochester L , Maetzler W , Aarsland D ((2023) ) Digital endpoints in clinical trials of Alzheimer’s disease and other neurodegenerative diseases: Challenges and opportunities. Front Neurol 14: , 1210974. |
[52] | Alice de Vilhena Toledo M , Fernando Junqueira Jr L , Luiz Junqueira Jr PF ((2008) ) Cardiac sympathovagal modulation evaluated by short-term heart interval variability is subtly impaired in Alzheimer’s disease. Geriatr Gerontol Int 8: , 109–118. |
[53] | Hellman AM , Shah SP , Pawlowski SM , Duda JE , Morley JF ((2015) ) Continuous non-invasive monitoring to detect covert autonomic dysfunction in Parkinson’s disease. Parkinsonism Relat Disord 21: , 723–728. |
[54] | Wallén MB , Dohrn IM , Ståhle A , Franzén E , Hagströmer M ((2014) ) Comparison of pedometer and accelerometer derived steps in older individuals with Parkinson’s disease or osteoporosis under free-living conditions. J Aging Phys Act 22: , 550–556. |
[55] | Lord S , Rochester L , Baker K , Nieuwboer A ((2008) ) Concurrent validity of accelerometry to measure gait in Parkinson’s disease. Gait Posture 27: , 357–359. |
[56] | Esser P , Dawes H , Collett J , Feltham MG , Howells K ((2012) ) Validity and inter-rater reliability of inertial gait measurements in Parkinson’s disease: A pilot study. J Neurosci Methods 205: , 177–181. |
[57] | Wallén MB , Nero H , Franzén E , Hagströmer M ((2014) ) Comparison of two accelerometer filter settings in individuals with Parkinson’s disease. Physiol Meas 35: , 2287. |
[58] | Sánchez-Ferro Á , Elshehabi M , Godinho C , Salkovic D , Hobert MA , Domingos J , van Uem JMT , Ferreira JJ , Maetzler W ((2016) ) New methods for the assessment of Parkinson’s disease (2005 to 2015): A systematic review. Mov Disord 31: , 1283–1292. |
[59] | Louter M , Arends JB , Bloem BR , Overeem S ((2014) ) Actigraphy as a diagnostic aid for REM sleep behavior disorder in Parkinson’s disease. BMC Neurol 14: , 76. |
[60] | Higa E , Elbéji A , Zhang L , Fischer A , Aguayo GA , Nazarov PV , Fagherazzi G ((2022) ) Discovery and analytical validation of a vocal biomarker to monitor anosmia and ageusia in patients with COVID-19: Cross-sectional study. JMIR Med Inform 10: , e35622. |
[61] | Kruizinga MD , Stuurman FE , Exadaktylos V , Doll RJ , Stephenson DT , Groeneveld GJ , Driessen GJA , Cohen AF ((2020) ) Development of novel, value-based, digital endpoints for clinical trials: A structured approach toward fit-for-purpose validation. Pharmacol Rev 72: , 899–909. |
[62] | Holthe T , Halvorsrud L , Lund A ((2022) ) Digital assistive technology to support everyday living in community-dwelling older adults with mild cognitive impairment and dementia. Clin Interv Aging 17: , 519–544. |
[63] | Boyle LD , Husebo BS , Vislapuu M ((2022) ) Promotors and barriers to the implementation and adoption of assistive technology and telecare for people with dementia and their caregivers: A systematic review of the literature. BMC Health Serv Res 22: , 1573. |
[64] | Ferreira JJ , Godinho C , Santos AT , Domingos J , Abreu D , Lobo R , Gonçalves N , Barra M , Larsen F , Fagerbakke Ø , Akeren I , Wangen H , Serrano JA , Weber P , Thoms A , Meckler S , Sollinger S , van Uem J , Hobert MA , Maier KS , Matthew H , Isaacs T , Duffen J , Graessner H , Maetzler W ((2015) ) Quantitative home-based assessment of Parkinson’s symptoms: The SENSE-PARK feasibility and usability study. BMC Neurol 15: , 89. |