Embodied Spatial Navigation Training in Mild Cognitive Impairment: A Proof-of-Concept Trial
Abstract
Background:
Egocentric and allocentric spatial memory impairments affect the navigation abilities of older adults with mild cognitive impairment (MCI). Embodied cognition research hints that specific aids can be implemented into virtual reality (VR) training to enhance spatial memory.
Objective:
In this study, we preliminarily tested ‘ANTaging’, an embodied-based immersive VR training for egocentric and allocentric memory, compared to treatment as usual (TAU) spatial training in MCI.
Methods:
MCI patients were recruited for this controlled trial. A cognitive battery was administered at pre-test, after ten sessions of ANTaging or TAU intervention, and at 3-month follow-up (FU). The primary outcomes were spatial cognition tests (Corsi supra-span, CSS; Manikin test, MT). VR egocentric and allocentric performance was also collected.
Results:
We found that ANTaging significantly improved MT scores at FU compared to TAU. CSS slightly improved in both groups. Concerning secondary outcomes, auditory-verbal forgetting significantly improved at post-test in the ANTaging but not TAU group and significantly declined at FU in the TAU but not in the ANTaging group. Global cognition significantly improved at FU for TAU and remained stable for ANTaging. Other tests showed no improvement or deterioration. Clinical significance showed that ANTaging is effective for CSS. Virtual egocentric and allocentric memory performance improved across ANTaging sessions.
Conclusions:
ANTaging holds the potential to be superior for improving spatial cognition in MCI compared to TAU. Embodied cognition research provides insights for designing effective spatial navigation rehabilitation in aging.
INTRODUCTION
Spatial navigation is a complex and essential aspect of our daily functioning. During navigation, individuals encode, retain, and recall their position and route by using environmental cues, primarily local landmarks (e.g., a monument) and boundaries (e.g., walls, fences), as well as self-motion (bodily from now on) cues such as motor commands, proprioception, and vestibular information [1, 2]. This spatial information can be structured using the egocentric representation (body-dependent) and the allocentric representation (body-independent) [2]. In particular, environmental cues allow to correct spatial estimations provided by bodily information alone [3]. Research has shown that encoding and remembering locations relative to local landmarks involves the use of an egocentric representation. In contrast, engaging with the boundaries of the environment involves allocentric representation [4–6]. In the former a set of sensory states and actions are associated with a discrete landmark (e.g., “to reach the museum, I need to turn left at the monument”), in the latter case, locations are represented based on their specific allocentric direction and distance from a boundary. Crucially, the flexible and successful use of both representations is supported by a reciprocal egocentric-allocentric transition [7, 8].
A consistent body of literature indicates that a decline in spatial navigation can be considered one of the early indicators of progression from normal to pathological aging [1]. The mild cognitive impairment (MCI) syndrome stands as a critical stage in the continuum between typical aging and full-blown dementia and is considered a target population for non-pharmacological preventive interventions [9]. Crucially, both individuals with amnestic and non-amnestic MCI phenotypes report subjective navigation difficulties [10]. Indeed, a recent systematic review [11] found that individuals with MCI exhibit impairments in the ability to use both egocentric and allocentric spatial frames of reference, as well as difficulties in switching between them. Moreover, egocentric and allocentric deficits have been reported in both Alzheimer’s disease (AD) [12–14] and non-AD-related [15, 16] MCI syndrome.
Virtual reality (VR) occupies a significant position among advanced technologies, offering important benefits for implementing innovative spatial training in neurological conditions [17]. Another potential application facilitated using VR is the incorporation of navigational aids. Embodied cognition approaches to spatial cognition suggest that the body plays a key role in cognitive representations of the space and that encoding modality (e.g., bodily, visual information, symbolic format) of the environment might influence the formation of a modality-dependent cognitive map and subsequent spatial memory recall [18, 19]. Therefore, embodied cognition theories suggest that the cognitive representation of the environment can be enhanced by specific cues: bodily [19] and visual-cognitive [20]. The latter type can be thought of as a spatial affordance where patients could offload cognitive effort into the virtual environment. For instance, symbolic (e.g., arrows, maps) and environmental (salient landmarks/markers) affordances can be used to improve the representation of the space [21] and support immediate action through perception [22]. Conversely, the involvement of bodily information can be conceptualized as a form of virtual enactment [23, 24]. Virtual enactment is provided through input devices (joypad)/VR apparatus (especially with immersive VR) and is an excellent opportunity to enhance encoding through sensorimotor information and support memory retrieval with useful traces in the brain [25].
Evidence supports the idea that providing bodily information during virtual navigation, by exploiting immersive VR or the motor interaction with input devices (e.g., joypad, driving simulator), has been shown to improve spatial memory in MCI [25, 26]. The use of bodily cues during the encoding of a virtual spatial navigation task, compared to a condition in which only visual information (i.e., a passive navigation like a passenger on a car) was provided, led to enhanced spatial recall in this population [23, 25, 26]. Moreover, the association between VR immersion and the ability to use bodily information during spatial navigation tasks follows a linear relationship (e.g., the greater the immersion the greater the bodily representation of the navigation) [27]. Regarding visual-cognitive aids, assessment studies in MCI have shown that providing directional cues or salient cues to allocate attention to landmarks during virtual navigation has a positive impact on subsequent spatial memory recall [20, 28]. Conversely, the presence of an interactive bird-view map could be effective depending on its characteristics, such as the degree of interaction and details [20, 29].
Despite the increasing evidence of spatial navigation impairments in MCI, only one trial has been carried out in this population so far. Park [30] tested the usefulness of a 2D VR desktop spatial navigation training in amnestic MCI (aMCI) compared to a waiting list group. In this group, patients waited until the VR group completed 24 sessions. During training sessions, patients were immersed in an arena with allocentric cues (i.e., circular boundaries) and were asked to collect, across multiple trials, a series of gems presented one at a time and then go back to the starting location. After 24 sessions, patients in the VR group improved on visuospatial ability and verbal memory cognitive tests and virtual allocentric performance. Concerning the bodily cues used in this trial, patients were required to use the joypad while interacting with a low degree of immersion, consequently, only motor commands in addition to visual information were added to navigation to create a cognitive map of the virtual environment. Regarding visual-cognitive aids, only visual feedback concerning the patient’s response was given (no maps, directional cues, or spatial attention markers). Despite the results being encouraging, this trial only trained allocentric performance and used non-immersive VR (i.e., low bodily involvement) without visual-cognitive aids.
The objective of this study is to explore the preliminary short and mid-term efficacy and feasibility of ANTaging (Active Navigation Training in aging) [31] compared to standard “paper and pencil” training for spatial functions. The first version of the ANTaging software has been validated after a usability study in MCI [32] and the version used in this trial was improved after patients’ suggestions.
We expect that ANTaging will lead to greater improvement in long-term visuospatial memory and spatial manipulation abilities (primary outcomes) compared to the standard training at post-test. We expect maintenance at the post-test of the other cognitive tests (secondary outcomes) after both training types. Moreover, since ANTaging tracks egocentric and allocentric performance, we expect that these abilities will both increase across the training sessions. Lastly, we want to explore if results in primary and secondary outcomes are maintained, improved, or deteriorated at mid-term follow-up (FU).
METHODS
Participants
A total of 47 participants with a diagnosis of MCI were recruited for this study (see the Supplementary Material for CONSORT flowchart) from 1 April 2020 to 31 March 2023. Enrollment and planning were carried out according to COVID-19 Italian safety restrictions. A total of 30 patients with MCI were included in the analyses described below. Table 1 reports the demographics and clinical baseline characteristics of the participants included in the analyses.
Table 1
Descriptive statistics before the interventions
Variable | TAU, N = 16 | ANTaging, N = 14 | p |
Age (y) | 75 (6) | 74 (7) | 0.7 |
Sex | 0.14 | ||
F | 10 (62%) | 5 (36%) | |
M | 6 (38%) | 9 (64%) | |
Education (y) | 11 (5) | 11 (4) | 0.8 |
MMSE (points) | 25.80 (2.88) | 25.95 (3.01) | 0.9 |
SR-I (points) | 4.11 (2.65) | 4.61 (2.53) | 0.6 |
SR-D (points) | 4.68 (3.24) | 3.50 (2.99) | 0.3 |
CBT (points) | 4.05 (1.23) | 4.24 (0.78) | 0.6 |
CSS (points) | 7.5 (3.2) | 8.4 (4.5) | 0.5 |
DSF (points) | 5.37 (0.98) | 5.61 (0.81) | 0.5 |
TMT-A (s) | 71 (34) | 43 (14) | 0.001 |
TMT-B (s) | 172 (103) | 154 (141) | 0.7 |
TMT-BA (s) | 109 (83) | 115 (130) | 0.9 |
MT (accuracy) | 0.70 (0.23) | 0.69 (0.24) | >0.9 |
GDS (points) | 5 (3) | 5 (2) | >0.9 |
ADL (points) | 6 (0) | 6 (1) | 0.3 |
IADL (points) | 7 (2) | 7 (2) | 0.8 |
MMSE, Mini-Mental State Examination; SR-I, story recall immediate; SR-D, story recall delayed; CBT, Corsi block tapping test; CSS, Corsi supra-span test; DSF, digit span forward; TMT-AB, Trail Making Test (part A, part B, part BA) MT, manikin test; GDS, Geriatric Depression Scale brief form; ADL, Activities of Daily Living; IADL, Instrumental Activities of Daily Living; TAU, treatment as usual. p-values were computed with two sample t-test and Pearson’s Chi-squared test.
MCI was determined by a clinical neuropsychologist and the treating physician (EP, KMG, CSB), according to the patient history, neurological referral, and clinical neuropsychological diagnosis. The core clinical criteria of Albert and colleagues [33] were used to diagnose MCI syndrome: 1) concern about a change in cognition expressed by the patient, an informant, or a clinician; 2) cognitive impairment in one or more domains. Objective impairment was determined (liberal criteria) when one or more tests of the neuropsychological battery had an equivalent score (ES) ≤ 1 [34]; 3) maintenance of independence in functional abilities; and 4) absence of dementia diagnosis. Age above or equal to 60 years old and the absence of abnormal global cognition as determined by the Italian Mini-Mental Score Examination (MMSE) cut-off [35] were additional inclusion criteria. Winblad and colleagues’ [36] criteria were used to determine aMCI or non-amnestic phenotype.
The following conditions were required for exclusion: 1) the presence of an acute stroke or transient ischemic attack; 2) the presence of other comorbid severe neurological (e.g., multiple sclerosis, brain tumor, Huntington’s disease) and psychiatric disorders (e.g., schizophrenia) or non-treated psychiatric conditions (e.g., mood and anxiety disorders); 3) the history of traumatic brain injury with loss of consciousness; 4) physical or functional impairments (e.g., musculoskeletal disorders, limb paresis/plegia or paraesthesia) that could impair the use of VR and experimental procedure; 5) an uncorrected visual impairment; and 6) the presence of self-reported recurrent vertigo confirmed by objective neurological examination.
To compute the sample size, we used the medium effect size reported by Serino and colleagues [29] on a similar spatial memory training. For a mixed (3 within × 2 between), the final sample is 28. Considering a drop-out rate of 0.1 (28/0.9) a preferred sample size is approximately 32.
Participants were recruited at the Inpatient and Outpatient Clinic of the Department of Geriatrics and Cardiovascular Medicine, IRCCS Istituto Auxologico Italiano— Mosè Bianchi, Milan. The study was conducted following the Declaration of Helsinki and approved by the Ethics Committee of Istituto Auxologico Italiano (code: 2019_05_21_04) and registered in clinicalTrials.gov (NCT05768620). Written informed consent was obtained from the participants before they participated in the study.
Materials
A battery of seven neuropsychological tests was administered at pre-test, post-test, and 3-month FU. The assessment included tests of global cognition (MMSE), visuospatial short-term memory (Corsi block tapping test, CBT) [37] auditory-verbal short-term memory (digit span forward, DSF) [37], visuospatial long-term memory (Corsi supra-span, CSS) [38], auditory-verbal long-term memory (story recall immediate, delayed, and forgetting, SR-I, SR-D, SR-F) [39], executive functions/attention (Trail Making Test part A, part B, and part BA, TMT-AB) [40], spatial mental rotation (manikin test, MT) [41]. In addition to the MT total score, we computed derived scores depending on the manikin orientation: manikin front upward (Mfu), manikin back upward (Mbu), manikin front downward (Mfd), manikin back downward (Mbd). Pre-test assessment included a short version of the Geriatric Depression Scale (GDS) [42] and the Activity of Daily Living (ADL), and instrumental ADL [43, 44].
Concerning the primary outcomes, to assess visuospatial long-term memory, we used the CSS (score 1.08–29.16; greater score better performance). We employed the Italian CSS validation by Spinnler and Tognoni [38] with a correction by age, education level, and CBT span. In this test, patients are required to learn a fixed supra-span Corsi block [37] sequence within eighteen trials. For spatial mental rotation, we used the MT (correct proportion 0–1; greater score better performance). We employed the standard MT version [41]. In this test, the patient is presented with an upward or downward and front or back-facing manikin holding a black circle. The participant must tell in which hand the manikin is holding the black circle. We computed derived scores depending on the manikin orientation: Mfu, Mbu, Mfd, Mbd. MT is considered a task of egocentric transformation, where participants had to mentally change their egocentric coordinates to a new set of egocentric ones to answer correctly [45]. This change is sustained by additional allocentric processing that supports egocentric translocation [7]. Therefore, the Mbu condition requires no egocentric transformation (same coordinates as the patient), the Mbd requires no egocentric transformation but a vertical egocentric rotation (downward to upward), the Mfu requires egocentric transformation alone, and the Mfd requires egocentric transformation plus a vertical egocentric rotation. Details of the other tests are reported in the Supplementary Material.
General procedure
Participants were allocated to the ANTaging or TAU group with a non-randomized method [46]. Decisions for allocation were carried out taking into consideration usual/concurrent treatments, patients’ preferences [46], and any restrictions due to the pandemic. The former and the latter decision was taken by a clinical psychologist (CSB) and the principal investigator (CT) before the pre-test, whereas the patients could prefer standard ‘paper and pencil’ methods, try a new technological solution, or have no preference. The experimenter and statistician (CT) was not blinded regarding participant allocation.
Before the start of the study, patients read and signed the consent form to participate in the ANTaging protocol [47]. Pre-test and post-test battery were administered on separate days, so the entire program lasted twelve sessions. Each session in the TAU or ANTaging condition lasted approximately 45 min and each patient was asked to attend three training sessions per week. The fading cues and errorless learning method [48] were implemented in both interventions at the recall phase. Both interventions were standardized in terms of difficulty, fading cues, and errorless learning across the rehabilitation sessions. The whole program lasted 3/4 weeks approximately. After a 3-month FU, a testing session was scheduled if patients were available. From pre-test to FU all patients were not involved in any cognitive training program (pharmacological, psychological, or physical therapy were allowed).
ANTaging procedure
ANTaging technological set-up. ANTaging [47] was developed within the “Active Navigation Training: An Innovative Embodied-Based Training System for Spatial Navigation in Aging” project (code: SG-2018-12368175). The training was carried out using an immersive VR (cave automated virtual environment; CAVE) apparatus described in detail in the usability study [32]. Patients explored the virtual environment using a foot-motion pad (3dRudder) and 3D glasses. The 3dRudder is a circular platform that is used while sitting in a comfortable and safe position. The patient places the feet on the top to use it. It is in turn fixed on a semi-spheric lower section. This solution allows the user to manage the movement intuitively, tilting the feet in the desired direction (e.g., pointing the feet toes to the right to turn right, pushing the feet toes down to move forward).
ANTaging task structure. The virtual training scenario consisted of a modified version of a landmark and boundary-based spatial memory recall navigation task [6]. Patients were required to collect four items (one at a time) and memorize their locations in a circular city square during the encoding phase (the diameter of the square was 50 virtual meters). Object locations could be learned using the boundaries of the arena (i.e., square arcade), an intra-arena landmark (i.e., obelisk), and distal cues (i.e., mountain range, clouds). Items were randomly presented, and each object was collected four times in random order. To see the object, the participants had to ‘walk’ with the 3dRudder to the exact location of the item. Once over it, the object disappeared, and the patient had to find the next one. During the immediate recall phase, participants had to recall and go to the exact location where the item (shown at the bottom of the screen) was previously collected and press the ‘a’ button to respond on the joypad. Then the following object was shown. In a counterbalanced order, either the wall or the obelisk was removed. This forced the use of allocentric (i.e., arcade) or egocentric (i.e., obelisk) spatial memory recall [4]. Each object was tested two times with the egocentric and allocentric spatial recall cue for a total of 16 trials (eight trials for each allocentric and egocentric recall condition). The response variable was the Euclidean distance error for each object trial at recall (distance in virtual meters of the recalled position from the actual location). An invisible wall was used as a constraint during the egocentric condition so that both recalls werecomparable.
ANTaging rehabilitative procedure. Before the start of the encoding phase, a short spatial attention task was carried out in the CAVE. Participants were required to look for, while in the middle of the circular square, six markers (orange spheres) located one above the intra-arena landmark, four equidistant above the arcade of the square, and one above the distal mountain range. Markers were numbered and once found (patients had to spell out the number), the experimenter made them disappear to start the encoding phase.
During the encoding phase, patients were aided with a directional cue (yellow line) to search and follow to find the object to collect. This line provided the heading direction toward the item. Figure 1 shows the encoding environment with the directional cue.
Fig. 1
Encoding virtual environment.

The need to provide MCI patients with attentional and directional cues has been documented previously and allows them to reduce executive load during spatial memory tasks [19]. Such embodied cues (i.e., directional and attentive cues) allow to off-load cognitive load onto the (virtual) environment and can be used as spatial affordance (visual cues for action) and reduce executive load during virtual navigation [19]. Moreover, the use of immersive VR allowed the patients to recruit bodily information and enable, like a form of (virtual) enactment, deeper encoding and effortful retrieval thanks to sensorimotor traces at both memory phases [23, 24].
The encoding sessions were all the same during the ten training sessions, whereas the recall sessions were modified using the fading cues (i.e., correct location marker; a white circle in the virtual environment indicating the correct object location) and errorless learning method [48] in a standardized manner across participants. From sessions one to two, patients were asked to look for the white circle that represented the correct location of the item shown on the CAVE screen and remember the location. From sessions three to five, patients were asked to try to find the correct location of the item shown. The white marker was shown to provide visual feedback once the participant was satisfied with the recalled position or if cannot remember the object’s location. Such fading cues and errorless learning methods were removed after the first five sessions to have a pure metric of the embodied cues at encoding. Thus, from sessions six to ten, they could only rely on the software feedback (a correct response was within a six virtual meter radius from the encoding location). In all sessions, as an interference task between the encoding and recall phase, patients were asked to read a short story (2 minutes). See the Supplementary Material for specific training instructions given to the patients.
Standard ‘paper and pencil’ procedure
We used materials from a previous spatial memory rehabilitation [49], which was comparable to the usual (TAU) spatial memory training at the Outpatient Clinic of the Department of Geriatrics and Cardiovascular Medicine, IRCCS Istituto Auxologico Italiano— Mosè Bianchi, Milan. Patients were provided with a visuospatial grid memory task and a visuospatial scene memory task of increasing difficulty. The difficulty was standardized for all the patients and manipulated for the grid (complexity of the pattern inside the grid and number of stimuli inside) and real-life drawing (number of stimuli inside the picture). The visuospatial grid memory task was composed of an encoding session of five minutes, then an interference task (find all the numbers in a matrix of letters), then a recognition task (select the correct matrix without the items inside), then a free recall of the item/s and the respective grid location/s, and lastly a visual confrontation task between the encoded and recalled grid. Similarly, the visuospatial scene memory task was composed of an encoding phase of five minutes, then an interference task (find all the numbers in a matrix of letters), then a free recall of all the possible items, in the spatial location recall (the same picture was provided but a blank space was put above four item locations) patients had to select among a list the correct items and their locations, and lastly a visual confrontation task. As for the ANTaging procedure, recall cues (semantic or phonetic) were provided concerning the items to recall in both tasks only for the first five sessions.
Statistical analyses
R software (v 3.6.3) was used to analyze the data. Analyses were carried out for each of the outcome measures (neuropsychological tests) with a linear mixed-effects ANOVA [50]. Normality for pre-test scores by group was ensured with the Shapiro test. In addition, linear mixed-effects models’ assumptions were verified by visual inspection of the normality and heteroskedasticity plot. Participant IDs were put as random effect to account for inter-participant variability. Outcome variables were analyzed as “change from pre-test”. R formula was lmer(outcome ∼ time * group+TMT-A baseline+SR-F_baseline + (1|id), control=lmerControl(optimizer = “bobyqa”), REML = T). Post-hoc analyses were carried out with estimated marginal means (emmeans package) and adjusted with Bonferroni correction. We computed contrast by group along the three time points. Any missing values were imputed with mean by group (ANTaging versus TAU) and time (pre, post, FU). ANOVA effect size (η2p) was interpreted according to small = 0.01, medium = 0.06, and large = 0.14 [51]. To estimate clinical significance we used Cohen’s d effect size interpretation in the field of gerontology [52]. To estimate the impact of ANTaging on egocentric and allocentric virtual measures (recall error) a linear mixed-effect model with Participant and Object IDs as random effects and spatial recall cues (egocentric, allocentric) and session number as fixed effects. α level was set to 0.05.
RESULTS
The average training duration regardless of the interventions was 25.93 days (SD = 11.38); no adherence difference between TAU and ANTaging groups (respectively mean of 96.9 and 98.6, p = 0.4) was found. Only two patients were classified as non-amnestic (one allocated to TAU and one to ANTaging). One patient in ANTaging interrupted the training due to VR discomfort and one in the TAU due to lack of motivation. Groups were balanced for most of the variables in Table 1, except for TMT-A; however, such a difference was not clinically significant at the group level since a PE of 0 (defective score) in the TMT-A is present when the adjusted score is greater or equal to 93 s. Concerning derivate scores (SR-F, Mfu, Mbu, Mfd, Mbd), we did not find any significant results for the MT subscores (p > 0.05) but we found a significant difference for the SR-F (p = 0.02). Patients in the ANTaging group had higher forgetting (M = 1.23, SD = 1.60) than TAU (M = 0.38, SD = 0.10). Again, such a difference was not clinically significant at the group level since a PE of 0 in the SR-F is present when the adjusted score is greater than 2.32 points. Nevertheless, we adjusted our models for TMT-A and SR-F pre-test scores. We conducted 15 separate models, including the main ten outcomes (MMSE, TMT-A, TMT-B, TMT-BA, SR-I, SR-D, CBT, CSS, DSF, MT) and five derived scores (SR-F, Mfu, Mbu, Mfd, Mbd).
Primary outcome results
Models analyzed in this section were mixed (3×2) ANOVA adjusted for TMT-A and SR-F baseline scores. Time variable had three within-subjects levels (pre-test, post-test, and FU) and group had two between-subjects levels (TAU versus ANTaging).
In the MT (total score) model, we found a significant Time by Group interaction (F2 = 3.51, p = 0.037, η2p = 0.11). Additionally, we found a significant main effect of Group (F1 = 4.29, p = 0.048, η2p=0.14). No other result was found. Post-hoc contrasts by group showed that MT scores improved in the ANTaging group from pre-test to FU (est. diff. = 0.14, SE = 0.04, p = 0.007). No other contrasts were significant in the TAU or ANTaging group.
For the CSS model, we found a main effect of Time (F2 = 3.57, p = 0.036, η2p = 0.11). Post-hoc contrasts showed a statistical tendency (improvement in CCS scores) from pre-test to FU (est. diff. = 2.38, SE = 0.99, p = 0.059) regardless of the intervention. No other contrast was found to be significant. We also found a main effect of Group (F1 = 4.64, p = 0.041, η2p = 0.15), patients in the ANTaging compared to TAU group had higher change scores from pre-test (est. diff. = 2.4, SE = 1.12). The effect of the covariate SR-F was significant (p = 0.038). Any other effects were found to be significant. See Fig. 2 for these results.
Fig. 2
Main results of the ANTaging intervention. Positive MT and CSS scores (primary outcomes) indicate improvement, whereas negative SR-F (secondary outcome) score indicates improvement. TAU, treatment as usual; MT, manikin test; CSS, Corsi supra-span test; SR-F, Story recall forgetting. *p<0.05; **p<0.01.
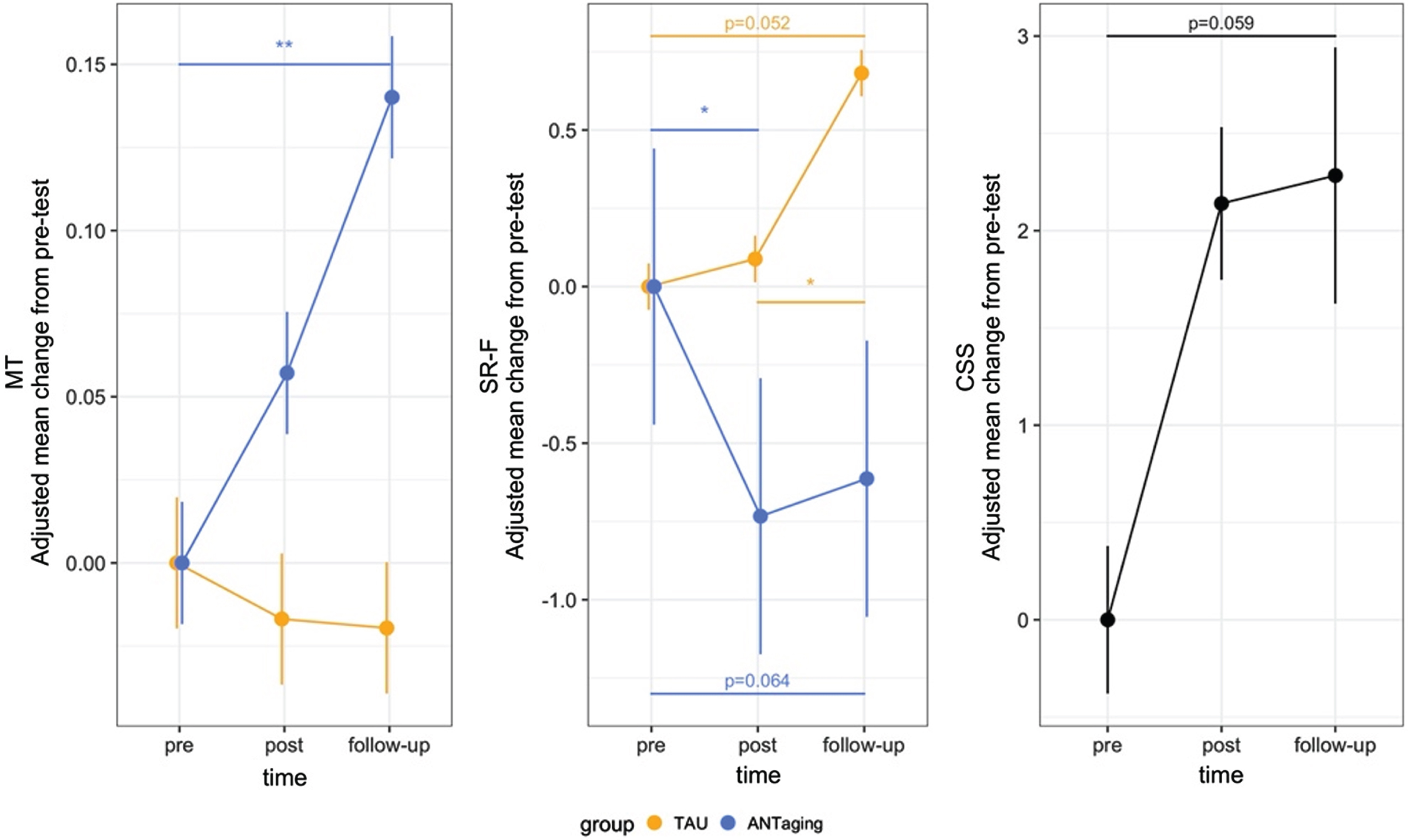
Lastly, concerning the Mbu MT derivate score, we found a significant Time by Group interaction (F2 = 4.29, p = 0.018, η2p = 0.13). However, post-hoc contrasts by group were not significant. We did not find any other significant main or interaction effects in the other models. No other significant improvement or deterioration was found for the other derivate MT scores. The Supplementary Material reports 2×2 pre-post test model results without missing imputed FU scores. aMCI sample results are reported in the Supplementary Material.
Secondary outcomes results
Models analyzed in this section were mixed (3×2) ANOVA adjusted for TMT-A and SR-F baseline scores. Time had three within-subjects levels (pre-test, post-test, and FU) and group had two between-subjects levels (TAU versus ANTaging).
For the MMSE model, we found a significant Time by Group interaction (F2 = 5.71, p = 0.006, η2p = 0.17). Any other effects were found to be significant. Post-hoc contrasts by group showed that MMSE scores improved in the TAU group from post-test to FU (est. diff. = 1.96, SE = 0.66, p = 0.014). No other contrast was significant in the TAU or ANTaging group.
For the SR-F derivate score, we found a significant Time by Group interaction (F2 = 6.82, p = 0.002, η2p = 0.2). Additionally, we found a main effect of SR-F baseline score (F1 = 21.49, p < 0.001, η2p = 0.45). Any other effects were found to be significant. Post-hoc contrasts by group revealed in the TAU group a significant deterioration from pre-test to FU (est. diff. = 0.68, SE = 0.24, p = 0.02) and a statistical tendency (deterioration) from post-test to FU (est. diff. = 0.59, SE = 0.24, p = 0.052). Conversely, in the ANTaging group, we found a significant improvement from pre-test to post-test (est. diff.=– 0.73, SE = 0.26, p = 0.019) and a statistical tendency (improvement) from pre-test to FU (est. diff. = – 0.61, SE = 0.26, p = 0.064). No other contrast was found to be significant. See Fig. 2 for this result.
No other significant improvement or deterioration was found for the other models. The Supplementary Material reports 2×2 pre-post test model results without missing imputed FU scores. aMCI sample results are reported in the Supplementary Material.
Pre-post clinical significance of the intervention
To assess the clinical significance of the ANTaging and TAU training, we separately computed paired Cohen’s d effect size on pre-post raw scores (no missing values) for ANTaging and TAU groups. Effect sizes were interpreted according to guidelines in the field of gerontology [52]. We found a large beneficial effect size (a positive d indicates that the pre-test mean is higher, a negative d indicates that the post-test mean is higher) in the ANTaging group for the CSS (d = – 0.81, 95% CI [–1.41, –0.19]) and a moderate detrimental effect size in the TAU group for the Mbu derived score (d = 0.61, 95% CI [0.07, 1.14]). In addition, we computed Cohen’s d effect size at the post-test between the ANTaging and TAU groups. We did not find any significant differences. See the Supplementary Material for each outcome effect size in the whole MCI sample and aMCI results.
Egocentric and allocentric spatial memory performance
We conducted a linear mixed-effects model with participants and objects as random factors and as fixed effects the type of landmark (egocentric versus allocentric) and the session number (continuous predictor). We included only the last five sessions since these represent pure patients’ performance without any help from the decreasing assistance procedure at recall (see ANTaging procedure above). The model had a root-mean-square error of 10.24 and a conditional R2 of 0.11. Table 2 shows the results of the linear model. We found a significant effect of the Session predictor (p < 0.001). No other effect was found to be significant (see Fig. 3). See the Supplementary Material for aMCI results.
Table 2
Virtual training linear model results
Predictors | Beta | 95% CI | p |
Session | –1.3 | –2.0, –0.65 | <0.001 |
Spatial recall cue | |||
Egocentric | –4.7 | –13, 3.6 | 0.3 |
Session by spatial recall cue | |||
Session by egocentric | 0.27 | –0.66, 1.2 | 0.6 |
CI, 95% confidence interval; reference class, allocentric cue.
Fig. 3
Intervention effect on egocentric and allocentric spatial memory. Gray shades show standard error.
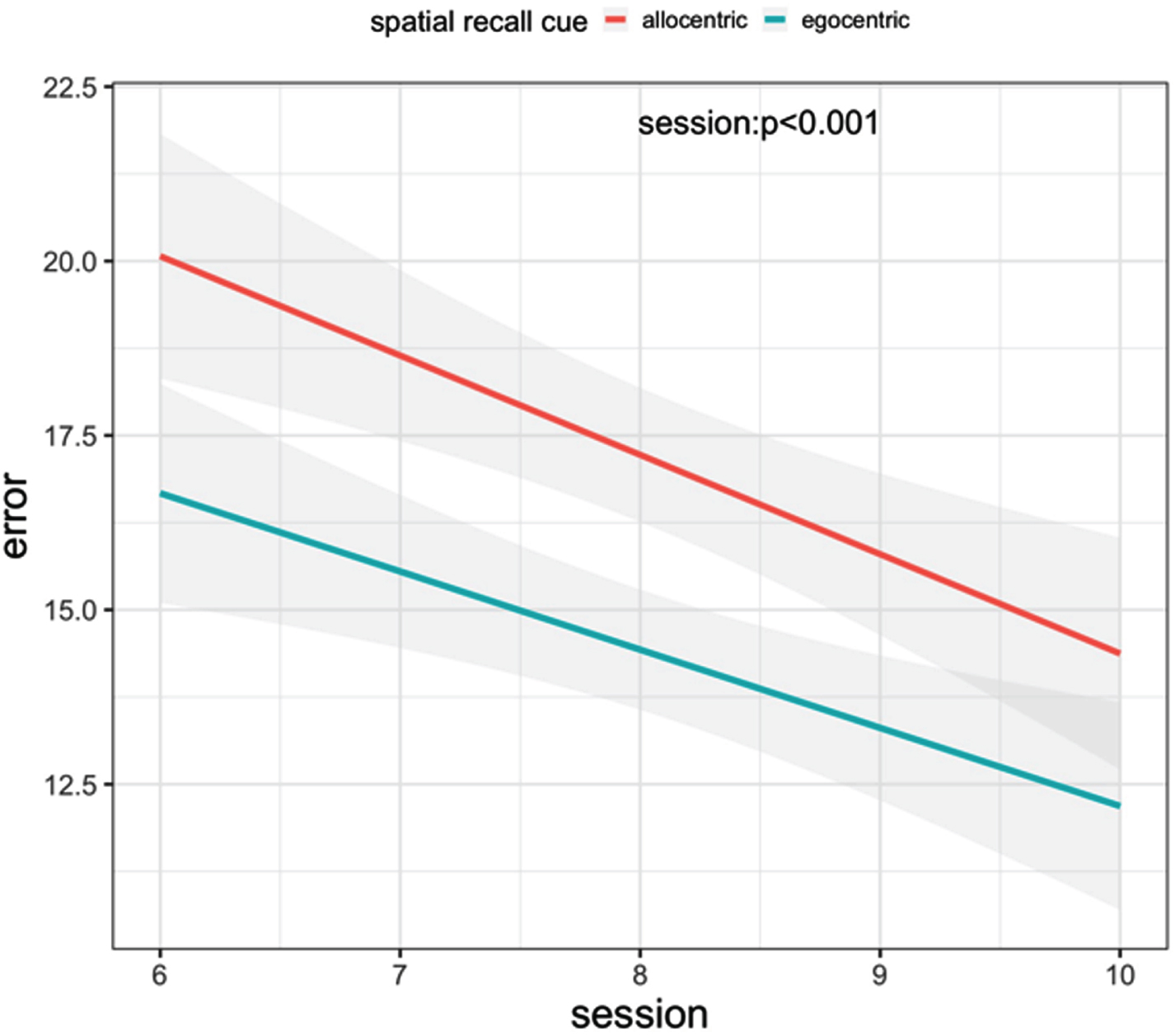
DISCUSSION
In this trial, we sought to explore the short and mid-term efficacy and feasibility of ANTaging compared to standard ‘paper and pencil’ training for spatial functions.
Concerning our primary outcomes, we found that the spatial mental rotation abilities (MT total score) improved in the ANTaging compared to the TAU group at FU, conversely, the visuospatial long-term memory (CSS) improved at FU in both groups with a statistical trend. In terms of clinically significant pre-post test results, it appears that ANTaging is effective for improving visuospatial long-term memory test (CSS); TAU had a detrimental effect on egocentric ability (Mbu). Lastly, virtual egocentric and allocentric recall accuracy was linearly associated with the session number, demonstrating that ANTaging improves spatial memory. Results remained mainly unchanged when looking at the aMCI group, which was the most prevalent phenotype in our sample. These results confirm our predictions that ANTaging is superior to the ‘paper and pencil’ method for improving spatial cognition abilities in MCI.
Our results align with a previous trial [30] in aMCI and extend it by showing that spatial navigation training, employing bodily (immersive VR) and visual-cognitive (directional and attentional) cues, can improve egocentric and allocentric spatial memory. Landmark (egocentric) and boundary (allocentric) based strategies [4] to train spatial memory can be used as a potential rehabilitative method. In addition, this training has a transfer effect on the standard cognitive tests of spatial cognition (CSS, MT subscore Mbu) in MCI. MT is considered a task of egocentric processing (Mbu, Mbd) and transformation (Mfu and Mfd), where participants had to mentally change their egocentric coordinates to a new set of egocentric ones to answer correctly [45]. It has been proposed that the process of changing the egocentric perspective to a new set of egocentric coordinates is supported by additional allocentric processing [7]. Therefore, the improvement in virtual memory performance might have transferred to different spatial tasks supported by the same underlying mechanisms. Concerning the visuospatial long-term memory (CSS) we only reported a clinically significant result for ANTaging and comparable statistically significant results for both interventions. This is in line with a study [14] on aMCI and AD patients that found that MT but not CSS was related to an egocentric and allocentric task. In a previous trial on patients who suffer from possible AD [29], it was shown that non-immersive virtual spatial training in a city with the aid of an interactive allocentric map improved CSS scores after the interventions in AD but not in healthy older adults. We did not observe the same result, possibly because we did not employ the interactive map.
Concerning our secondary outcomes, we found an improvement at the FU from the post-test in global cognition (MMSE) for the TAU group, whereas in the ANTaging the score was stable. Then, we found that auditory-verbal forgetting (SR-F) declined from post-test to FU in the TAU group but improved from pre-test to post-test in the ANTaging group and was maintained at FU. All the other test scores neither statistically improved nor worsened at the post-test nor FU. This set of results is partially in line with our predictions since we expected stable performance in non-spatial-related cognitive functions.
Concerning this, we showed that the ANTaging method can improve forgetting compared to TAU. This could be possible since allocentric tasks [4, 30] could have a positive effect on hippocampal functioning [53]. Conversely, the improvement we reported in the TAU for global cognition could be due to the nature of the training, since ANTaging was developed to focus on spatial abilities, whereas the ‘paper and pencil’ might have included also other functions, such as spatial verbal recall or more general cognitive functions (see, standard ‘paper and pencil’ training procedure).
Lastly, our training based on embodied cognition principles supports the notion that cognitive representation of the space could be modality-dependent (body-based, symbolic, visual) and supported by aids that can act as affordances [18, 19]. Such a view is in contrast to the so-called modality-independent representation of the space, which affirms that cognitive maps are transformed into an amodal format [54]. Indeed, we showed that immersive VR supports bodily information at encoding and that such body-based information can be used to enhance recall. This confirms previous studies that found that the body can be used to improve spatial memory in MCI [23, 25, 26]. Concerning visual-cognitive, our trial demonstrated that directional cues and attentional markers are useful to support navigation and subsequent spatial recall in MCI. This is in line with previous assessment studies that found a beneficial effect of these aids in this population [20, 28].
Despite the promising results, we need to acknowledge some limitations. First, due to pandemic restrictions and patients’ motivation, we had some missing values at FU. However, the results are maintained when analyzing pre-post results. Second, to properly test if embodied bodily cues are relevant to spatial training, a passive navigation control (no interaction during encoding) condition should have been implemented; nevertheless, the primary aim of this pilot trial was to assess the effectiveness and feasibility of ANTaging VR. Therefore, we initially set the objective of comparing it with the TAU. Moreover, it could be useful to add a pre-post measure on subjective navigation impairment in daily life and asses any transfer to real-world ability. Future research could try to overcome such limitations and advance research for spatial memory and navigation impairments in MCI. Lastly, results should be taken with caution as this trial used a non-randomized allocation method; a randomized controlled trial is mandatory for stronger evidence in favor of ANTaging.
To conclude, virtual spatial navigation training based on embodied principles could be a promising way to enhance cognition and in particular spatial navigation in aging and dementia and provide insights into the structure of (possibly embodied) cognitive representations.
AUTHOR CONTRIBUTIONS
Cosimo Tuena (Conceptualization; Data curation; Formal analysis; Funding acquisition; Investigation; Methodology; Project administration; Writing – original draft; Writing – review & editing); Silvia Serino (Conceptualization; Writing – original draft; Writing – review & editing); Elisa Pedroli (Investigation); Chiara Stramba-Badiale (Investigation; Writing – review & editing); Karine Marie Goulene (Investigation); Marco Stramba-Badiale (Supervision); Giuseppe Riva (Supervision).
ACKNOWLEDGMENTS
The authors wish to thank the patients involved in this study and Sara Maestri and Giulia Magni for their support during experimental sessions.
FUNDING
The publication fee has been supported by Ricerca Corrente from Italian Ministry of Health. The project has been supported by the Grant (Starting Grant Ricerca Finalizzata, code: SG-2018-12368175).
CONFLICT OF INTEREST
The authors have no conflict of interest to report.
DATA AVAILABILITY
Data are available upon reasonable request at 10.5281/zenodo.10201061.
SUPPLEMENTARY MATERIAL
[1] The supplementary material is available in the electronic version of this article: https://dx.doi.org/10.3233/JAD-240200.
REFERENCES
[1] | Lester AW , Moffat SD , Wiener JM , Barnes CA , Wolbers T ((2017) ) The aging navigational system. Neuron 95: , 1019–1035. |
[2] | Barry C , Burgess N ((2014) ) Neural mechanisms of self-location. Curr Biol 24: , R330–R339. |
[3] | Harootonian SK , Ekstrom AD , Wilson RC ((2022) ) Combination and competition between path integration and landmark navigation in the estimation of heading direction. PLoS Comput Biol 18: , e1009222. |
[4] | Chersi F , Burgess N ((2015) ) The cognitive architecture of spatial navigation: Hippocampal and striatal contributions. Neuron 88: , 64–77. |
[5] | Doeller CF , King JA , Burgess N ((2008) ) Parallel striatal and hippocampal systems for landmarks and boundaries in spatial memory. Proc Natl Acad Sci U S A 105: , 5915–5920. |
[6] | Guderian S , Dzieciol XAM , Gadian DG , Jentschke XS , Doeller CF , Burgess N , Mishkin XM , Vargha-Khadem F ((2015) ) Hippocampal volume reduction in humans predicts impaired allocentric spatial memory in virtual-reality navigation. J Neurosci 35: , 14123–14131. |
[7] | Vogeley K , Fink GR ((2003) ) Neural correlates of the first-person-perspective. Trends Cogn Sci 7: , 38–42. |
[8] | Byrne P , Becker S , Burgess N ((2009) ) Remembering the past and imagining the future: A neural model of spatial memory and imagery. Psychol Rev 114: , 340–375. |
[9] | Petersen RC ((2011) ) Mild cognitive impairment. N Engl J Med 364: , 2227–2234. |
[10] | Cerman J , Andel R , Laczo J , Vyhnalek M , Nedelska Z , Mokrisova I , Sheardova K , Hort J ((2018) ) Subjective spatial navigation complaints – a frequent symptom reported by patients with subjective cognitive decline, mild cognitive impairment and Alzheimer’s disease. Curr Alzheimer Res 15: , 219–228. |
[11] | Tuena C , Mancuso V , Stramba-Badiale C , Pedroli E , Stramba-Badiale M , Riva G , Repetto C ((2021) ) Egocentric and allocentric spatial memory in mild cognitive impairment with real-world and virtual navigation tasks: A systematic review. J Alzheimers Dis 79: , 95–116. |
[12] | Laczó J , Andel R , Vlček K , Macoška V , Vyhnálek M , Tolar M , Bojar M , Hort J ((2011) ) Spatial navigation and APOE in amnestic mild cognitive impairment. Neurodegener Dis 8: , 169–177. |
[13] | Boccia M , Silveri MC , Sabatini U , Guariglia C , Nemmi F ((2016) ) Neural underpinnings of the decline of topographical memory in mild cognitive impairment. Am J Alzheimers Dis Other Demen 31: , 618–630. |
[14] | Serino S , Morganti F , Di Stefano F , Riva G ((2015) ) Detecting early egocentric and allocentric impairments deficits in Alzheimer’s disease: An experimental study with virtual reality. Front Aging Neurosci 7: , 88. |
[15] | Lowry E , Puthusseryppady V , Coughlan G , Jeffs S , Hornberger M ((2020) ) Path integration changes as a cognitive marker for vascular cognitive impairment?— A pilot study. Front Hum Neurosci 14: , 131. |
[16] | Schneider CB , Linse K , Schönfeld R , Brown S , Koch R , Reichmann H , Leplow B , Storch A ((2017) ) Spatial learning deficits in Parkinson’s disease with and without mild cognitive impairment. Parkinsonism Relat Disord 36: , 83–88. |
[17] | Montana JI , Tuena C , Serino S , Cipresso P , Riva G ((2019) ) Neurorehabilitation of spatial memory using virtual environments: A systematic review. J Clin Med 8: , 1516. |
[18] | Steel A , Robertson CE , Taube JS ((2021) ) Current promises and limitations of combined virtual reality and functional magnetic resonance imaging research in humans: A commentary on Huffman and Ekstrom (2019). J Cogn Neurosci 33: , 159–166. |
[19] | Tuena C , Serino S , Pedroli E , Stramba-Badiale M , Riva G , Repetto C ((2021) ) Building embodied spaces for spatial memory neurorehabilitation with virtual reality in normal and pathological aging. Brain Sci 11: , 1067. |
[20] | Cogné M , Auriacombe S , Vasa L , Tison F , Klinger E , Sauzéon H , Joseph P , N’Kaoua B ((2018) ) Are visual cues helpful for virtual spatial navigation and spatial memory in patients with mild cognitive impairment or Alzheimer’s disease? Neuropsychology 32: , 385–400. |
[21] | König SU , Goeke C , Meilinger T , König P ((2019) ) Are allocentric spatial reference frames compatible with theories of Enactivism? Psychol Res 83: , 498–513. |
[22] | Morganti F ((2018) ) Enacting space in virtual reality: A comparison between Money’s Road Map test and its virtual version. Front Psychol 9: , 2410. |
[23] | Tuena C , Serino S , Dutriaux L , Riva G , Piolino P ((2019) ) Virtual enactment effect on memory in young and aged populations: A systematic review. J Clin Med 8: , 620. |
[24] | Engelkamp J ((1998) ) Memory for Actions, Psychology Press/Taylor & Francis (UK) . |
[25] | Plancher G , Tirard A , Gyselinck V , Nicolas S , Piolino P ((2012) ) Using virtual reality to characterize episodic memory profiles in amnestic mild cognitive impairment and Alzheimer’s disease: Influence of active and passive encoding. Neuropsychologia 50: , 592–602. |
[26] | Tuena C , Riva G , Murru I , Campana L , Goulene KM , Pedroli E , Stramba-Badiale M ((2022) ) Contribution of cognitive and bodily navigation cues to egocentric and allocentric spatial memory in hallucinations due to Parkinson’s disease: A case report. Front Behav Neurosci 16: , 992498. |
[27] | Huffman DJ , Ekstrom AD ((2021) ) An important step toward understanding the role of body-based cues on human spatial memory for large-scale environments. J Cogn Neurosci 33: , 167–179. |
[28] | Davis R , Ohman JM , Weisbeck C ((2017) ) Salient cues and wayfinding in Alzheimer’s disease within a virtual senior residence. Environ Behav 49: , 1038–1065. |
[29] | Serino S , Pedroli E , Tuena C , De Leo G , Stramba-Badiale M , Goulene K , Mariotti NG , Riva G ((2017) ) A novel virtual reality-based training protocol for the enhancement of the “mental frame syncing” in individuals with Alzheimer’s disease: A development-of-concept trial. Front Aging Neurosci 9: , 240. |
[30] | Park JH ((2020) ) Effects of virtual reality-based spatial cognitive training on hippocampal function of older adults with mild cognitive impairment. Int Psychogeriatr 3: , 157–163. |
[31] | Tuena C , Serino S , Pedroli E , Cipresso P , Stramba-Badiale M , Riva G , Repetto C ((2022) ) ANTaging: A research protocol for active navigation training with virtual reality in mild cognitive impairment. Annu Rev CyberTherapy Telemed 20: , 115–119. |
[32] | Tuena C , Serino S , Maestri S , Pedroli E , Stramba-Badiale C , Brizzi G , Goulene K , Cipresso P , Stramba-Badiale M , Riva G ((2023) ) Usability of an embodied CAVE system for spatial navigation rehabilitation in mild cognitive impairment. J Clin Med 12: , 1949. |
[33] | Albert MS , Dekosky ST , Dickson D , Dubois B , Feldman HH , Fox NC , Gamst A , Holtzman DM , Jagust WJ , Petersen RC , Snyder PJ , Carrillo MC , Thies B , Phelps CH ((2011) ) The diagnosis of mild cognitive impairment due to Alzheimer’s disease: Recommendations from the National Institute on Aging-Alzheimer’s Association workgroups on diagnostic guidelines for Alzheimer’s disease. Alzheimers Dement 7: , 270–279. |
[34] | Capitani E , Laiacona M ((1988) ) Aging and psychometric diagnosis of intellective impairment: Some considerations on test scores and their use. Dev Neuropsychol 4: , 325–330. |
[35] | Magni E , Binetti G , Bianchetti A , Rozzini R , Trabucchi M ((1996) ) Mini-mental state examination: A normative study in Italian elderly population. Eur J Neurol 3: , 198–202. |
[36] | Winblad B , Palmer K , Kivipelto M , Jelic V , Fratiglioni L , Wahlund LO , Nordberg A , Bäckman L , Albert M , Almkvist O , Arai H , Basun H , Blennow K , De Leon M , Decarli C , Erkinjuntti T , Giacobini E , Graff C , Hardy J , Jack C , Jorm A , Ritchie K , Van Duijn C , Visser P , Petersen RC ((2004) ) Mild cognitive impairment – Beyond controversies, towards a consensus: Report of the International Working Group on Mild Cognitive Impairment. J Intern Med 256: , 240–246. |
[37] | Monaco M , Costa A , Caltagirone C , Carlesimo GA ((2012) ) Forward and backward span for verbal and visuo-spatial data: Standardization and normative data from an Italian adult population. Neurol Sci 34: , 749–754. |
[38] | Spinnler H , Tognoni G ((1987) ) Standardizzazione e taratura italiana di test neuropsicologici. Ital J Neurol Sci 8: , 1–120. |
[39] | Carlesimo GA , Buccione I , Fadda L , Graceffa A , Mauri M , Lorusso S , Bevilacqua G , Caltagirone C ((2002) ) Stan-dardizzazione di due test di memoria per uso clinico. Breve racconto e figura di Rey. Nuova Riv di Neurol 12: , 1–13. |
[40] | Mondini S , Mapelli D , Vestri A , Bisiacchi PS ((2003) ) Esame neuropsicologico breve. Una batteria di test per lo screening neuropsicologico.. |
[41] | Ratcliff G ((1979) ) Spatial thought, mental rotation and the right cerebral hemisphere. Neuropsychologia 17: , 49–54. |
[42] | Laudisio A , Antonelli Incalzi R , Gemma A , Marzetti E , Pozzi G , Padua L , Bernabei R , Zuccalà G ((2018) ) Definition of a Geriatric Depression Scale cutoff based upon quality of life: A population-based study, Int J Geriatr Psychiatry 33: , e58–e64. |
[43] | Katz S ((1983) ) Assessing self-maintenance: Activities of daily living, mobility, and instrumental activities of daily living. J Am Geriatr Soc 31: , 532–5415. |
[44] | Lawton MP , Brody EM ((1969) ) Assessment of older people: Self-maintaining and instrumental activities of daily living. Gerontologist 9: , 179–186. |
[45] | Kaltner S , Riecke BE , Jansen P ((2014) ) Embodied mental rotation: A special link between egocentric transformation and the bodily self. Front Psychol 5: , 505. |
[46] | Sedgwick PM ((2017) ) Nonrandomized trials: Designs and methodology. In Wiley StatsRef: Statistics Reference Online, pp. 1–7. |
[47] | Tuena C , Riva G ((2022) ) Active navigation training: An innovative embodied-based training system for spatial navigation in aging. Cyberpsychol Behav Soc Netw 25: , 77–78. |
[48] | Riley GA , Heaton S ((2000) ) Guidelines for the selection of a method of fading cues. Neuropsychol Rehabil 10: , 133–149. |
[49] | Pedroli E , Mancuso V , Stramba-Badiale C , Cipresso P , Tuena C , Greci L , Goulene K , Stramba-Badiale M , Riva G , Gaggioli A ((2022) ) Brain M-App’s structure and usability: A new application for cognitive rehabilitation at home. Front Hum Neurosci 16: , 898633. |
[50] | Luke SG ((2017) ) Evaluating significance in linear mixed-effects models in R. Behav Res 49: , 1494–1502. |
[51] | Richardson JTE ((2011) ) Eta squared and partial eta squared as measures of effect size in educational research. Educ Res Rev 6: , 135–147. |
[52] | Brydges CR ((2019) ) Effect size guidelines, sample size calculations, and statistical power in gerontology. Innov Aging 3: , igz036. |
[53] | Tromp D , Dufour A , Lithfous S , Pebayle T , Després O ((2015) ) Episodic memory in normal aging and Alzheimer disease: Insights from imaging and behavioral studies. Ageing Res Rev 24: , 232–262. |
[54] | Huffman DJ , Ekstrom AD ((2019) ) A modality-independent network underlies the retrieval of large-scale spatial environments in the human brain. Neuron 104: , 611–622.e7. |