Bodily and Visual-Cognitive Navigation Aids to Enhance Spatial Memory Recall in Mild Cognitive Impairment
Abstract
Background:
Individuals with mild cognitive impairment (MCI) syndrome often report navigation difficulties, accompanied by impairments in egocentric and allocentric spatial memory. However, studies have shown that both bodily (e.g., motor commands, proprioception, vestibular information) and visual-cognitive (e.g., maps, directional arrows, attentional markers) cues can support spatial memory in MCI.
Objective:
We aimed to assess navigation cues for innovative spatial training in aging.
Methods:
Fifteen MCI patients were recruited for this study. Their egocentric and allocentric memory recall performances were tested through a navigation task with five different virtual reality (VR) assistive encoding navigation procedures (bodily, vision only, interactive allocentric map, reduced executive load, free navigation without cues). Bodily condition consisted of an immersive VR setup to engage self-motion cues, vision only condition consisted of passive navigation without interaction, in the interactive allocentric map condition patients could use a bird-view map, in the reduced executive load condition directional cues and attentional markers were employed, and during free navigation no aid was implemented.
Results:
Bodily condition improved spatial memory compared to vision only and free navigation without cues. In addition, the interactive allocentric map was superior to the free navigation without cues. Surprisingly, the reduced executive load was comparable to vison only condition. Moreover, a detrimental impact of free navigation was observed on allocentric memory across testing trials.
Conclusions:
These findings challenge the notion of an amodal representation of space in aging, suggesting that spatial maps can be influenced by the modality in which the environment was originally encoded.
INTRODUCTION
The design of effective non-pharmacological interventions that harness the potential of cutting-edge technologies to halt cognitive decline in aging has received much attention in recent decades. In particular, understanding how to improve technological-based training of spatial navigation with valuable assistive aids has great theoretical and practical relevance for the prevention and treatment of dementia [1–3]. This may have unique value, particularly in the aging population with mild cognitive impairment (MCI) syndrome, which denotes the stage between aging normally and dementia [4]. Indeed, to date no effective drug has been approved for treating cognitive deficits in MCI and non-pharmacological interventions should be preferred to slow down cognitive decline [4]. Amnestic MCI (aMCI), which affects the memory domain, is typically associated with an increased risk of Alzheimer’s disease (AD); in contrast, non-amnestic MCI, which affects other cognitive domains than memory, could lead to frontotemporal, vascular, or Lewy body dementias [5].
It is recognized that impairments in spatial memory and navigation could be considered a crucial behavioral marker for AD diagnosis (see for example, [6, 7]). However, recent studies showed that such deficits can be observed also in other types of neurodegenerative diseases linked to cognitive decline [8–11]. Importantly, subjective navigation complaints have been reported in individuals with amnestic and non-amnestic MCI phenotype [12].
Navigation is supported by successful encoding, storage, and retrieval of one’s position and path using environmental (e.g., landmarks and boundaries mainly sustained by vision) and self-motion (bodily from now on) cues (i.e., motor commands, proprioception, vestibular information) [13, 14]. This spatial information can be organized using two alternative spatial frames of reference: the egocentric (body-centered) and the allocentric (world-centered) representation [15].
A recent systematic review [16] found that the ability to use egocentric and allocentric spatial frames of reference for an effective spatial memory and navigation is affected in MCI population. Indeed, individuals suffering from aMCI with genetic risk for AD (positive APOE ɛ4 allele) compared to those without a genetic risk (i.e., aMCI ɛ4 negative group) manifested an impairment in the egocentric and allocentric spatial navigation [17]. Moreover, individuals with aMCI, regardless of their genetic risk profile, demonstrated a poorer spatial performance when compared to the control group. Similar patterns have been demonstrated in aMCI individuals with hippocampal and frontal deficit [18]. In another study, individuals with aMCI showed difficulties in the storage of new topographical memories from an allocentric perspective and retrieval of this stored information while performing a spatial egocentric task [19]. Another study showed that aMCI patients with memory impairment alone had deficits in allocentric spatial memory, whereas aMCI patients with deficits in memory and other cognitive domains had both egocentric and allocentric impairments; individuals with non-amnestic MCI showed like-control group performance [20]. Egocentric and allocentric impairments have also been reported in the non-AD-related MCI population. Recently, it has been shown that patients with cognitive impairment due to cerebrovascular lesions showed a deficit in egocentric spatial memory [10]. In another study, it emerged that patients who suffer from MCI due to Parkinson’s disease have impaired allocentric and egocentric spatial learning compared to healthy participants and similar abilities to individuals with Parkinson’s disease without MCI [21]. Overall, this set of results suggests that the MCI population, despite its heterogeneity, has both subjective navigation complaints and objective deficits in the underlying spatial cognition mechanisms; thus suggesting that spatial-navigation-based training might alleviate such impairments [16].
Of all the technologies available today, virtual reality (VR) appears to be an up-and-coming tool for developing spatial navigation training for older people, exploiting the possibility of using procedures and assistive aids to navigation [22–24]. Cues that can support spatial memory for older adults can be classified into two categories: bodily cues [22] and visual-cognitive [23].
Regarding bodily cues, Plancher and colleagues [25] administered a virtual navigation task to groups consisting of cognitively healthy older adults, individuals with aMCI, and those with AD. Participants were required to drive (driving simulator with pedals and steering wheel) in a virtual city or to passively watch (i.e., passive navigation supported by visual information only) a pre-recorded navigation. Then, participants were asked to recall events and their spatiotemporal information that occurred along the city path. The authors found that motor interaction (namely, driving condition) compared to relying solely on vision improved allocentric memory when assessed with a free recall method in healthy older adults, aMCI, and AD groups. This effect was not found for the egocentric memory. More recently, patients with Parkinson’s disease with and without psychosis were asked to encode item locations in a virtual arena and then recall these spatial positions using egocentric and allocentric cues in two different conditions: one involving bodily cues (i.e., immersive VR) and one relying only on visual inputs (i.e., passive navigation) [26]. The authors found that when bodily cues were incorporated during both encoding and recall stages, it can reduce the deficit in egocentric memory observed in individuals with MCI due to Parkinson’s disease with psychosis. In contrast, relying only on vision at encoding impaired egocentric rather than allocentric virtual recall. VR allows researchers to gradually modulate the bodily information recruited during navigation; some authors, indeed, suggest that the link between this information and spatial memory enhancement follows a linear association [1, 22]. Despite this evidence, no impact of bodily cues has been found in young adults, suggesting that this information does not affect the cognitive map of the environment (e.g., [27]).
Concerning visual-cognitive aids, Cognè and co-authors [23] tested the usefulness of providing such cues compared to a free navigation condition without cues in three groups: individuals with MCI, patients with AD, and healthy controls. Participants were asked to passively watch a non-immersive VR navigation in a city (namely, the learning phase) and then reproduce the path with the joystick. Subsequently, egocentric (landmark memory and landmark ordering) and allocentric (path outline) representations were tested. The authors assessed the usefulness of enhancing spatial memory by incorporating directional arrows, allocentric map, and salient (vivid color) landmarks during both the learning and recall phases. They found that directional cues and the presence of salient landmarks to sustain spatial attention improved spatial memory (particularly the egocentric ones) in patients who suffer from AD or MCI. They found no effect of the allocentric map on performance, possibly because of the map’s characteristics-small and non-interactive. Davis and colleagues [28] administered a non-immersive virtual task to cognitively impaired (AD and MCI) and healthy older adults. Participants were asked to interact with VR with a joystick to find a target location with and without salient cues to allocate spatial attention during the path and were tested on two consecutive days. Both groups of participants groups benefited from the salient cues condition, which had a beneficial effect on both learning and retrieval trials.
Virtual visual-cognitive cues provide powerful information to assist patients during navigation and memory tasks [29]; moreover, these types of aids could be thought of as spatial affordances (symbolic or environmental). The first type can be immediately used as a compensatory aid (e.g., a map, a directional arrow) and the second is processed by the individual according to the subjective salience of the environmental landmark. In this way, patients can reduce effort by off-loading cognitive processes with immediate action [22].
Lastly, another aspect to consider is the interplay between executive functions and navigation on spatial memory. Cognè and co-authors [23] showed that dysexecutive deficits hamper the use of directional cues during the previously mentioned virtual navigation task. Consistently with this aspect, Jebara and colleagues [30] administered a task similar to that described previously [25] and found that in healthy older adults the possibility to choose the itinerary during passive virtual navigation led to better spatial item location compared to motor interaction (i.e., driving simulator).
Research is still preliminary in MCI population, but it seems that bodily, directional, and attentional cues may enhance spatial memory. In particular, it could be possible that the greater the bodily involvement the greater the improvement on spatial memory (immersive VR > desktop VR > vision only condition). The inclusion of directional and attentional aids seems to have a positive impact regardless of the bodily involvement of the patient; conversely, if no cognitive cue is provided passive navigation combined with itinerary-decision making should be preferred. The presence of the allocentric map could positively affect memory but this might depend on its characteristics (e.g., degree of interaction, visual details). Nevertheless, the impact of visual-cognitive aids on navigation in comparison to a vision only condition or when these are compared to an immersive virtual navigation manipulation is not known. Lastly, it is still unclear how bodily and visual-cognitive aids impact egocentric and allocentric processing [1, 22, 31].
Therefore, the objective of this study is to investigate the effectiveness of various types of navigational aids at encoding on spatial memory recall performance. We aimed to test the impact of bodily (bodily versus vision only) and visual-cognitive cues (interactive allocentric map versus free navigation without cues versus reduced executive load conditions) used during the encoding on subsequent egocentric and allocentric spatial recall in MCI syndrome.
Based on the previous literature we expect that bodily condition will have a beneficial effect when compared to the vision only condition, as well as compared to free navigation without cues and with desktop VR. Then, we expect that the conditions involving the interactive allocentric map and reduced executive load conditions will lead to enhanced spatial memory compared to the condition of free navigation without cues. In addition, we want to explore whether these five conditions affect allocentric and egocentric memory recall.
METHODS AND MATERIALS
Participants
Fifteen participants (mean age = 75.5, SD = 6.2; mean years of education = 10.7, SD = 4.2; females = 6) diagnosed with the MCI core clinical criteria were recruited for this study. According to the patient history, neurological referral, and clinical neuropsychological assessment, MCI diagnosis was determined by a clinical neuropsychologist and the treating physician (EP, KMG) reports. The core clinical criteria of Albert and colleagues [32] were used to diagnose MCI syndrome: (1) concern about a change in cognition expressed by the patient, an informant, or a clinician; (2) objective cognitive impairment in one or more domains; (3) maintenance of independence in functional abilities; and (4) absence of dementia diagnosis. Age above or equal to 60 years old and the absence of abnormal global cognition as determined by the Italian Mini-Mental State Examination (MMSE) cut-off (adjusted for age and education) [33] were additional inclusion criteria.
The following conditions were required for exclusion: (i) the presence of an acute stroke or transient ischemic attack; (ii) the presence of other comorbid severe neurological (e.g., multiple sclerosis, brain tumor, Huntington’s disease) and psychiatric disorders (e.g., schizophrenia) or non-treated psychiatric conditions (e.g., mood and anxiety disorders); (iii) the history of traumatic brain injury with loss of consciousness; (iv) physical or functional impairments (e.g., musculoskeletal disorders, limb paresis or paraesthesia) that could impair the use of VR and experimental procedure; (v) a severe visual impairment; and (vi) the presence of recurrent vertigo.
To compute the sample size, we used the effect size reported by Plancher and colleagues [25]. The sample size required for a 5 (bodily versus vision only interactive allocentric map versus free navigation without cues versus reduced executive load conditions)×2 (egocentric versus allocentric) full-within interaction (numerator degree of freedom = 4), using an effect size f of 0.3, an alpha of 0.05, and a power of 0.8, is 15 participants.
Participants were recruited from 1 April 2020 to 1 April 2023 at the Outpatient Clinic of the Department of Geriatrics and Cardiovascular Medicine, IRCCS Istituto Auxologico Italiano—Mosè Bianchi, Milan. The study was conducted in accordance with the Declaration of Helsinki and approved by the Ethics Committee of Istituto Auxologico Italiano (code: 2019_05_21_04). Written informed consent was obtained from the participants before they participated in the study. Table 1 shows the demographics and clinical variables of the included sample.
Table 1
Demographics and clinical variables of the group
Variable | N = 15 |
Age | 75.5 (6.2) |
Education | 10.7 (4.2) |
Gender | |
F | 6 (40%) |
M | 9 (60%) |
MCI phenotype | |
aMCI | 10 (67%) |
naMCI | 5 (33%) |
MMSE | 26.69 (1.45) |
FAB | 14.28 (2.16) |
TMT-A | 45.53 (15.90) |
TMT-B | 138.87 (121.48) |
FCSRT-I | 22.91 (5.24) |
FCSRT-D | 7.96 (3.60) |
RCPM | 25.87 (5.69) |
CBT | 4.57 (0.39) |
CSS | 10.1 (4.9) |
GDS | 5.14 (2.60) |
ADL | 5.67 (0.49) |
IADL | 5.47 (2.10) |
MCI, mild cognitive impairment; aMCI, amnestic MCI; naMCI, non-amnestic MCI; MMSE, Mini-Mental State Examination; FAB, Frontal Assessment Battery; TMT-AB, Trail Making Test part A and B; FCSRT-I, Free and Cued Selective Reminding Test – immediate; FCSRT-D, Free and Cued Selective Reminding Test – delayed; RCPM, Raven’s Colored Progressive Matrices; CBT, Corsi Block Tapping test; CSS, Corsi Supra-Span test; GDS, Geriatric Depression Scale short-form; ADL, Activities of Daily Living; IADL, Instrumental Activities of Daily Living.
Virtual navigation task
We used a modified version of a landmark and boundary-based spatial memory recall navigation task [34]. Five different assistive procedures were administered to the patients. All five interfaces had the same underlying encoding-recall structure similar to the original one.
Participants were required to collect four items at a time and memorize their locations in a circular arena during the encoding phase (the diameter of the arena was 50 virtual meters). Object locations could be learned using the boundaries of the arena (i.e., wall), an intra-arena landmark (i.e., obelisk), and distal cues (i.e., mountain range, clouds). Items were randomly presented, and each object was collected four times in random order. To see the object, the participants had to go to the exact location of the item. Once over it, the object disappeared, and the patient had to find the next one. During the immediate recall phase, participants had to recall and go to the exact location where the item (shown at the bottom of the screen) was previously collected and press a button to respond. Then the following object was shown. In random order, either the wall or the obelisk was removed. This forced the use of an allocentric (i.e., wall) or an egocentric (i.e., obelisk) spatial memory recall [35]. Each object was tested two times with the egocentric and allocentric spatial frame for a total of 16 trials (eight trials for each allocentric and egocentric recall condition). The response variable was the distance error for each object trial at recall (Euclidean distance in virtual meters of the recalled position from the actual location). An invisible wall was used as a constraint during the obelisk condition so that both recalls were comparable.
To create the bodily and visual-cognitive cues at encoding we manipulated the degree of immersion (2D desktop VR versus fully immersive VR), the degree of interaction at encoding versus recall (active versus passive), and encoding visual-cognitive aids provided during encoding (interactive map, directional cues, and attentional markers on environmental cues). We named the five virtual interfaces we designed as follows: bodily, vision only, interactive allocentric map, reduced executive load, and free navigation without cues. Table 2 shows a summary of the conditions.
Table 2
Summary of the assistive encoding navigation cues
Bodily conditions | Cognitive conditions | ||||
Bodily | Vision only | Interactive allocentric map | Reduced executive load | Free navigation without cues | |
Bodily involvement (virtual set up) | Oculus Rift S + 3dRudder | PC + mouse and keyboard | PC + mouse and keyboard | PC + mouse and keyboard | PC + mouse and keyboard |
Encoding interaction | Active | Passive | Active | Active | Active |
Encoding visual-cognitive aids | Directional cues | No cues | Interactive map + directional cues | Attentional markers + directional cues | No cues |
Recall interaction | Active | Active | Active | Active | Active |
Recall visual-cognitive aids | No cues | No cues | No cues | No cues | No cues |
Participants in the “bodily” condition were immersed in the environment with a 3D visor (Oculus Rift S) and could move with the 3dRudder, a foot-motion pad. In addition, in the encoding phase, they used directional cues to encode the spatial location of the target (namely, they follow a line to reach the item) but no interactive map (see below for description) or attentional cues (see below for description).
This is to separate the bodily component (i.e., motor commands, proprioception, and vestibular system) from other processes that might support spatial encoding processes (i.e., route decision-making inhibition, spatial manipulation, and spatial attention). In addition, the use of directional cues was designed to make this encoding condition more comparable to vision only by reducing the degrees of freedom involved during free exploration.
In the recall phase, the same VR apparatus is used, but without the possibility of using directional cues, maps, or attention cues. Patients could respond using the Oculus Rift controller once they were satisfied with the location of the object.
Participants in the “vision only” condition simply observed the experimenter’s navigation on a 2D-VR setup (15-inch PC screen) without visual-cognitive cues (directional lines, maps, and attention cues) and interaction during encoding. This is to isolate the visual system during encoding as if the participant was seated in a car like a passenger [36]. At recall, they used a 2D-VR apparatus (15-inch PC screen, keyboard keys, and mouse) to find the item locations and used the space bar to respond.
To reduce the involvement of bodily information in immersive VR (motor commands but especially proprioception and vestibular information; [37]), in the cognitive session, 2D-VR (15 inch PC screen, keyboard keys, and mouse) was used during the encoding and recall phases.
The participant in the “interactive allocentric map” condition explored the environment with the aid of an interactive bird-view map and directional cues but without attentional cues. The map rotates depending on the direction, provides cardinal points, landmarks, and item positions, and shows current and target locations and the directional line. The use of direction cues helped the patient to focus on the information provided by the interactive map rather than route decision-making. During the recall phase, all the cues were not provided, and the patients used the 2D-VR set-up to find the items’ locations.
Before the learning phase in the “reduced executive load” condition, the participant was at the center of the arena with the obelisk, wall, and distal cues, and was asked to look around with the mouse, discover six orange markers, and loudly say the number above each marker. Markers were placed one on the obelisk, one on the mountain range, and four on the top of the wall (circularly equidistant). This circumstance necessitated the employment of attentional resources to the spatial layout and environment before the navigation task. The experimenter made all the markers vanish once they were correctly found. Then the encoding phase started, and the patient was given directional cues (to reduce cognitive load during item search) but no allocentric map. Attentional, map, and directional cues were not presented during recall and the patients used the 2D-VR set to find the item locations and respond.
Finally, all cues were removed in the “free navigation without cues” interface at encoding. In this condition, the participant was free to choose where to go without any visual-cognitive aid. This condition required older participants to make greater use of executive/route decision-making resources during the encoding phase [30]. No cues were given during the recall to participants, who used the 2D-VR apparatus to find the item locations.
Procedure
Before the start of the study patients read and signed the consent forms to participate in the ANTaging protocol [2]. Participants were tested on the virtual spatial memory task with different virtual navigation interfaces. Participants’ egocentric and allocentric spatial memory was assessed in two experimental sessions (1 h 15 min for each session; 2–3 days distance). The “vision only” and “bodily” interfaces were tested in the bodily session, while the remaining three were evaluated in the cognitive session. The two blocks were counterbalanced across participants, and the interfaces were randomized within each session. Four objects were chosen in each condition by the VR software from a random list of eight. Each random pick balanced the living and non-living categories of items.
Neuropsychological tests were carried out at the end of the bodily block so that each block consisted of the same amount of cognitive load (three cognitive tasks for each block). These tests included the Corsi block-tapping (CBT) [38] task and the Corsi supra-span (CSS) [39] as measures of short and long-term visuospatial memory.
Neuropsychological measures
CBT (score range 0–9) was corrected by age and education and considered pathological when the score was ≤ 3.46. At the same time, CSS (score range 1.08–29.16) was corrected by age, education, and CBT span and was considered pathological for scores ≤ 4.99 (CBT = 4) and ≤ 7.25 (CBT = 5). Other standardized psychological measures were taken in a separate session. MMSE [33], frontal assessment battery (FAB) [40], trail making test part A and B (TMT-AB) [41], free and cued selective reminding test (FCSRT) [42], the Raven’s colored progressive matrices (RCPM) [43], and the geriatric depression scale (GDS) [44] were also included.
Statistical analyses
R software (v 3.6.3) was used for the analyses. We conducted linear mixed-effects ANOVA with restricted maximum likelihood according to Luke’s guidelines [45] with lme4 package. Post-hoc analyses (i.e., pairwise comparisons) with Bonferroni correction were carried out with emmeans package [47]. To account for subject and object level variability, we added these terms to control them as random factors with random intercept. R formula for the models was lmer(error∼landmark * encoding navigation cues + (1|participant ID) + (1|object ID), control = lmerControl(optimizer = “bobyqa”), REML = T). Model assumptions were checked by visual inspection of the normality of residuals, collinearity, homogeneity of variance, and linearity plots.
ANOVA effect size (η2p) was interpreted according to small = 0.01, medium = 0.06, and large = 0.14 [48]. One participant could not complete the immersive condition due to VR discomfort (16 trials missing), and immersive and vision only data from another participant could not be saved due to technical issues during the experiment (32 trials missing). GDS and CSS for one participant were missing. Nevertheless, linear mixed-effects is a great method that handles missing values properly without deleting the whole case [49]. α level was set to 0.05.
RESULTS
Table 1 shows the cognitive characteristics of the patients (the majority had an aMCI phenotype). All had the above pathology cut-off scores for global cognition (MMSE) and short-term visuospatial memory (CBT); however, long-term visuospatial memory (CSS) showed high variability across participants (range = 5.64–23.60). Therefore, we decided to adjust the model with MCI phenotype and CSS. At the sample level, average scores of FAB, TMT-A, TMT-B, FCSRT (immediate and delayed), RCPM were within the normal range (one patient had missing FAB, FCSRT, and RCPM). This confirmed balanced MCI phenotype in the sample.
The bodily and cognitive blocks were analyzed with one linear mixed-effects model. We analyzed 480 the bodily block (48 missing trials) and 720 cognitive block (no missing trials) observations from 15 patients. For each navigation condition, the software randomly chose four items out of eight possible, as a result, apple was tested at 15.67%, bike at 13.33%, carrot at 11%, cat at 10%, chair at 11.67%, goose at 11.33%, hammer at 12.67%, trumpet at 10.33% of the times. Encoding time for the five conditions was not statistically significant (p > 0.05).
Effect of the bodily and visual-cognitive cues on spatial memory
We conducted a unique model to compare the performances (virtual error in meters) across the five encoding navigation cue conditions (5 levels) on egocentric and allocentric landmarks (2 levels) at recall. A (5×2) within-subjects ANOVA adjusting for CSS and MCI phenotype was carried out. We found a main effect of the Encoding Navigation Cues conditions (F4 = 4.06, p = 0.001, η2p = 0.02). No other significant effect was found. Post-hoc analyses with Bonferroni showed that the bodily condition yielded better spatial memory compared to vision only (adj. est. diff =–2.73, p = 0.047), the interactive allocentric map significantly improved spatial memory compared to free navigation (adj. est. diff. =–2.79, p = 0.021), and the bodily condition was superior compared to the free navigation (adj. est. diff. =–3.48, SE = 0.96, p = 0.003). No other comparisons were found to be significant. See Fig. 1 for this finding. In addition to CSS and MCI type, we added age and gender and results were confirmed.
Fig. 1
Main effect of the assistive virtual interfaces.
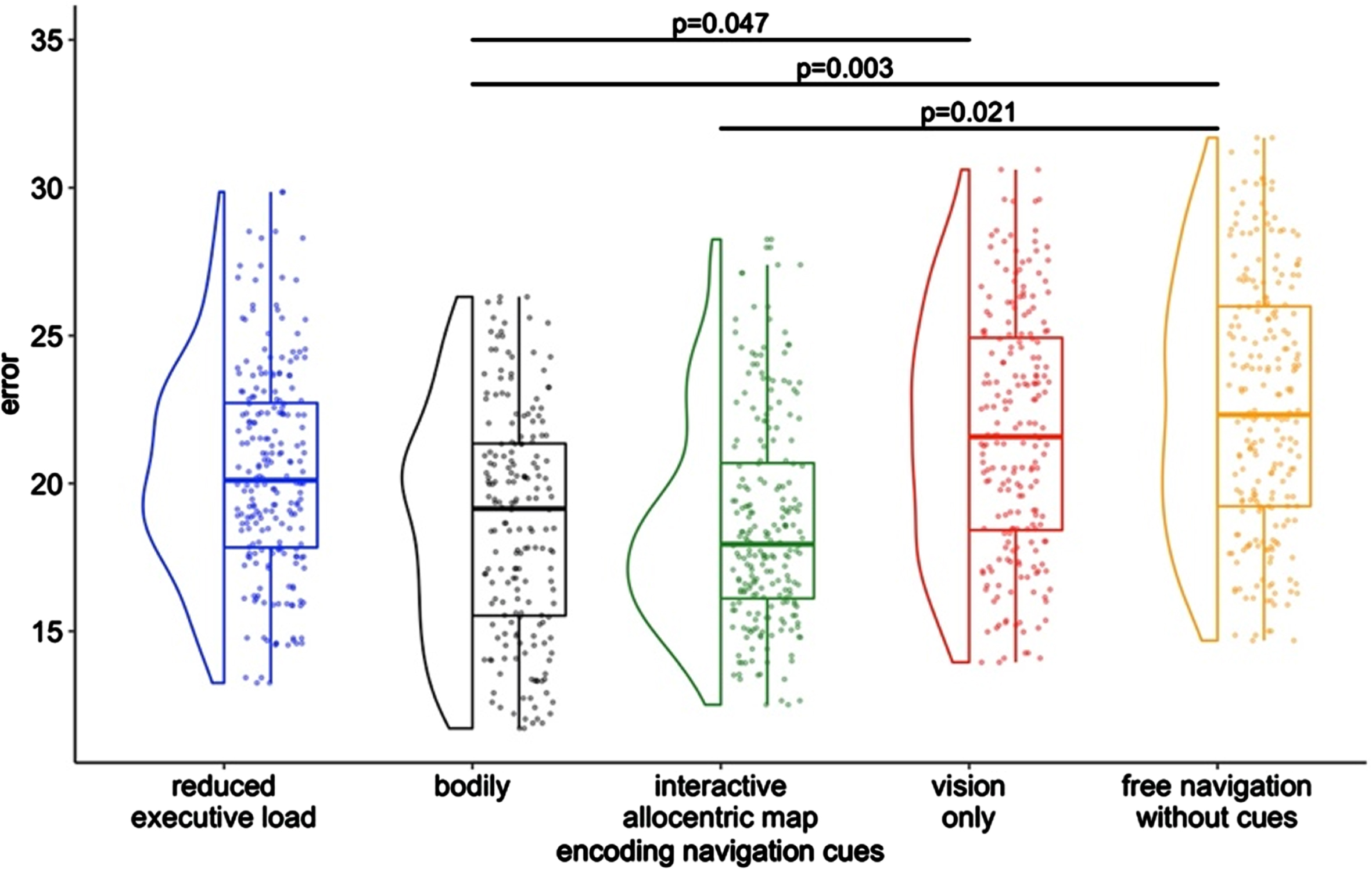
In addition, we computed for each object location the maximum possible error within the constraint of the arena (50 virtual meters). Since objects differ in locations within the arena, their maximum score is relative to arena constraint. We divided such distances by two and dichotomized object trials below or above each item mid-point (cut-off; i.e., if maximum error is 35, the cut-off is 17.5). We used a χ2 test to verify if the frequencies of object cut-offs differed in the five navigational interfaces, regardless of landmark since we did not find any significant effects. The association between navigation type and error cut-offs was found to be statistically significant (χ2 = 11, p = 0.027). In particular, the bodily and map navigation were positively associated with below the mean performance (lower error), whereas the free and vision only navigation were positively associated with above the mean performance (higher error). The reduced executive load condition showed a balanced pattern. Figure 2 shows residuals indicating the direction and strength of the associations between the two categorical variables.
Fig. 2
Associations between object performance and the encoding navigation cue. Higher residuals indicate stronger associations. Positive numbers a positive association, whereas negative residuals a negative association. Above cut-off represents higher error.
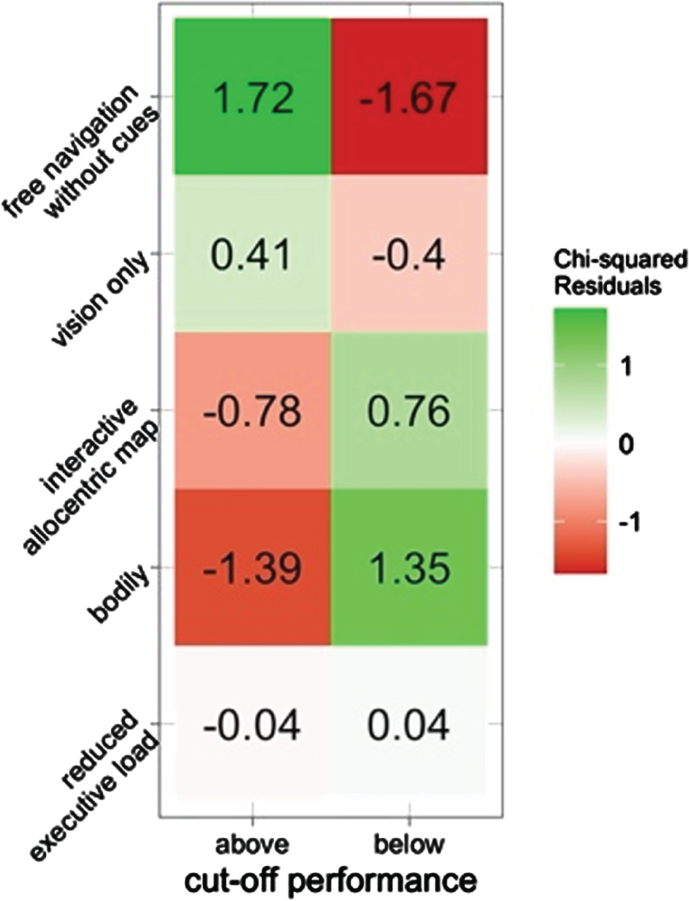
To verify the presence of testing effect in the conditions we computed repeated measure Pearson correlations [50] between the trial numbers and the participants’ error for each encoding navigation cues and type of landmark at recall. We only found a positive correlation (rrm(104) = 0.23, 95% CI[0.043, 0.405], p = 0.017) between allocentric error and trials in the free navigation without cues. No other effect was found. Figure 3 shows the repeated measure correlation plot for the significant result.
Fig. 3
Significant allocentric memory testing effect in the free navigation. Each dot represents the average error, color identifies the participant, and colored lines show repeated measure correlation fits for each participant.
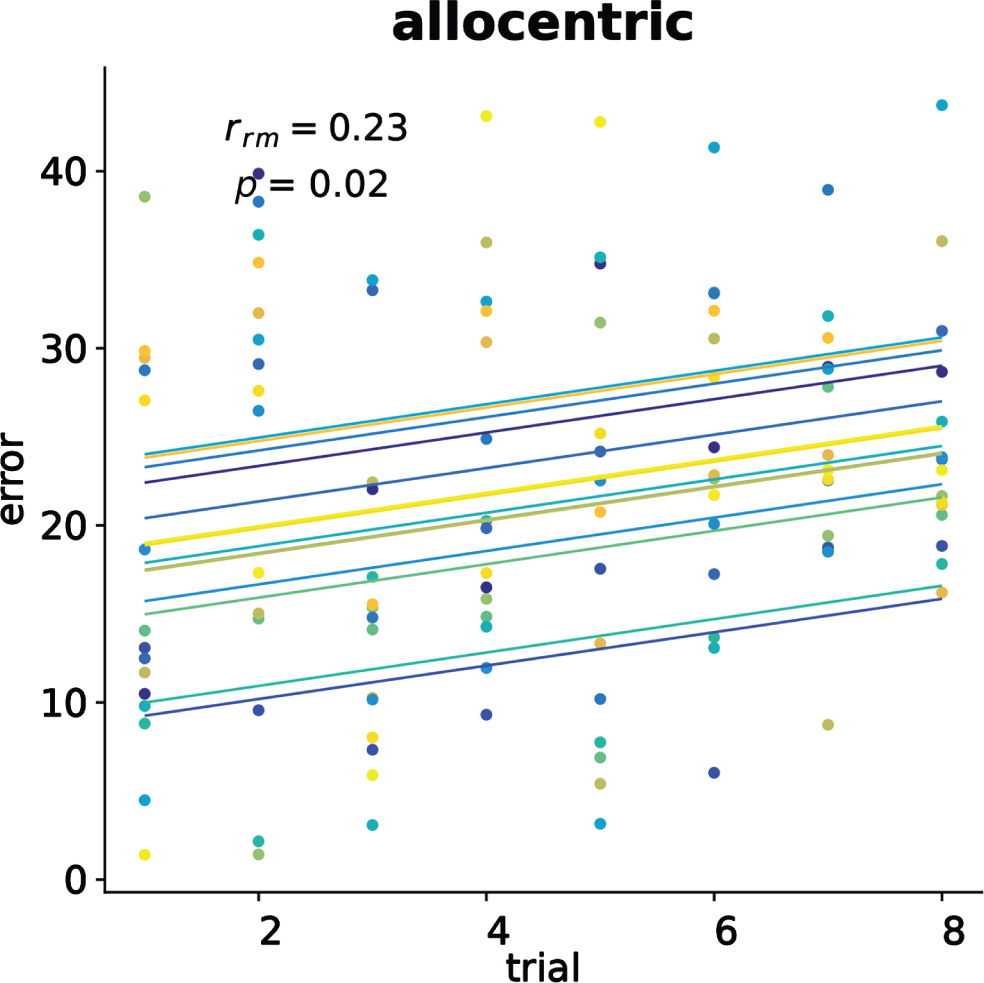
DISCUSSION
In this study, we sought to understand the effectiveness of various types of navigational encoding aids for enhancing egocentric and allocentric spatial memory recall in the MCI population.
Consistent with our predictions we found that bodily cues involved during immersive VR enhanced spatial memory in contrast to the conditions involving the vision only and free navigation without cues. Again, the interactive allocentric map condition was superior to the free navigation without cues. Conversely, one result was unexpected: the reduced executive load condition was not superior to the free navigation. Lastly, no differences were found between the vision only and the visual-cognitive aids conditions. Correlation analysis between aids conditions and performance above and below the cut-off for each object tested confirmed that bodily and allocentric map conditions were positively related to better performances, the vision only and free navigation without cues were positively associated with lower performance, and the reduced executive load had no association with performance. Intriguingly, bodily or visual-cognitive cues did not influence egocentric and allocentric spatial memory recall. Nevertheless, testing effect analyses showed free navigation negatively affected testing trials in the allocentric condition.
Previous research has revealed that bodily involvement (motor information, proprioception, vestibular information) could enhance spatial memory in older adults [36] and individuals with MCI [25, 26]. Our findings extend and confirm the idea that incorporating bodily information during navigation can enhance cognitive spatial representations. Indeed, following the modality-dependent hypothesis [1] and embodied cognition theories [51], cognitive representations of the environment arise also from sensorimotor and bodily information acquired and retrieved during navigation. Our finding is also consistent with re-enactment studies in which sensorimotor traces in the brain enable deeper encoding and effortful retrieval [30, 36, 52, 53]. Multisensory bodily information can be stored in sensorimotor responsive cortices and reactivated during retrieval, suggesting that encoding and retrieval are interdependent, hence the extent of recall facilitation depends on the degree to which recollection information overlaps with encoding one [54, 55]. It could be argued that information involved during bodily immersive VR compared to vision only has led to enriched cognitive representations of the space. This is also supported by an enhancement of spatial memory in the bodily compared to the free navigation condition. This result can be explained because the former manipulation has a higher degree of bodily involvement (immersive VR versus desktop PC) and the presence of directional cues, which were absent in the free navigation. Such a set of findings is in contrast to the so-called modality-independent hypothesis that states that cognitive representations of space are amodal [27]. However, research is still in its infancy and researchers are currently debating the role of the body in cognitive representations of the environment.
We found that using an interactive allocentric map can enhance spatial memory performance for individuals MCI when compared to navigating freely without aids. This is in line with previous hypotheses on the role of spatial reference frames synchronization in aging and AD dementia [56–58]. Within this theoretical framework, the map could serve as an external aid that helps the process of synchronizing egocentric and allocentric representations of space by providing a real-time interactive allocentric information of the experienced environment. The importance of an interactive map versus a less enriched map in MCI is also highlighted by Cognè and colleagues [23], who suggested that a map with low interactivity and visual details is not helpful in this population. Concerning free navigation, our result is in line with previous studies that indicated an excessive load on executive functions during navigation could hamper spatial memory [30, 36]. On the other hand, we found that the performance in the reduced executive load condition was comparable to the interactive map condition but surprisingly not superior to the free navigation. This contrasts previous research, that suggests the role of attentional markers and directional cues in improving spatial memory in individuals with MCI [23, 28]. It could be argued that attentional markers are more useful when used during navigation (e.g., [28]) rather than before (as in our case).
We found no difference in the bodily assistive interfaces on egocentric and allocentric spatial memory recall in MCI syndrome. This is in divergence with previous studies which found that motor interaction during virtual navigation in aMCI compared to vision only (passive navigation) enhances allocentric memory [25]. Moreover, immersive virtual navigation seems to enhance egocentric memory compared to the passive condition in MCI due to Parkinson’s disease with psychosis [26]. It could be possible that specific effects on the spatial frames of reference arise when focusing on the underlying etiology of cognitive impairment (e.g., MCI due to AD or Parkinson’s disease), which is associated with specific brain lesions. For instance, hippocampal lesions impair allocentric memory, whereas striatal lesions egocentric memory [34, 59].
Similarly, we found no impact of visual-cognitive cues on the spatial frames of reference. This contrasts with research on MCI, where studies have found that directional cues ameliorate egocentric memory [23]. Nevertheless, we demonstrated that allocentric recall across trials was hampered by free navigation. Research is still preliminary, and caution should be made regarding the impact of visual-cognitive cues on spatial frames of reference. In addition, our results show that the visual-cognitive aids are not superior to the vision only condition (i.e., passive navigation). It is possible that passive navigation reduces the cognitive load due to technological interaction [30, 36] and this allows for comparable effects with visual-cognitive conditions.
This research presents some limitations that we need to acknowledge. The immersive VR set-up used in the current study is not comparable to a walking tracking system; however, the use of 3dRudder has already been successfully used to study spatial navigation with immersive VR for cognitive-motor task [60]. Then, we adopted clinical criteria for MCI, it would be interesting to explore the performance in a group of MCI with specific etiology/biomarker patients, like MCI due to AD with hippocampal degeneration or MCI due to Parkinson’s disease. Lastly, we collected data after the COVID-19 pandemic Italian restrictions (first patient tested in April 2021 and the last one March 2023); it could be argued that spatial navigation abilities might have declined after the quarantine period or that patients assessed after the quarantine were more impaired than patients assessed after two years of the restrictions.
To conclude, both bodily and visual-cognitive navigation encoding cues provide effective information to enhance spatial memory and navigation in individuals with MCI clinical syndrome. These findings challenge the concept of the amodal cognitive representation of space, suggesting that it can be influenced by the modality in which the environment was initially processed.
AUTHOR CONTRIBUTIONS
Cosimo Tuena (Formal analysis; Funding acquisition; Investigation; Methodology; Project administration; Writing – original draft; Writing – review & editing); Silvia Serino (Conceptualization; Writing – review & editing); Karine Marie Goulene (Investigation); Elisa Pedroli (Investigation); Marco Stramba-Badiale (Supervision); Giuseppe Riva (Supervision).
ACKNOWLEDGMENTS
The authors wish to thank the patients involved in this study.
FUNDING
The publication fee has been supported by Ricerca Corrente from Italian Ministry of Health – The project has been supported by the Grant (Starting Grant Ricerca Finalizzata, code: SG-2018-12368175).
CONFLICT OF INTEREST
The authors have no conflict of interest to report.
DATA AVAILABILITY
Data are available upon reasonable request at https://doi.org/10.5281/zenodo.10069081.
REFERENCES
[1] | Steel A , Robertson CE , Taube JS ((2021) ) Current promises and limitations of combined virtual reality and functional magnetic resonance imaging research in humans: A commentary on Huffman and Ekstrom (2019). J Cogn Neurosci 33: , 159–166. |
[2] | Tuena C , Riva G ((2022) ) Active navigation training: An innovative embodied-based training system for spatial navigation in aging. Cyberpsychol Behav Soc Netw 25: , 77–78. |
[3] | Kaoua BN , Landuran A , Sauzéon H ((2019) ) Wayfinding in a virtual environment and down syndrome: The impact of navigational aids. Neuropsychology 33: , 1045–1056. |
[4] | Petersen RC ((2011) ) Mild cognitive impairment. N Engl J Med 364: , 2227–2234. |
[5] | Petersen RC , Doody R , Kurz A , Mohs RC , Morris JC , Rabins P V. , Ritcihie K , Rossor M , Thal L , Winblad B ((2001) ) Current concepts in mild cognitive impairment. Arch Neurol 58: , 1985–1992. |
[6] | Coughlan G , Laczó J , Hort J , Minihane A-M , Hornberger M ((2018) ) Spatial navigation deficits — overlooked cognitive marker for preclinical Alzheimer disease? Nat Rev Neurol 14: , 496–506. |
[7] | Serino S , Cipresso P , Morganti F , Riva G ((2014) ) The role of egocentric and allocentric abilities in Alzheimer’s disease: A systematic review. Ageing Res Rev 16: , 32–44. |
[8] | Nedelska Z , Laczo J , Uller M , Vyhnalek M , Fripp J , Vlcek K , Parizkova M , Hort J ((2017) ) Dementia with Lewy bodies: Severe impairment of real-space navigation skills examined with human analogue of Morris water maze and their structural underpinnings. J Neurol Sci 381: , 83–84. |
[9] | Uc EY , Rizzo M , Anderson SW , Sparks JD , Rodnitzky RL , Dawson JD ((2007) ) Impaired navigation in drivers with Parkinson’s disease. Brain 130: , 2433–2440. |
[10] | Lowry E , Puthusseryppady V , Coughlan G , Jeffs S , Hornberger M ((2020) ) Path Integration changes as a cognitive marker for vascular cognitive impairment?—a pilot study. Front Hum Neurosci 14: , 131. |
[11] | Tu S , Spiers HJ , Hodges JR , Piguet O , Hornberger M ((2017) ) Egocentric versus allocentric spatial memory in behavioral variant frontotemporal dementia and Alzheimer’s disease. J Alzheimers Dis 59: , 883–892. |
[12] | Cerman J , Andel R , Laczo J , Vyhnalek M , Nedelska Z , Mokrisova I , Sheardova K , Hort J ((2018) ) Subjective spatial navigation complaints – a frequent symptom reported by patients with subjective cognitive decline, mild cognitive impairment and Alzheimer’s disease. Curr Alzheimer Res 15: , 219–228. |
[13] | Lester AW , Moffat SD , Wiener JM , Barnes CA , Wolbers T ((2017) ) The aging navigational system. Neuron 95: , 1019–1035. |
[14] | Barry C , Burgess N ((2014) ) Neural mechanisms of self-location. Curr Biol 24: , R330–R339. |
[15] | Burgess N ((2008) ) Spatial cognition and the brain. Ann N Y Acad Sci 1124: , 77–97. |
[16] | Tuena C , Mancuso V , Stramba-Badiale C , Pedroli E , Stramba-Badiale M , Riva G , Repetto C ((2021) ) Egocentric and allocentric spatial memory in mild cognitive impairment with real-world and virtual navigation tasks: A systematic review. J Alzheimers Dis 79: , 95–116. |
[17] | Laczó J , Andel R , Vlček K , Macoška V , Vyhnálek M , Tolar M , Bojar M , Hort J ((2011) ) Spatial navigation and APOE in amnestic mild cognitive impairment. Neurodegener Dis 8: , 169–177. |
[18] | Laczó J , Vlček K , Vyhnálek M , Vajnerová O , Ort M , Holmerová I , Tolar M , Andel R , Bojar M , Hort J ((2009) ) Spatial navigation testing discriminates two types of amnestic mild cognitive impairment. Behav Brain Res 202: , 252–259. |
[19] | Boccia M , Silveri MC , Sabatini U , Guariglia C , Nemmi F ((2016) ) Neural underpinnings of the decline of topographical memory in mild cognitive impairment. Am J Alzheimers Dis Other Demen 31: , 618–630. |
[20] | Laczó J , Andel R , Vyhnalek M , Vlcek K , Magerova H , Varjassyova A , Tolar M , Hort J ((2010) ) Human analogue of the Morris water maze for testing subjects at risk of Alzheimer’s disease. Neurodegener Dis 7: , 148–152. |
[21] | Schneider CB , Linse K , Schönfeld R , Brown S , Koch R , Reichmann H , Leplow B , Storch A ((2017) ) Spatial learning deficits in Parkinson’s disease with and without mild cognitive impairment. Parkinsonism Relat Disord 36: , 83–88. |
[22] | Tuena C , Serino S , Pedroli E , Stramba-Badiale M , Riva G , Repetto C ((2021) ) Building embodied spaces for spatial memory neurorehabilitation with virtual reality in normal and pathological aging. Brain Sci 11: , 1067. |
[23] | Cogné M , Auriacombe S , Vasa L , Tison F , Klinger E , Sauzéon H , Joseph P , N’Kaoua B ((2018) ) Are visual cues helpful for virtual spatial navigation and spatial memory in patients with mild cognitive impairment or Alzheimer’s disease? Neuropsychology 32: , 385–400. |
[24] | Neguţ A , Matu SA , Sava FA , David D ((2016) ) Virtual reality measures in neuropsychological assessment: A meta-analytic review. Clin Neuropsychol 30: , 165–184. |
[25] | Plancher G , Tirard A , Gyselinck V , Nicolas S , Piolino P ((2012) ) Using virtual reality to characterize episodic memory profiles in amnestic mild cognitive impairment and Alzheimer’s disease: Influence of active and passive encoding. Neuropsychologia 50: , 592–602. |
[26] | Tuena C , Riva G , Murru I , Campana L , Goulene KM , Pedroli E , Stramba-Badiale M ((2022) ) Contribution of cognitive and bodily navigation cues to egocentric and allocentric spatial memory in hallucinations due to Parkinson’s disease: A case report. Front Behav Neurosci 16: , 992498. |
[27] | Huffman DJ , Ekstrom AD ((2019) ) A modality-independent network underlies the retrieval of large-scale spatial environments in the human brain. Neuron 104: , 611–622.e7. |
[28] | Davis R , Ohman JM , Weisbeck C ((2017) ) Salient cues and wayfinding in Alzheimer’s disease within a virtual senior residence. Environ Behav 49: , 1038–1065. |
[29] | Cogné M , Taillade M , N’Kaoua B , Tarruella A , Klinger E , Larrue F , Sauzéon H , Joseph PA , Sorita E ((2017) ) The contribution of virtual reality to the diagnosis of spatial navigation disorders and to the study of the role of navigational aids: A systematic literature review. Ann Phys Rehabil Med 60: , 164–176. |
[30] | Jebara N , Orriols E , Zaoui M , Berthoz A , Piolino P ((2014) ) Effects of enactment in episodic memory: A pilot virtual reality study with young and elderly adults. Front Aging Neurosci 6: , 338. |
[31] | Huffman DJ , Ekstrom AD ((2021) ) An important step toward understanding the role of body-based cues on human spatial memory for large-scale environments. J Cogn Neurosci 33: , 167–179. |
[32] | Albert MS , Dekosky ST , Dickson D , Dubois B , Feldman HH , Fox NC , Gamst A , Holtzman DM , Jagust WJ , Petersen RC , Snyder PJ , Carrillo MC , Thies B , Phelps CH ((2011) ) The diagnosis of mild cognitive impairment due to Alzheimer’s disease: Recommendations from the National Institute on Aging-Alzheimer’s Association workgroups on diagnostic guidelines for Alzheimer’s disease. Alzheimers Dement 7: , 270–279. |
[33] | Magni E , Binetti G , Bianchetti A , Rozzini R , Trabucchi M ((1996) ) Mini-mental state examination: A normative study in Italian elderly population. Eur J Neurol 3: , 198–202. |
[34] | Guderian S , Dzieciol XAM , Gadian DG , Jentschke XS , Doeller CF , Burgess N , Mishkin XM , Vargha-Khadem F ((2015) ) Hippocampal volume reduction in humans predicts impaired allocentric spatial memory in virtual-reality navigation. J Neurosci 35: , 14123–14131. |
[35] | Chersi F , Burgess N ((2015) ) The cognitive architecture of spatial navigation: Hippocampal and striatal contributions. Neuron 88: , 64–77. |
[36] | Tuena C , Serino S , Dutriaux L , Riva G , Piolino P ((2019) ) Virtual enactment effect on memory in young and aged populations: A systematic review. J Clin Med 8: , 620. |
[37] | Taube JS , Valerio S , Yoder RM ((2013) ) Is navigation in virtual reality with fMRI really navigation? J Cogn Neurosci 25: , 1008–1019. |
[38] | Monaco M , Costa A , Caltagirone C , Carlesimo GA ((2012) ) Forward and backward span for verbal and visuo-spatial data: Standardization and normative data from an Italian adult population. Neurol Sci 34: , 749–754. |
[39] | Spinnler H , Tognoni G ((1987) ) Standardizzazione e taratura italiana di test neuropsicologici. Ital J Neurol Sci Suppl 8: , 1–120. |
[40] | Appollonio I , Leone M , Isella V , Piamarta F , Consoli T , Villa ML , Forapani E , Russo A , Nichelli P ((2005) ) The Frontal Assessment Battery (FAB): Normative values in an Italian population sample. Neurol Sci 26: , 108–116. |
[41] | Mondini S , Mapelli D , Vestri A , Bisiacchi PS ((2003) ), Esame neuropsicologico breve. Una batteria di test per lo screening neuropsicologico. |
[42] | Frasson P , Ghiretti R , Catricalà E , Pomati S , Marcone A , Parisi L , Rossini PM , Cappa SF , Mariani C , Vanacore N , Clerici F ((2011) ) Free and cued selective reminding test: An Italian normative study. Neurol Sci 32: , 1057–1062. |
[43] | Basso A , Capitani E , Laiacona M ((1982) ) Raven’s coloured progressive matrices: Normative values on 305 adult normal controls. Funct Neurol 2: , 189–194. |
[44] | Laudisio A , Antonelli Incalzi R , Gemma A , Marzetti E , Pozzi G , Padua L , Bernabei R , Zuccalà G ((2018) ) Definition of a Geriatric Depression Scale cutoff based upon quality of life: A population-based study. Int J Geriatr Psychiatry 33: , e58–e64. |
[45] | Luke SG ((2017) ) Evaluating significance in linear mixed-effects models in R. Behav Res 49: , 1494–1502. |
[46] | Bates DM , Mächler M , Bolker BM , Walker S ((2015) ) Package Lme4: Linear mixed-effects models using Eigen and S4. J Stat Softw 67: , 1–48. |
[47] | Lenth RV ((2018) ) emmeans: Estimated marginal means, aka least-squares means. |
[48] | Richardson JTE ((2011) ) Eta squared and partial eta squared as measures of effect size in educational research. Educ Res Rev 6: , 135–147. |
[49] | Brown VA ((2021) ) An introduction to linear mixed-effects modeling in R. Adv Methods Pract Psychol Sci 4: , 1–19. https://doi.org/10.1177/2515245920960351. |
[50] | Bakdash JZ , Marusich LR ((2017) ) Repeated measures correlation. Front Psychol 8: , 456. |
[51] | Barsalou LW ((2008) ) Grounded cognition. Annu Rev Psychol 59: , 617–645. |
[52] | Kent C , Lamberts K ((2008) ) The encoding-retrieval relationship: Retrieval as mental simulation. Trends Cogn Sci 12: , 92–98. |
[53] | Engelkamp J ((1998) ) Memory for Actions, Psychology Press/Taylor & Francis (UK). |
[54] | Nyberg L , Habib R , McIntosh AR , Tulving E ((2000) ) Reactivation of encoding-related brain activity during memory retrieval. Proc Natl Acad Sci U S A 97: , 11120–11124. |
[55] | Madan CR , Singhal A ((2012) ) Using actions to enhance memory: Effects of enactment, gestures, and exercise on human memory. Front Psychol 3: , 507. |
[56] | Serino S , Riva G ((2015) ) How different spatial representations interact in virtual environments: The role of mental frame syncing. Cogn Process 16: , 191–201. |
[57] | Serino S , Pedroli E , Tuena C , De Leo G , Stramba-Badiale M , Goulene K , Mariotti NG , Riva G ((2017) ) A novel virtual reality-based training protocol for the enhancement of the “Mental Frame Syncing” in individuals with Alzheimer’s disease: A development-of-concept trial. Front Aging Neurosci 9: , 240. |
[58] | Serino S , Morganti F , Di Stefano F , Riva G ((2015) ) Detecting early egocentric and allocentric impairments deficits in Alzheimer’s disease: An experimental study with virtual reality. Front Aging Neurosci 7: , 88. |
[59] | Kuehn E , Perez-Lopez MB , Diersch N , Döhler J , Wolbers T , Riemer M ((2018) ) Embodiment in the aging mind. Neurosci Biobehav Rev 86: , 207–225. |
[60] | Credé S , Thrash T , Hölscher C , Fabrikant SI ((2020) ) The advantage of globally visible landmarks for spatial learning. J Environ Psychol 67: , 101369. |