Sildenafil as a Candidate Drug for Alzheimer’s Disease: Real-World Patient Data Observation and Mechanistic Observations from Patient-Induced Pluripotent Stem Cell-Derived Neurons
Abstract
Background:
Alzheimer’s disease (AD) is a chronic neurodegenerative disease needing effective therapeutics urgently. Sildenafil, one of the approved phosphodiesterase-5 inhibitors, has been implicated as having potential effect in AD.
Objective:
To investigate the potential therapeutic benefit of sildenafil on AD.
Methods:
We performed real-world patient data analysis using the MarketScan® Medicare Supplemental and the Clinformatics® databases. We conducted propensity score-stratified analyses after adjusting confounding factors (i.e., sex, age, race, and comorbidities). We used both familial and sporadic AD patient induced pluripotent stem cells (iPSC) derived neurons to evaluate the sildenafil’s mechanism-of-action.
Results:
We showed that sildenafil usage is associated with reduced likelihood of AD across four new drug compactor cohorts, including bumetanide, furosemide, spironolactone, and nifedipine. For instance, sildenafil usage is associated with a 54% reduced incidence of AD in MarketScan® (hazard ratio [HR] = 0.46, 95% CI 0.32– 0.66) and a 30% reduced prevalence of AD in Clinformatics® (HR = 0.70, 95% CI 0.49– 1.00) compared to spironolactone. We found that sildenafil treatment reduced tau hyperphosphorylation (pTau181 and pTau205) in a dose-dependent manner in both familial and sporadic AD patient iPSC-derived neurons. RNA-sequencing data analysis of sildenafil-treated AD patient iPSC-derived neurons reveals that sildenafil specifically target AD related genes and pathobiological pathways, mechanistically supporting the beneficial effect of sildenafil in AD.
Conclusions:
These real-world patient data validation and mechanistic observations from patient iPSC-derived neurons further suggested that sildenafil is a potential repurposable drug for AD. Yet, randomized clinical trials are warranted to validate the causal treatment effects of sildenafil in AD.
INTRODUCTION
Alzheimer’s disease (AD) is a progressive neurodegenerative disorder clinically characterized by memory impairment, cognitive dysfunction, and visuospatial impairment [1]. It is the most common cause of dementia in people aged 65 and older and ranked as the seventh leading cause of death in the United States [2]. The clinical diagnosis of the disease is difficult due to its variability among individuals and its overlapping phenotypes with other types of dementia [3]. The brain changes in AD involve neuronal accumulation of amyloid-β (Aβ) plaques and hyperphosphorylated tau (neurofibrillary tangles) which ultimately cause neurodegeneration [4, 5]. Risk factors for late-onset AD include age, genetics, family history, and non-genetic factors such as heart health and lifestyle influences [6]. Treatment options for AD historically focus on symptomatic relief by cholinesterase inhibitors and N-methyl-D-aspartate antagonists [7, 8]. Recently, FDA approved two drugs, aducanumab and lecanemab, aimed at removing Aβ plaques and demonstrating significant slowing of clinical symptoms [9, 10]. These agents are applicable to a relatively small proportion of the AD population, those with early, mild symptoms and no factors that might increase side effects associated with these agents [2].
Sildenafil, a phosphodiesterase-5 (PDE5) inhibitor, has shown potential as a treatment for AD. It has a protective effect on AD by modulating various pathways involved in the disease progression [11]. Sildenafil administration has been associated with improvements in memory performance, histopathological changes, and oxidative stress markers. It promotes peroxisome proliferator-activated receptor-γ coactivator 1α (PGC1α) signaling, which inhibits β-secretase 1 expression, reduces Aβ generation, increases levels of antioxidant enzymes, enhances mitochondrial biogenesis, and ultimately improves brain perfusion, insulin sensitivity, long-term potentiation, and neurogenesis (reviewed in [12]). Sildenafil treatment in animal models of AD reverses cognitive impairment, reduces tau hyperphosphorylation, and increases levels of brain-derived neurotrophic factor (BDNF) without modifying amyloid burden [11]. A previous study of our group utilized an endophenotype network-based methodology for in silico drug repurposing in AD and discovered that sildenafil is associated with a reduced incidence of AD in real-world patient data [13]. This study also showed in vitro mechanistic observations in human microglia cells and AD patient-induced pluripotent stem cell (iPSC)-derived neurons indicative of a therapeutic effect of sildenafil. The advancement in the past decade of techniques to generate human iPSCs and an ability to differentiate them into the different cell types, including brain cells, has unleashed a new era of neurodegenerative disease research [14, 15]. Human iPSC-derived neural progenitors are an effective drug discovery model as, unlike most other AD model systems, these cultures do not require exogenous expression of mutant proteins to mimic disease-relevant pathologies. They provide several advantages over other systems and are likely to facilitate novel insights into AD patho-mechanisms.
In this study, we used MarketScan® Medicare Supplemental and Clinformatics® databases and performed new patient data analyses. We used four new comparator drug cohorts for sildenafil, including a calcium channel blocker (nifedipine) and three diuretics (bumetanide, furosemide, and spironolactone). Results here complement our earlier findings in large patient cohort data and strengthen the hypothesis of significant association of sildenafil usage with reduced risk of AD [13]. We used multiple AD patient iPSC derived neurons to test the role of sildenafil in reducing tau phosphorylation. Finally, RNA-seq analysis of sildenafil-treated patients derived neuron provided critical insights for potential mechanism-of-actions on various AD-related pathobiological pathways.
METHODS
Study cohorts
The pharmacoepidemiology study utilized the MarketScan® Medicare Supplemental data (years: 2012– 2017) and the Clinformatics® data (years: 2007– 2020). Pharmacy claims of sildenafil, bumetanide, furosemide, nifedipine, and spironolactone were identified by using RxNorm, and the drug lookup tables of the MarketScan data and the Clinformatics® data. We used the same approach as in our previous work to define drug exposure episodes (e.g., from drug initiation to drug discontinuation) [13]. We searched all individuals in the MarketScan data and individuals with ≥3 years of continuous enrollment in the Clinformatics® data, as the Clinformatics® data covered more calendar years. Based on the European Society of Cardiology (ESC) and the European Respiratory Society (ERS) Guidelines for treatment of pulmonary hypertension (PH), calcium channel blockers (e.g., nifedipine) and diuretics (bumetanide, furosemide, and spironolactone) have been used to treat patients with PH [16– 18]. For both MarketScan and Clinformatics® data, the sildenafil cohort included the first sildenafil episode for everyone, as well as the bumetanide cohort, the furosemide cohort, the nifedipine cohort, and the spironolactone cohort. We chose these four drugs as compactor drug cohorts because these four drugs were used in treatment of PH, which reduces the indication confounding for sildenafil. Further, we excluded observations that started drug within 180 days of insurance enrollment. The demographics of the final cohorts are presented in Supplementary Tables 1 and 2.
Outcome definition
We used the same algorithm to define AD as described in our previous work [13]. A recent study determined this method has a positive predictive value of 74.8% and sensitivity of 71.3% [19]. The primary outcome in pharmacoepidemiologic studies is time from drug initiation to AD diagnosis. Observations without diagnosis of AD were censored at end of drug episodes.
Measurement of confounders
We incorporated comorbidities common among older adults and/or associated with AD. We used the R package comorbidity to define various chronic conditions, including cancer, cerebrovascular disease, chronic pulmonary disease, diabetes, heart disease (myocardial infarction and congestive heart failure), liver disease, and renal disease (Gasparini, A. Package ‘comorbidity’. Vol. 2022 (2022)). We used the algorithm developed by Yang et al. [20] to define Parkinson’s disease, the algorithm derived by Chen et al. [21] to define fall and hypertension, the algorithm derived by Quan et al. [22] to define depression, and the algorithm derived by Anna Nordström and Peter Nordström [23] to define traumatic brain injury. In MarketScan® data analysis, we used the same algorithm to define hypertension as described previously [13]. Additionally, we used ICD codes recommended from the National Cancer Institute Division of Cancer Control and Population Sciences to define comorbidities in the Charlson Comorbidity Index (e.g., myocardial infarction, congestive heart failure, peripheral vascular disease, cerebrovascular disease, dementia, chronic pulmonary disease, connective tissue disease-rheumatic disease, peptic ulcer disease, mild liver disease, diabetes without complications, diabetes with complications, paraplegia and hemiplegia, renal disease, cancer, moderate or severe liver disease, metastatic carcinoma, and AIDS/HIV) (National Cancer Institute division of cancer control and population sciences. SEER-Medicare: Comorbidity SAS Macros. Vol. (2021). URL: https://healthcaredelivery.cancer.gov/seermedicare/considerations/calculation.html).
Statistical analysis
We used logistic regression modeling to estimate the propensity score (PS) for each comparison. We estimated the PS by using the covariates in Supplementary Tables 1 and 2 in both Clinformatics® and MarketScan® analyses, respectively. Subsequently, we perform PS-stratified survival analyses using the Cox model. We estimated the hazard ratios, 95% confidence intervals, and p-values as described in our previous study [13].
iPSCs culture and generation of mature neurons
Forebrain neurons were generated from four AD patient-derived iPSC lines (iPSC detail: Supplementary Table 3). Briefly, iPSC lines were cultured and maintained in mTeSRtrademark1 medium. At day 0, the cells were dissociated with accutase and plated into Matrigel-coated 10 cm tissue culture dish with a density of 1– 1.5×105 cells/cm2. Cells were cultured in neural induction medium (05839, STEMCELL Technologies) in a 37°C, 5% CO2 incubator to form monolayer neural progenitor cells (NPCs). These NPCs were passaged at day 6, day 12. At day 18, cells were dissociated and cultured in forebrain neuron differentiation medium (08600, STEMCELL Technologies). At day 25, neuronal precursors cells were harvested and plated in 6 well tissue culture plate coated with Matrigel with a density of 2– 2.5× 105 cells/cm2 in forebrain neuron maturation medium (08605, STEMCELL Technologies). After 10 days of maturation (day 35), neurons were treated with sildenafil at different concentrations (10– 100μM) along with DMSO for 5 days.
Western blot and ELISA
At day 40, mature forebrain neurons treated with sildenafil were harvested, washed with cold PBS, and then lysed with N-PERtrademark Neuronal Protein Extraction Reagent (Cat# 87792, Thermo Scientific) adding 1% Protease/Phosphatase Inhibitor (Cat# PI78442, Thermo Scientific). Total protein concentration was measured using the Bradford assay kit (Bio-Rad, USA) according to the manufacturer’s manual. Samples were electrophoresed by sodium dodecyl sulfate-polyacrylamide gel electrophoresis (SDS-PAGE) and blotted onto a polyvinylidene difluoride (PVDF; EMD Millipore, Darmstadt, Germany) membrane. After transferring, the membranes were probed with specific primary antibodies (1 : 1000) at 4°C overnight. Specific protein bands were detected using a chemiluminescence reagent after hybridization with a horseradish peroxidase (HRP)-conjugated secondary antibody (1 : 8000). Band intensities were normalized with GAPDH and compared by performing densitometry analysis (ImageJ). For pTau231/total tau measurement, lysate was run on a Phospho (Thr231)/Total Tau Kit (K15121D, MSD, USA) following manufacturers protocol with minor modifications.
mRNA isolation and Illumina transcriptome library preparation
Cells were collected after 5 days treatment of sildenafil. Total RNA was extracted using a RNeasy mini kit, in combination with RNAase-free DNAase to remove the potential genomic DNA contamination (Qiagen). RNA concentration was quantified with a Nanodrop 2000C Spectrophotometer.
RNA-seq data analysis
Paired-end RNA sequencing was performed using the Illumina NovaSeq platform for a male FAD patient derived iPSCs treated with different concentration of sildenafil, i.e., 10, 25, 50, 75, and 100μM with replicates. Raw fastq reads were first subjected to the quality filtering using fastp v0.23.2 [24]. Quality filtered reads were aligned to the reference human genome hg38 (GRCh38/108 build) employing Rsubread 2.8.1 [25] package with default settings except multiple threads. Gene-wise read counts were obtained with feature Counts utilizing inbuilt hg38 RefSeq annotation and duplicates were ignored in read summarization [25, 26]. Gene symbol is retrieved using org.Hs.eg.db v3.15.0 human annotation package. Further, edgeR v3.38.4 [27] and limma-voom v3.52.4 [28, 29] packages were used for preprocessing, filtering, normalization and evaluating differentially expressed genes (DEGs). Low expressed genes were filtered and normalized using edgeR package. Design matrix and contrasts were created. Genes with false discovery rate (FDR) adjusted p-value of 0.05 or less were considered as significantly regulated. DEGs were depicted thorough volcano plot using EnhancedVolcano v1.16.0 package. Gene ontology and pathway enrichment analysis is performed using ShinyGo v0.77 [30], KEGG [31], PANTHER [32], and Pathview [33]. Genes-pathways relationship network is visualized in Cytoscape v3.9.1 [34].
Reagents
Sildenafil: Sildenafil Citrate (S0986, TCI). Primary antibodies used are Tuj1 (ab18207, Abcam), pTau181 (Phospho-Tau (Thr181) (D9F4G) Rabbit mAb #12885, CST), pTau205 (Phospho-Tau (Thr205) (E7D3E) Rabbit mAb #49561, CST), and GAPDH (GAPDH (D4C6R) Mouse mAb #97166, CST)). Secondary antibodies: HRP-conjugated anti-rabbit and anti-mouse antibodies (Open Biosystems, USA) were used in the study.
RESULTS
Patient data analysis reveals sildenafil’s association with reduced AD incidence
Our previous studies identified that sildenafil’s user was associated with reduced incidence of AD in a single health insurance claim database [13]. However, these preliminary findings should be further validated in independent real-world patient databases. Further, other studies showed that PDE5 inhibitors were not associated with reduced risk of dementia in individuals with PH using active comparator design [35]. We thus performed new patient data analyses using both the MarketScan® Medicare Supplemental database and OPTUM database as described in our original study [13]. We selected a new calcium channel blocker (nifedipine) and three diuretics (bumetanide, furosemide, and spironolactone) as comparator drugs because these four drugs were used to treat pulmonary hypertension, which will reduce drug’s indication confounding factor for sildenafil. In total, we adjusted sex, age, race, and 13 comorbidities in OPTUM analyses, as well as sex, age, geographic location, and 18 comorbidities in the MarketScan analyses. We found that sildenafil usage is significantly associated with reduced incidence of AD across all four drug cohorts, including bumetanide, furosemide, spironolactone, and nifedipine in both MarketScan supplemental and OPTUM databases (Fig. 1). Subgroup analysis further revealed sildenafil usage in individuals with hypertension to be associated with reduced likelihood of AD across all four drug cohorts as well. Bumetanide exposure was associated with significantly lower AD prevalence in two electronic health record databases, and this was conceptually validated in APOE4-knock-in AD mice [36]. We found sildenafil to be associated with reduced AD prevalence compared to bumetanide in the treated population, and in the treated individuals with hypertension, for two independent patient claims databases (Fig. 1).
Fig. 1
Longitudinal analyses reveal that sildenafil usage is associated with reduced incidence of AD in two independent patient databases. We used real-world patient data from the MarketScan® Medicare Supplemental Database and Clinformatics® (OPTUM) database (see Methods). Four drug cohorts in both all population and individuals with hypertension (HTN) were conducted respectively: sildenafil vs. a calcium channel blocker (nifedipine) and sildenafil vs. three diuretics (bumetanide, furosemide, and spironolactone) respectively. We chose calcium channel blockers and diuretics as comparator drugs as they have been used to treat pulmonary hypertension (PH) on the European Society of Cardiology and the European Respiratory Society Guidelines for treatment of PH.
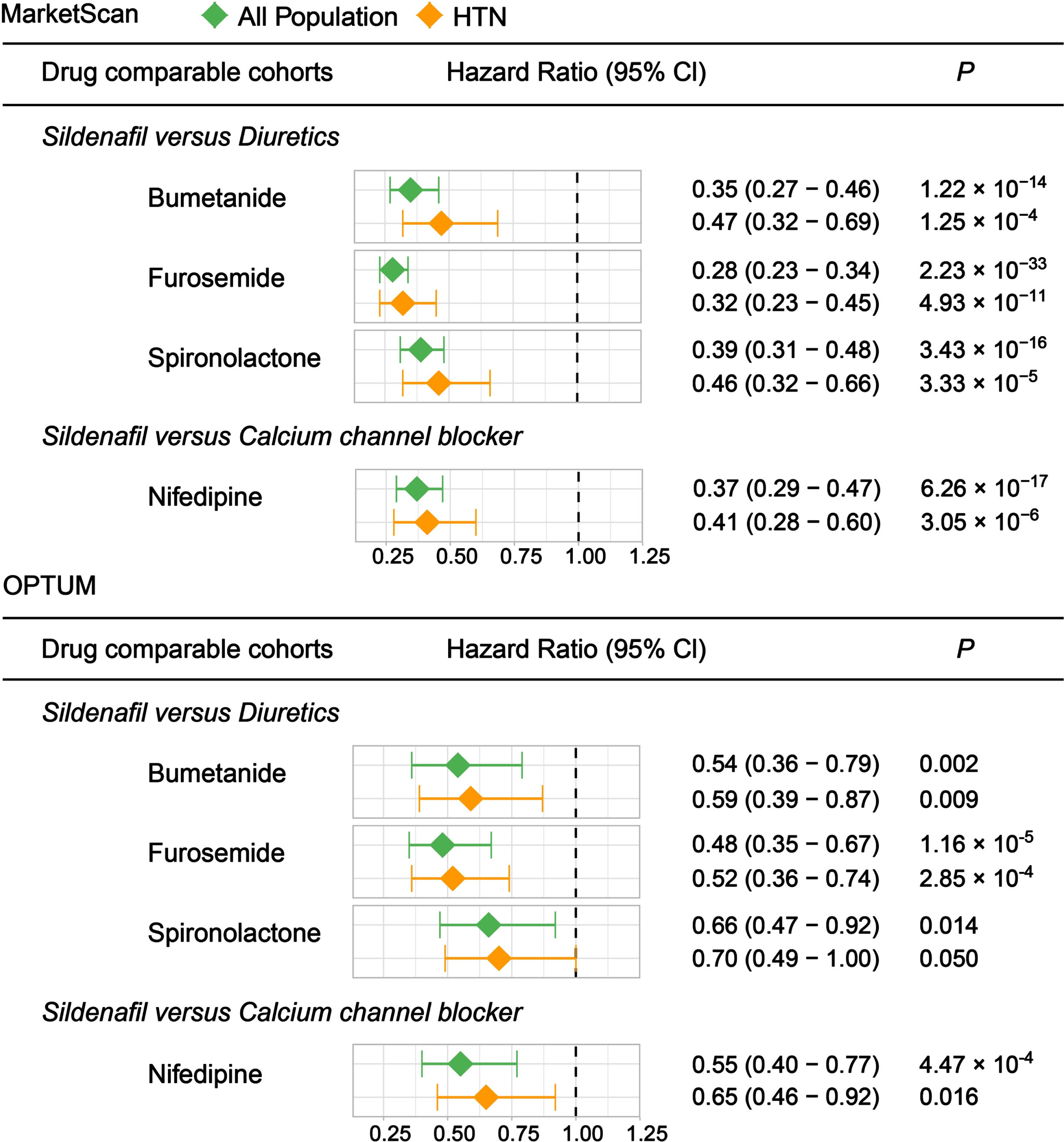
Overall, we found that clinical effect size measured by hazard ratio (HR) is smaller in the OPTUM database compared with MarketScan. Spironolactone, a potassium-sparing diuretic (water pill) used in the treatment of PH, is under investigation to treat African Americans with mild cognitive impairment and early AD (ClinicalTrials.gov Identifier: NCT04522739). We found that sildenafil usage was associated with a 54% reduced prevalence of AD in MarketScan (HR = 0.46, 95% CI 0.32– 0.66, p = 3.33×10–5) and a 30% reduced prevalence of AD in OPTUM (HR = 0.70, 95% CI 0.49– 1.00, p-value=0.05) compared to spironolactone usage. The heterogeneous effect sizes could be due to intrinsic differences between OPTUM and MarketScan data, as previously noted. Thus, the new patient data provide further support for reduced AD prevalence in patients exposed to sildenafil and suggest that future experimental and clinical studies are warranted.
Sildenafil reduces tau phosphorylation in AD patient iPSC-derived neurons
Understanding a drug’s mechanism-of-action is crucial for development of efficacious and safe therapeutics [37]. Human iPSC models have the advantage of mimicking in vitro pathogenic conditions and have been found to be a superior approach to drug screening and accelerating the drug development process [38, 39]. Hence, to test the effect of sildenafil on AD, we first used two iPSC lines derived from familial Alzheimer’s disease (FAD) patients and differentiated them into forebrain neurons (Fig. 2A). At first, iPSCs were differentiated into neural progenitor cells (NPCs) (day 18), and then differentiated into neural precursors (day 24). Neural precursors were further matured for 11 days. The conversion of mature neurons (at day 35) was confirmed by visualizing expression of Tuj1, neuronal marker under fluorescence microscope (Fig. 2B). Western blot analysis further suggested the increased tauopathy associated with AD patient iPSC derived neurons as compared to neurons derived from control (Fig. 2B). These AD neurons were treated with range of doses (10– 100μM) of sildenafil at day 35, when the process of differentiation and maturation are complete. We purposely chose the high range of sildenafil dose for the treatment considering its low cytotoxicity and side effects. After 5 days of treatment, the AD neurons were checked for their expression levels of phosphorylated tau (pTau181, pTau205). Phosphorylated-tau181 (pTau181) is an early biomarker of AD pathology and correlates well with cognitive declines [40] while, in contrast, Phosphorylated-tau205 (pTau205) and total tau levels increase at later stages of disease progression [41]. To investigate whether sildenafil reduces pTau accumulation in AD neurons, cells were lysed, and western blotting was performed to measure the levels of pTau181 and pTau205. We found that sildenafil significantly reduced the accumulation of both pTau181 and pTau205 in AD neurons (Fig. 2C, D). We further performed Phospho (Thr231)/Total Tau ELISA to check the pTau231 and total tau levels. The levels of both pTau231 and total tau were decreased with sildenafil treatment in both AD neurons (Fig. 2E, 2F). We tested two additional AD patient iPSC derived neurons (P10, P11) to further validate the effect of sildenafil on tau phosphorylation. We found decreased tau phosphorylation for both T181 and T205 following sildenafil treatment in P10 (Fig. 3A) and P11 (Fig. 3B). In summary, these data suggest protective effects of sildenafil by reducing tau and its phosphorylation in AD, complementing our earlier endophenotype-based prediction and population-based validation [13].
Fig. 2
iPSC model to evaluate effect of sildenafil on tau phosphorylation. In vitro neuro development model: Neural progenitor cells (NPCs) were differentiated from hiPSCs, which were then further induced to differentiate into mature forebrain neurons (A). Most cells differentiated from iPSCs stained positive for Tuj1 (marker of neuron), indicating the cells were mature neurons, western blot (WB) analysis of phosphorylated pTau181 and pTau205 in control and AD patient derived neurons (B). WB analysis (along with densitometry) of tau phosphorylation upon sildenafil treatment (5 days) (C, D), pTau231 and total tau were estimated using Phospho(Thr231)/Total Tau Kit (MSD, USA) (E, F) in two AD patients’ iPSC (P1,P2) derived neurons. Number of replicates (n): 3, p > 0.05 non-significant (ns), p≥0.05 (*), p≤0.01 (**), p≤0.001 (***), p≤0.0001 (****).
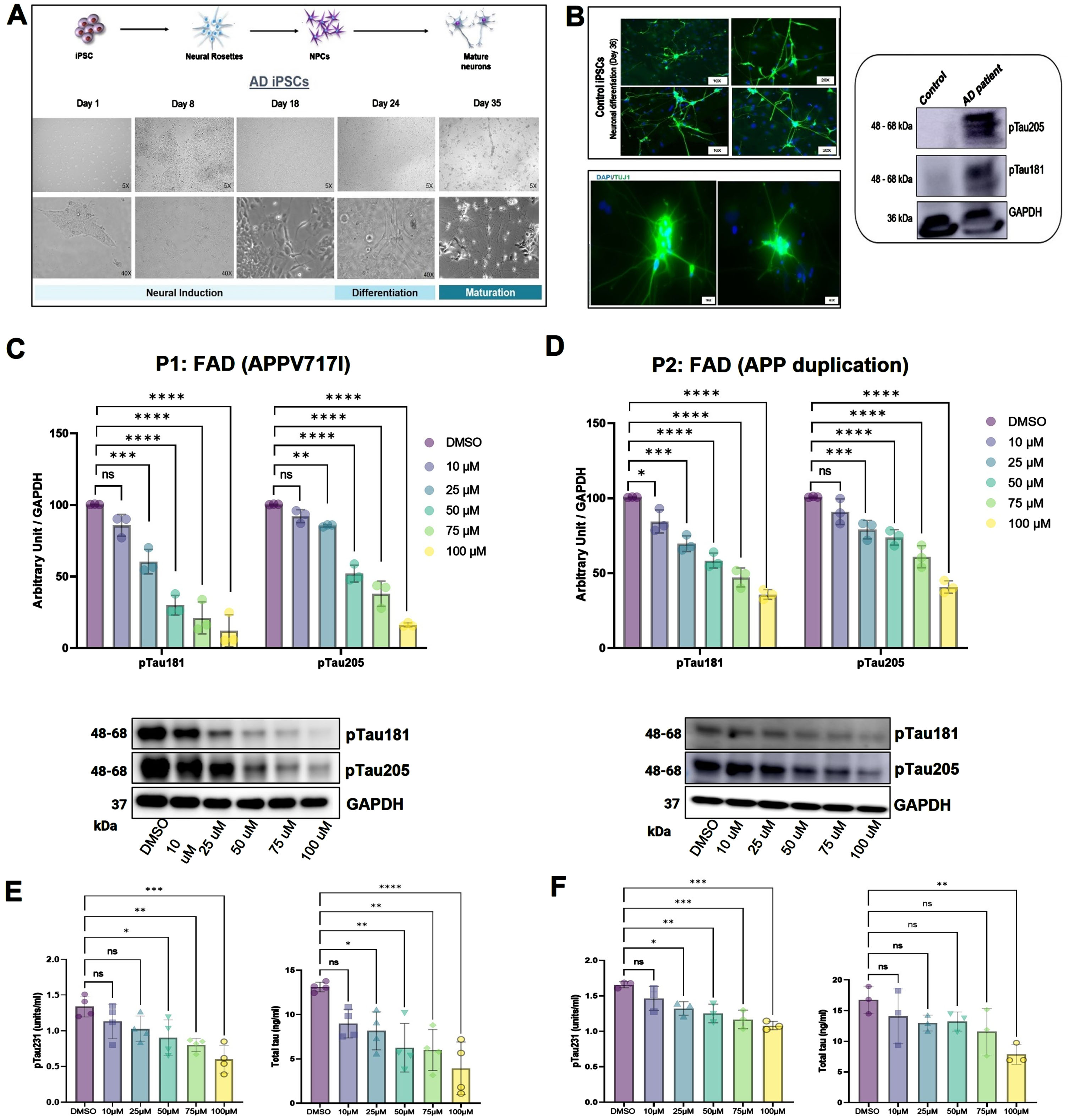
Fig. 3
Western blot (WB) analysis (along with densitometry) showing expression levels of pTau181 and pTau205 following 5 days of sildenafil treatment in two Alzheimer’s disease (AD) patients’ iPSC derived neurons P10 (A) and P11(B). Number of replicates (n): 3, p > 0.05 non-significant (ns), p≤0.05 (*), p≤0.01 (**), p≤0.001 (***), p≤0.0001 (****).
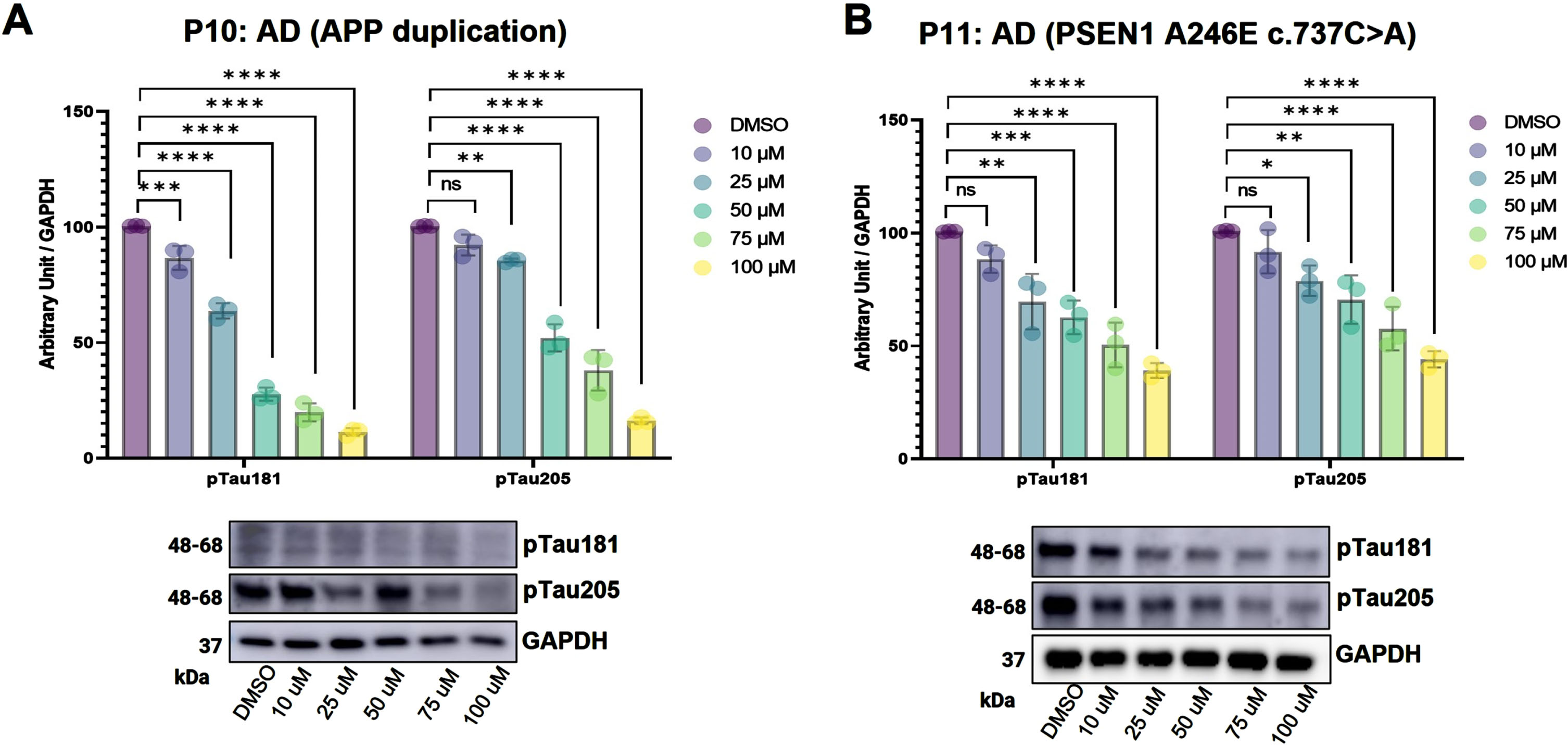
Mechanistic insights of sildenafil’s protective effects in AD patient iPSC-derived neurons
We performed transcriptome sequencing of sildenafil-treated FAD patient (P1) iPSC-derived neurons to further explore potential therapeutic effects and gain mechanistic insights for sildenafil. We identified 817 DEGs between DMSO and 100μM sildenafil treated group. Among these, 559 genes were downregulated, and 258 genes were upregulated (Supplementary Table 4). Differential expression is depicted through volcano plot highlighting some of the AD-associated genes among all (Fig. 4A). Further, functional annotations and enrichment analysis highlighted the significantly affected pathways (Fig. 4B) and biological processes related to AD and other neurodegenerative disorders. KEGG enrichment analysis identify multiple disease-associated genes-pathways relationships mainly protein digestion and absorption (1.24E– 06), Axon guidance (1.25E– 05), vascular smooth muscle contraction (VSMC) (2.75E– 05), and the cyclic guanosine monophosphate (cGMP)-protein kinase G (PKG) signaling pathway (0.000118271) (Supplementary Figure 1). We identified various dysregulated key target families viz. EPHA, EPHB, SLIT, WNT, SEMA, COL, SLC, MYLK, TRP, ADRA, PLA2, NPR, BMPR, PDE5A, FYN, CREB, PLXN, etc. associated with these pathways (Supplementary Table 5). These are mainly involved in AD pathogenesis, neurogenesis, neuroinflammation, signaling, synaptic dysfunction and plasticity [5, 42– 45]. Likewise, VSMC pathway genes are known to contribute to AD neurodegeneration, neuroinflammation, and tau phosphorylation [46, 47]. Significant KEGG pathways were depicted using Pathview tool with highlighted sildenafil regulated key genes (Supplementary Figures 2–4). These genes are also involved in multiple other pathways and cellular mechanisms.
Fig. 4
Transcriptome data analysis, regulation, and functional enrichments. Volcano plot depicting differentially expressed genes (DEGs) between DMSO and 100μM sildenafil treated group (A), Genes-pathways relationship network using Cytoscape. Edge width represent the enrichment FDR (false discovery rate) in the network (B). PANTHER pathways are shown using Octagons and KEGG pathways are marked through diamonds. NS, not significant; FC, fold change; cGMP-PKG, cyclic guanosine monophosphate-protein kinase G.
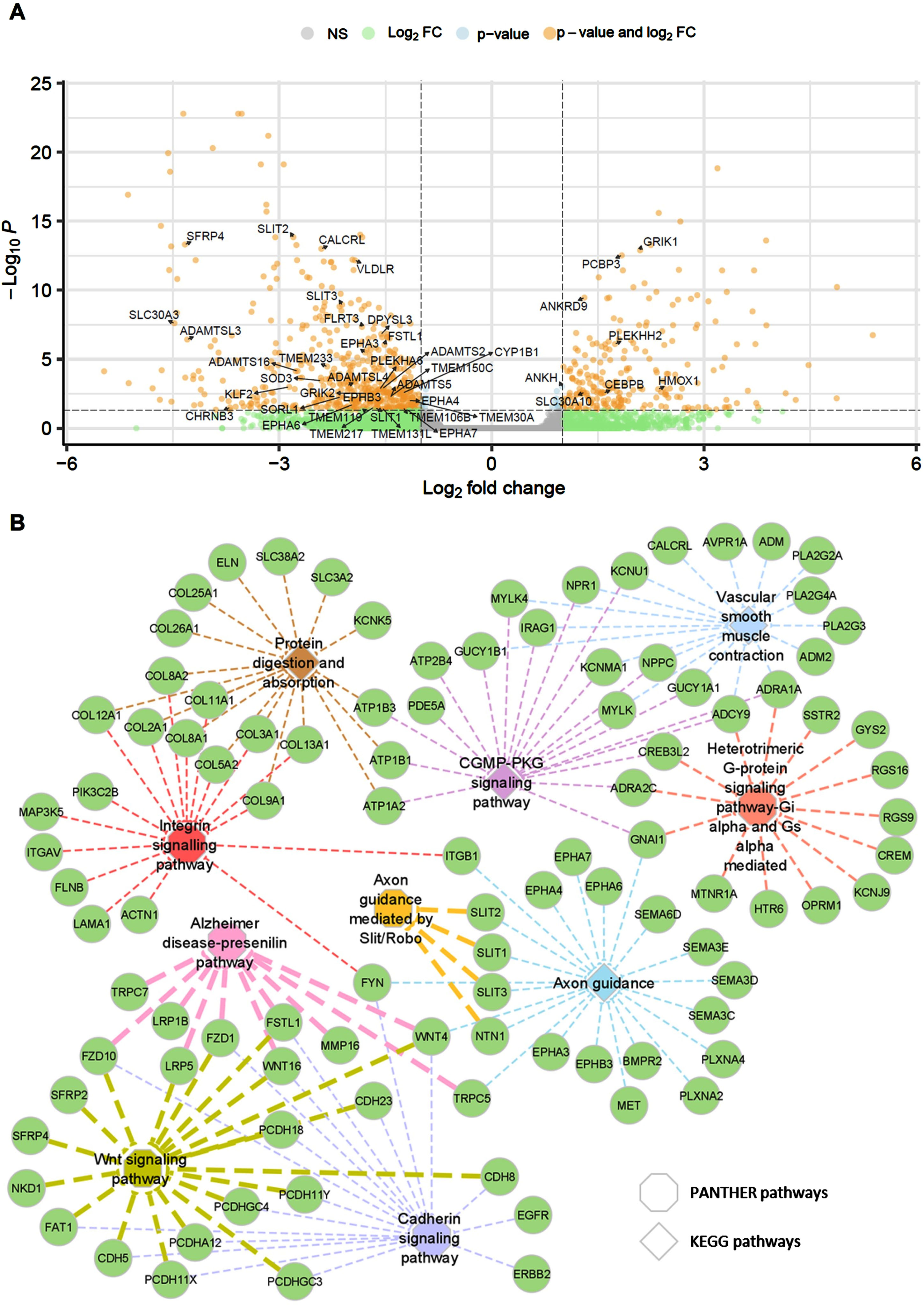
We have performed enrichment analysis using PANTHER database (Supplementary Table 6). This suggests six significantly enriched PANTHER pathways, i.e., Cadherin signaling pathway (0.000105902), Integrin signaling pathway (0.000703446), Heterotrimeric G-protein signaling pathway (0.007644778), Axon guidance mediated by Slit/Robo (0.038120988), Wnt signaling pathway (0.038120988), and Alzheimer disease-presenilin pathway (0.04450996) as also depicted in gene-pathway network mapping (Fig. 4B). These pathways are related to the AD pathobiology and genes. We have identified aberrant expression of cadherin signaling genes (FYN, ERBB2, EGFR) and Wnt signaling genes (LRP5, SFRP, NKD1) and shared common gene families mainly PCDH, WNT, CDH, FZD, FSTL1, FAT1, etc. Fyn is reported as a key regulator in AD pathology. It has a critical role in tau aggregation [48]. Cadherin regulates complex signaling cascades including amyloid-β protein precursor (APP), vascular endothelial growth factor (VEGF), morphogenesis, plasticity, Wnt-mediated signaling, etc., in neurodegenerative disorders [49]. These have important role for synaptic loss and decline in synaptic plasticity [50]. Likewise, various integrins signaling pathway genes and gene families (collagens (COL), integrins (ITG), FYN, PIK3C2B, MAP3K5, FLNB, ACTN1, LAMA1) were found to be dysregulated after sildenafil treatment. Integrins are critical signaling receptors, which mediate cell-cell communication and contribute to cognitive functions particularly in synaptic transmission [51]. This signaling pathway is known to have pathophysiological role in neurodevelopment, plasticity, and central nervous system (CNS) diseases [51– 53]. Also, integrin signaling is known to mediate Aβ-induced neurotoxicity [54]. Furthermore, we detected changes in important genes associated with the axon guidance pathway after treatment. The gene families EPHA, EPHB, SLIT, SEMA, PLXN, and MTN1 are highly dysregulated. Among these, semaphorins (SEMA) and its receptors plexins (PLXN) are the key players in the CNS. They contribute to neurodevelopment, neurogenesis, gliogenesis, neuroinflammation, neural cell migration, etc. [55, 56]. Likewise, ephrins (EPHs) and their receptor family are crucial members from receptor tyrosine kinases involved in signaling, synaptic dysregulation, neurophysiology, and neuronal plasticity [57, 58]. Finally, we also observed regulation of the AD-presenilin pathway. Key pathway genes, i.e., TRP, LRP, FZD1, MMP16, and FSTL1, are downregulated and WNT, FZD10 found upregulated. Presenilin 1 (PSEN1) and PSEN2 are key players in early-onset AD and regulate Notch/Wnt signaling, cell adhesion, and neuronal development [59].
We found enriched regulation of relevant biological processes (Supplementary Figure 1B) namely neurogenesis, generation of neurons, ion transport, adhesion, etc. (Supplementary Table 7) and molecular functions (Supplementary Figure 1C), i.e., binding (growth-factor, calcium-ion, integrin, signaling-receptor etc.) and transmembrane transporter activities (Supplementary Table 8). Overall, there is a strong crosstalk among enriched AD-related pathways and in biological processes affected by sildenafil treatment as they share multiple common differentially regulated targets. These findings support the critical role of sildenafil as a potential drug-repurposing candidate for AD. Additional functional or biomarker studies are warranted to establish more in-depth understanding of the influence of sildenafil on AD pathogenesis.
DISCUSSION
Sildenafil is a selective PDE5 inhibitor and PDEs are broadly expressed in human brain [60]. Recent studies have shown that sildenafil has beneficial effects in various preclinical models of AD by modulating neuronal plasticity, reducing tau phosphorylation, improving cognitive impairment, decreasing Aβ plaque accumulation, and enhancing the level of BDNF [11, 12, 61]. Our previous study found that sildenafil increased neurite growth and decreased phospho-tau (pTau181) in AD patient iPSC-derived neurons, mechanistically supporting its potential beneficial effect in AD [13]. In vivo studies from other groups have also shown that sildenafil improves memory, amyloid plaque load, inflammation, and neurogenesis in APP/PS1 mice. Sildenafil was shown to improve memory, and reduced tau hyperphosphorylation and GSK3β phosphorylation in J20 mice [62]. Furthermore, two pilot trials demonstrated beneficial effects of sildenafil in treatment of AD, where a single dosage of 50 mg sildenafil reduced spontaneous neural activity in the right hippocampus in 10 patients [63]; and increased the cerebral metabolic rate of oxygen and cerebral blood flow in 12 patients; and decreased cerebral vascular reactivity in 8 patients [64].
To further test association of AD incidence and Sildenafil usage, our new population-based cohort study identified a decreased risk of incident AD in users of sildenafil versus users of comparator drugs including nifedipine, bumetanide, furosemide, and spironolactone, in two independent patient databases of the MarketScan Medicare Supplemental and OPTUM (Fig. 1). These results were consistent across studies under different designs and/or using different comparator drug cohorts, as well as across subgroup analyses. Recent observations involving data from over 30 million insured members validates the association between sildenafil use and reduced risk of AD [65]. This study showed that sildenafil could reduce the risk of AD by 60% which in consonant with our findings. We further observed improved molecular phenotypes relevant to AD neuropathology following sildenafil treatment in matured neurons derived from four AD patient iPSC lines.
Our findings are at odds with a recent study which did not find evidence for a difference in the risk of incident ADRD in patients treated with PDE5 inhibitors (sildenafil/tadalafil) versus endothelin receptor antagonists [35]. Differences in the design of these two investigations may explain the inconsistency. The first explanation may be that overall dosage of sildenafil is much lower in PH patients (Revatio, less than 20 mg daily) tested in Desai et al. [35] compared to individuals with erectile dysfunction (Viagra, 25 mg– 100 mg) tested in other studies [13, 65]. Another possible reason may be caused by under power issue. Desai et al. focused on PH, a rare disease with overall low survival rate, using an active comparator design and each drug arm only included 1,000 drug users, which is much less than previous studies [13, 65]. Because of lack of right active comparator drugs for sildenafil in erectile dysfunction individuals, further real-world patient data validation using likely causal inference approaches [66] and clinical trials are highly warranted in the future.
The study by Desai et al. performed phenotypic assays using primary mouse hippocampal and cortical neurons, as well as immortalized microglia-like cells, neuroblastoma cells, and neuroglioma cells in assays to assess the effects of sildenafil on several distinct AD related phenotypes. They did not report significant mechanistic effects of sildenafil on most AD relevant outcomes, although sildenafil treatment was associated with a reduction in secretion of several pro-inflammatory cytokines (i.e., IL-12p70 and IL-1β) in microglial cells [35]. This might be due to low dose range (0.1– 10μM) for 24 h used for the sildenafil treatment in the study compared to the dose range (10– 100μM) for 5 days that we used here based on previous studies [13, 67]. Furthermore, we used four complementary AD patient iPSC derived neurons which include both familial and sporadic AD patients and reflects discrete aspect of AD pathology. We found consistent dose dependent effect of sildenafil on reducing tau phosphorylation. However, we haven’t used microglia or astrocytes derived from patient iPSCs to evaluate the effect of sildenafil on neuroinflammation and other AD associated phenotypes.
Transcriptome sequencing of sildenafil-treated iPSC-derived neurons provide further mechanistic insights into potential effects of sildenafil. We showed biological processes were significantly dysregulated in the sildenafil treated group. We identified multiple key gene families, which are known to play pivotal roles in neurodegeneration, neuroinflammation, synaptic dysfunction, axon guidance, and other signaling (Cadherin, Integrin, Wnt) pathways (Fig. 4). Transcriptional regulations and functional enrichment analysis show possible role of sildenafil towards beneficial outcome in AD. We have identified differential regulation of key known potential AD therapeutic targets. Like, negative regulation of phosphodiesterase 5A (PDE5A), a major player in cGMP-PKG signaling pathway (Supplementary Figure 4). PDE5 inhibition leads to the improved cognitive functions and synaptic plasticity, in turn benefit in AD and that effectively control the level of phosphorylated Tau (pTau). Concurrently, we also identified significant down regulation of other important genes, such as CREB3L2, MYLK, MYLK4, NPPC, KCNMA1, NPR1, and GUCY1A1 from cGMP-PKG signaling pathway (Supplementary Figure 4). Recently, CREB3L2-ATF4, a pathological transcription factor heterodimers stated in activating AD transcriptional network and tau hyperphosphorylation [68]. Interestingly, we also found substantial impact of sildenafil on axon guidance pathway genes (Supplementary Figure 2). Erythropoietin-producing hepatocellular (Eph) tyrosine kinase receptors are key members in axon guidance pathway. We have identified deregulation of EPHA4, EPHA3, EPHA6, EPHA7, and EPHB3 after sildenafil treatment. Studies suggests EPHA4 promotes AD progression through synaptic dysregulation. It is evident that inhibiting EPHA4 could be protective in AD. Sildenafil also downregulate the semaphorins (SEMA3C, SEMA6D) and its receptors plexins (PLXNA2, PLXNA4). Another acting mechanism of sildenafil could be impacting the AD-presenilin pathway as PSEN1 and PSEN2 are critical factor in early-onset AD. It negatively regulates the key components of this pathway, i.e., TRPC5, TRPC7, LRP1B, LRP5, FZD1, MMP16, and FSTL1. These results suggest the likely mechanistic roles of sildenafil as a potential repurposing candidate for AD subjected to the further validations.
Our population-based study has limitations. First, the PS-based analysis accounts only for common confounders in older adults and/or related to AD. Our results are subject to unmeasured confounding including genetic risk factors, socioeconomic status, blood pressure records, etc. Second, the analytical datasets are derived from claims data. Although we use validated and specific methods, the variables could be misclassified. We therefore performed cross data source validation and comprehensive subgroup analyses across different exposure groups. We showed sildenafil exposure for individuals with hypertension (Fig. 1), which suggests that disease comorbidities or comparator drug’s indication bias are likely not confounders.
In summary, by combining AD patient iPSC-derived neuron models and real-world patient data observation, we suggested that sildenafil can be a potential repurposable drug for the treatment of AD and warrants further testing in more functional models and randomized controlled clinical trials.
AUTHOR CONTRIBUTIONS
Feixiong Cheng (Conceptualization; Data curation; Funding acquisition; Investigation; Project administration; Resources; Software; Supervision; Validation; Writing – original draft; Writing – review & editing); Dhruv Gohel (Data curation; Formal analysis; Investigation; Methodology; Validation; Writing – original draft; Writing – review & editing); Pengyue Zhang (Data curation; Formal analysis; Investigation; Methodology; Writing – original draft; Writing – review & editing); Amit Gupta (Data curation; Formal analysis; Investigation; Methodology; Writing – original draft); Yichen Li (Data curation; Formal analysis); Chien-Wei Chiang (Formal analysis; Investigation; Methodology); Lang Li (Funding acquisition; Supervision); Yuan Hou (Data curation; Formal analysis); Andrew A. Pieper (Formal analysis; Funding acquisition; Writing – review & editing); Jeffrey Cummings (Funding acquisition; Resources; Supervision; Writing – review & editing).
ACKNOWLEDGMENTS
The iPSC samples from the National Centralized Repository for Alzheimer’s Disease and Related Dementias (NCRAD), which receives government support under a cooperative agreement grant (U24 AG21886) awarded by the National Institute on Aging (NIA), were used in this study. Samples and associated data are contributed by the National Institute on Aging (NIA) grant: 1 RF1AG048083-01 (PI Lawrence Goldstein, PhD) and R56 AG057478 (PI Suman Jayadev, MD). We thank contributors who collected samples used in this study, as well as patients and their families, whose help and participation made this work possible.
FUNDING
This work was primarily supported by the National Institute of Aging (NIA) under Award Number R01AG066707, U01AG073323, R01AG076448, R01AG082118, RF1AG082211, R01AG084250, R56AG074001, and R21AG083003, and the National Institute of Neurological Disorders and Stroke (NINDS) under Award Number RF1NS133812 to F.C. This work was partly supported by the Alzheimer’s Association award (ALZDISCOVERY-1051936) to F.C. This work was supported in part by the Translational Therapeutics Core of the Cleveland Alzheimer’s Disease Research Center (NIH/NIA: P30AG072959) to F.C., A.A.P., and J.C. A.A.P. was also supported by The Valour Foundation, and by the Rebecca E. Barchas MD Chair in Translational Psychiatry of Case Western Reserve University and the Morley-Mather Chair in Neuropsychiatry of University Hospitals of Cleveland Medical Center, as well as the American Heart Association and Paul Allen Foundation Initiative in Brain Health and Cognitive Impairment (19PABH134580006), Department of Veterans Affairs Merit Award I01BX005976, and the Louis Stokes VA Medical Center resources and facilities. This work was supported in part by Keep Memory Alive (KMA), NIGMS grant P20GM109025, NINDS grant U01NS093334, NIA grant R01AG053798 and R35AG071476, and the Alzheimer’s Disease Drug Discovery Foundation (ADDF) to J.C.
CONFLICT OF INTEREST
Dr. Cummings has provided consultation to AB Science, Acadia, Alkahest, AlphaCognition, ALZPathFinder, Annovis, AriBio, Artery, Avanir, Biogen, Biosplice, Cassava, Cerevel, Clinilabs, Cortexyme, Diadem, EIP Pharma, Eisai, GatehouseBio, GemVax, Genentech, Green Valley, Grifols, Janssen, Karuna, Lexeo, Lilly, Lundbeck, LSP, Merck, NervGen, Novo Nordisk, Oligomerix, Ono, Otsuka, PharmacotrophiX, PRODEO, Prothena, ReMYND, Renew, Resverlogix, Roche, Signant Health, Suven, Unlearn AI, Vaxxinity, VigilNeuro pharmaceutical, assessment, and investment companies. The other authors have declared no competing interests.
DATA AVAILABILITY
The data supporting the findings of this study are available within the article and its supplementary material. The RNA-sequencing data is available at the GEO database (GEO ID: GSE254742).
SUPPLEMENTARY MATERIAL
[1] The supplementary material is available in the electronic version of this article: https://dx.doi.org/10.3233/JAD-231391.
REFERENCES
[1] | DeTure MA , Dickson DW ((2019) ) The neuropathological diagnosis of Alzheimer’s disease. Mol Neurodegener 14: , 32. |
[2] | ((2023) ) 2023 Alzheimer’s disease facts and figures. Alzheimers Dement 19: , 1598–1695. |
[3] | Ryan J , Fransquet P , Wrigglesworth J , Lacaze P ((2018) ) Phenotypic heterogeneity in dementia: A challenge for epidemiology and biomarker studies. Front Public Health 6: , 181. |
[4] | Rajmohan R , Reddy PH ((2017) ) Amyloid-beta and phosphorylated tau accumulations cause abnormalities at synapses of Alzheimer’s disease neurons. J Alzheimers Dis 57: , 975–999. |
[5] | Hampel H , Hardy J , Blennow K , Chen C , Perry G , Kim SH , Villemagne VL , Aisen P , Vendruscolo M , Iwatsubo T , Masters CL , Cho M , Lannfelt L , Cummings JL , Vergallo A ((2021) ) The amyloid-β pathway in Alzheimer’s disease. Mol Psychiatry 26: , 5481–5503. |
[6] | Silva MVF , Loures CMG , Alves LCV , de Souza LC , Borges KBG , Carvalho MDG ((2019) ) Alzheimer’s disease: Risk factors and potentially protective measures. J Biomed Sci 26: , 33. |
[7] | Yiannopoulou KG , Papageorgiou SG ((2013) ) Current and future treatments for Alzheimer’s disease. Ther Adv Neurol Disord 6: , 19–33. |
[8] | Cummings J ((2021) ) New approaches to symptomatic treatments for Alzheimer’s disease. Mol Neurodegener 16: , 2. |
[9] | Shi M , Chu F , Zhu F , Zhu J ((2022) ) Impact of anti-amyloid-β monoclonal antibodies on the pathology and clinical profile of Alzheimer’s disease: A focus on aducanumab and lecanemab. Front Aging Neurosci 14: , 870517. |
[10] | Söderberg L , Johannesson M , Nygren P , Laudon H , Eriksson F , Osswald G , Möller C , Lannfelt L ((2023) ) Lecanemab, aducanumab, and gantenerumab – binding profiles to different forms of amyloid-beta might explain efficacy and side effects in clinical trials for Alzheimer’s disease. Neurotherapeutics 20: , 195–206. |
[11] | Cuadrado-Tejedor M , Hervias I , Ricobaraza A , Puerta E , Pérez-Roldán JM , García-Barroso C , Franco R , Aguirre N , García-Osta A ((2011) ) Sildenafil restores cognitive function without affecting β-amyloid burden in a mouse model of Alzheimer’s disease. Br J Pharmacol 164: , 2029–2041. |
[12] | Sanders O ((2020) ) Sildenafil for the treatment of Alzheimer’s disease: A systematic review. J Alzheimers Dis Rep 4: , 91–106. |
[13] | Fang J , Zhang P , Zhou Y , Chiang CW , Tan J , Hou Y , Stauffer S , Li L , Pieper AA , Cummings J , Cheng F ((2021) ) Endophenotype-based in silico network medicine discovery combined with insurance record data mining identifies sildenafil as a candidate drug for Alzheimer’s disease. Nat Aging 1: , 1175–1188. |
[14] | Shi Y , Inoue H , Wu JC , Yamanaka S ((2017) ) Induced pluripotent stem cell technology: A decade of progress. Nat Rev Drug Discov 16: , 115–130. |
[15] | Pandey S , Jirásko M , Lochman J , Chvátal A , Chottova Dvorakova M , Kučera R ((2022) ) iPSCs in neurodegenerative disorders: A unique platform for clinical research and personalized medicine. J Pers Med 12: , 1485. |
[16] | Sitbon O , Humbert M , Jais X , Ioos V , Hamid AM , Provencher S , Garcia G , Parent F , Herve P , Simonneau G ((2005) ) Long-term response to calcium channel blockers in idiopathic pulmonary arterial hypertension. Circulation 111: , 3105–3111. |
[17] | Medarov BI , Judson MA ((2015) ) The role of calcium channel blockers for the treatment of pulmonary arterial hypertension: How much do we actually know and how could they be positioned today? . Respir Med 109: , 557–564. |
[18] | Galie N , Humbert M , Vachiery JL , Gibbs S , Lang I , Torbicki A , Simonneau G , Peacock A , Vonk Noordegraaf A , Beghetti M , Ghofrani A , Gomez Sanchez MA , Hansmann G , Klepetko W , Lancellotti P , Matucci M , McDonagh T , Pierard LA , Trindade PT , Zompatori M , Hoeper M , ESC Scientific Document Group ((2016) ) 2015 ESC/ERS Guidelines for the diagnosis and treatment of pulmonary hypertension: The Joint Task Force for the Diagnosis and Treatment of Pulmonary Hypertension of the European Society of Cardiology (ESC) and the European Respiratory Society (ERS): Endorsed by: Association for European Paediatric and Congenital Cardiology (AEPC), International Society for Heart and Lung Transplantation (ISHLT). Eur Heart J 37: , 67–119. |
[19] | Ponjoan A , Garre-Olmo J , Blanch J , Fages E , Alves-Cabratosa L , Martí-Lluch R , Comas-Cufí M , Parramon D , García-Gil M , Ramos R ((2019) ) How well can electronic health records from primary care identify Alzheimer’s disease cases? . Clin Epidemiol 11: , 509–518. |
[20] | Yang W , Hamilton JL , Kopil C , Beck JC , Tanner CM , Albin RL , Ray Dorsey E , Dahodwala N , Cintina I , Hogan P , Thompson T ((2020) ) Current and projected future economic burden of Parkinson’s disease in the U.S. NPJ Parkinsons Dis 6: , 15. |
[21] | Xu J , Zhang P , Huang Y , Zhou Y , Hou Y , Bekris LM , Lathia J , Chiang CW , Li L , Pieper AA , Leverenz JB , Cummings J , Cheng F ((2021) ) Multimodal single-cell/nucleus RNA sequencing data analysis uncovers molecular networks between disease-associated microglia and astrocytes with implications for drug repurposing in Alzheimer’s disease. Genome Res 31: , 1900–1912. |
[22] | Quan H , Li B , Couris CM , Fushimi K , Graham P , Hider P , Januel JM , Sundararajan V ((2011) ) Updating and validating the Charlson comorbidity index and score for risk adjustment in hospital discharge abstracts using data from 6 countries. Am J Epidemiol 173: , 676–682. |
[23] | Nordström A , Nordström P ((2018) ) Traumatic brain injury and the risk of dementia diagnosis: A nationwide cohort study. PLoS Med 15: , e1002496. |
[24] | Chen S , Zhou Y , Chen Y , Gu J ((2018) ) fastp: An ultra-fast all-in-one FASTQ preprocessor. , . Bioinformatics 34: , i884–i890. |
[25] | Liao Y , Smyth GK , Shi W ((2019) ) The R package Rsubread is easier, faster, cheaper and better for alignment and quantification of RNA sequencing reads. Nucleic Acids Res 47: , e47. |
[26] | Liao Y , Smyth GK , Shi W ((2014) ) featureCounts: An efficient general purpose program for assigning sequence reads to genomic features. Bioinformatics 30: , 923–930. |
[27] | Robinson MD , McCarthy DJ , Smyth GK ((2010) ) edgeR: A Bioconductor package for differential expression analysis of digital gene expression data. Bioinformatics 26: , 139–140. |
[28] | Ritchie ME , Phipson B , Wu D , Hu Y , Law CW , Shi W , Smyth GK ((2015) ) limma powers differential expression analyses for RNA-sequencing and microarray studies. Nucleic Acids Res 43: , e47. |
[29] | Law CW , Chen Y , Shi W , Smyth GK ((2014) ) voom: Precision weights unlock linear model analysis tools for RNA-seq read counts. Genome Biol 15: , R29. |
[30] | Ge SX , Jung D , Yao R ((2020) ) ShinyGO: A graphical gene-set enrichment tool for animals and plants. Bioinformatics 36: , 2628–2629. |
[31] | Kanehisa M , Furumichi M , Sato Y , Kawashima M , Ishiguro-Watanabe M ((2023) ) KEGG for taxonomy-based analysis of pathways and genomes. Nucleic Acids Res 51: , D587–D592. |
[32] | Mi H , Muruganujan A , Casagrande JT , Thomas PD ((2013) ) Large-scale gene function analysis with the PANTHER classification system. Nat Protoc 8: , 1551–1566. |
[33] | Luo W , Brouwer C ((2013) ) Pathview: An R/Bioconductor package for pathway-based data integration and visualization. Bioinformatics 29: , 1830–1831. |
[34] | Shannon P , Markiel A , Ozier O , Baliga NS , Wang JT , Ramage D , Amin N , Schwikowski B , Ideker T ((2003) ) Cytoscape: A software environment for integrated models of biomolecular interaction networks. Genome Res 13: , 2498–2504. |
[35] | Desai RJ , Mahesri M , Lee SB , Varma VR , Loeffler T , Schilcher I , Gerhard T , Segal JB , Ritchey ME , Horton DB , Kim SC , Schneeweiss S , Thambisetty M ((2022) ) No association between initiation of phosphodiesterase-5 inhibitors and risk of incident Alzheimer’s disease and related dementia: Results from the Drug Repurposing for Effective Alzheimer’s Medicines study. , fcac. Brain Commun 4: , 247. |
[36] | Taubes A , Nova P , Zalocusky KA , Kosti I , Bicak M , Zilberter MY , Hao Y , Yoon SY , Oskotsky T , Pineda S , Chen B , Aery Jones EA , Choudhary K , Grone B , Balestra ME , Chaudhry F , Paranjpe I , De Freitas J , Koutsodendris N , Chen N , Wang C , Chang W , An A , Glicksberg BS , Sirota M , Huang Y ((2021) ) Author Correction: Experimental and real-world evidence supporting the computational repurposing of bumetanide for APOE4-related Alzheimer’s disease. Nat Aging 1: , 1202. |
[37] | Davis RL ((2020) ) Mechanism of action and target identification: A matter of timing in drug discovery. iScience 23: , 101487. |
[38] | Ebert AD , Liang P , Wu JC ((2012) ) Induced pluripotent stem cells as a disease modeling and drug screening platform. J Cardiovasc Pharmacol 60: , 408–416. |
[39] | Sharma A , Sances S , Workman MJ , Svendsen CN ((2020) ) Multi-lineage human iPSC-derived platforms for disease modeling and drug discovery. Cell Stem Cell 26: , 309–329. |
[40] | Mielke MM , Hagen CE , Xu J , Chai X , Vemuri P , Lowe VJ , Airey DC , Knopman DS , Roberts RO , Machulda MM , Jack CR Jr. , Petersen RC , Dage JL ((2018) ) Plasma phospho-tau181 increases with Alzheimer’s disease clinical severity and is associated with tau- and amyloid-positron emission tomography. Alzheimers Dement 14: , 989–997. |
[41] | Barthélemy NR , Saef B , Li Y , Gordon BA , He Y , Horie K , Stomrud E , Salvadó G , Janelidze S , Sato C , Ovod V , Henson RL , Fagan AM , Benzinger TLS , Xiong C , Morris JC , Hansson O , Bateman RJ , Schindler SE ((2023) ) CSF tau phosphorylation occupancies at T217 and T205 represent improved biomarkers of amyloid and tau pathology in Alzheimer’s disease. Nat Aging 3: , 391–401. |
[42] | Gadhave K , Kumar D , Uversky VN , Giri R ((2021) ) A multitude of signaling pathways associated with Alzheimer’s disease and their roles in AD pathogenesis and therapy. Med Res Rev 41: , 2689–2745. |
[43] | Calabrò M , Rinaldi C , Santoro G , Crisafulli C ((2021) ) The biological pathways of Alzheimer disease: A review. AIMS Neurosci 8: , 86–132. |
[44] | Sung PS , Lin PY , Liu CH , Su HC , Tsai KJ ((2020) ) Neuroinflammation and neurogenesis in Alzheimer’s disease and potential therapeutic approaches. Int J Mol Sci 21: , 701. |
[45] | Fu WY , Ip NY ((2023) ) The role of genetic risk factors of Alzheimer’s disease in synaptic dysfunction. Semin Cell Dev Biol 139: , 3–12. |
[46] | Aguilar-Pineda JA , Vera-Lopez KJ , Shrivastava P , Chávez-Fumagalli MA , Nieto-Montesinos R , Alvarez-Fernandez KL , Goyzueta Mamani LD , Davila Del-Carpio G , Gomez-Valdez B , Miller CL , Malhotra R , Lindsay ME , Lino Cardenas CL ((2021) ) Vascular smooth muscle cell dysfunction contribute to neuroinflammation and Tau hyperphosphorylation in Alzheimer disease. iScience 24: , 102993. |
[47] | Hayes G , Pinto J , Sparks SN , Wang C , Suri S , Bulte DP ((2022) ) Vascular smooth muscle cell dysfunction in neurodegeneration. Front Neurosci 16: , 1010164. |
[48] | Briner A , Götz J , Polanco JC ((2020) ) Fyn kinase controls tau aggregation. Cell Rep 32: , 108045. |
[49] | Punovuori K , Malaguti M , Lowell S ((2021) ) Cadherins in early neural development. Cell Mol Life Sci 78: , 4435–4450. |
[50] | Tzioras M , McGeachan RI , Durrant CS , Spires-Jones TL ((2023) ) Synaptic degeneration in Alzheimer disease. Nat Rev Neurol 19: , 19–38. |
[51] | Biose IJ , Ismael S , Ouvrier B , White AL , Bix GJ ((2023) ) The potential role of integrin signaling in memory and cognitive impairment. Biomolecules 13: , 108. |
[52] | Pang X , He X , Qiu Z , Zhang H , Xie R , Liu Z , Gu Y , Zhao N , Xiang Q , Cui Y ((2023) ) Targeting integrin pathways: Mechanisms and advances in therapy. Signal Transduct Target Ther 8: , 1. |
[53] | Wu X , Reddy DS ((2012) ) Integrins as receptor targets for neurological disorders. Pharmacol Ther 134: , 68–81. |
[54] | Wright S , Malinin NL , Powell KA , Yednock T , Rydel RE , Griswold-Prenner I ((2007) ) Alpha2beta1 and alphaVbeta1 integrin signaling pathways mediate amyloid-beta-induced neurotoxicity. Neurobiol Aging 28: , 226–237. |
[55] | Limoni G , Niquille M ((2021) ) Semaphorins and Plexins in central nervous system patterning: The key to it all? . Curr Opin Neurobiol 66: , 224–232. |
[56] | Xuan FL , Yan L , Li Y , Fan F , Deng H , Gou M , Chithanathan K , Heinla I , Yuan L , Seppa K , Zharkovsky A , Kalda A , Hong LE , Hu GF , Tan Y , Tian L ((2022) ) Glial receptor PLXNB2 regulates schizophrenia-related stress perception via the amygdala. Front Immunol 13: , 1005067. |
[57] | Kania A , Klein R ((2016) ) Mechanisms of ephrin-Eph signalling in development, physiology and disease. Nat Rev Mol Cell Biol 17: , 240–256. |
[58] | Buhl E , Kim YA , Parsons T , Zhu B , Santa-Maria I , Lefort R , Hodge JJL ((2022) ) Effects of Eph/ephrin signalling and human Alzheimer’s disease-associated EphA1 on Drosophila behaviour and neurophysiology. Neurobiol Dis 170: , 105752. |
[59] | Van Cauwenberghe C , Van Broeckhoven C , Sleegers K ((2016) ) The genetic landscape of Alzheimer disease: Clinical implications and perspectives. Genet Med 18: , 421–430. |
[60] | Teich AF , Sakurai M , Patel M , Holman C , Saeed F , Fiorito J , Arancio O ((2016) ) PDE5 exists in human neurons and is a viable therapeutic target for neurologic disease. J Alzheimers Dis 52: , 295–302. |
[61] | Orejana L , Barros-Miñones L , Jordán J , Puerta E , Aguirre N ((2012) ) Sildenafil ameliorates cognitive deficits and tau pathology in a senescence-accelerated mouse model. Neurobiol Aging 33: , 625.e611-620. |
[62] | García-Barroso C , Ricobaraza A , Pascual-Lucas M , Unceta N , Rico AJ , Goicolea MA , Sallés J , Lanciego JL , Oyarzabal J , Franco R , Cuadrado-Tejedor M , García-Osta A ((2013) ) Tadalafil crosses the blood-brain barrier and reverses cognitive dysfunction in a mouse model of AD. Neuropharmacology 64: , 114–123. |
[63] | Samudra N , Motes M , Lu H , Sheng M , Diaz-Arrastia R , Devous M , Hart J , Womack KB ((2019) ) A pilot study of changes in medial temporal lobe fractional amplitude of low frequency fluctuations after sildenafil administration in patients with Alzheimer’s disease. J Alzheimers Dis 70: , 163–170. |
[64] | Sheng M , Lu H , Liu P , Li Y , Ravi H , Peng SL , Diaz-Arrastia R , Devous MD , Womack KB ((2017) ) Sildenafil improves vascular and metabolic function in patients with Alzheimer’s disease. J Alzheimers Dis 60: , 1351–1364. |
[65] | Huo X , Finkelstein J ((2023) ) Using big data to uncover association between sildenafil use and reduced risk of Alzheimer’s disease. Stud Health Technol Inform 302: , 866–870. |
[66] | Charpignon ML , Vakulenko-Lagun B , Zheng B , Magdamo C , Su B , Evans K , Rodriguez S , Sokolov A , Boswell S , Sheu YH , Somai M , Middleton L , Hyman BT , Betensky RA , Finkelstein SN , Welsch RE , Tzoulaki I , Blacker D , Das S , Albers MW ((2022) ) Causal inference in medical records and complementary systems pharmacology for metformin drug repurposing towards dementia. Nat Commun 13: , 7652. |
[67] | Son Y , Kim K , Cho HR ((2018) ) Sildenafil protects neuronal cells from mitochondrial toxicity induced by beta-amyloid peptide via ATP-sensitive K(+) channels. Biochem Biophys Res Commun 500: , 504–510. |
[68] | Gouveia Roque C , Chung KM , McCurdy EP , Jagannathan R , Randolph LK , Herline-Killian K , Baleriola J , Hengst U ((2023) ) CREB3L2-ATF4 heterodimerization defines a transcriptional hub of Alzheimer’s disease gene expression linked to neuropathology. Sci Adv 9: , eadd2671. |