Communication Regarding the Myocardial Ischemia/Reperfusion and Cognitive Impairment: A Narrative Literature Review
Abstract
Coronary artery disease is a prevalent ischemic disease that results in insufficient blood supply to the heart muscle due to narrowing or occlusion of the coronary arteries. Various reperfusion strategies, including pharmacological thrombolysis and percutaneous coronary intervention, have been developed to enhance blood flow restoration. However, these interventions can lead to myocardial ischemia/reperfusion injury (MI/RI), which can cause unpredictable complications. Recent research has highlighted a compelling association between MI/RI and cognitive function, revealing pathophysiological mechanisms that may explain altered brain cognition. Manifestations in the brain following MI/RI exhibit pathological features resembling those observed in Alzheimer’s disease (AD), implying a potential link between MI/RI and the development of AD. The pro-inflammatory state following MI/RI may induce neuroinflammation via systemic inflammation, while impaired cardiac function can result in cerebral under-perfusion. This review delves into the role of extracellular vesicles in transporting deleterious substances from the heart to the brain during conditions of MI/RI, potentially contributing to impaired cognition. Addressing the cognitive consequence of MI/RI, the review also emphasizes potential neuroprotective interventions and pharmacological treatments within the MI/RI model. In conclusion, the review underscores the significant impact of MI/RI on cognitive function, summarizes potential mechanisms of cardio-cerebral communication in the context of MI/RI, and offers ideas and insights for the prevention and treatment of cognitive dysfunction following MI/RI.
INTRODUCTION
Cardiovascular disease is a significant global health concern associated with high mortality and disability rates [1]. Reperfusion strategies have been widely implemented to restore blood flow following a cardiovascular event, improving patient outcomes [2]. However, this approach can lead to myocardial ischemia/reperfusion injury (MI/RI), causing further damage to the heart tissue [3, 4]. At the same time, cognitive impairment and dementia are prevalent among cardiovascular disease patients [5, 6], particularly after cardiac surgery, with a prevalence ranging from 9% to 54% [7]. Post-operative neurocognitive deficit poses significant clinical challenges due to their association with increased mortality and limited treatment options [8]. Additionally, these deficits contribute to reduced long-term quality of life and increased economic burden [9]. Growing evidence suggests that I/R in peripheral organs, including the liver [10–12], kidneys [13, 14], intestines [15, 16], and limbs [17], is a risk factor for cognitive impairment. Cardiac I/R has been specifically identified as an important contributor to cognitive dysfunction following cardiac surgery [18]. Animal studies have revealed that cognitive decline associated with cardiac I/R is linked to various brain pathological manifestations, such as blood-brain barrier (BBB) breakdown, increased oxidative stress and neuroinflammation, mitochondrial dysfunction, amyloid-β (Aβ) accumulation, neuronal apoptosis, and dendritic spine loss [19].
Considering the distinct relationship between the heart and the brain, it is intriguing how the brain undergoes pathological changes in response to MI/RI. This review aims to compile and discuss potential mechanisms underlying cognitive impairment following MI/RI, as well as provide insights into treatment and protective strategies against cognitive decline in this context.
SEARCH STRATEGY
We performed a search of all literature available in PubMed and Google scholar. The literature search was performed without any language or study publication year restrictions, and the following key words were used: (“myocardial ischemia reperfusion injury” OR “MI/RI” OR “cardiac ischemia reperfusion” OR “cardiac I/R” OR “Cardiac Surgery” OR “percutaneous coronary intervention” OR “PCI” OR “percutaneous coronary transluminal angioplasty” OR “coronary artery bypass grafting” OR “CABG” OR “coronary artery revascularization”) AND (“cognition” OR “cognitive dysfunction” OR “cognitive decline” OR “cognitive impairment” OR “dementia” OR “perioperative neurocognitive dysfunction” OR “PND” OR “post operative cognitive dysfunction” OR “POCD”). We evaluated the abstracts of all articles and screened them for relevance to the purpose of this review. We also searched the reference lists of the articles identified through this search strategy and selected those considered relevant. Ultimately, we screened 22 clinical articles and three preclinical articles that met our research objectives. The characteristics of these studies are shown in Tables 1 and 2.
Table 1
Study Characteristics of MI/RI-Related Postoperative Cardiac Cognitive Dysfunction
Study (year) | Patients and Sample size | Type of procedure | Exclusion criteria | Age (SD) | Adjustment for confounders | Follow-up period | Prevalence of cognitive dysfunction | Findings | Ref. |
Bergh et al. (2002) | 114 patients underwent cardiac procedures | CABG: 50% percutaneous coronary transluminal angioplasty: 50% | Participating in aconcurrent study of postoperative neuropsychologicalfunction | CABG:67.8±8.8PTCA:68.4±6.7 | Sex, age | 1 to 2 y after intervention | – | Percutaneous coronary transluminal angioplasty and CABG patients had decreased memory 1 to 2 y after treatment, and no subjective differences were found in PTCA and CABG patients | [37] |
Ahlgren et al. (2003) | 42 patients with stable angina pectoris | CABG with CPB: 55% Percutaneous coronary intervention (PCI): 45% | A history of alcohol abuse or a documented neurological or psychiatric disorder or cerebral lesion | CABG:65.7±3.3PCI: 64.4±4.5 | age, gender, education, extent of driving | 4–6 wk after intervention | CABG: 48% PCI: 10% | Cognitive functions important for safe driving may be influenced after CABG and PCI | [38] |
Währborg et al. (2004) | 135 patients for 6 mo evaluation130 patients for 12 mo evaluation | 6 m (CABG: 47%; PCI: 53%)12 mo (CABG: 49%; PCI: 51%) | – | PCI: 62±8.0CABG: 63±9.1 | – | 6 and 12 mo after procedure | – | No significant differences in neuropsychological outcomes could be demonstrated in patients treated with different revascularization strategies | [39] |
Keizer et al. (2005) | 281 CABG patients112 healthy volunteers | CABG: 100% | – | Control: 60.5±6.2CABG:61.3±9 | – | 3 and 12 mo after baseline | 3 mo (control: 4.6%; CABG: 7.7%)12 mo: (control:4.1%; CABG:12.3) | These data suggest that the incidence of cognitive dysfunction after CABG has previously been greatly overestimated | [40] |
Vedin et al. (2006) | 70 patients with stable angina pectoris, and lack of tight main stem stenosis | off-pump CABG: 47.1% on-pump CABG: 52.9% | Age under 50 or over 80 y, ejection fraction < < 30%, serum creatinine > 150μmol/l, tight main stem stenosis (>70%), redo operation, diffuse distal coronary artery disease and unstable angina | Off-pump CABG: 65On-pump CABG: 65 | – | 1 wk, 1 and 6 mo after surgery | 1 wk (on-pump: 57%; off-pump: 58%)1 mo (on-pump: 30%; off-pump: 12%)6 mo (on-pump: 19%; off-pump: 15%) | This study showed no differences in post-operative cognitive function after on pump compared to off pump CABG in low-risk patients | [41] |
Dieleman et al. (2009) | 281 patients undergoing first-time CABG | patients were randomly assigned to on-pump or off-pump CABG | – | 61.3±9 | Type of surgery (i.e., on-pump or off-pump treatment) | 3 and 12 mo and 5 y after surgery | 3 mo: 8% 12 mo: 12% 5 y: 34% | Presence of coronary collaterals is associated with a decreased risk of cognitive decline at both 3 and 12 mo, this trend persists at 5-y follow-up | [25] |
Mutch et al. (2009) | 36,261ischemic heart disease (IHD) patients | PCI: 1.7% CABG: 3.1% IHD-medical management group:95.2% | Any patients with existing diagnosed dementia or depression | PCI: 67.3CABG: 66.9IHD-medical management group: 74.1 | income | 4.7 y/5.2 y/4.9 y | 4.9% for the PCI group (4.7 y)7.0% for the CABG group (5.2 y)12.1% for the medical management group (4.9 y) | Patients managed with PCI had the lowest likelihood of dementia-only 65% of the risk for medical management (whether it is a reperfusion strategy is unknown)There was no significant difference between CABG and PCI interventions | [42] |
Liu et al. (2009) | 227 Patients for elective CABG75 Control (friends and families of CABG patients) | off-pump CABG: 74% on-pump CABG: 26% | Previous cardiothoracic surgery and psychiatric disease; preoperative renal dysfunction or active hepatic disease; severely impaired left ventricular function, inability to complete preoperative neuropsychological tests; absence of an acoustic window for transcranial Doppler ultrasonography and concomitant surgery other than CABG | off-pump CABG: 59±8on-pump CABG:60±8Control: 61±7 | History of previous cardiac infarction,Smoking, status of CBP use, duration of mechanical ventilation in intensive care unit | 1 wk or 3 mo after surgery | 1 wk (Control: 1.5% –13.1%; on-pump: 41.5% –68.3%; off-pump:39.2% –54.9%)3 mo (Control: 0.32% –9.3%; on-pump: 1.3% –17.5%; off-pump: 7.7% –20.4%) | In Chinese population, avoidance of CPB during CABG surgery significantly decreased the number of cerebral microemboli, but it did not decrease the incidence of POCD at either 1 wk or 3 mo after CABG. Neither CPB nor cerebral microemboli was independently associated with the risk of POCD | [43] |
Schwarz et al. (2011) | 37 coronary heart disease patients33 healthy volunteers | coronary catheterization:32% elective CABG patients:40% healthy volunteer:28% | – | Catheter group: 67.3±6.9CABG group: 66.9±5.9Control group: 64.1±6.5 | – | 3 mo after the interventions | – | Cognitive decline was detected in both catheter group and CABG group | [44] |
Bruce et al. (2013) | 16 on-pump CABG patients15 thoracic surgical patients15 nonsurgical controls | On-pump CABG patients:34% thoracic surgical patients:33% nonsurgical controls:33% | Previous cardiac operation,history of psychiatric disorders, previous neurologic complications, or traumatic brain injury, age older than 80 y or younger than 50 y, and inadequate English reading and writing skills to perform the required tasks | On-pump CABG patients: 63.6±9.0thoracic surgical patients: 60.9±7.1nonsurgical controls: 65.5±9.0 | – | 1 and 8 wk postoperatively | 1 wk: (CABG: 44%; surgical control: 33%)8 wk: nearly all patients had recovered to preoperative levels | Most patients recover to, or exceed, preoperative levels of cognition within 8 wk | [45] |
Sauër et al. (2013) | 280 patients PCI or off-pump CABG | PCI: 49% CABG: 51% | – | PCI: 60±9Off-pump CABG: 59±10 | Age and educational level | 7.5 y after procedure | – | At 7.5 y follow-up, off-pump CABG patients had a similar or perhaps even better cognitive performance compared with PCI patients | [46] |
Habib et al. (2014) | 134 first-time CABG patients | CABG: 100% | Emergency CABG, with additional cardiac procedures, myocardial infarction (MI) within 1 mo and known psychiatric illness | 53.7±8.4 | – | At discharge, 6 wk and 6 mo post-CABG | Baseline: 44.8% At discharge:54.5% 6 mo: 39.7% | Postoperative cognitive deficit was common and remained persistent at short-term | [47] |
Belmonte et al. (2015) | 36 patients undergoing off-pump CABG | off-pump CABG: 100% | – | 65.9±1.4 | – | 1, 6, and 12 mo after surgery | 6 mo: >50% 12 mo: >30% | Significant deterioration in cognition occurred after CABG, with the most severe at 6 mo and partial recovery at 12 mo | [48] |
Xu et al. (2015) | 813 patients undergoing off-pump CABG | off-pump CABG: 100% | Patients withunstable angina or critical disease that required immediate intervention | – | – | 7 days after operation | 12.9% | Postoperative cognitive dysfunctionoccurred in 12.9% of patients | [49] |
Everedet al. (2016) | 193first-time elective CABG patients51 subjects from the community as control group | CABG: 100% | History of stroke or transient ischemic attack, treatment with sedatives, and a clinical history of dementia | POCD or dementia: 67.5±8.2POCD and dementia: 67.7±7.0Control:72.0±7.2 | Age | 7.5 y after the surgery | 32.8% | The prevalence of dementia at 7.5 y after CABG surgery is greatly increased compared to population prevalence | [50] |
Jurga et al. (2016) | 93 patients with stable angina pectoris | PCI: 15% Coronary angiograph (CA): 100% | A history of CABG surgery, or advanced kidney disease (eGFR)<30 ml/min | 66.8±8.7 | SexTreated with PCIRadial access | 3 and 30 days after the procedure | – | No impairment of cognitive function as assessed by MoCA testing was found after CA or PCI | [26] |
Kuźma et al. (2017) | 3155Patients fromCardiovascular Health Study | CABG: 5.1% No CABG: 94.9% | Prevalent dementia at baseline, insufficient data to determine dementia status, or a missing history of CABG at baseline | CABG: 74.9±4.2No CABG: 74.5±4.9 | age, sex, ethnicity, education status, hypertension; treated hypertension, diabetes, clinical cardiovascular disease or subclinical CVD, body mass index, depressive symptoms, smoking, alcohol consumption; physical activity and apolipoprotein E status | 6.0 y (interquartile range, 4.7 to 6.5) | – | CABG history is associated with long-term dementia risk | [51] |
Whitlock et al. (2019) | 3,105 Health and Retirement Study (HRS) participants | Cardiac Catheterization:61.9% Cardiac Surgery:38.1% | – | PCI:74.8±6.5CABG: 74.6±6.3 | age, sex, race/ethnicity education, tobacco use, hypertension, diabetes, lung disease, stroke, heart problems, total financial assets, marital status, depression symptoms, independence in daily activities, moderate-severe pain | Participants may have undergone the index procedure any time in the preceding 2 y | – | The memory declines following heart surgery and cardiac catheterization were similar. Intermediate-term population-level adverse cognitive effects of cardiac surgery, if any, are likely subtle | [22] |
Gu et al. (2019) | 271 patients with non-ST-elevation acute coronary syndrome | PCI: 83% CABG: 3.7% Medical therapy: 13.3% | Cardiac arrest, ventricular arrhythmia or cardiogenic shock, moderate to severe valvular heart disease, active infection, malignancy with expected survival < 1 y | 80.5±4.8 | Periprocedural complicationLength of hospital stay | 1 y after procedure | 35.1% | More than one-third of patients experienced cognitive decline 1 y after invasive treatment | [52] |
Relander et al. (2020) | 103 elective CABGpatients17 volunteers as Control | CABG with CPB: 100% | Cerebrovascular events or any other disability within 6 mo, independence, or compliance (such as neurological disability or psychoses) | Control:63.6±6.9CABG with CPB: 60.4±8.6 | baseline cognitive performance, age, gender, education, and cardiovascular risks factors | 1 wk, 3 mo, and a mean of 6.7 y after operation | 1 wk after surgery: 71% 3 mo after surgery:47% | Although POCD is mostly transient at the group level, the occurrence of POCD after CABG predicts cognitive decline 6 y after surgery | [53] |
Whitlock et al. (2021) | 1680 HRS participants | PCI: 60% CABG: 40% | Did not participate in an HRS assessment, or did not complete the HRS cognitive assessment, within 3 y prior to the cardiac procedure, or if they were assigned zero sampling weight at the interview prior to the cardiac procedure | PCI:75.0±7.2CABG: 74.8±6.6 | sex; education; age; marital status; financial assets; body mass index; smoking; moderate-severe pain; difficulty in daily living; depressive symptoms; hypertension, diabetes, stroke, lung and heart disease. | 6 mo after procedure to 5 y after procedure | – | The revascularization procedure type (CABG or PCI) was not significantly associated with differences in the change of rate of memory decline. And there was no significant difference in memory score rates between preoperative and postoperative 6 mo to 5 y | [23] |
Tang et al. (2023) | 1390 HRSparticipants | PCI: 60% CABG: 40% | – | 75±6 | Sex, financial assets, education, diabetes, stroke, depressive symptoms | At a median of 1.1 (interquartile range, 0.6–1.6) y after procedure | PCI:(Mild memory decline: 10.6%; Major memory decline: 3.5%)CABG:(Mild memory decline: 8.6%; Major memory decline: 2.9%) | Preoperative factors (older age, frailty, and off-pump CABG) can be used to predict late memory decline after coronary revascularization in an epidemiological cohort with high specificity | [54] |
Table 2
Behavioral assessment of MI/RI-related cognitive impairment
Study (year) | Animal | Cardiac I/R | Cognitive-related behavioral tests | Time of testing | Results | Ref. |
Yuan et al. (2014) | SD rats | Ligation of the left main coronary artery (LCA) for 30 min, followed by reperfusion | Eight-arm maze test | 1–7 days after cardiac I/R | The cardiac I/R group showed more working memory errors 1–4 days after I/R and more reference memory errors 1–7 days after I/R relative to the sham group, respectively | [65] |
Hovens et al. (2016) | Wistar rats | Ligation of the left anterior descending branch (LAD) for 45 min, followed by reperfusion | Novel location test, novel object test, and Morris water maze test | 11 days after cardiac I/R | Spatial learning, spatial memory, and object recognition were impaired in the cardiac surgery group | [59] |
Evonuk et al. (2017) | C57BL/6 mice | Ligation of the LAD for 30 min, followed by reperfusion | Contextual fear conditioning and object location memory task | 2 months after cardiac I/R | Compared to sham-operated mice, cardiac I/R mice performed worse in contextual fear conditioning and in object location memory task | [18] |
CLINICAL CLUES SUGGEST THAT MI/RI MAY BE THE CAUSE OF COGNITIVE IMPAIRMENT
In contemporary medical practice, a significant proportion of cardiac-related procedures involves intervention aimed at reconstructing coronary blood flow, including percutaneous coronary intervention (PCI), percutaneous coronary transluminal angioplast, and coronary artery bypass grafting (CABG) [20, 21]. Despite the inevitable occurrence of varying degrees of MI/RI during these interventions, it is noteworthy that this phenomenon is seldom regarded as a potential risk factor for cognitive changes following cardiac surgery or procedures from a clinical standpoint. This may be attributed to the challenge of clinically distinguishing, monitoring, or predicting MI/RI. However, it is crucial to emphasize that MI/RI should not be discounted as a contributing factor to cognitive dysfunction post-cardiac surgery or procedures.
A large cohort study involving 3,105 participants revealed comparable levels of memory loss following both cardiac surgery and cardiac catheterization [22]. Moreover, a recent high-quality retrospective study indicated that, under average population characteristics, the anticipated probability of dementia five years post-revascularization stood at 10.5% for the CABG group and 9.6% for the PCI group, with no statistically significant difference [23]. In addition, evidence from a systematic review proposed that the type of reconstructive surgery (PCI and CABG) is not significantly linked to variations in cognitive performance deterioration [24]. It is widely recognized that PCI, as an alternative coronary revascularization method to CABG, mitigates exposures to several medical factors believed to contribute to cognitive decline after CABG, such as extracorporeal circulation pumps, general anesthesia, and surgical trauma. Consequently, this evidence underscores the importance of considering factors like MI/RI that manifest following both PCI and CABG in relation to cognitive decline post-cardiac surgery. Additionally, the presence of collateral circulation has the potential to attenuate MI/RI, and existing evidence suggests that collateral circulation in coronary arteries diminishes the risk of postoperative cognitive impairment [25]. Remarkably, the vast majority of the patient population (85%) who exclusively underwent coronary angiography displayed no postoperative cognitive dysfunction [26]. Collectively, this body of evidence strongly implies that MI/RI may play a pivotal role in postoperative cognitive decline. Detailed characteristics of MI/RI-related postoperative cardiac cognitive dysfunction studies are listed in Table 1.
Indirect clinical evidence suggests a potential association between MI/RI and cognitive dysfunction. In clinical scenarios, MI/RI can manifest as reperfusion arrhythmias, myocardial failure, low cardiac output, and perioperative myocardial infarction [27]. For instance, a study reported that approximately 9% of patients with acute ST-segment elevation myocardial infarction develop atrial fibrillation during or immediately after PCI [28], potentially induced by MI/RI. A well-established causal relationship between atrial fibrillation and cognitive dysfunction has been documented [29–31]. Furthermore, the low cardiac output or myocardial infarction resulting from MI/RI leads to inadequate cerebral perfusion, mirroring the pathophysiological features of cognitive impairment observed in heart failure cases [32]. In addition, systemic inflammation is activated after MI/RI, releasing substantial amounts of inflammatory factors into the bloodstream [33]. Also, the role of postoperative serum or plasma cytokines, particularly interleukin 6 (IL-6), in postoperative cognitive dysfunction has been extensively validated in patients [34, 35].
Despite the compelling evidence, drawing a definitive causal link between MI/RI and cognitive impairment from available clinical data proves challenging. This difficulty likely arises because MI/RI cannot be isolated as a singular factor in clinical settings. Nonetheless, avenue for investigation persist. Focusing on new MI/RI-related cardiovascular events at specific time points (e.g., during and after cardiac reperfusion surgery) and exploring their correlation with long-term cognitive status could prove valuable. Additionally, patients with acute coronary syndromes undergoing acute PCI are more prone to exhibit higher levels of depression and fatigue, along with poorer concentration and attention 0–5 days after PCI compared to patients undergoing elective PCI [36]. This implies the importance of considering population characteristics, suggesting that the impact on postoperative cognitive outcomes after coronary revascularization may differ for acute and chronic coronary syndromes. Furthermore, urgent attention is needed for exploring markers or measures of MI/RI in the clinic settings. This exploration is crucial not only to understand MI/RI-related complications but also to delve beyond cognitive function.
COGNITIVE-RELATED BRAIN PATHOLOGY AND BRAIN REGIONS FOLLOWING MI/RI
Significant pathological changes occur in the brain after MI/RI, and these pathological manifestations are critical for understanding the role of the condition in cognitive dysfunction. One prominent pathological feature after cardiac I/R is the disruption of the BBB, which allows the entry of numerous inflammatory mediators into the brain [19]. This disruption is accompanied by a decrease in the number of CD 11b/CD 45++low cells and an increase in the number of CD 11b/CD 45++++high cells, along with morphological changes indicative of microglia proliferation and hyperactivation [55]. Additionally, reactive astrogliosis leads to a cascade of neuroinflammation amplification [56]. Another potential mechanism of brain damage during cardiac I/R is increased oxidative stress brought on by fission and malfunction of brain mitochondria [57]. Consequently, these pathological events lead to neuronal apoptosis and dendritic spine damage, predominantly in the hippocampal region [56, 58]. Interestingly, cardiac I/R-induced pathological events also exhibited features associated with Alzheimer’s disease (AD) in the brain, such as Aβ formation and aggregation, as well as tau hyperphosphorylation [58]. In summary, the pathological events induced by cardiac I/R shown in Fig. 1 appear to contribute to the development of cognitive dysfunction collectively.
Fig. 1
Pathological manifestations of the brain after MI/RI. At the subcellular level, mitochondrial fusion and mitochondrial dysfunction, amyloid-β (Aβ) formation and tau hyperphosphorylation occur after MI/RI (left part of the figure). At the cellular level, astrocyte activation, microglia activation, overproliferation, neuronal apoptosis combined with dendritic spine damage, and increased macrophage infiltration were observed after MI/R I (middle of the figure). At the tissue level (right part of the figure), MI/RI caused damage to the blood-brain barrier (BBB). MI/RI, Myocardial ischemia/reperfusion injury.
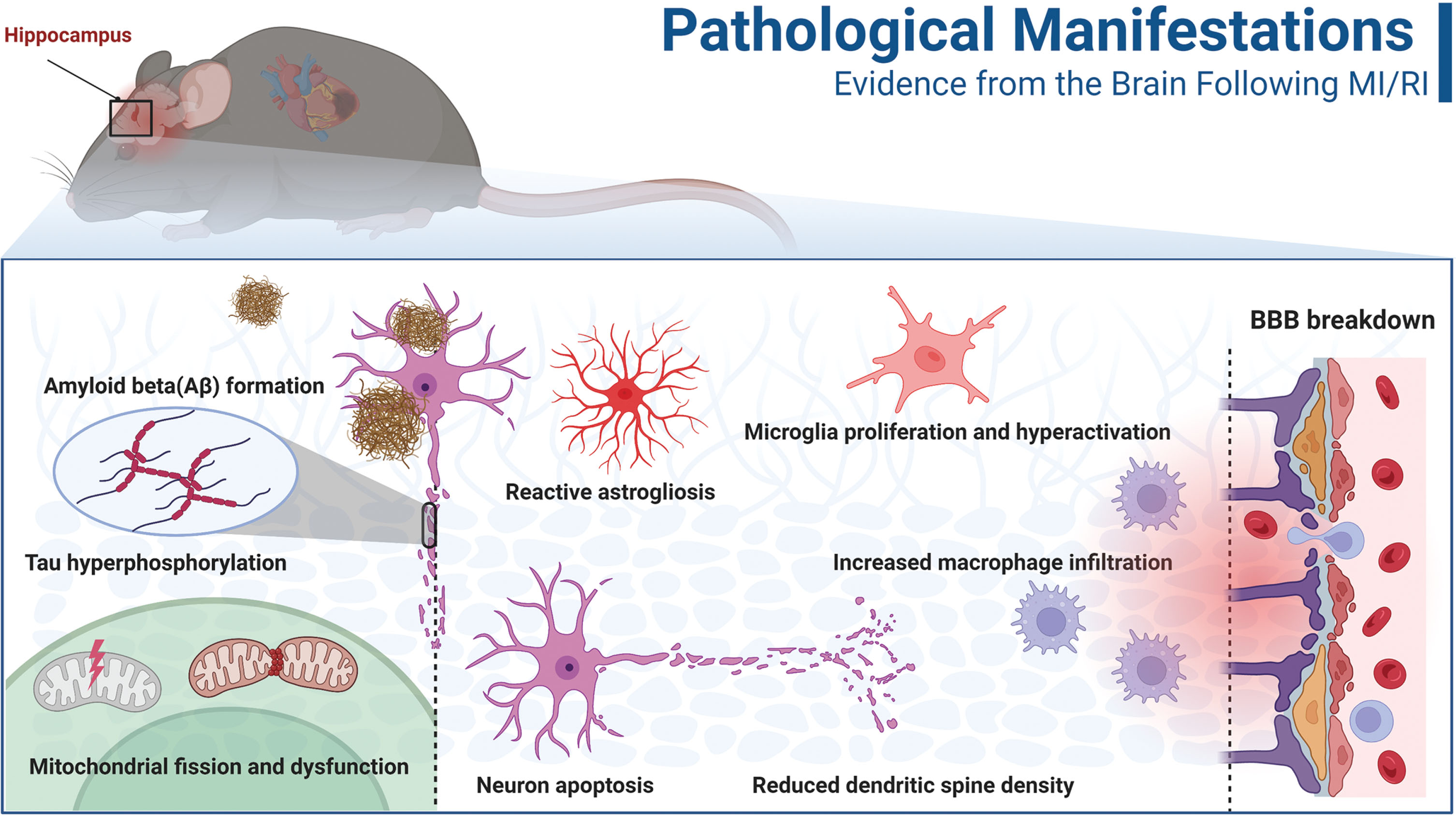
The hippocampus is one of the most extensively studied brain regions involved in cognitive decline after MI/RI. As mentioned earlier, most brain pathologies associated with cognition and memory are found in the hippocampus [18]. Furthermore, the paraventricular nucleus of the hypothalamus and prefrontal cortex have also been implicated [59]. Although the hypothalamus is primarily known for controlling physiological processes such as appetite, body temperature, sexual behaviors, and hormone release, it is interconnected with the hippocampus, amygdala, and prefrontal cortex. It may serve as an interface for various cognitive functions [60]. Moreover, a study utilizing proton magnetic resonance spectroscopy revealed alternation in metabolites in the hippocampus, striatum, thalamus, and temporal cortex following MI/RI [61]. Interestingly, the relevance of these brain regions to the formation and control of various cognitive functions is receiving increasing attention [62–64]. Overall, there is limited current research on cognition-related brain regions after MI/RI, and significant efforts are warranted in the future.
MANIFESTATIONS OF COGNITIVE IMPAIRMENT DUE TO MI/RI IN ANIMALS
Rats exhibit degradation of spatial learning and memory after cardiac I/R [59]. In a study by Evonuk and colleagues, cognitive function in mice was assessed through behavioral measures two months after cardiac I/R. The results revealed that cardiac I/R mice performed worse in contextual fear conditioning and object location memory tasks compared to sham-operated mice [18]. Similarly, rats subjected to cardiac I/R also demonstrated poorer short-term cognitive performance than sham rats in the eight-arm maze test [65]. Our unpublished data also support a significant decline in cognitive function in mice following cardiac I/R, with this decline occurring in both the short-term (7 days) and long-term (a month). In conclusion, despite insufficient attention from most investigators, converging evidence indicates that MI/RI is indeed a crucial factor contributing to cognitive dysfunction after cardiac surgery. A summary of the cognitive behavioral assessment findings in animal studies following MI/RI is presented in Table 2.
POTENTIAL MECHANISMS OF MI/RI-INDUCED COGNITIVE DYSFUNCTION
To understand the mechanisms underlying cognitive impairment induced by MI/RI is to identify the specific pathological changes and processes that occur in the body following MI/RI, ultimately contributing to or directly causing cognitive deficits. Indeed, MI/RI-induced cognitive dysfunction is an outcome of a complex series of pathological processes at the molecular, cellular, tissue, and even systemic levels. Based on the existing literature, we categorize the potential mechanisms of cognitive decline in the brain following MI/RI into three domains.
The role of circulating inflammatory molecules or cells in cognitive dysfunction after MI/RI
It is widely recognized that activating inflammation, specifically sterile inflammation, contributes to the progress of MI/RI [3]. Surprisingly, in the context of the I/R model, inflammatory mediators can act as implicated agents in the emergence of systemic complications [66]. The reliable marker of systemic inflammation, C-reactive protein (CRP)/high sensitivity C-reactive protein (hsCRP), has been shown as an indicator of MI/RI [67]. Clinical data indicate that CRP levels remain elevated after PCI, peaking at three days and persisting up to 7–14 days post-procedure [68, 69]. Notably, the relative variation of hsCRP (ΔhsCRP) from pre- to 24-h post-PCI has shown superior predictive value for future major adverse cardiac events compared to pre- or post-procedure hsCRP levels alone [70]. Importantly, elevated levels of CRP due to MI/RI play a significant role in predicting short- and long-term cardiac complications. Given the strong association between CRP and cognitive dysfunction, this predictive advantage of ΔhsCRP may also be reflected in cognitive decline after cardiac I/R [71, 72]. Several studies have reported noteworthy links between CRP and postoperative neurocognitive dysfunction (PND) after off-pump CABG and heart valve replacement [73, 74]. Moreover, in animal models, interleukin-6 (IL-6), interleukin-8 (IL-8), and tumor necrosis factor-alpha (TNF-α) levels in the blood were significantly elevated after myocardial I/R [75, 76]. Similar findings were observed in patients, with serum TNF-α and IL-6 levels substantially increased after PCI compared to baseline, particularly TNF-α, which continued to rise 14 days after PCI in acute myocardial infarction cases [69]. Deterioration in the systemic condition can profoundly impact the brain, with significant consequences. A meta-analysis and systematic review of 170 studies on AD and mild cognitive impairment (MCI) highlighted the influence of peripheral inflammation on cognitive function and that the elevated levels of circulating TNF-α have been associated with postoperative cognitive dysfunction [77]. Increased TNF-α and IL-6 have been linked to cognitive decline after cardiac surgery [78] and CABG [79], and the cytokines in the cerebrospinal fluid may serve as predictors of impaired cognition.
The systemic inflammation response index (SIRI), a novel micro-inflammatory marker based on peripheral blood neutrophil, monocyte, and lymphocyte counts, has recently gained attention. Like ΔhsCRP, this composite inflammatory index is an independent risk factor following PCI and reflects cellular-level inflammation responses [80, 81]. Furthermore, SIRI is correlated with cognitive function, as older individuals with higher SIRI levels are at a heightened risk of developing MCI [82]. Strikingly, there appears to be a logical relationship in cardiac revascularization and vascularization procedures. In patients undergoing PCI post-acute ST-segment elevation myocardial infarction (STEMI), SIRI levels have been independently associated with the no-reflow phenomenon (NRP) [83], which is a clinical characteristic manifestation of MI/RI [84]. Therefore, SIRI may serve as a representation of the degree of MI/RI in patients following PCI. Furthermore, the systemic inflammatory response syndrome score is a robust and independent risk factor for of developing PND in patients after CABG [85]. Hence, SIRI may provide additional prognostic information for the development of cognitive dysfunction after MI/RI. Additionally, the neutrophil-lymphocyte ratio (NLR), a readily available marker of systemic inflammation, has shown involvement in the pathogenesis of AD, PND, and MCI in the elderly [86–88]. However, its role in cognitive impairment after MI/RI remains unexplored. Notably, these systemic inflammation markers are inexpensive, readily available, and widely employed in clinical practice. Large-scale, well-controlled clinical studies on the relationship between these inflammatory indicators and cognitive function after cardiac I/R would be valuable in providing predictive biomarkers for impaired cognitive decline.
A growing body of evidence highlights the crucial role of platelets in inflammatory and immune responses. After cardiac I/R, platelets undergo activation via various modes, such as the release of platelet-activating factor (PAF), soluble CD40 ligand (sCD40 L), and P-selectin [89, 90]. Platelet activation is a common occurrence following PCI, and despite employing multiple antiplatelet and anticoagulant drugs, a significant and prolonged elevation of sCD40 L in plasma is observed [68]. Moreover, plasma calprotectin, associated with platelet activation, may serve as an early predictive biomarker of NRP in patients with acute coronary syndromes [91]. Platelets play a crucial role in neuroinflammation [92], and studies have shown a strong association between the degree of platelet activation and decreased cognitive function in AD [93]. However, one study reported that neuroprotective PAF antagonists (lexipafant) did not differentially reduce the level of cognitive impairment following CABG [94]. The authors suggest that this finding may be attributed to the lower-than-expected incidence of cognitive impairment in their experiment, which was insufficient to detect a protective effect of lexipafant [94]. Overall, evidence regarding the role of platelet activation in cognitive function after MI/RI is still preliminary and requires further validation.
How systemic inflammation contributes to impaired cognitive performance after MI/RI is another interesting topic. For these circulating elevated inflammatory molecules and inflammatory cells to exert adverse effects on the brain, they must transverse the BBB. Unfortunately, the BBB sustains significant damage after MI/RI. Data indicated impaired BBB integrity in 50% of post-operative cardiac patients [95]. Animal evidence also confirms a decrease in the expression levels of the BBB protein Claudin5 following cardiac I/R [19, 96]. Current evidence suggests that MI/RI induces the release of pro-inflammatory mediators from coronary endothelial cells and various inflammatory cells into the bloodstream, activating the immune response [27]. These heightened peripheral blood factors subsequently assault the compromised BBB alongside inflammatory cells [97]. On the one hand, microglia and astrocytes become activated in response to these inflammatory factors, further promoting the development of neuroinflammation. On the other hand, these blood factors and inflammatory cells can induce brain remodeling by modulating endothelial cells and neuronal signaling, resulting in the secretion of pro-inflammatory factors, oxidative stress, and the aggregation of AD-related proteins [98–100]. Moreover, inflammatory factors can directly adversely affect neuronal survival and neuronal signaling, leading to neuronal death and synaptic dysfunction [101]. The underlying mechanisms of systemic inflammation induced by MI/RI and the subsequent development of cognitive dysfunction are depicted in Fig. 2.
Fig. 2
Systemic inflammation after MI/RI promotes neuroinflammation leading to cognitive decline. MI/RI activates systemic inflammation including an increase in inflammatory mediators (IL-1β, IL-6, IL-8, TNF-α, CRP), an increase in inflammatory cells manifested by SIRI, and the activation of platelets and induction of inflammatory substances released by platelets. These systemic inflammatory mediators g attack and enter the CNS via the BBB, leading to BBB leakage and neuroinflammation. BBB, blood-brain barrier; CNS, central nervous system; CRP, C-reactive protein; IL-1β, Interleukin 1 beta; IL-6, Interleukin 6; IL-8, Interleukin 8; SIRI, Systemic inflammation response index; TNF-α, Tumor necrosis factor-alpha.
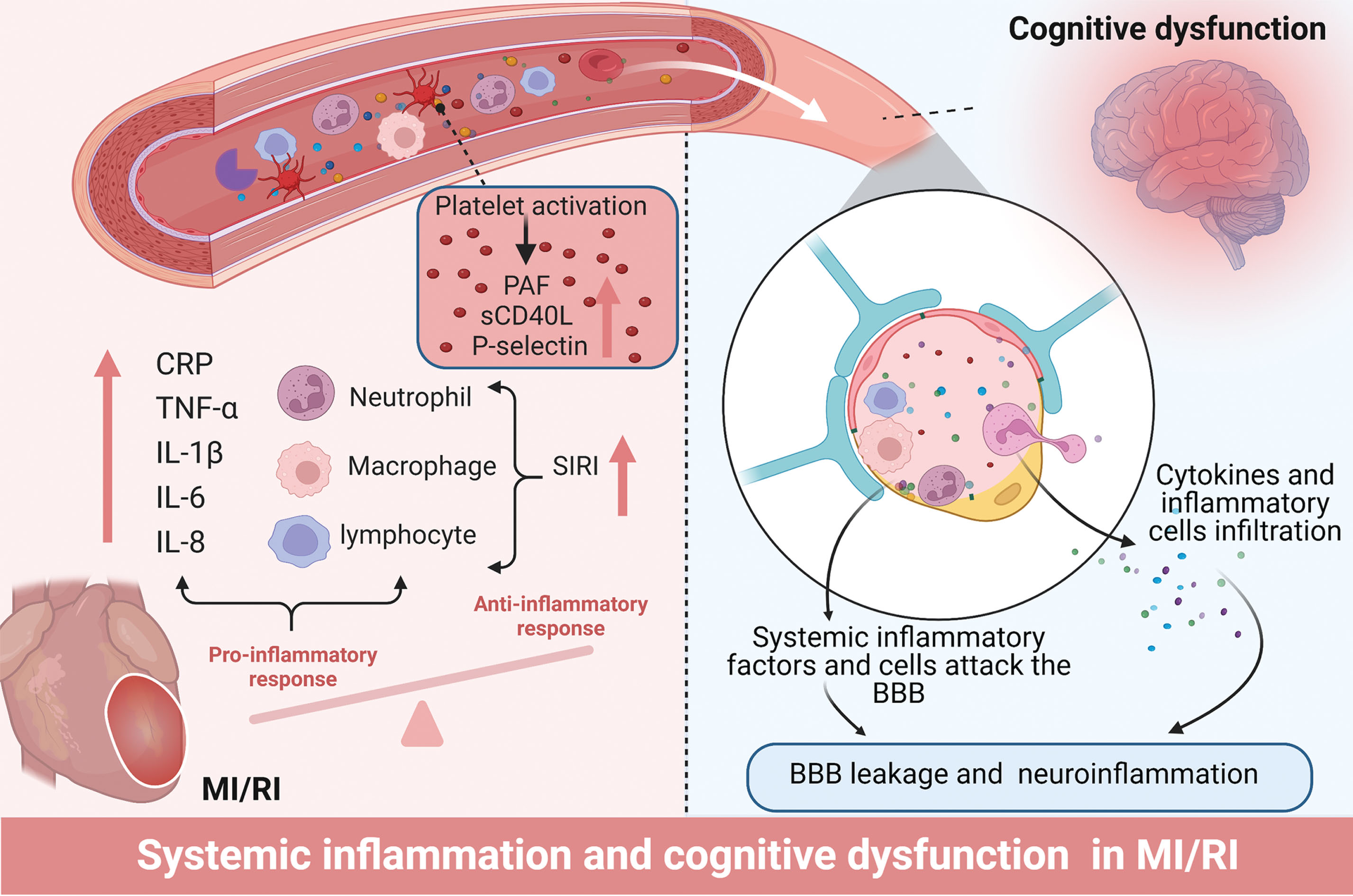
Production of local cardiac pathological mediators and heart-to-brain transport after MI/RI
Is there a mechanism other than direct invasion of the brain by soluble blood factors and peripheral immune cells that can cause cognitive changes in the brain following MI/RI? Given the considerable distance between the heart and brain, it is necessary to consider the potential for heart-brain crosstalk. Extracellular vesicles (EVs) have emerged as novel messengers facilitating information exchange between organs, including the heart [102].
EVs refer to cell-released membrane organelles such as exosomes, microvesicles, and microparticles [103]. In this review, we refer to all types of EVs collectively. These EVs are synthesized by cells and released into circulation, allowing them to reach various organs, including the brain. By transporting diverse contents, such as proteins, lipids, DNA, mRNAs, microRNAs (miRNAs), and circular RNAs (circRNAs), EVs exert significant biological effects on recipient cells. The concept that EVs mediate communication between the heart and brain is not novel [104, 105]. Evidence shows that EVs from peripheral organs can carry harmful contents into the brain via circulation and contribute to the development of cognitive dysfunction [106]. Similar evidence is emerging for brain injury and cognitive impairment after peripheral organ I/R [107, 108]. Encouragingly, EVs have been implicated in pathophysiological processes after MI/RI [109, 110].
Among the EVs’ contents, miRNAs have been extensively studied. Research by Deddens and Gidlöf et al. demonstrated increased release of cardiac-derived EVs after cardiac I/R in an animal model [111, 112], as evidenced by plasma levels of muscle-specific extracellular miRNAs (miR-1, miR-133b, miR-208b, and miR-499) were found to increase up to 750-fold [112]. Surprisingly, the increased release of EVs carrying miR-1, miR-208b, and miR-499 also occurred in plasma samples collected from patients before and after PCI, particularly after reperfusion [113]. Similar findings include increased plasma EVs concentrations in patients undergoing CABG and increased expression of miR-1, miR-24, miR-133a, and miR-133b in EVs [114]. These findings consistently suggest increased cardiac secretion and release of circulating EVs containing miR-1, miR-208b, miR-133a, and miR-133b, miR-499 post-MI/RI. Can these EVs potentially enter the brain and lead to impaired cognitive function after MI/RI? The answer appears to be affirmative. Mice with cardiac overexpression of microRNA-1 experience cognitive impairment [115]. Inhibition of miR-1 in hippocampal EVs significantly attenuates infarct-induced neuronal microtubule damage [116]. Additionally, miR-1 from cardiac EVs has been shown to attenuate infarct-induced synaptic vesicle exocytosis in the hippocampus by regulating the target gene synaptosomal-associated protein 25 (SNAP-25) [117]. Furthermore, miR-499 was significantly increased among the miRNAs carried by EVs after MI/R. Notably, miR-499-5p is implicated in neurocognitive dysfunction in central nervous system disorders by causing dendritic disruption and dysregulation of calcium homeostasis [118]. Apart from miRNAs, circRNAs within EVs may also contribute to this theory. CircRNA-089763 was remarkably increased in plasma EVs of patients with cardiac I/R occurrence (post-CABG), and the functional annotation analysis and target gene prediction suggested a significant enrichment in GO keywords and pathways associated with PND [119].
MI/RI-induced EVs not only exacerbate local inflammation in the heart but also trigger systemic inflammation in distant organs [120]. This raises the question of how EVs may facilitate inflammation spreading to the central nervous system (CNS). Oxidative phosphorylation function in mitochondria is impaired after MI/RI, leading to increased formation of free radicals/reactive oxygen species [121]. A recent study reveals that under graded oxidative stress in vitro, mitochondria isolated from the myocardium actively generate vesicles known as mitochondria-derived vesicles, which carry many mitochondrial proteins demonstrated by a proteomic analysis [122]. Interestingly, several of these mitochondrial proteins are also present in circulating EV isolated from the blood of patients with diseases associated with oxidative stress disorders [123]. Furthermore, mitochondrial DNA (mtDNA) in cardiac cells is not protected from oxidative stress damage during MI/RI, and mitochondria-derived vesicles can act as intermediaries, mediating the transfer of mtDNA into EVs [124]. Once cardiac cells release mtDNA into circulation during MI/RI, it can remain protected within EVs and travel through bloodstream to mediate the inflammatory response in the target organ [125]. Circulating mtDNA has been shown to activate TLR9 (toll-like receptor 9), promoting inflammation through activation of the myeloid differentiation primary response 88 (MyD88) and p38 MAPK (mitogen-activated protein kinase) pathways [126, 127]. Additionally, mtDNA activates NLRP3 inflammasomes, triggering the production of IL-1β and IL-18 [128, 129]. The mtDNA in EVs can potentially activate neuroinflammation in neurodegenerative diseases [125]. Interestingly, it was also demonstrated that injection of mtDNA into the hippocampus of mice induces neuroinflammation [130]. These finding also provides directions for future research. It has also been shown that MI/RI-induced EVs contribute to the dissemination of inflammation, as evidenced by the significant elevation of high mobility group box 1 (HMGB1), an injury-associated molecular pattern, in plasma after cardiac I/R [131]. These increased HMGB1 levels in reperfused coronary arteries mainly originate from platelet-derived particles and are predominant in patients with MI/RI injury presenting as no flow phenomenon [132].
These aforementioned pieces of evidence support the possibility that MI/RI triggers the production of EVs, which can transport harmful mediators (miRNAs, circRNAs, inflammatory proteins, cytokines, and mitochondrial components) through circulation into the brain, thereby activating neuroinflammation and resulting in neurocognitive impairment (Fig. 3). However, despite the burgeoning interest in EVs, there is currently insufficient data to evaluate the brain effects of EVs released into the circulation after MI/RI.
Indirect mechanisms of cognitive dysfunction due to altered cardiac function after MI/RI
Fig. 3
EVs assist in the relay of harmful signals from the heart to the CNS after MI/RI, mediating cognitive dysfunction. After MI/RI, mitochondria in cardiomyocytes release MDVS encapsulating mitochondrial proteins and mtDNA, while cardiomyocytes release some EVs containing microRNAs (MiR-1, MiR-499) and circRNAs (CircRNA-089763). These EVs from cardiomyocytes are released into the circulation, and together with platelet release PMP as well as plasma EVs containing HMGB1 reach the CNS via TLR9 activating MyD88, p38MAPK, activating NLRP3 and some pro-inflammatory responses together leading to cognitive dysfunction after MI/RI. EVs, Extracellular vesicles; HMGB1, High mobility group box 1; MDVs, Mitochondria-derived vesicles; mtDNA, mitochondrial DNA; NLRP3, NOD-like receptor thermal protein domain associated protein 3; PMS, Platelet-derived particles; TLR9, Toll-like receptor 9.
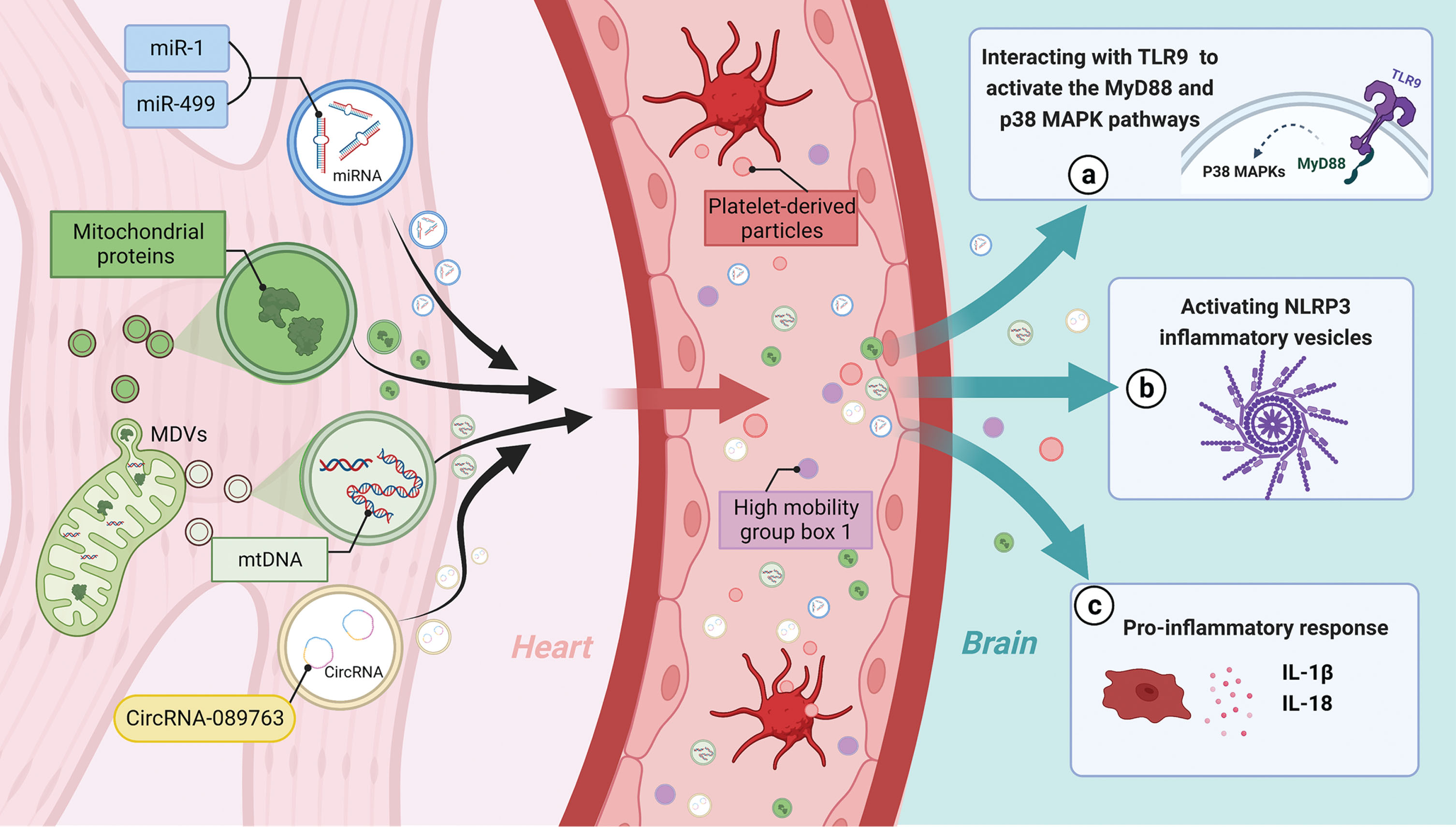
One well-known consequence of MI/RI is the impairment of cardiac function. This can manifest as arrhythmias, heart failure, and myocardial infarction. A direct consequence of these manifestations is a decrease in cardiac output, which may lead to varying degrees of cerebral hypoperfusion. A study showed that MI/RI rats exhibited cardiac insufficiency and reduced cerebral blood flow in the accumbens core, left caudate putamen, hippocampus, left hypothalamus, olfactory, superior colliculus, right midbrain, ventral tegmental area, inferior colliculus and left thalamus whole [133]. The hypothesis that heart failure exacerbates cerebral blood flow (CBF) and contributes to cognitive decline is supported by existing literature [134]. However, it has been argued that lower CBF does not fully explain cognitive impairment in patients with hemodynamic dysfunction along the cardio-cerebral axis [135]. Nevertheless, the study conducted by the authors established a cross-sectional association between CBF and cognitive function in patients with heart failure, but not a longitudinal analysis. In patients undergoing CABG, postoperative changes in CBF are commonly associated with PND [136, 137]. On the other hand, there is inconsistent evidence suggesting that a significant reduction in CBF after CABG does not correlate directly with neuropsychological test results [138]. In fact, patients with PND showed an average decrease of approximately 7% in regional CBF (rCBF), while patients without PND exhibited an increase of about 2% in rCBF. These results did not reach statistical significance, possibly due to the small sample size and the large standard deviation. Additionally, ischemic heart disease is strongly linked to cognitive status after cardiac surgery [139], a factor not addressed in this study. In addition, inadequate cerebral perfusion leads to decreased cerebral oxygen saturation, which can be noninvasively measured using near-infrared spectroscopy. Studies suggest that long-term declines in regional cerebral oxygen saturation (rSO2) predict cognitive decline [140]. Though there are no long-term monitoring data on brain oxygen saturation after MI/RI, indirect evidence supports this theory. During CABG, intraoperative declines in brain oxygen saturation significantly correlate with early and late cognitive decline in patients [141, 142]. In addition, a study monitoring oxygen saturation after cardiac surgery found that decreased postoperative brain saturation was significantly associated with postoperative delirium [143]. Taken together, low cerebral perfusion or cerebral oxygen saturation is a risk factor for cognitive dysfunction resulting from impaired cardiac function. However, whether MI/RI specifically drives this phenomenon remains largely unknown and requires further investigation. Notably, it is essential to highlight that the cognitive outcome for patients undergoing On-Pump CABG is worse than that of those for patients undergoing Off-Pump CABG [144]. The acknowledged adverse effect of cardiopulmonary bypass (CBP) on cognition is partly attributed to reduced CBF [137]. This suggests that cognitive dysfunction after On-Pump CABG may be more related to CBP-induced cerebral hypoperfusion. Additionally, atrial fibrillation occurring after MI/RI may lead to micro-clots formation and occlude small cerebral vessels, potentially exacerbating cognitive dysfunction [145]. Although anesthesia also has the potential to influence cognitive function in cardiac surgery, it is not further discussed in our review, as our main focus is determining the role of MI/RI in postoperative cardiac cognitive dysfunction and elucidating potential mechanisms [146]. It is essential to emphasize that the mechanisms described above are indirect pathways through which MI/RI may contribute to cognitive dysfunction, and they are not specific to MI/RI. Future research is needed to establish causal evidence for these indirect mechanisms.
PREVENTION STRATEGIES FOR COGNITIVE DYSFUNCTION AFTER MI/RI
Research focused on preventing the cognitive changes associated with MI/RI is primarily driven by efforts to circumvention MI/RI. Although the precise mechanisms underlying MI/RI-related cognitive impairment remain incompletely understood, there are methods to prevent MI/RI partially and thereby address the fundamental cause of mental issues associated with it. Ischemic preconditioning (IPC), which involves subjecting the heart to brief ischemic stress, is the most effective intrinsic preventive measure against MI/R. IPC reduces subsequent MI/RI and mitigates the range of extracardiac injuries resulting from MI/RI [147]. However, the translational relevance of IPC is limited. Therefore, remote ischemic preconditioning (RIPC) has gained more interest, and it is a protective strategy focused on the circulation between ischemia and reperfusion within a specific vascular region (e.g., the arm) [148–150]. Heusch et al. comprehensively summarized the cardiac impact of RIPC in prior elective I/R studies (PCI and CABG). They provided compelling evidence supporting the beneficial effects of RIPC in mitigating MI/RI, particularly when applied to the upper limb [151]. Thus, RIPC holds promise as an approach to alleviate MI/RI-induced cognitive dysfunction.
Encouragingly, studies have demonstrated RIPC improved performance on behavioral tests in rats subjected to brain I/R and enhanced cognitive control in healthy adults [152, 153]. Investigation on the role of RIPC in MI/RI-induced cognitive dysfunction has yielded mixed results. One study reported that RIPC prevented postoperative decline in cognitive function over the short term in patients undergoing cardiac surgery, the majority of whom underwent surgery for CABG [154]. Three other studies observed a tendency of RIPC to attenuate MI/RI-induced neurocognitive outcomes, as indicated by reduced serum markers of brain damage and a non-significant decrease in incidence [155–157]. Contrastingly, two studies found no impact of RIPC on neurocognition [158, 159]. Notably, one of these studies did not specify the composition and proportion of cardiac surgery type performed on the patient, and surgery under propofol anesthesia was involved [159]. It is noteworthy that the occurrence and severity of MI/RI vary among different types of cardiac procedure, predominantly PCI and CABG, and multiple factors, including age, hypertension, beta-blocker, sex, diabetes, heart failure, and propofol, may influence the clinical utilization and effectiveness of RIPC [160]. Furthermore, propofol has been shown to diminish the protective effects of RIPC [161, 162]. In another study, cognitive assessment was conducted using a six-category test, and cognitive morbidity was used as an indicator [158]. Although the results suggested a lower cognitive decline in five out of the six categories among participants in the RIPC group compared to the control group, the overall prevalence rate of cognitive impairment was not significantly different or even higher in the RIPC group [158]. The methodology for calculating cognitive prevalence was not clearly defined in the study, and the interpretation of the results may be due to consideration of poor performance on any one of the six tests as cognitive impairment. Consequently, more individuals in the RIPC group have a lower level of impairment, while fewer people in the control group have a higher level of impairment. It remains uncertain whether the assessment of morbidity in this context provides an objective evaluation of the patient overall cognitive profile. The issue of confounding by procedure type was also present in the two studies that reported trends but lacked statistically significant differences, where valve placement was performed in 75% and 45% of the respective patients’ cohorts [156, 157].
The aforementioned unpromising results raise concern due to the heterogeneity of the patient population and potential bias introduced by variations in surgery type and anesthesia. The clinical translation of RIPC is also influenced by the number of cycles and the duration of I/R. Although most human studies on CABG utilize 3–4 cycles of I/R (5 min/5 min), the most common approach in PCI is three cycles of I/R (3 min/3 min). Nonetheless, 3–4 cycles of I/R (5 min/5 min) yield greater effectiveness in preventing MI/RI. It is disappointing that no studies to date have specifically examined the effect of RIPC on cognitive function after PCI, although such research is encouraged, given the lower confounding factors compared to CABG and the relevance to MI/RI. Furthermore, most of the studies primarily focus on short-term cognitive impairment, whereas investigating the long-term cognitive protective effects of RIPC is an important direction for future research.
In conclusion, the favorable cognitive outcomes observed in these studies enhance the credibility of RIPC as a preventive measure against MI/RI-induced cognitive decline. However, larger, standardized multicenter studies are still required to determine the extent to which RIPC translates into clinical benefit. It is important to emphasize that blood pressure control, glucose control, cerebral perfusion and cerebral oxygen saturation management, temperature management, use of some preoperative medications, preoperative cognitive training, avoidance of some anesthetics as well as CPB, etc. have the potential to prevent cognitive impairment after cardiac surgery [144, 163–168] However, the subject of our review is the relationship between MI/RI and cognitive dysfunction after cardiac surgery, thus highlighting the neuroprotection of ischemic preconditioning in MI/RI. However, we are in no way dismissing the role of other strategies in MI/RI-related cognitive deficits; moreover, the evidence for other strategies has been detailed in previous reviews [146, 169].
TREATMENTS OF COGNITIVE IMPAIRMENT DUE TO MI/RI
The post-ischemic treatment corresponding to ischemic preconditioning is ischemic postconditioning (I-postC), involving multiple brief reperfusion/ischemic treatments before sustained reperfusion following ischemia. Studies have shown the beneficial effect of I-postC on brain injury after MI/RI, partly through inducing glycogen synthase kinase-3 beta (GSK-3β) autophosphorylation [136]. However, similar to IPC, the clinical application of I-postC is not promising. Animal experiments have suggested alternative effective interventions for MI/RI-induced brain cognition, including electroacupuncture [65]. Extensive researchers have also explored the pharmacological treatment of brain injury after MI/RI, using inhibitors such as p-donepezil (a well-known inhibitor of acetylcholinesterase), and proprotein convertase subtilisin/kexin type 9 inhibitor, as well as various cell death inhibitors [19, 55, 58] (Table 3). Neuroprotective and cytoprotective peptides like humanin and the pharmacological mitochondrial dynamics regulator mitochondrial fusion promoter (M1) have demonstrated the ability to reverse brain pathology caused by MI/RI [57, 96]. Additionally, evidence supports the effectiveness of metformin and sevoflurane, among other clinical agents [170–172]. Notably, gas anesthetics (desflurane, isoflurane, and sevoflurane) have been associated with better postoperative cognitive performance in CABG patients compared to propofol, suggesting a preference for gas anesthetics in CABG patients at risk for MI/RI impairment [172–174]. In summary, the current strategies for the treatment of MI/RI-induced brain injury are still in the early stage, calling for future attention and research in this area.
Table 3
Treatment of brain injury and cognitive dysfunction following MI/RI
Treatment | Time | Animal | Major findings | Ref. | ||||||
BBB breakdown | Brain | Oxidative stress | Aβ production/Tau | Neuronal | Dendritic | Cognitive | ||||
breakdown | inflammation (BI)/ | (OS)/mitochondrial | hyperphosphorylation | apoptosis | spine | dysfunction | ||||
Peripheral | function (MD) | density | ||||||||
inflammation (PI) | (DSD)/LTP | |||||||||
Donepezil | 15 min before ischemia/15 min after ischemia/Onset of reperfusion | Wistar Rats | ↓ | BI↓ | OS↓MD↑ | Aβ↓ | ↓ | DSD↑ | – | [19] |
Humanin | 15 min after ischemia/onset of reperfusion | Wistar rats | ⟶ | – | OS⟶MD↑ | Aβ⟶p-Tau/Tau↓ | ↓ | – | – | [57] |
Mitochondrial fusion promoter (M1) | 15 min prior to I/R | Wistar rats | ↓ | BI↓ | MD↑ | Aβ⟶p-Tau/Tau↓ | ↓ | – | – | [96] |
Z-VAD-FMK | 15 min prior to I/R | Wistar rats | ⟶ | BI↓ | OS↓MD↑ | Aβ↓p-Tau/Tau↓ | ↓ | DSD↑ | – | [58], [56] |
Necrostatin-1 | 15 min prior to I/R | Wistar rats | ↓ | p-NF-κB/NF-κB⟶ | ⟶ | Aβ↓p-Tau/Tau↓ | ↓ | DSD↑ | – | [58] |
Ferrostatin-1 | 15 min prior to I/R | Wistar rats | ⟶ | p-NF-κB/NF-κB⟶ | OS↓MD↑ | Aβ↓p-Tau/Tau↓ | ⟶ | DSD↑ | – | [58] |
Metformin | 15 min prior to ischemia/15 min after ischemia | Wistar rats | ⟶ | BI↓ | MD↑ | Aβ↓ | ↓ | DSD↑ | – | [171] |
Metformin | 15 min prior to ischemia | Wistar rats | – | BI↓ | MD↑ | Aβ↓p-Tau/Tau↓ | – | DSD↑ | – | [170] |
Proprotein convertase subtilisin/kexin type 9 inhibitor (PCSK 9i) | 15 min prior to ischemia/15 min after ischemia/onset of reperfusion | Wistar rats | – | BI↓ | – | Aβ↓ | – | DSD↑ | – | [55] |
Sevoflurane | Preconditioning | Wistar rats | – | BI↓ | OS↓ | – | – | LTP↑ | – | [172] |
SRT1720 | 5 h after I/R | C57BL/6 mice | – | BI↓PI↓ | – | – | – | – | ↑ | [175] |
Electroacupunture | Onset of reperfusion | Sprague Dawleyrats | – | BI↓PI↓ | OS↓ | – | ↓ | – | ↑ | [65] |
CLINICAL IMPLICATION
Previous studies have indicated that patients who undergo CABG may experience PND, as evidenced by biomarker changes similar to those seen in AD [176]. Additionally, mouse models have shown AD-like manifestations in brain pathology after MI/RI, suggesting a potential connection between MI/RI and AD. However, the etiology of postoperative cognitive decline in current clinical studies still does not take M/RI into account, so it is difficult to conclude the causal relationship between MI/RI and cognitive impairment from the limited clinical evidence available. Consequently, it is crucial to investigate the impact of MI/RI on cognitive dysfunction after cardiac surgery. Recognition of this role may promote the exploration of safe and non-invasive methods like IPC or RIPC for preventing and protecting against cognitive impairment after cardiac surgery. Additionally, studying the mechanisms by which MI/RI leads to cognitive impairment is essential. One promising avenue is the use of EVs that contain a significant amount of non-coding RNAs and serve as specific biomarkers for MI/RI-related cognitive decline [177]. Furthermore, EVs can potentially be both pathogenic and therapeutic mediators in cardiovascular diseases [178], and EVs inhibitors hold promise for various clinical applications [179]. In addition, actively improving cardiac function and reducing inflammation can help mitigate or prevent cognitive decline associated with MI/RI. In conclusion, this opens up new avenues for identifying and intervening in MI/RI-related cognitive impairment in clinical settings, providing valuable insights and directions.
SUMMARY AND OUTLOOK
MI/RI is common in CAD patients undergoing cardiac procedures, but the underlying mechanism linking MI/RI to brain pathologies remains unclear. Besides systemic inflammation, cardiac insufficiency following MI/RI is recognized as a factor contributing to cerebral under-perfusion and cognitive impairment. Based on recent clues, we hypothesize that releasing harmful molecules after MI/RI may induce brain pathology through circulation, either directly or within encapsulated EVs. Moreover, various pharmacologic treatments and interventions have shown promise in reducing brain pathology and improving cognition in animal models of MI/RI. Despite substantial research demonstrating the impact of MI/RI on cognitive impairment, studies in this area, particularly in the clinical setting, have been limited. Currently, clinical practice primarily focuses on myocardial injury factors such as creatine kinase-MB and cardiac troponin I, neglecting the significance of MI/RI for cognitive impairment. Thus, future studies should prioritize the development of markers for predicting MI/RI in clinical settings to provide direct evidence of its role in cognitive impairment. Mechanistically, there are gaps in the understanding of cognitive decline after MI/RI, as research has primarily focused on the initial event (MI/RI) and subsequent brain pathology without establishing a clear connection between them. Therefore, comprehensive investigations are needed to establish a cohesive pathogenesis narrative. In conclusion, the available evidence is preliminary, and mechanisms, preventive and therapeutic measures, as well as additional interventions, requires exploration and validation in in both clinical and basic aspects.
ACKNOWLEDGMENTS
The authors have no acknowledgments to report.
FUNDING
This work was supported by the National Natural Science Foundation of China (No.82171185 and No.U23A20422 to Dr. Chan Chen); Central Guidance on Local Science and Technology Development Fund of Sichuan Province (No.23ZYZYTS0135 to Dr. Chan Chen); The Post-Doctor Research Project, West China Hospital, Sichuan University (No. 2021HXBH061 to Dr. Erya Chen); The Youth Project of Natural Science Foundation of Sichuan Provincial Department of Science and Technology (No. 2023NSFSC1563 to Dr. Erya Chen); The China Postdoctoral Science Foundation (No.2022M722254 to Dr. Erya Chen).
CONFLICT OF INTEREST
The authors have no conflict of interest to report.
REFERENCES
[1] | Roth GA , Mensah GA , Johnson CO , Addolorato G , Ammirati E , Baddour LM , Barengo NC , Beaton AZ , Benjamin EJ , Benziger CP , Bonny A , Brauer M , Brodmann M , Cahill TJ , Carapetis J , Catapano AL , Chugh SS , Cooper LT , Coresh J , Criqui M , De Cleene N , Eagle KA , Emmons-Bell S , Feigin VL , Fernández-Solà J , Fowkes G , Gakidou E , Grundy SM , He FJ , Howard G , Hu F , Inker L , Karthikeyan G , Kassebaum N , Koroshetz W , Lavie C , Lloyd-Jones D , Lu HS , Mirijello A , Temesgen AM , Mokdad A , Moran AE , Muntner P , Narula J , Neal B , Ntsekhe M , Moraes de Oliveira G , Otto C , Owolabi M , Pratt M , Rajagopalan S , Reitsma M , Ribeiro ALP , Rigotti N , Rodgers A , Sable C , Shakil S , Sliwa-Hahnle K , Stark B , Sundström J , Timpel P , Tleyjeh IM , Valgimigli M , Vos T , Whelton PK , Yacoub M , Zuhlke L , Murray C , Fuster V ((2020) ) GlobalBurden of Cardiovascular Diseases and Risk Factors, 1990-2019:Update From the GBD 2019 Study. J Am Coll Cardiol 76: , 2982–3021. |
[2] | Lawton JS , Tamis-Holland JE , Bangalore S , Bates ER , Beckie TM , Bischoff JM , Bittl JA , Cohen MG , DiMaio JM , Don CW , Fremes SE , Gaudino MF , Goldberger ZD , Grant MC , Jaswal JB , Kurlansky PA , Mehran R , Metkus TS Jr. , Nnacheta LC , Rao SV , Sellke FW , Sharma G , Yong CM , Zwischenberger BA ((2022) ) 2021 ACC/AHA/SCAI Guideline for Coronary Artery Revascularization: Executive Summary: A Report of the American College of Cardiology/American Heart Association Joint Committee on Clinical Practice Guidelines. J Am Coll Cardiol 79: , 197–215. |
[3] | Frank A , Bonney M , Bonney S , Weitzel L , Koeppen M , Eckle T ((2012) ) Myocardial ischemia reperfusion injury: From basic science to clinical bedside. Semin Cardiothorac Vasc Anesth 16: , 123–132. |
[4] | Fröhlich GM , Meier P , White SK , Yellon DM , Hausenloy DJ ((2013) ) Myocardial reperfusion injury: Looking beyond primary PCI. Eur Heart J 34: , 1714–1722. |
[5] | Liang X , Huang Y , Han X ((2021) ) Associations between coronary heart disease and risk of cognitive impairment: A meta-analysis. Brain Behav 11: , e02108. |
[6] | Hooghiemstra AM , Leeuwis AE , Bertens AS , Biessels GJ , Bots ML , Brunner-La Rocca HP , Greving JP , Kappelle LJ , van Oostenbrugge RJ , van Rossum AC , van der Flier WM ((2019) ) Frequent cognitive impairment in patients with disorders along the heart-brain axis. Stroke 50: , 3369–3375. |
[7] | Bhushan S , Li Y , Huang X , Cheng H , Gao K , Xiao Z ((2021) ) Progress of research in postoperative cognitive dysfunction in cardiac surgery patients: A review article. Int J Surg 95: , 106163. |
[8] | Tang VL , Jing B , Boscardin J , Ngo S , Silvestrini M , Finlayson E , Covinsky KE ((2020) ) Association of functional, cognitive, and psychological measures with 1-year mortality in patients undergoing major surgery. JAMA Surg 155: , 412–418. |
[9] | Boone MD , Sites B , von Recklinghausen FM , Mueller A , Taenzer AH , Shaefi S ((2020) ) Economic burden of postoperative neurocognitive disorders among US Medicare patients. JAMA Netw Open 3: , e208931. |
[10] | Wang Y , Qiu G , Li Y ((2022) ) The effects of hepatic ischemia/reperfusion injury on postoperative cognitive function in aged rats. Arch Med Sci 18: , 1357–1363. |
[11] | Wu W , Wu Y , Cheng G , Zhang C , Wang H , Li Y ((2019) ) A mouse model of hepatic ischemia-reperfusion injury demonstrates potentially reversible effects on hippocampal neurons and postoperative cognitive function. Med Sci Monit 25: , 1526–1536. |
[12] | Wang YQ , Wu WW , Wang LK , Chen K , Li YH ((2014) ) Influence of hepatic ischemia-reperfusion on postoperative spatial cognitive function in mice. Genet Mol Res 13: , 5767–5777. |
[13] | Tahamtan M , Nazari A , Aghaei I , Shabani M ((2021) ) Ischemic postconditioning attenuates bilateral renal ischemia-induced cognitive impairments. Basic Clin Neurosci 12: , 789–804. |
[14] | Tahamtan M , Sheibani V , Shid Moosavi SM , Asadi-Shekaari M , Esmaeili-Mahani S , Aghaei I , Shabani M ((2018) ) Pre-treatment with erythropoietin attenuates bilateral renal ischemia-induced cognitive impairments. Iran J Pharm Res 17: , 601–612. |
[15] | Zhou J , Huang WQ , Li C , Wu GY , Li YS , Wen SH , Lei WL , Liu KX ((2012) ) Intestinal ischemia/reperfusion enhances microglial activation and induces cerebral injury and memory dysfunction in rats. Crit Care Med 40: , 2438–2448. |
[16] | Yang B , Zhang LY , Chen Y , Bai YP , Jia J , Feng JG , Liu KX , Zhou J ((2020) ) Melatonin alleviates intestinal injury, neuroinflammation and cognitive dysfunction caused by intestinal ischemia/reperfusion. Int Immunopharmacol 85: , 106596. |
[17] | Chen Y , Zhou J , Li J , Yang SB , Mo LQ , Hu JH , Yuan WL ((2012) ) Electroacupuncture pretreatment prevents cognitive impairment induced by limb ischemia-reperfusion via inhibition of microglial activation and attenuation of oxidative stress in rats. Brain Res 1432: , 36–45. |
[18] | Evonuk KS , Prabhu SD , Young ME , DeSilva TM ((2017) ) Myocardial ischemia/reperfusion impairs neurogenesis and hippocampal-dependent learning and memory. Brain Behav Immun 61: , 266–273. |
[19] | Ongnok B , Khuanjing T , Chunchai T , Kerdphoo S , Jaiwongkam T , Chattipakorn N , Chattipakorn SC ((2021) ) Donepezil provides neuroprotective effects against brain injury and Alzheimer’s pathology under conditions of cardiac ischemia/reperfusion injury. Biochim Biophys Acta Mol Basis Dis 1867: , 165975. |
[20] | Alkhouli M , Alqahtani F , Kalra A , Gafoor S , Alhajji M , Alreshidan M , Holmes DR , Lerman A ((2020) ) Trends in characteristics and outcomes of patients undergoing coronary revascularization in the United States, 2003–2016. JAMA Netw Open 3: , e1921326. |
[21] | Grant SW , Kendall S , Goodwin AT , Cooper G , Trivedi U , Page R , Jenkins DP ((2021) ) Trends and outcomes for cardiac surgery in the United Kingdom from 2002 to 2016. JTCVS Open 7: , 259–269. |
[22] | Whitlock EL , Diaz-Ramirez LG , Smith AK , Boscardin WJ , Avidan MS , Glymour MM ((2019) ) Cognitive change after cardiac surgery versus cardiac catheterization: A population-based study. Ann Thorac Surg 107: , 1119–1125. |
[23] | Whitlock EL , Diaz-Ramirez LG , Smith AK , Boscardin WJ , Covinsky KE , Avidan MS , Glymour MM ((2021) ) Association of coronary artery bypass grafting vs percutaneous coronary intervention with memory decline in older adults undergoing coronary revascularization. JAMA 325: , 1955–1964. |
[24] | Lappalainen L , Rajamaki B , Tolppanen AM , Hartikainen S ((2022) ) Coronary artery revascularizations and cognitive decline - A systematic review. Curr Probl Cardiol 47: , 100960. |
[25] | Dieleman J , Sauër AM , Klijn C , Nathoe H , Moons K , Kalkman C , Kappelle J , Van Dijk D ((2009) ) Presence of coronary collaterals isassociated with a decreased incidence of cognitive decline aftercoronary artery bypass surgery.. Eur J Cardiothorac Surg 35: , 48–53. |
[26] | Jurga J , Tornvall P , Dey L , van der Linden J , Sarkar N , von Euler M ((2016) ) Does coronary angiography and percutaneous coronary intervention affect cognitive function? . Am J Cardiol 118: , 1437–1441. |
[27] | Turer AT , Hill JA ((2010) ) Pathogenesis of myocardial ischemia-reperfusion injury and rationale for therapy. Am J Cardiol 106: , 360–368. |
[28] | Terkelsen CJ , Sørensen JT , Kaltoft AK , Nielsen SS , Thuesen L , Bøtker HE , Lassen JF ((2009) ) Prevalence and significance of accelerated idioventricular rhythm in patients with ST-elevation myocardial infarction treated with primary percutaneous coronary intervention. Am J Cardiol 104: , 1641–1646. |
[29] | Madhavan M , Graff-Radford J , Piccini JP , Gersh BJ ((2018) ) Cognitive dysfunction in atrial fibrillation. Nat Rev Cardiol 15: , 744–756. |
[30] | Rivard L , Friberg L , Conen D , Healey JS , Berge T , Boriani G , Brandes A , Calkins H , Camm AJ , Yee Chen L , Lluis Clua Espuny J , Collins R , Connolly S , Dagres N , Elkind MSV , Engdahl J , Field TS , Gersh BJ , Glotzer TV , Hankey GJ , Harbison JA , Haeusler KG , Hills MT , Johnson LSB , Joung B , Khairy P , Kirchhof P , Krieger D , Lip GYH , Løchen ML , Madhavan M , Mairesse GH , Montaner J , Ntaios G , Quinn TJ , Rienstra M , Rosenqvist M , Sandhu RK , Smyth B , Schnabel RB , Stavrakis S , Themistoclakis S , Van Gelder IC , Wang JG , Freedman B ((2022) ) Atrial fibrillation and dementia: A report from the AF-SCREEN International Collaboration. Circulation 145: , 392–409. |
[31] | Diener HC , Hart RG , Koudstaal PJ , Lane DA , Lip GYH ((2019) ) Atrial fibrillation and cognitive function: JACC Review Topic of the Week. J Am Coll Cardiol 73: , 612–619. |
[32] | Toledo C , Andrade DC , Díaz HS , Inestrosa NC , Del Rio R ((2019) ) Neurocognitive disorders in heart failure: Novel pathophysiological mechanisms underpinning memory loss and learning impairment. Mol Neurobiol 56: , 8035–8051. |
[33] | Chen S , Hua F , Lu J , Jiang Y , Tang Y , Tao L , Zou B , Wu Q ((2015) ) Effect of dexmedetomidine on myocardial ischemia-reperfusion injury. Int J Clin Exp Med 8: , 21166–21172. |
[34] | Kline R , Wong E , Haile M , Didehvar S , Farber S , Sacks A , Pirraglia E , de Leon MJ , Bekker A ((2016) ) Peri-operative inflammatory cytokines in plasma of the elderly correlate in prospective study with postoperative changes in cognitive test scores. Int J Anesthesiol Res 4: , 313–321. |
[35] | Liu X , Yu Y , Zhu S ((2018) ) Inflammatory markers in postoperative delirium (POD) and cognitive dysfunction (POCD): A meta-analysis of observational studies. PLoS One 13: , e0195659. |
[36] | Duijndam S , Denollet J , Nyklíček I , Kupper N ((2017) ) Perceived cognition after percutaneous coronary intervention: Association with quality of life, mood and fatigue in the THORESCI Study. Int J Behav Med 24: , 552–562. |
[37] | Bergh C , Bäckström M , Jönsson H , Havinder L , Johnsson P ((2002) ) In the eye of both patient and spouse: Memory is poor 1 to 2 years after coronary bypass and angioplastydiscussion. Ann Thorac Surg 74: , 689–693discussion 694. |
[38] | Ahlgren E , Lundqvist A , Nordlund A , Aren C , Rutberg H ((2003) ) Neurocognitive impairment and driving performance after coronary artery bypass surgery. Eur J Cardiothorac Surg 23: , 334–340. |
[39] | Währborg P , Booth JE , Clayton T , Nugara F , Pepper J , Weintraub WS , Sigwart U , Stables RH ((2004) ) Neuropsychological outcome after percutaneous coronary intervention or coronary artery bypass grafting: Results from the Stent or Surgery (SoS) Trial. Circulation 110: , 3411–3417. |
[40] | Keizer AM , Hijman R , Kalkman CJ , Kahn RS , van Dijk D ((2005) ) The incidence of cognitive decline after (not) undergoing coronary artery bypass grafting: The impact of a controlled definition. Acta Anaesthesiol Scand 49: , 1232–1235. |
[41] | Vedin J , Nyman H , Ericsson A , Hylander S , Vaage J ((2006) ) Cognitive function after on or off pump coronary artery bypass grafting. Eur J Cardiothorac Surg 30: , 305–310. |
[42] | Mutch WA , Fransoo RR , Campbell BI , Chateau DG , Sirski M , Warrian RK ((2011) ) Dementia and depression with ischemic heart disease: A population-based longitudinal study comparing interventional approaches to medical management. PLoS One 6: , e17457. |
[43] | Liu YH , Wang DX , Li LH , Wu XM , Shan GJ , Su Y , Li J , Yu QJ , Shi CX , Huang YN , Sun W ((2009) ) The effects of cardiopulmonary bypass on the number of cerebral microemboli and the incidence of cognitive dysfunction after coronary artery bypass graft surgery. Anesth Analg 109: , 1013–1022. |
[44] | Schwarz N , Schoenburg M , Möllmann H , Kastaun S , Kaps M , Bachmann G , Sammer G , Hamm C , Walther T , Gerriets T ((2011) ) Cognitive decline and ischemic microlesions after coronary catheterization. A comparison to coronary artery bypass grafting. Am Heart J 162: , 756–763. |
[45] | Bruce KM , Yelland GW , Smith JA , Robinson SR ((2013) ) Recovery of cognitive function after coronary artery bypass graft operations. Ann Thorac Surg 95: , 1306–1313. |
[46] | Sauër AC , Nathoe HM , Hendrikse J , Peelen LM , Regieli J , Veldhuijzen DS , Kalkman CJ , Grobbee DE , Doevendans PA , van Dijk D ((2013) ) Cognitive outcomes 7.5 years after angioplasty compared withoff-pump coronary bypass surgery. Ann Thorac Surg 96: , 1294–1300. |
[47] | Habib S , Khan A , Afridi MI , Saeed A , Jan AF , Amjad N ((2014) ) Frequency and predictors of cognitive decline in patients undergoing coronary artery bypass graft surgery. J Coll Physicians Surg Pak 24: , 543–548. |
[48] | Pérez-Belmonte LM , San Román-Terán CM , Jiménez-Navarro M , Barbancho MA , García-Alberca JM , Lara JP ((2015) ) Assessment of long-term cognitive impairment after off-pump coronary-artery bypass grafting and related risk factors. J Am Med Dir Assoc 16: , 263.e269–211. |
[49] | Xu B , Qiao Q , Chen M , Rastogi R , Luo D , Bi Q ((2015) ) Relationship between neurological complications, cerebrovascular and cerebral perfusion following off-pump coronary artery bypass grafting. Neurol Res 37: , 421–426. |
[50] | Evered LA , Silbert BS , Scott DA , Maruff P , Ames D ((2016) ) Prevalence of dementia 7.5 years after coronary artery bypass graft surgery. Anesthesiology 125: , 62–71. |
[51] | Kuźma E , Airdrie J , Littlejohns TJ , Lourida I , Thompson-Coon J , Lang IA , Scrobotovici M , Thacker EL , Fitzpatrick A , Kuller LH , Lopez OL , Longstreth WT Jr , Ukoumunne OC , Llewellyn DJ ((2017) ) Coronaryartery bypass graft surgery and dementia risk in the CardiovascularHealth Study. Alzheimer Dis Assoc Disord 31: , 120–127. |
[52] | Gu SZ , Beska B , Chan D , Neely D , Batty JA , Adams-Hall J , Mossop H , Qiu W , Kunadian V ((2019) ) Cognitive decline in older patients with non-ST elevation acute coronary syndrome. J Am Heart Assoc 8: , e011218. |
[53] | Relander K , Hietanen M , Rantanen K , Rämö J , Vento A , Saastamoinen KP , Roine RO , Soinne L ((2020) ) Postoperative cognitive change after cardiac surgery predicts long-term cognitive outcome. Brain Behav 10: , e01750. |
[54] | Tang AB , Diaz-Ramirez LG , Smith AK , Lee SJ , Whitlock EL ((2023) ) Preoperative factors predict memory decline after coronary artery bypass grafting or percutaneous coronary intervention in an epidemiological cohort of older adults. J Am Heart Assoc 12: , e027849. |
[55] | Apaijai N , Moisescu DM , Palee S , McSweeney CM , Saiyasit N , Maneechote C , Boonnag C , Chattipakorn N , Chattipakorn SC ((2019) ) Pretreatment with PCSK9 inhibitor protects the brain against cardiac ischemia/reperfusion injury through a reduction of neuronal inflammation and amyloid beta aggregation. J Am Heart Assoc 8: , e010838. |
[56] | Liao S , Luo Y , Chunchai T , Singhanat K , Arunsak B , Benjanuwattra J , Apaijai N , Chattipakorn N , Chattipakorn SC ((2022) ) An apoptosis inhibitor suppresses microglial and astrocytic activation after cardiac ischemia/reperfusion injury. Inflamm Res 71: , 861–872. |
[57] | Kumfu S , Charununtakorn ST , Jaiwongkam T , Chattipakorn N , Chattipakorn SC ((2018) ) Humanin exerts neuroprotection during cardiac ischemia-reperfusion injury. J Alzheimers Dis 61: , 1343–1353. |
[58] | Liao S , Apaijai N , Luo Y , Wu J , Chunchai T , Singhanat K , Arunsak B , Benjanuwattra J , Chattipakorn N , Chattipakorn SC ((2021) ) Cell death inhibitors protect against brain damage caused by cardiac ischemia/reperfusion injury. Cell Death Discov 7: , 312. |
[59] | Hovens IB , van Leeuwen BL , Mariani MA , Kraneveld AD , Schoemaker RG ((2016) ) Postoperative cognitive dysfunction and neuroinflammation; Cardiac surgery and abdominal surgery are not the same. Brain Behav Immun 54: , 178–193. |
[60] | Burdakov D , Peleg-Raibstein D ((2020) ) The hypothalamus as a primary coordinator of memory updating. Physiol Behav 223: , 112988. |
[61] | Feng MH , Li ZX , Wang Q , Manyande A , Li YJ , Li SY , Xu W , Xiang HB ((2020) ) Neurochemical alterations of different cerebral regions in rats with myocardial ischemia-reperfusion injury based on proton nuclear magnetic spectroscopy analysis. Aging (Albany NY) 13: , 2294–2309. |
[62] | Miller EK ((2000) ) The prefrontal cortex and cognitive control. Nat Rev Neurosci 1: , 59–65. |
[63] | Beste C , Moll CKE , Pötter-Nerger M , Münchau A ((2018) ) Striatal microstructure and its relevance for cognitive control. Trends Cogn Sci 22: , 747–751. |
[64] | Berron D , van Westen D , Ossenkoppele R , Strandberg O , Hansson O ((2020) ) Medial temporal lobe connectivity and its associations with cognition in early Alzheimer’s disease. Brain 143: , 1233–1248. |
[65] | Yuan S , Zhang X , Bo Y , Li W , Zhang H , Jiang Q ((2014) ) The effects of electroacupuncture treatment on the postoperative cognitive function in aged rats with acute myocardial ischemia-reperfusion. Brain Res 1593: , 19–29. |
[66] | Park SW , Kim M , Brown KM , D’Agati VD , Lee HT ((2011) ) Paneth cell-derived interleukin-17A causes multiorgan dysfunction after hepatic ischemia and reperfusion injury. Hepatology 53: , 1662–1675. |
[67] | Valtchanova-Matchouganska A , Gondwe M , Nadar A ((2004) ) The role of C-reactive protein in ischemia/reperfusion injury and preconditioning in a rat model of myocardial infarction. Life Sci 75: , 901–910. |
[68] | Munk PS , Breland UM , Aukrust P , Skadberg O , Ueland T , Larsen AI ((2011) ) Inflammatory response to percutaneous coronary intervention in stable coronary artery disease. J Thromb Thrombolysis 31: , 92–98. |
[69] | Solheim S , Grøgaard HK , Hoffmann P , Arnesen H , Seljeflot I ((2008) ) Inflammatory responses after percutaneous coronary intervention in patients with acute myocardial infarction or stable angina pectoris. Scand J Clin Lab Invest 68: , 555–562. |
[70] | Saleh N , Svane B , Hansson LO , Jensen J , Nilsson T , Danielsson O , Tornvall P ((2005) ) Response of serum C-reactive protein to percutaneous coronary intervention has prognostic value. Clin Chem 51: , 2124–2130. |
[71] | Zheng F , Xie W ((2018) ) High-sensitivity C-reactive protein and cognitive decline: The English Longitudinal Study of Ageing. Psychol Med 48: , 1381–1389. |
[72] | Hoth KF , Haley AP , Gunstad J , Paul RH , Poppas A , Jefferson AL , Tate DF , Ono M , Jerskey BA , Cohen RA ((2008) ) Elevated C-reactive protein is related to cognitive decline in older adults with cardiovascular disease. J Am Geriatr Soc 56: , 1898–1903. |
[73] | Joudi M , Fathi M , Harati H , Joudi M , Izanloo A , Rahdari A , Soltani G ((2014) ) Evaluating the incidence of cognitive disorder following off-pump coronary artery bypasses surgery and its predisposing factors. Anesth Pain Med 4: , e18545. |
[74] | Nurcahyo WI , Arifin A , Primatika AD , Muttaqin Z , Elfira Boom C , Harahap MS , Mochamat M , Nugroho TE , Wicaksono SA ((2021) ) An association between C-reactive protein levels and the occurrence of cognitive dysfunction after heart valve replacement. Vasc Health Risk Manag 17: , 713–720. |
[75] | Hu X , Ma R , Lu J , Zhang K , Xu W , Jiang H , Da Y ((2016) ) IL-23 promotes myocardial I/R injury by increasing the inflammatory responses and oxidative stress reactions. Cell Physiol Biochem 38: , 2163–2172. |
[76] | Shen Y , Wang Y , Li D , Wang C , Xu B , Dong G , Huang H , Jing H ((2010) ) Recombinant human erythropoietin pretreatment attenuates heart ischemia-reperfusion injury in rats by suppressing the systemic inflammatory response. Transplant Proc 42: , 1595–1597. |
[77] | Shen XN , Niu LD , Wang YJ , Cao XP , Liu Q , Tan L , Zhang C , Yu JT ((2019) ) Inflammatory markers in Alzheimer’s disease and mild cognitive impairment: A meta-analysis and systematic review of 170 studies. J Neurol Neurosurg Psychiatry 90: , 590–598. |
[78] | Zhu Y , Zhou M , Jia X , Zhang W , Shi Y , Bai S , Rampes S , Vizcaychipi MP , Wu C , Wang K , Ma D , Yang Q , Wang L ((2023) ) Inflammation disrupts the brain network of executive function after cardiac surgery. Ann Surg 277: , e689–e698. |
[79] | Kálmán J , Juhász A , Bogáts G , Babik B , Rimanóczy A , Janka Z , Penke B , Palotás A ((2006) ) Elevated levels ofinflammatory biomarkers in the cerebrospinal fluid after coronaryartery bypass surgery are predictors of cognitive decline. Neurochem Int 48: , 177–180. |
[80] | Han K , Shi D , Yang L , Wang Z , Li Y , Gao F , Liu Y , Ma X , Zhou Y ((2022) ) Prognostic value of systemic inflammatory response index in patients with acute coronary syndrome undergoing percutaneous coronary intervention. Ann Med 54: , 1667–1677. |
[81] | Huang J , Zhang Q , Wang R , Ji H , Chen Y , Quan X , Zhang C ((2019) ) Systemic immune-inflammatory index predicts clinical outcomes for elderly patients with acute myocardial infarction receiving percutaneous coronary intervention. Med Sci Monit 25: , 9690–9701. |
[82] | Wang X , Li T , Li H , Li D , Wang X , Zhao A , Liang W , Xiao R , Xi Y ((2022) ) Association of dietary inflammatory potential with blood inflammation: The prospective markers on mild cognitive impairment. Nutrients 14: , 2417. |
[83] | Esenboğa K , Kurtul A , Yamantürk YY , Tan TS , Tutar DE ((2022) ) Systemic immune-inflammation index predicts no-reflow phenomenonafter primary percutaneous coronary intervention. ActaCardiol 77: , 59–65. |
[84] | Collard CD , Gelman S ((2001) ) Pathophysiology, clinical manifestations, and prevention of ischemia-reperfusion injury. Anesthesiology 94: , 1133–1138. |
[85] | Ge Y , Ma Z , Shi H , Zhao Y , Gu X , Wei H ((2014) ) [Incidence and risk factors of postoperative cognitive dysfunction in patients underwent coronary artery bypass grafting surgery]. Zhong Nan Da Xue Xue Bao Yi Xue Ban 39: , 1049–1055. |
[86] | Kuyumcu ME , Yesil Y , Oztürk ZA , Kizilarslanoğlu C , EtgülS , Halil M , Ulger Z , Cankurtaran M , Arıoğul S ((2012) ) Theevaluation of neutrophil-lymphocyte ratio in Alzheimer’s disease. Dement Geriatr Cogn Disord 34: , 69–74. |
[87] | An P , Zhou X , Du Y , Zhao J , Song A , Liu H , Ma F , Huang G ((2019) ) Association of neutrophil-lymphocyte ratio with mild cognitive impairment in elderly Chinese adults: A case-control study. Curr Alzheimer Res 16: , 1309–1315. |
[88] | Halazun HJ , Mergeche JL , Mallon KA , Connolly ES , Heyer EJ ((2014) ) Neutrophil-lymphocyte ratio as a predictor of cognitive dysfunction in carotid endarterectomy patients. J Vasc Surg 59: , 768–773. |
[89] | Schanze N , Bode C , Duerschmied D ((2019) ) Platelet contributions to myocardial ischemia/reperfusion injury. Front Immunol 10: , 1260. |
[90] | Stangl V , Baumann G , Stangl K , Felix SB ((2002) ) Negative inotropic mediators released from the heart after myocardial ischaemia-reperfusion. Cardiovasc Res 53: , 12–30. |
[91] | Song NP , Zhen XW , Li LD , Zhong L , Wang H , An Y ((2020) ) Plasma calprotectin was associated with platelet activation and no-reflow phenomenon in acute coronary syndrome. BMC Cardiovasc Disord 20: , 443. |
[92] | Rawish E , Nording H , Münte T , Langer HF ((2020) ) Platelets as mediators of neuroinflammation and thrombosis. Front Immunol 11: , 548631. |
[93] | Stellos K , Panagiota V , Kögel A , Leyhe T , Gawaz M , Laske C ((2010) ) Predictive value of platelet activation for the rate of cognitive decline in Alzheimer’s disease patients. J Cereb Blood Flow Metab 30: , 1817–1820. |
[94] | Taggart DP , Browne SM , Wade DT , Halligan PW ((2003) ) Neuroprotection during cardiac surgery: A randomised trial of a platelet activating factor antagonist. Heart 89: , 897–900. |
[95] | Merino JG , Latour LL , Tso A , Lee KY , Kang DW , Davis LA , Lazar RM , Horvath KA , Corso PJ , Warach S ((2013) ) Blood-brain barrier disruption after cardiac surgery. AJNR Am J Neuroradiol 34: , 518–523. |
[96] | Surinkaew P , Apaijai N , Sawaddiruk P , Jaiwongkam T , Kerdphoo S , Chattipakorn N , Chattipakorn SC ((2020) ) Mitochondrial fusion promoter alleviates brain damage in rats with cardiac ischemia/reperfusion injury. J Alzheimers Dis 77: , 993–1003. |
[97] | Tan XX , Qiu LL , Sun J ((2021) ) Research progress on the role of inflammatory mechanisms in the development of postoperative cognitive dysfunction. Biomed Res Int 2021: , 3883204. |
[98] | Hovens IB , Schoemaker RG , van der Zee EA , Heineman E , Izaks GJ , van Leeuwen BL ((2012) ) Thinking through postoperative cognitive dysfunction: How to bridge the gap between clinical and pre-clinical perspectives. Brain Behav Immun 26: , 1169–1179. |
[99] | Jeon MT , Kim KS , Kim ES , Lee S , Kim J , Hoe HS , Kim DG ((2021) ) Emerging pathogenic role of peripheral blood factors following BBB disruption in neurodegenerative disease. Ageing Res Rev 68: , 101333. |
[100] | Carbone F , Bonaventura A , Montecucco F ((2019) ) Neutrophil-related oxidants drive heart and brain remodeling after ischemia/reperfusion injury. Front Physiol 10: , 1587. |
[101] | Alam A , Hana Z , Jin Z , Suen KC , Ma D ((2018) ) Surgery, neuroinflammation and cognitive impairment. EBioMedicine 37: , 547–556. |
[102] | Gabisonia K , Khan M , Recchia FA ((2022) ) Extracellular vesicle-mediated bidirectional communication between heart and other organs. Am J Physiol Heart Circ Physiol 322: , H769–h784. |
[103] | Théry C , Witwer KW , Aikawa E , Alcaraz MJ , Anderson JD , Andriantsitohaina R , Antoniou A , Arab T , Archer F , Atkin-Smith GK , et al ((2018) ) Minimal information for studies of extracellular vesicles 2018 (MISEV2018): A position statement of the International Society for Extracellular Vesicles and update of the MISEV2014 guidelines. J Extracell Vesicles 7: , 1535750. |
[104] | Tian C , Gao L , Rudebush TL , Yu L , Zucker IH ((2022) ) Extracellular vesicles regulate sympatho-excitation by Nrf2 in heart failure. Circ Res 131: , 687–700. |
[105] | Chen Z , Venkat P , Seyfried D , Chopp M , Yan T , Chen J ((2017) ) Brain-heart interaction: Cardiac complications after stroke. Circ Res 121: , 451–468. |
[106] | Wang J , Li L , Zhang Z , Zhang X , Zhu Y , Zhang C , Bi Y ((2022) ) Extracellular vesicles mediate the communication of adipose tissue with brain and promote cognitive impairment associated with insulin resistance. Cell Metab 34: , 1264–1279.e1268. |
[107] | Zhang L , Liu H , Jia L , Lyu J , Sun Y , Yu H , Li H , Liu W , Weng Y , Yu W ((2019) ) Exosomes mediate hippocampal and cortical neuronal injury induced by hepatic ischemia-reperfusion injury through activating pyroptosis in rats. Oxid Med Cell Longev 2019: , 3753485. |
[108] | Chen XD , Zhao J , Yang X , Zhou BW , Yan Z , Liu WF , Li C , Liu KX ((2021) ) Gut-derived exosomes mediate memory impairment after intestinal ischemia/reperfusion via activating microglia. Mol Neurobiol 58: , 4828–4841. |
[109] | Luo H , Li X , Li T , Zhao L , He J , Zha L , Qi Q , Yu Z ((2019) ) microRNA-423-3p exosomes derived from cardiac fibroblasts mediates the cardioprotective effects of ischaemic post-conditioning. Cardiovasc Res 115: , 1189–1204. |
[110] | Wu X , Iroegbu CD , Peng J , Guo J , Yang J , Fan C ((2021) ) Cell death and exosomes regulation after myocardial infarction and ischemia-reperfusion. Front Cell Dev Biol 9: , 673677. |
[111] | Gidlöf O , Andersson P , van der Pals J , Götberg M , Erlinge D ((2011) ) Cardiospecific microRNA plasma levels correlate with troponin and cardiac function in patients with ST elevation myocardial infarction, are selectively dependent on renal elimination, and can be detected in urine samples. Cardiology 118: , 217–226. |
[112] | Deddens JC , Vrijsen KR , Colijn JM , Oerlemans MI , Metz CH , van der Vlist EJ , Nolte-’t Hoen EN , den Ouden K , Jansen Of Lorkeers SJ , van der Spoel TI , Koudstaal S , Arkesteijn GJ , Wauben MH , van Laake LW , Doevendans PA , Chamuleau SA , Sluijter JP ((2016) ) Circulating extracellular vesicles contain miRNAs and are released as early biomarkers for cardiac injury. J Cardiovasc Transl Res 9: , 291–301. |
[113] | Ellis BW , Ronan G , Ren X , Bahcecioglu G , Senapati S , Anderson D , Handberg E , March KL , Chang HC , Zorlutuna P ((2022) ) Human Heart Anoxia and Reperfusion Tissue (HEART) model for the rapid study of exosome bound miRNA expression as biomarkers for myocardial infarction. Small 18: , e2201330. |
[114] | Emanueli C , Shearn AI , Laftah A , Fiorentino F , Reeves BC , Beltrami C , Mumford A , Clayton A , Gurney M , Shantikumar S , Angelini GD ((2016) ) Coronary artery-bypass-graft surgery increases the plasma concentration of exosomes carrying a cargo of cardiac microRNAs: An example of exosome trafficking out of the human heart with potential for cardiac biomarker discovery. PLoS One 11: , e0154274. |
[115] | Ma JC , Duan MJ , Sun LL , Yan ML , Liu T , Wang Q , Liu CD , Wang X , Kang XH , Pei SC , Zong DK , Chen X , Wang N , Ai J ((2015) ) Cardiac over-expression of microRNA-1 induces impairment of cognition in mice. Neuroscience 299: , 66–78. |
[116] | Sun LL , Duan MJ , Ma JC , Xu L , Mao M , Biddyut D , Wang Q , Yang C , Zhang S , Xu Y , Yang L , Tian Y , Liu Y , Xia SN , Li KX , Jin Z , Xiong Q , Ai J ((2018) ) Myocardial infarction-induced hippocampal microtubule damage by cardiac originating microRNA-1 in mice. J Mol Cell Cardiol 120: , 12–27. |
[117] | Duan MJ , Yan ML , Wang Q , Mao M , Su D , Sun LL , Li KX , Qu Y , Sun Q , Zhang XY , Huang SY , Ma JC , Ban T , Ai J ((2018) ) Overexpression of miR-1 in the heart attenuates hippocampal synaptic vesicle exocytosis by the posttranscriptional regulation of SNAP-25 through the transportation of exosomes. Cell Commun Signal 16: , 91. |
[118] | Martins HC , Gilardi C , Sungur A , Winterer J , Pelzl MA , Bicker S , Gross F , Kisko TM , Malikowska-Racia N , Braun MD , Brosch K , Nenadic I , Stein F , Meinert S , Schwarting RKW , Dannlowski U , Kircher T , Wöhr M , Schratt G ((2022) ) Bipolar-associated miR-499-5p controls neuroplasticity by downregulating the Cav1.2 subunit CACNB2. EMBO Rep 23: , e54420. |
[119] | Wang M , Su P , Liu Y , Zhang X , Yan J , An X , Wang X , Gu S ((2019) ) Abnormal expression of circRNA_089763 in the plasma exosomes of patients with post-operative cognitive dysfunction after coronary artery bypass grafting. Mol Med Rep 20: , 2549–2562. |
[120] | Ge X , Meng Q , Wei L , Liu J , Li M , Liang X , Lin F , Zhang Y , Li Y , Liu Z , Fan H , Zhou X ((2021) ) Myocardial ischemia-reperfusion induced cardiac extracellular vesicles harbour proinflammatory features and aggravate heart injury. J Extracell Vesicles 10: , e12072. |
[121] | Marin W , Marin D , Ao X , Liu Y ((2021) ) Mitochondria as a therapeutictarget for cardiac ischemia-reperfusion injury (Review). Int JMol Med 47: , 485–499. |
[122] | Vasam G , Nadeau R , Cadete VJJ , Lavallée-Adam M , Menzies KJ , Burelle Y ((2021) ) Proteomics characterization ofmitochondrial-derived vesicles under oxidative stress. FASEBJ 35: , e21278. |
[123] | Jiang R , Rong C , Ke R , Meng S , Yan X , Ke H , Wu S ((2019) ) Differential proteomic analysis of serum exosomes reveals alterations in progression of Parkinson disease. Medicine (Baltimore) 98: , e17478. |
[124] | Liu H , Liu X , Zhou J , Li T ((2022) ) Mitochondrial DNA is a vital driving force in ischemia-reperfusion injury in cardiovascular diseases. Oxid Med Cell Longev 2022: , 6235747. |
[125] | Picca A , Guerra F , Calvani R , Coelho-Júnior HJ , Landi F , Bernabei R , Romano R , Bucci C , Marzetti E ((2020) ) Extracellularvesicles and damage-associated molecular patterns: A pandora’s boxin health and disease. Front Immunol 11: , 601740. |
[126] | Wei X , Shao B , He Z , Ye T , Luo M , Sang Y , Liang X , Wang W , Luo S , Yang S , Zhang S , Gong C , Gou M , Deng H , Zhao Y , Yang H , Deng S , Zhao C , Yang L , Qian Z , Li J , Sun X , Han J , Jiang C , Wu M , Zhang Z ((2015) ) Cationic nanocarriers induce cell necrosis through impairment of Na(+)/K(+)-ATPase and cause subsequent inflammatory response. Cell Res 25: , 237–253. |
[127] | Zhang Q , Raoof M , Chen Y , Sumi Y , Sursal T , Junger W , Brohi K , Itagaki K , Hauser CJ ((2010) ) Circulating mitochondrial DAMPs cause inflammatory responses to injury. Nature 464: , 104–107. |
[128] | Nakahira K , Haspel JA , Rathinam VA , Lee SJ , Dolinay T , Lam HC , Englert JA , Rabinovitch M , Cernadas M , Kim HP , Fitzgerald KA , Ryter SW , Choi AM ((2011) ) Autophagy proteins regulate innate immune responses by inhibiting the release of mitochondrial DNA mediated by the NALP3 inflammasome. Nat Immunol 12: , 222–230. |
[129] | Zhou R , Yazdi AS , Menu P , Tschopp J ((2011) ) A role for mitochondria in NLRP3 inflammasome activation. Nature 469: , 221–225. |
[130] | Wilkins HM , Koppel SJ , Weidling IW , Roy N , Ryan LN , Stanford JA , Swerdlow RH ((2016) ) Extracellular mitochondria and mitochondrial components act as damage-associated molecular pattern molecules in the mouse brain. J Neuroimmune Pharmacol 11: , 622–628. |
[131] | Tian Y , Charles EJ , Yan Z , Wu D , French BA , Kron IL , Yang Z ((2019) ) The myocardial infarct-exacerbating effect of cell-free DNA is mediated by the high-mobility group box 1-receptor for advanced glycation end products-Toll-like receptor 9 pathway. J Thorac Cardiovasc Surg 157: , 2256–2269e2253. |
[132] | Zhao X , Han J , Zhou L , Zhao J , Huang M , Wang Y , Kou J , Kou Y , Jin J ((2023) ) High mobility group box 1 derived mainly from platelet microparticles exacerbates microvascular obstruction in no reflow. Thromb Res 222: , 49–62. |
[133] | Pan XC , Li ZX , Wu DZ , Li SY , Xiang HB , Song YT ((2019) ) Mapping changes of whole brain blood flow in rats with myocardial ischemia/reperfusion injury assessed by positron emission tomography. Curr Med Sci 39: , 653–657. |
[134] | Ovsenik A , Podbregar M , Fabjan A ((2021) ) Cerebral blood flow impairment and cognitive decline in heart failure. Brain Behav 11: , e02176. |
[135] | Leeuwis AE , Hooghiemstra AM , Bron EE , Kuipers S , Oudeman EA , Kalay T , Brunner-La Rocca HP , Kappelle LJ , van Oostenbrugge RJ , Greving JP , Niessen WJ , van Buchem MA , van Osch MJP , van Rossum AC , Prins ND , Biessels GJ , Barkhof F , van der Flier WM ((2020) ) Cerebral blood flow and cognitive functioning in patients with disorders along the heart-brain axis: Cerebral blood flow and the heart-brain axis. , e. Alzheimers Dement (N Y) 6: , 12034. |
[136] | Chernov VI , Efimova NY , Efimova IY , Akhmedov SD , Lishmanov YB ((2006) ) Short-term and long-term cognitive function and cerebral perfusion in off-pump and on-pump coronary artery bypass patients. Eur J Cardiothorac Surg 29: , 74–81. |
[137] | Efimova N , Chernov V , Efimova I , Akhmedov S , Lishmanov Y ((2015) ) [Changes in cerebral blood flow and cognitive function in patients undergoing coronary bypass surgery with cardiopulmonary bypass]. Kardiologiia 55: , 40–46. |
[138] | Abildstrom H , Høgh P , Sperling B , Moller JT , Yndgaard S , Rasmussen LS ((2002) ) Cerebral blood flow and cognitive dysfunction after coronary surgery. Ann Thorac Surg 73: , 1174–1178discussion 1178-1179. |
[139] | Roberts RO , Knopman DS , Geda YE , Cha RH , Roger VL , Petersen RC ((2010) ) Coronary heart disease is associated with non-amnestic mild cognitive impairment. Neurobiol Aging 31: , 1894–1902. |
[140] | Colak Z , Borojevic M , Bogovic A , Ivancan V , Biocina B , Majeric-Kogler V ((2015) ) Influence of intraoperative cerebral oximetry monitoring on neurocognitive function after coronary artery bypass surgery: A randomized, prospective study. Eur J Cardiothorac Surg 47: , 447–454. |
[141] | de Tournay-Jetté E , Dupuis G , Bherer L , Deschamps A , Cartier R , Denault A ((2011) ) The relationship between cerebral oxygen saturationchanges and postoperative cognitive dysfunction in elderly patientsafter coronary artery bypass graft surgery. J Cardiothorac VascAnesth 25: , 95–104. |
[142] | Slater JP , Guarino T , Stack J , Vinod K , Bustami RT , Brown JM , 3rd , Rodriguez AL , Magovern CJ , Zaubler T , Freundlich K , Parr GV ((2009) ) Cerebral oxygen desaturation predicts cognitive decline and longer hospital stay after cardiac surgery. Ann Thorac Surg 87: ,36–44discussion 44-35. |
[143] | Eertmans W , De Deyne C , Genbrugge C , Marcus B , Bouneb S , Beran M , Fret T , Gutermann H , Boer W , Vander Laenen M , Heylen R , Mesotten D , Vanelderen P , Jans F ((2020) ) Association between postoperative delirium and postoperative cerebral oxygen desaturation in older patients after cardiac surgery. Br J Anaesth 124: , 146–153. |
[144] | Van Dijk D , Jansen EW , Hijman R , Nierich AP , Diephuis JC , Moons KG , Lahpor JR , Borst C , Keizer AM , Nathoe HM , Grobbee DE , De Jaegere PP , Kalkman CJ ((2002) ) Cognitive outcome after off-pump and on-pump coronary artery bypass graft surgery: A randomized trial. Jama 287: , 1405–1412. |
[145] | Manolis TA , Manolis AA , Apostolopoulos EJ , Melita H , Manolis AS ((2020) ) Atrial fibrillation and cognitive impairment: An associated burden or burden by association? Angiology 71: , 498–519. |
[146] | Berger M , Terrando N , Smith SK , Browndyke JN , Newman MF , Mathew JP ((2018) ) Neurocognitive function after cardiac surgery: From phenotypes to mechanisms. Anesthesiology 129: , 829–851. |
[147] | Murry CE , Jennings RB , Reimer KA ((1986) ) Preconditioning with ischemia: A delay of lethal cell injury in ischemic myocardium. Circulation 74: , 1124–1136. |
[148] | Donato M , Bin EP , V DA , Gelpi RJ ((2021) ) Myocardial remote ischemic preconditioning: From cell biology to clinical application. Mol Cell Biochem 476: , 3857–3867. |
[149] | Thielmann M , Kottenberg E , Boengler K , Raffelsieper C , Neuhaeuser M , Peters J , Jakob H , Heusch G ((2010) ) Remote ischemic preconditioning reduces myocardial injury after coronary artery bypass surgery with crystalloid cardioplegic arrest. Basic Res Cardiol 105: , 657–664. |
[150] | Hausenloy DJ , Mwamure PK , Venugopal V , Harris J , Barnard M , Grundy E , Ashley E , Vichare S , Di Salvo C , Kolvekar S , Hayward M , Keogh B , MacAllister RJ , Yellon DM ((2007) ) Effect of remote ischaemic preconditioning on myocardial injury in patients undergoing coronary artery bypass graft surgery: A randomised controlled trial. Lancet 370: , 575–579. |
[151] | Heusch G , Bøtker HE , Przyklenk K , Redington A , Yellon D ((2015) ) Remote ischemic conditioning. J Am Coll Cardiol 65: , 177–195. |
[152] | Xu T , Gong Z , Zhu WZ , Wang JF , Li B , Chen F , Deng XM ((2011) ) Remote ischemic preconditioning protects neurocognitive function of rats following cerebral hypoperfusion. Med Sci Monit 17: , Br299–304. |
[153] | Li Y , Huang P , Huang J , Zhong Z , Zhou S , Dong H , Xie J , Wu Y , Li P ((2022) ) Remote ischemic preconditioning improves cognitive control in healthy adults: Evidence from an event-related potential study. Front Neurosci 16: , 936975. |
[154] | Hudetz JA , Patterson KM , Iqbal Z , Gandhi SD , Pagel PS ((2015) ) Remote ischemic preconditioning prevents deterioration of short-term postoperative cognitive function after cardiac surgery using cardiopulmonary bypass: Results of a pilot investigation. J Cardiothorac Vasc Anesth 29: , 382–388. |
[155] | Gasparovic H , Kopjar T , Rados M , Anticevic A , Rados M , Malojcic B , Ivancan V , Fabijanic T , Cikes M , Milicic D , Gasparovic V , Biocina B ((2019) ) Impact of remote ischemic preconditioning preceding coronary artery bypass grafting on inducing neuroprotection. J Thorac Cardiovasc Surg 157: , 1466–1476.e1463. |
[156] | Zhu S , Zheng Z , Lv W , Ouyang P , Han J , Zhang J , Dong H , Lei C ((2022) ) Neuroprotective effect of remote ischemic preconditioning in patients undergoing cardiac surgery: A randomized controlled trial. Front Cardiovasc Med 9: , 952033. |
[157] | Meybohm P , Renner J , Broch O , Caliebe D , Albrecht M , Cremer J , Haake N , Scholz J , Zacharowski K , Bein B ((2013) ) Postoperative neurocognitive dysfunction in patients undergoing cardiac surgery after remote ischemic preconditioning: A double-blind randomized controlled pilot study. PLoS One 8: , e64743. |
[158] | Joung KW , Rhim JH , Chin JH , Kim WJ , Choi DK , Lee EH , Hahm KD , Sim JY , Choi IC ((2013) ) Effect of remote ischemic preconditioning on cognitive function after off-pump coronary artery bypass graft: A pilot study. Korean J Anesthesiol 65: , 418–424. |
[159] | Meybohm P , Kohlhaas M , Stoppe C , Gruenewald M , Renner J , Bein B , Albrecht M , Cremer J , Coburn M , Schaelte G , Boening A , Niemann B , Sander M , Roesner J , Kletzin F , Mutlak H , Westphal S , Laufenberg-Feldmann R , Ferner M , Brandes IF , Bauer M , Stehr SN , Kortgen A , Wittmann M , Baumgarten G , Meyer-Treschan T , Kienbaum P , Heringlake M , Schoen J , Treskatsch S , Smul T , Wolwender E , Schilling T , Fuernau G , Bogatsch H , Brosteanu O , Hasenclever D , Zacharowski K ((2018) ) RIPHeart (Remote Ischemic Preconditioning for Heart Surgery) study: Myocardial dysfunction, postoperative neurocognitive dysfunction, and 1 year follow-up. J Am Heart Assoc 7: , e008077. |
[160] | Lang JA , Kim J ((2022) ) Remote ischaemic preconditioning - translating cardiovascular benefits to humans. J Physiol 600: , 3053–3067. |
[161] | Kottenberg E , Thielmann M , Bergmann L , Heine T , Jakob H , Heusch G , Peters J ((2012) ) Protection by remote ischemic preconditioning during coronary artery bypass graft surgery with isoflurane but not propofol –a clinical trial. Acta Anaesthesiol Scand 56: , 30–38. |
[162] | Kottenberg E , Musiolik J , Thielmann M , Jakob H , Peters J , Heusch G ((2014) ) Interference of propofol with signal transducer and activator of transcription 5 activation and cardioprotection by remote ischemic preconditioning during coronary artery bypass grafting. J Thorac Cardiovasc Surg 147: , 376–382. |
[163] | Butz M , Meyer R , Gerriets T , Sammer G , Doerr JM , El-Shazly J , Doeppner TR , Choi YH , Schoenburg M , Juenemann M ((2022) ) Increasing preoperative cognitive reserve to prevent postoperative delirium and postoperative cognitive decline in cardiac surgical patients (INCORE): Study protocol for a randomized clinical trial on cognitive training. Front Neurol 13: , 1040733. |
[164] | Larsen MH , Draegert C , Vedel AG , Holmgaard F , Siersma V , Nilsson JC , Rasmussen LS ((2020) ) Long-term survival and cognitive function according to blood pressure management during cardiac surgery. A follow-up. Acta Anaesthesiol Scand 64: , 936–944. |
[165] | Glumac S , Kardum G , Sodic L , Bulat C , Covic I , Carev M , Karanovic N ((2021) ) Longitudinal assessment of preoperative dexamethasone administration on cognitive function after cardiac surgery: A 4-year follow-up of a randomized controlled trial. BMC Anesthesiol 21: , 129. |
[166] | Siepe M , Pfeiffer T , Gieringer A , Zemann S , Benk C , Schlensak C , Beyersdorf F ((2011) ) Increased systemic perfusion pressure during cardiopulmonary bypass is associated with less early postoperative cognitive dysfunction and delirium. Eur J Cardiothorac Surg 40: , 200–207. |
[167] | Jiang Y , Fang P , Shang Z , Zhu W , Gao S , Liu X ((2023) ) Cognitive training in surgical patients: A systematic review and meta-analysis. Anesthesiol Perioperative Sci 1: , 18. |
[168] | Schricker T , Sato H , Beaudry T , Codere T , Hatzakorzian R , Pruessner JC ((2014) ) Intraoperative maintenance of normoglycemia with insulin and glucose preserves verbal learning after cardiac surgery. PLoS One 9: , e99661. |
[169] | Vu T , Smith JA ((2022) ) An update on postoperative cognitive dysfunction following cardiac surgery. Front Psychiatry 13: , 884907. |
[170] | Leech T , Apaijai N , Palee S , Higgins LA , Maneechote C , Chattipakorn N , Chattipakorn SC ((2020) ) Acute administration of metformin prior to cardiac ischemia/reperfusion injury protects brain injury. Eur J Pharmacol 885: , 173418. |
[171] | Benjanuwattra J , Apaijai N , Chunchai T , Kerdphoo S , Jaiwongkam T , Arunsak B , Wongsuchai S , Chattipakorn N , Chattipakorn SC ((2020) ) Metformin preferentially provides neuroprotection following cardiac ischemia/reperfusion in non-diabetic rats. Biochim Biophys Acta Mol Basis Dis 1866: , 165893. |
[172] | Zhu J , Jiang X , Shi E , Ma H , Wang J ((2009) ) Sevoflurane preconditioning reverses impairment of hippocampal long-term potentiation induced by myocardial ischaemia-reperfusion injury. Eur J Anaesthesiol 26: , 961–968. |
[173] | Kanbak M , Saricaoglu F , Akinci SB , Oc B , Balci H , Celebioglu B , Aypar U ((2007) ) The effects of isoflurane, sevoflurane, and desflurane anesthesia on neurocognitive outcome after cardiac surgery: A pilot study. Heart Surg Forum 10: , E36–41. |
[174] | Schoen J , Husemann L , Tiemeyer C , Lueloh A , Sedemund-Adib B , Berger KU , Hueppe M , Heringlake M ((2011) ) Cognitive function after sevoflurane- vs propofol-based anaesthesia for on-pump cardiac surgery: A randomized controlled trial. Br J Anaesth 106: , 840–850. |
[175] | Shi J , Zou X , Jiang K , Wang F ((2020) ) SIRT1 mediates improvement of cardiac surgery-induced postoperative cognitive dysfunction via the TLR4/NF-κB pathway. World J Biol Psychiatry 21: , 757–765. |
[176] | Palotás A , Reis HJ , Bogáts G , Babik B , Racsmány M , Engvau L , Kecskeméti E , Juhász A , Vieira LB , Teixeira AL , Mukhamedyarovi MA , Rizvanov AA , Yalvaç ME , Guimaràes MM , Ferreira CN , Zefirov AL , Kiyasov AP , Wang L , Janka Z , Kálmán J ((2010) ) Coronary artery bypass surgery provokes Alzheimer’sdisease-like changes in the cerebrospinal fluid. J Alzheimers Dis 21: , 1153–1164. |
[177] | Marinescu MC , Lazar AL , Marta MM , Cozma A , Catana CS ((2022) ) Non-coding RNAs: Prevention, diagnosis, and treatment in myocardial ischemia-reperfusion injury. Int J Mol Sci 23: , 2728. |
[178] | de Abreu RC , Fernandes H , da Costa Martins PA , Sahoo S , Emanueli C , Ferreira L ((2020) ) Native and bioengineered extracellular vesicles for cardiovascular therapeutics. Nat Rev Cardiol 17: , 685–697. |
[179] | Zhang H , Lu J , Liu J , Zhang G , Lu A ((2020) ) Advances in the discovery of exosome inhibitors in cancer. J Enzyme Inhib Med Chem 35: , 1322–1330. |