The Effects of Medium Chain Triglyceride for Alzheimer’s Disease Related Cognitive Impairment: A Systematic Review and Meta-Analysis
Abstract
Background:
The current lack of effective drug therapies for Alzheimer’s disease (AD) has prompted researchers to seek alternative nutritional therapies, such as medium chain triglycerides (MCTs). However, results are inconclusive.
Objective:
This systematic review and meta-analysis aims to summarize current evidence on the effect of MCT on cognitive function in patients with mild cognitive impairment (MCI) or AD.
Methods:
A systematic search was conducted up until December 16, 2022, to identify human interventions reporting the effects of MCT on cognitive functioning of MCI or AD patients. 995 non-duplicated publications were identified, of which nine (n = 10 studies) met the inclusion criteria.
Results:
Meta-analysis showed cognitive improvements in general (SMD = 0.64; 95% CI [0.05, 1.24]), but not in memory, language, and attention domains after oral MCT administration, compared to placebo. The effect of MCT was greater among APOE ɛ4 (−) subjects than APOE ɛ4 (+) subjects (SMD = 1.87; 95% CI [0.35, 3.40]).
Conclusion:
This review provides some evidence that treatment with MCT could improve general cognitive function in APOE ɛ4 (−) cognitive impaired patients. Better characterized clinical studies are warranted before making a definitive conclusion on the use of MCT for MCI and AD management.
INTRODUCTION
The global population is aging rapidly, leading to a rise in global epidemic of age-related diseases. One condition of great concern is dementia. Dementia is a term that is used to describes neurocognitive conditions that involve decline in cognition in one or more cognitive domains as outlined in Diagnostic and Statistical Manual of Mental Disorders (DSM) [1]. AD is the most common form of dementia worldwide, and the risk increases by two times every five years after the age of 65 [2]. One of the early symptoms of AD is the loss of short-term memory, follow by gradual decline in other cognitive domains leading to the eventual loss of functional abilities in patients [3]. Mild cognitive impairment (MCI) is a transitional stage between the expected cognitive decline that happens with age and the more serious decline seen in AD [4]. People with MCI are at a higher risk of developing AD or a related dementia [5]. AD continues to be a health challenge worldwide as the cause appears to be multifactorial, influenced by an interplay of environmental, genetics and lifestyle factors. Most drug therapies solely provide symptomatic relief or delay disease progression, and none has shown to improve cognition in AD patients. Hence, many researchers have shifted attention towards the use of nutritional therapy such as medium chain triglyceride (MCT).
Treatment of AD requires an understanding of the underlying pathogenesis. It has been shown that compared to a healthy brain, an AD brain has reduced efficacy of glucose uptake and glycolysis, which are essential to provide energy for the basic functions of the brain [6]. This leads to a state of hypometabolism, which results in a domino effect of an increased production of reactive oxidative species, apoptosis of neuronal cells and subsequent oxidative damage [7, 8]. Oxidative damage is further exacerbated by neuroinflammation of microglia, triggered by deposition of extracellular amyloid-β (Aβ) and intracellular tau protein [9]. The summary of these mechanisms is shown in Fig. 1.
Fig. 1
Mechanistic pathways contributing to the development of AD. Created with MedPeer. (http://www.medpeer.cn).
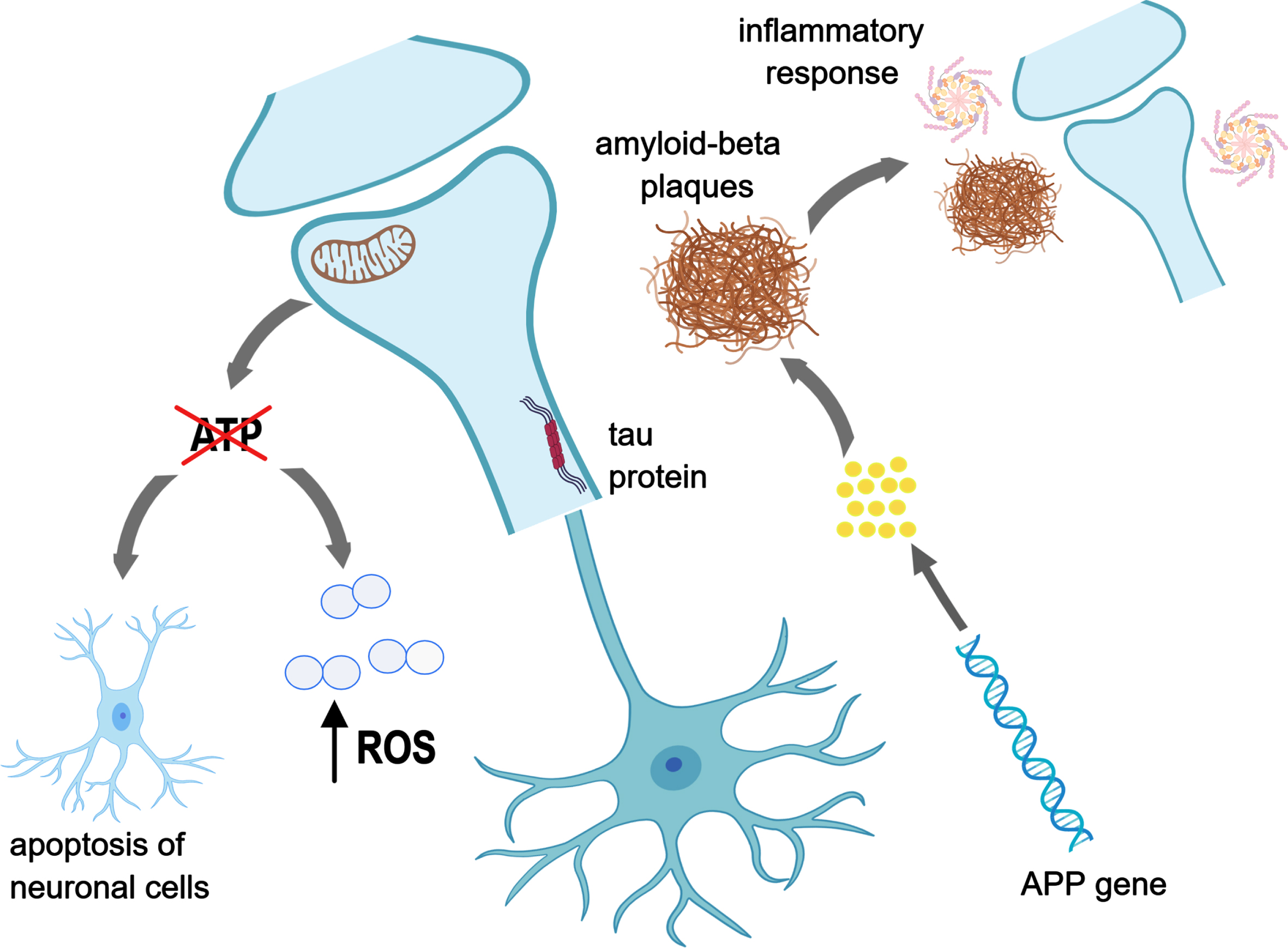
In the absence of glucose, ketone bodies are the alternative energy source for the brain [10]. Ketone bodies are able to cross the blood-brain barrier through monocarboxylate transporters in endothelial cells and astroglia [11], and provide a source of energy for the brain cells [12]. Ketone bodies namely β-hydroxybutyrate (β-HB), acetoacetate and acetone [13] are normally derived from fat stores. Some studies have shown that the overall decline of brain energy metabolism in MCI and AD patients is mainly due to glucose hypometabolism, while brain ketone metabolism remains unwounded [14, 15]. Supplementation with ketone bodies can increase brain β-HB concentrations [16].
MCTs are a source of ketone bodies and are hydrolyzed to produce medium chain fatty acids (MCFAs) by the enzyme lipase [17]. MCFAs are absorbed and metabolized faster than long-chain fatty acids [18], as it is not dependent on the transporter protein for mitochondrial entry [19]. MCTs can be obtained from diet or exogenous supplementation [20]. Multiple studies have suggested that increased ketone levels, obtained through the consumption of MCT supplementation or coconut oil (CO), may provide an alternative energy source in AD, and alleviate the severity of symptoms in patients [21–23].
Despite the potential benefits of MCT in patients with AD or those at higher risk of AD (i.e., MCI individuals), the use of MCT remains controversial due to the concern of increased cardiovascular disease risk. MCTs are a type of saturated fat, and intakes of saturated fats have been shown to increase total cholesterol and LDL cholesterol levels [24]. Thus, the potential harmful health effects on chronic consumption of MCT remain a concern for its use in the treatment of AD.
AD and associated cognitive impairment negatively impact on the quality of life of afflicted individuals, takes an emotional toll on caregivers and increases economic burden [25]. With an increasing AD patient population, an efficacious and safe therapy for the management of AD is therefore crucial. While clinical trials have been conducted to explore the potential use of MCT for improvement of cognitive functioning in MCI and AD patients, results have been controversial and inconclusive. Of knowledge, two systematic reviews have attempted to summarize the overall effect of MCT on cognition. Avgerinos et al. [26], with literature search up to March, 2019, concluded that MCTs may improve cognition in patients with MCI and AD. Conversely, Castro et al. [18] with literature search up to January 2021, reported otherwise. The discrepancy in findings could be due to the inclusion of different studies. Moreover, previous reviews did not summarize the effect of MCT on individual domains of cognitive function, which have clinical importance. Therefore, the current systematic review and meta-analysis aims to provide the most up-to-date evidence from human clinical studies on the effect of MCT on different cognitive domains of MCI and AD patients; and further investigate on the efficacy of MCT in patients with different APOE ɛ4 status.
METHODS
Search strategy
Comprehensive search was conducted in PubMed, Cochrane Library, EMBASE, Scopus, and Web of Science. Relevant studies published up to December 16, 2022, were identified. No exclusions were made on the language of the studies, and studies written in other languages were translated when required. The two key concepts of AD and MCT were searched for using index terms and keywords. Index terms were standardized across the databases. Medical Subject Headings (MeSH) were used in PubMed and Cochrane Library, while MeSH terms were mapped to Emtree in Embase. Keywords such as “dementia”, “Alzheimer”, “ketone bodies”, “medium chain triglycerides”, “coconut”, and “coconut oil” were also included in the search strategy. Boolean operators such as ‘or’ were used to combine index terms and keywords of the same concepts while ‘and’ was used for different concepts. The detailed search strategy can be found in Supplementary Table 1.
Study selection
Studies obtained from the electronic database search were managed using the reference manager EndNote. After removing duplicates, studies were screened based on the title and abstract by two independent reviewers (LS and KXY) to exclude irrelevant studies. The full text of the remaining studies was obtained and further evaluated for eligibility by the same independent reviewers. Any discrepancies on the studies to be included in the review were discussed before seeking the opinion of a third independent reviewer (FL).
Inclusion criteria
Studies included met the inclusion criteria according to the PICOs as follows: P: human subjects with MCI and AD (including borderline, mild, moderate, or severe AD); I: oral administration of MCT or CO; C: compared with control or placebo; O: improvement in cognitive functions. Subjects on a stable dose of currently approved AD medications, with no changes to dosing throughout the follow-up period of the study, were also included. Cognitive function scored using any cognitive function assessments were accepted.
Exclusion criteria
Study exclusion criteria were as follows: 1) review articles, pilot studies, case reports, observational studies and conference abstracts; 2) studies that did not involve humans; 3) studies with incomplete or unpublished data; 4) non-oral administration of MCT or CO; or 5) analysis of the role of a ketogenic diet on AD. References of review articles were hand-searched for additional studies not identified by electronic database search.
Quality assessment
Methodological quality of included studies was assessed qualitatively by two independent reviewers (LS and KXY) using relevant tools. Discrepancies were first discussed to reach consensus before seeking the opinion of a third independent reviewer (FL). Randomized controlled trials (RCTs) were assessed based on the Cochrane’s Risk of Bias Tool on five primary domains (selection, performance, attrition, reporting, and others).
Open label clinical trials and single-arm studies were assessed using the Newcastle-Ottawa Assessment for cohort studies on three primary domains (selection, comparability, and outcome). For each item in the selection and outcome domains, a maximum of one star can be awarded. In the outcome domain, the acceptable length of follow up period is 90 days [27] and subjects lost to follow-up should be less than 5% [28], where reasons accounting for the loss to follow-up should be stated. In the comparability domain, a maximum of two stars can be given for each item. The Newcastle-Ottawa scale is then converted to AHRQ standards [29] (good, fair, and poor) based on the total number of stars scored from all individual domains.
Data extraction
Two independent reviewers (LS and KXY) extracted the data from included studies, and conflicts were worked out via discussion. If a conflict remained unresolved, a third reviewer (FL) was included. Basic information extracted from each study included: author, year of publication, number of subjects (n) in treatment and control group, duration of follow up, characteristics of subjects (age, gender, and disease state), intervention used, and time point measurement of cognitive function. Other data extracted included the assessments used for cognitive function measurement and results of the assessments. Primary outcomes were domains of cognition function, such as general, memory, language, and attention domains. Secondary outcomes were change in triglyceride and cholesterol levels from baseline, and adverse events related to MCT or CO consumption. In the case of a cross-over study, only the first phase was used to prevent carry-over effect.
Data extracted for meta-analysis were the mean and standard deviation (SD) of change (post-intervention minus pre-intervention) in cognitive function from baseline. In situations where required data were not reported, an attempt was made to contact the study authors via email. However, in the events that replies were not received and a graph was used to display the results obtained, values were estimated off the graph. Standard error mean (SEM) is converted to SD using the following formula:
Meta-analysis
Meta-analysis was conducted using Review Manager (RevMan) [mac OS], Version 5.4, The Cochrane Collaboration, 2020, to obtain a quantitative summary of the effect of MCT on cognitive function in AD subjects. Analysis was conducted to estimate between group effect size by comparing the change in cognitive function score before and after treatment between the treatment and control group. A secondary meta-analysis was also conducted to investigate if the effect of MCT on cognitive function was impacted by the apolipoprotein E ɛ4 allele (APOE ɛ4) status of the subjects.
The heterogeneity between studies were tested using Cochrane’s Q and I2 test. A p-value less than 0.10 from Cochrane Q’s test indicates significant statistical heterogeneity; an I2 value of 0% indicates no heterogeneity, and a higher I2 value indicates increasing heterogeneity between studies employed in the meta-analysis. A random effect model was used if I2 > 50%. A random effect model allowed accommodation of studies that differed largely in various aspects, including the interventions used (MCT and CO) and characteristics of subjects (age, gender, and disease state) [30].
In order to compare studies that employed different assessment tools for the measurement of cognitive function in the meta-analysis, standardized mean difference (SMD) was used to estimate the effect size [31]. SMD was determined using the extracted mean and standard deviation of the change in cognitive function tests score from baseline, as well as the number of subjects in the treatment/control group. For assessment tools using scales of different direction, the data with negative mean values were multiplied by –1 to ensure all scales used for comparison were in the same direction. The effect size was interpreted based on the criteria set by Cohen, where SMD < 0.5 represents a small effect size, SMD < 0.8 represents a medium effect size and SMD > 0.8 represents a large effect size [32]. The SMD was considered significant, favoring effect of treatment when p < 0.05 [33].
RESULTS
Search results
A total of 1862 publications were identified through the electronic database search. After removing duplicates (n = 867) and irrelevant topics (n = 957), 38 studies remained for full text assessment. Two additional publications were identified through citation searching. Altogether, nine publications, with total 10 studies, were eligible for inclusion into the systematic review. Among these, four were included in the meta-analyses. The process for study selection is summarized in Supplementary Figure 1.
Study characteristics
A total of 10 clinical trials (848 subjects) studying the effect of MCT (n = 8) and CO (n = 2) on cognitive function in MCI and AD subjects were eligible for inclusion. A summary of the included clinical trials is in Table 1. Of the 10 clinical trials included, three were open-label clinical trials [23, 34, 35] and two were single-arm design [23, 34]. Ota et al. [23] contributed to two studies, one RCT and one single-arm trial. All trials included subjects of both male and female gender with different stages of cognitive impairment. Duration of follow-up ranged from 120 minutes to 15 months. Most studies employed Mini Mental State Examination (MMSE), Montreal Cognitive Assessment (MoCA), and/or Alzheimer’s Disease Assessment Scale-Cognitive Subscale (ADAS-Cog), which are tools commonly used in clinical settings, for the measurement of general cognitive function (Supplementary Table 2, description of general cognitive function test) [36]. An increase in MMSE and MoCA score while a decrease in ADAS-Cog score reflects an improvement in cognitive function. Three studies [34, 37, 38] administered CO and eight studies [23, 27, 35, 39, 40] administered MCT to the treatment group. Studies that involved MCT treatment used either caprylic acid (n = 4), a mixture of caprylic acid and capric acid (n = 3), or a non-specified MCT (n = 1). All studies conducted on CO used extra virgin coconut oil. Different doses of MCT and CO were used in each study. All studies administered the cognitive function assessments at baseline and post-treatment.
Table 1
Summary of included studies
Author (Publication Year); country | Study design | Sample size and subject characteristics | Intervention | Duration of follow-up | Cognitive function assessments | Results |
Ota et al. (2019) [23] (Postprandial); Japan | RCT | N = 20 Age = 73.40±6.0, Male = 55% Mild-moderate AD | I: MCTs (10.9 g caprylic acid and 3.5 g capric acid) Dosage: 20 g administered in conjunction with their daily diet. P: Isocaloric placebo formula containing emulsified LCTs | 120 min | Memory: WMS-R (logical memory - immediate and delayed, digital span and spatial span) Attention: WAIS-III (block design and digit symbol coding), Stroop Test and TMT. | No significant difference between scores after taking the ketogenic formula and those after the placebo formula in any cognitive test. |
Ota et al. (2019) [23] (chronic feeding); Japan | Open-label CT, single-arm | N = 16 Age = 73.40±6.0,Male = 55% Mild-moderate AD | I: MCTs (caprylic acid and capric acid) Dosage: 20 g administered in conjunction with their daily diet. C: Baseline | 12 weeks | Memory: WMS-R (logical memory - immediate and delayed, digital span and spatial span) Attention: WAIS-III (block design and digit symbol coding), Stroop Test and TMT. | Memory: significant improvements in the logical memory (immediate and delayed) test score post-treatment (p = 0.001 and p = 0.005). Attention: significant improvements in the digit-symbol coding test (p = 0.004) |
Henderson et al. (2009) [27]; USA | RCT | 152(I:86, P:66)Age = 76.9±8.9; 76.8±7.4Male = 41.7%; 47% Mild-moderate AD | I: AC-1202 (10 grams caprylic acid per 30 g sachet) Dosage: 30 g daily for the first seven days. Subsequently increased to two 30 g sachets till day 90.P: isocaloric to treatment (51% gum acacia, 37% dextrose, 10% safflower oil, and 2% syloid) | 90 days + 2 weeks washout | General: MMSE, ADAS-Cog and ADCS-CGIC APOE ɛ4: ADAS-Cog | General: Improvement was observed in ADAS-Cog score post-treatment, when compared to the placebo group (–0.31 vs 1.23), but was not significant (p = 0.077).APOE ɛ4: APOE-ɛ4 (−) patients had significantly better improvement on the ADAS-Cog relative to Placebo (p = 0.0148), whereas APOE ɛ4 (+) patients showed no significant changes in ADAS-Cog post-treatment. |
Gandotra et al. (2014) [34]; Netherlands | Open-label CT, single-arm | N = 31Age = 81.9±9.63 Male = 32.3% Moderate (54.8%) and severe (41.9%)AD | I: 20 g of virgin coconut oil daily after breakfast during the first 4 weeks. | 4 weeks + 2 weeks washout | General: ADAS-Cog | General: Improvement observed in ADAS-Cog score post-treatment was significant (p = 0.00). |
Juby et al. (2022) [35]; Canada | RCT, crossover study with an open label extension | N = 20 (I:10; P:10) Age = 75.7±8.8; 68.4±9.9 Male = 40%; 70% Mild-moderate AD | I: Bulletproof Brain Octane (100% caprylic acid triglycerides, MCT, from coconut oil) Dosage: 15 ml once daily to three times daily by week three, if clinically tolerated, or to the maximum tolerated dose. P: Olive oil | 8 months (crossover at 4 months) + 7 months extension with MCT treatment | General: Cognigram tests (1 and 2), MMSE and MoCA | General: a statistically significant difference in Cognigram 1 scores between the two groups at the study completion (p = 0.003), occurred in those who has started with the placebo oil (i.e., subjects who had 11 months of uninterrupted MCT treatment. No statistically significant difference in MMSE and MoCA between the two groups. |
Ortí et al. (2017) [37]; Spain | RCT | N = 44 (I:22, C:22) Age = 79.32±4.47 Male = 18.2% Mild-moderate (36.36%) and severe (63.64%) AD | I: Extra virgin coconut oil Dosage: 20 ml two times a day (breakfast and lunch) | 21 days | General: MEC-Lobo (orientation, calculus-concentration, fixation, memory and language-construction) | General: Improvement observed in MEC-Lobo score post-treatment was significant (p < 0.001). Memory: Improvement was observed in the MEC-Lobo memory subtest score post-treatment though not significant (p = 0.312).Language: Improvement was observed in MEC-Lobo language construction subtest score post-treatment (p = 0.003) Attention: Improvement was observed in MEC-Lobo calculus concentration subtest score post-treatment though not significant(p = 0.961) |
Fortier et al. (2021) [39]; Canada | RCT | N = 83 (I:39; P:44) Age = 71.4±7.2; 72.9±6.9 Male = 51.3%; 39.5% MCI | I: Emulsion made up of 12% Captext 355 (60% caprylic acid, 40% capric acid) in lactose-free skim milk. Dosage: twice a day in equal volumes between two meals. The initial dose of 50 ml/day was increased gradually to the final dose of 250 ml/day within two weeks. P: High-oleic acid sunflower oil emulsified in lactose-free skim milk | 6 months | Memory: RL/RI-16, BVMT-R, and VFT Language: BNT Attention: TMT, Stroop Colour and Word Interference (Stroop), and Digit Symbol Substitution Test | Memory: the raw scores of the first free recall trial of the RL/RI-16 test improved significantly in the kMCT group (p = 0.047). No significant changes were observed in either group on the BVMT-R. Language: BNT scores improved post-intervention in the kMCT group with +1.1 total correct responses, while the placebo group had 0.2 fewer total correct responses (p = 0.018) Attention: the kMCT group had significantly fewer errors on all conditions of the TMT (p = 0.02) and Stroop tests (p = 0.042) |
Reger et al. (2004) [40]; USA | Open-label CT crossover | N = 20 Age = 74.7±6.7 Male = NR probable AD (n = 15) and amnestic MCI(n = 5) | I: MCTs (caprylic acid) Dosage: 40 ml blended with 152 ml heavy whipping cream. P: Heavy whipping cream | 120 minutes (no washout period) | General: ADAS-Cog and MMSE Attention: Stroop Colour Word Interference Task APOE ɛ4: ADAS-Cog | General: Improvement was observed in ADAS-Cog score post-treatment within APOE ɛ4 negative group. (p = 0.04), but not in APOE ɛ4 positive group Attention: There was no improvement observed in the Stroop Color Word test post-treatment (p = 0.33). |
Xu et al. (2020) [41]; China | RCT, crossover | N = 49 Age = 75.1±7.5 yMale = 36.7% Mild-moderate AD | I: MCTs jelly Dosage: three times daily (total 17.3 g)P: Canola oil jelly | 30 days (30 days washout) | General: ADAS-Cog-C Memory: WdRcl, WdRcg, remembering test in- structions on word recognition (RTI), and orientation (Ori)Language: Nof, Com, Rlang, ComSpk, and WFD. Attention: Attn score APOE ɛ4: ADAS-Cog-C | General: after 30 days of intervention, the MCT group showed a significant decrease (p < 0.01) in the total scores of ADAS-Cog-C compared with that of the placebo participants.Memory: MCT administration was associated with significant differences in mean changes from baseline in WdRcl (p < 0.05), Ori (p < 0.01), WdRcg (p < 0.01), and total memory (p < 0.01) scores compared to placebo. Language: MCT administration showed a significant difference in mean change from baseline in Rlang (p < 0.01), ComSpk (p < 0.01), and total language (p < 0.05) scores compared to placebo. Attention: a significant difference in mean change from baseline in attn score (p < 0.05) compared to placebo. APOE ɛ4: compared to the placebo group, only people with APOE ɛ4(−) displayed a significant improvement in the total scores of ADAS-Cog-C scores (p < 0.01), while people with APOE ɛ4(+) did not in the MCT group. |
Henderson et al. (2020) [42]; USA | RCT | N = 413 (I:208, P:205) Age = 76.76±6.47,77.2±6.48 Male = 40.7%; 41% Mild to moderate cognitive impairment APOE ɛ4 (−) subjects only | I: AC-1204 (20 grams of caprylic triglyceride per 40grams) Dosage: 40 grams of AC-1204 daily. Dosage increased gradually during the first 2 weeks (starting with 10 grams of AC-1204, and an increment of 10 grams every fourth day until a final dose of 40 grams.) P: an isocaloric mixture of sunflower oil and maltodextrin containing the same excipients as AC-1204. | 26 weeks | General: ADAS-Cog11 APOE ɛ4: ADAS-Cog11 | General: the change in ADAS-Cog11 scores between both treatment groups were not significant (p = 0.25). APOE ɛ4: no significant treatment-related differences in scores were detected in ADAS-Cog11 regardless of APOE ɛ4 status(p > 0.05). |
AD, Alzheimer’s disease; RCT, Randomized controlled trial; NR, Not reported; I, Intervention; C, Control; P, Placebo; MCT, Medicum Chain Triglyceride; MCI, Mild cognitive impairment; MMSE, Mini Mental State Examination; MoCA, Montreal Cognitive Assessment; ADAS-Cog, Alzheimer’s Disease Assessment Scale-Cognitive Subscale; ADAS-Cog-C, Alzheimer’s Disease Assessment Scale-Cognitive Subscale, Chinese version; ADCS-CGIC, AD Cooperative Study-Clinical Global Impression of Change;RL/RI-16, 16 item free/cued word learning and recall test; BVMT-R, Brief Visuospatial Memory Test-Revised; VFT, Verbal Fluency Test; BNT, Boston Naming Test; TMT, Trail Making Test; WMS-R, Wechsler Memory Scale-Revised; WAIS-III, Wechsler Adult Intelligence Scale-3rd; MEC-Lobo, Mini Cognitive Examination-Lobo Test; APOE-ɛ4, apolipoprotein E ɛ4 allele; β-OHB, β-hydoxybutyrate; WdRcl, word recall; WdRcg, word recognition task; RTI, word recognition; Ori, orientation; Nof, naming objects and fingers; Com, commands; Rlang, receptive language; ComSpk, comprehension of spoken language; WFD, word- finding difficulty.
Study quality
A summary of the methodological quality assessment of the 10 studies can be found in Table 2A and 2B. Of the eight RCTs, six were deemed poor quality, while two had fair quality according to AHRQ standards after assessment with Cochrane Risk of Bias Tool. The poor quality was mainly due to presence of performance and detection bias, where there was inadequate blinding of participants and personnel by not using a placebo investigational product in the control group [37], and unblinding of outcome assessors [37, 41]. The Newcastle-Ottawa Assessment scale was used for assessing the single arm trials. The studies conducted by Ota et al. [23] was rated as poor due to the lack of information regarding the characteristics of subjects; hence, it was difficult to determine whether exposed and non-exposed AD subjects were comparable. Despite the rating of good quality for Gandotra et al. [34], conclusion drawn contains a high risk of bias since they had no control groups, which places them low in the evidence-based pyramid.
Table 2
Methodological quality assessment of included studies. A) Methodological quality assessment of RCTs based on Cochrane Risk of Bias Tool. B) Methodological quality assessment of open label clinical trials based on the Newcastle-Ottawa Assessment scale. A
Study | Random Sequence Generation (selection Bias) | Allocation Concealment (selection Bias) | Blinding of subjects & investigators (performance Bias) | Blinding of outcome assessment (detection bias) | Incomplete Outcome Data (attrition bias) | Selective reporting (reporting bias) | Other bias | Overall Quality of Study (AHRQ standards) |
Ota et al. (2019) [23] (Postprandial) | Unclear | Unclear | Low | Unclear | Low | Low | Low | Poor |
Henderson et al. (2009) [27] | Low | High | Low | Low | Low | Low | Low | Poor |
Judy et al. (2022) [35] | Unclear | Low | Low | Low | Low | Low | Unclear | Fair |
Orti et al. (2017) [37] | Low | Unclear | High | High | Low | Low | Low | Poor |
Fortier et al. (2021) [39] | Low | Unclear | Unclear | Unclear | Low | Low | High | Poor |
Reger et al. (2004) [40] | Unclear | Unclear | Unclear | Unclear | Low | High | Low | Poor |
Xu et al. (2020) [41] | Low | Unclear | Low | High | Low | Low | Low | Poor |
Henderson et al. (2020) [42] | Unclear | Unclear | Low | Low | Low | Low | Low | Fair |
Table 2B
Selection | Comparability | Outcome | Overall Quality of Study | |
Domain | Domain | Domain | (AHRQ standards) | |
Ota et al. (2019) [23] (Chronic feeding) | Fair | Not applicable | Fair | Poor |
Gandotra et al. (2014) [34] | Good | Good | Fair | Good |
*Good quality: 3 or 4 stars in selection domain AND 1 or 2 stars in comparability domain AND 2 or 3 stars in outcome/exposure domain. Fair quality: 2 stars in selection domain AND 1 or 2 stars in comparability domain AND 2 or 3 stars in outcome/exposure domain. Poor quality: 0 or 1 star in selection domain OR 0 stars in comparability domain OR 0 or 1 stars in outcome/exposure domain.
Role of MCT on cognitive function
Of 10 studies, eight studies [27, 34, 35, 37, 38, 40–42] conducted assessments for general cognitive function, and all studies, except Henderson et al. [42], showed a significant improvement in general cognitive function post treatment. Meta-analysis was conducted on four studies that [27, 37, 41, 42] reported pre-post change. A significantly greater improvement in general cognitive function was found in MCT treated group, compared to control/placebo group (SMD = 0.64; 95% CI [0.05, 1.24]). However, the p and I2 value indicated heterogeneity between the studies (p < 0.00001, I2 = 89%). The result of this meta-analysis is shown in Fig. 2a.
Fig. 2
Forest plots of cognitive function outcomes. (a) General cognitive function between treatment (MCT) and control group. (b) Memory cognition between treatment (MCT) and control group. (c) Language cognition between treatment (MCT) and control group. (d) Attention cognition between treatment (MCT) and control group. (e) Cognitive function between APOE ɛ4(−) and APOE ɛ4(+).
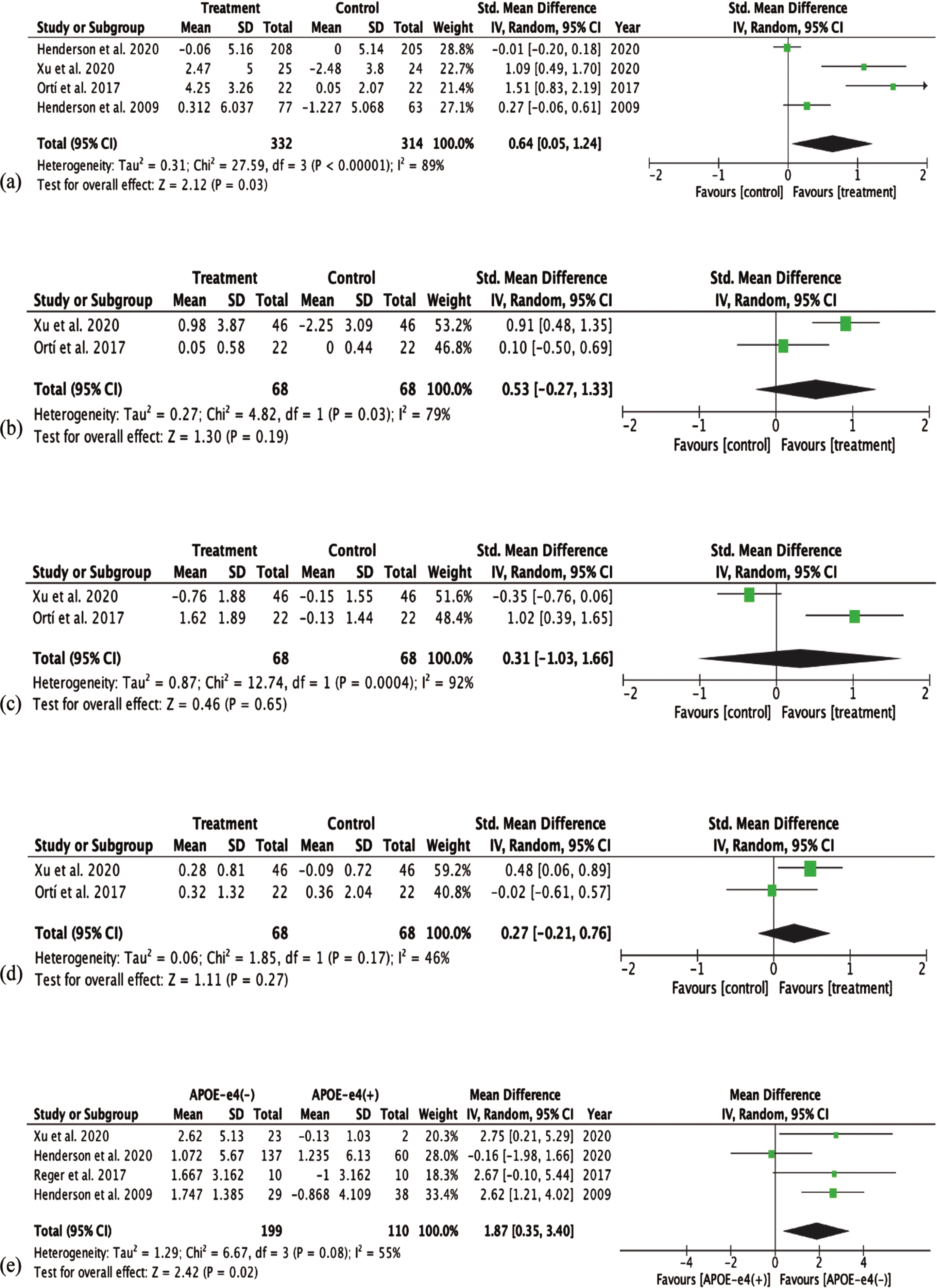
Of the five studies [23, 37, 39, 41] that assessed the memory domain of cognitive function, two studies [23, 41] including the chronic feeding study by Ota et al. showed significant improvement in memory domain post-treatments in the treatment group (p < 0.05 and p < 0.001); and one [39] showed significant improvement on the 6-item free and cued recall task (RL/RI-16) test (p = 0.047), but not on the Brief Visuospatial Memory Test-revised (BVMT-R) test. Ortí et al. [37] also showed improvement in memory post-treatment but result was not significant (p = 0.312). Fortier et al. [39] did not provide mean and standard deviation of change in memory test score; while Ota et al. [23] is an open clinical trial with no control group. Hence meta-analysis on the effect of MCT on memory domain was conducted using Ortí et al. [37] and Xu et al. [41]. As shown in Fig. 2b, meta-analysis yielded a medium effect size (SMD = 0.53, 95% CI [−0.27, 1.33]). However, the effect was found to be non-significant (z = 1.30, p = 0.19). The p and I2 value indicated heterogeneity between the studies (p = 0.03, I2 = 79%).
Three studies [37, 39, 41] assessed the language domain of cognition. All three studies reported a significant improvement in language function post-treatment in the treatment group. Meta-analysis conducted on two studies [37, 41] that provided pre-post treatment change in language domain yielded a small effect size (SMD = 0.31, 95% CI [−1.03,1.66]), and this was found to be non-significant (z = 0.46, p = 0.65). The p and I2 value indicated high heterogeneity between the studies (p = 0.0004, I2 = 92%). Result is shown in Fig. 2C.
Six studies [23, 37, 39–41] assessed the attention domain of cognition. A significant improvement in the number sequencing trail making test (TMT) (p = 0.02) and Stroop tests (p = 0.042) was observed in Frontier et al. [39]; and a significant difference in mean change from baseline in attention score compared to placebo (p < 0.05) was observed in [43]. The single-arm design chronic feeding study by Ota et al. [23] observed an improvement in only the digit-symbol coding test (0.004), but not other Wechsler Adult Intelligence Scale-III (WAIS-III) tests. The double blinded control postprandial study by Ota el. showed no significant difference in any of the tests. Similarly, Ortí et al. [37] and Reger et al. [40] did not observe improvements in the attention tests. Meta-analysis was perform on Ortí et al. [37] and Xu et al. [41]. Result in Fig. 2d shows a small effect size (SMD = 0.27, 95% CI [−0.21, 0.76]), which was found to be non-significant (z = 1.11, p = 0.27) between the treatment and the control group. The I2 value indicated some level of heterogeneity between the studies (p = 0.17, I2 = 46%).
Long-term effect of MCT on cognitive function
Two studies [27, 34] further studied the persistency of the effect of MCT for CO following cessation of treatment. Gandotra et al. found that improvement in cognitive function remained stable after discontinuation of CO for two weeks; the mean difference in ADAS-Cog score when compared to baseline was 4.1 (p < 0.001); while Henderson et al. found no significant difference in ADAS-Cog score after discontinuation of MCT.
Role of MCT on cognitive function stratified according to APOE ɛ4 status
Four studies [27, 40–42] investigated the influence of APOE ɛ4 status on the effect of MCT on changes in general cognitive function. All four studies used the ADAS-Cog assessment as the measurement of cognitive function, and therefore, meta-analysis was performed on mean difference (MD) in change from baseline between APOE ɛ4(−) and APOE ɛ4(+) group. A large effect size (MD = 1.87, 95% CI [0.35, 3.40]) was yielded, favoring APOE ɛ4(−) subjects. This was found to be statistically significant (z = 2.42, p = 0.02). The p and I2 value indicated some heterogeneity between the studies (p = 0.08; I2 = 55%). Result of meta-analysis is shown in Fig. 2e.
Effect of MCT on blood lipid profile
Secondary outcome analysis on the effect of MCT consumption on triglyceride and cholesterol level in AD patients was looked at by four studies [34, 35, 39, 41]. Gandotra et al. using CO [34] and Fortier et al. [39] and Judy et al. using MCT [35] showed no statistically significant change in triglyceride and cholesterol levels in either groups post-treatment, while Xu et al. using MCT [41] showed that treatment with MCT significantly increased the concentrations of total cholesterol (TC) and High-density lipoprotein cholesterol (HDL-C) compared with baseline (p < 0.01), and the placebo group (p < 0.05).
Adverse effects associated with the use of MCT
Adverse events experienced by subjects which could have contributed to dropouts during the clinical trial were reported by six studies [23, 27, 35, 39, 41, 42]. Particularly, diarrhea [23, 27, 35, 42] was a highly reported adverse event leading to subjects’ intolerance to MCT. Other minimal side effects reported by the subjects included abdominal or stomach discomfort [23, 35, 42], nausea [42], vomiting [42], dyspepsia [42] and/or constipation [42].
DISCUSSION
This systematic review summarized the effect of MCT on AD and related cognitive impairment and provided a quantitative measure of the effect of MCT on different domains of cognitive impairment through meta-analysis. Pooled results from available studies showed a statistically significant trend favoring the use of MCT for improvements in general cognitive function. Positive effect sizes were also found for individual cognitive domains (memory, language, attention), but the effects failed to reach statistical significance. This could be due to the limited number of studies (two studies only) available for quantitative analysis. Current meta-analysis used mean change from baseline (pre-post change) instead of final measurements. By doing so, the effect of baseline difference in cognitive function between the treatment groups, a potential source of bias, was minimized. Only a small number of studies reported pre-post change or provided information where pre-post change could be calculated for quantitative analysis. Moreover, high levels of heterogeneity were detected between studies, primarily due to the use of different study designs, cognitive assessment tools and patient characteristics. Therefore, more studies are warranted before making a definite conclusion on the use of MCT in AD related cognitive impairment treatment.
Studies have investigated the mode of action of MCT in pursuit of a better understanding of AD pathophysiology. AD is characterized by the gradual accumulation of cerebral extracellular Aβ peptides [44] and substantial progressive cognitive impairment affecting several domains [45]. Bansal et al. [46] showed that treatment of neuronal cells with CO result in a significant decrease in Aβ protein accumulation (p < 0.05); and the same result was observed in animal studies [47, 48]. Consequently, CO is postulated to have antioxidative properties. Studies showed that treatment with CO led to decreased oxidative stress in both neuronal cell and AD rat model [49, 50]. Thus, CO is postulated to improve cognitive function through a series of mechanisms leading to reduced neuroinflammation. In addition, CO is thought to preserve the structure of neurons [49, 51]. Alghamdi [49] reported that CO preserved cortical and hippocampal neurons in AD rats when viewed under light and electron microscopes. This supports the finding of a previous in-vitro model, where treatment of neuronal cells with CO significantly increased survival rate (p < 0.001) [51].
Clinical trials have also suggested a neuroprotective effect of MCT, whereby metabolism of MCT to ketone bodies may provide an alternative fuel source other than ATP in neurons that are unable to utilize glucose [52]. In a clinical trial conducted by Croteau et al., blood ketones level and cerebral metabolic rate (CMR) increased post-treatment with MCT, and a positive correlation between blood ketones level and CMR was noted [52]. Moreover, when plasma ketone is raised as a result of diet or infusion, ketone bodies are metabolized in a concentration-dependent manner in the brain [10]. Improvements in cognitive functions in MCI and AD patients were seen in the general (n = 7) [27, 34, 35, 37, 38, 40, 41], memory (n = 3) [23, 39, 41], language (n = 3) [37, 39, 41], and attention (n = 3) [23, 39, 41] domains, after MCT treatments; and hyperketonemia induced by MCT intake might be responsible for the changes in cognitive function [21]. The increase in blood ketone levels following MCT administration was seen in seven of the included studies [23, 27, 39–42]. Correlating blood ketone levels to cognitive function assessment scores found a significant positive correlation, and hence, improvement in cognitive function assessment score is directly related to an increased blood ketone level.
One of the major genetic risk factors associated with AD is the presence of APOE ɛ4 allele in individuals [53]. APOE ɛ4 allele carriers were found to have decreased ability to metabolize glucose and lipids, and thus potentially affecting therapeutics targeting these pathways in the management of AD [54]. Subgroup analysis conducted found significant cognitive improvements in APOE ɛ4 (−) individuals in response to MCT treatment, while APOE ɛ4 (+) showed no benefit [27, 40, 41]. This is consistent with the previous finding that cognitive response to elevated energetic substrates is dependent on APOE genotype [55]. It is suggested that APOE ɛ4 carriers may have a different dose-response pattern compared to non-carriers, and hence differences in pathophysiology [40]. In some other AD therapies, APOE ɛ4 (−) patients have shown to have greater benefits compared to APOE ɛ4 (+) patients, such as in glucose and insulin [55], nasal insulin [56], and insulin sensitizing agent rosiglitazone therapies [57]. One possible explanation could be that APOE ɛ4 (+) patients have lower mitochondrial enzyme function compared to APOE ɛ4 (−) patients as seen in AD brain tissue samples [58, 59]. Reduced mitochondrial function is associated with reduced ability in ketone utilization, which could explain the unresponsiveness to MCT treatment in APOE ɛ4 (+) patients despite a significant increase in blood ketone [40]. Further studies examining the role of APOE genotype on the pharmacokinetic response patterns in AD patients may provide better explanations to the observed phenomenon.
The use of MCT in the management of AD remains controversial due to the concern for increased cardiovascular risk. MCT is a type of saturated fatty acid (SFA), and SFA has been shown to increase LDL cholesterol, with consequent development of cardiovascular diseases [60]. However, SFAs of MCT are of median-chain length (C8:0−C12:0), which consists of 7.8% caprylic acid (C8:0), 6.7% capric acid (C10:0), and approximately 47.5% lauric acid (C12:0) [61]. SFAs with carbon chain lengths C6:0−10:0 are rapidly absorbed into the portal vein and metabolized [62], while carbon chain lengths≥12:0 are taken up by chylomicrons and transported into the circulation before metabolizing [63]. Given the difference in metabolizing properties, the rise in blood LDL cholesterol concentrations following SFA consumption may depend on type of SFAs. Of the three reviewed studies that looked at the effect of MCT on blood lipid profile, two showed no change in triglyceride and cholesterol level [35, 39], and one showed that MCT significantly increased the concentrations of total cholesterol (TC) and high-density lipoprotein cholesterol (HDL-C) [41]. This is in consensus with previous studies, which suggested no effect of MCT on cholesterol metabolism [64, 65]. Moreover, a recent systematic review and meta-analysis demonstrated that MCT consumption could increase HDL-C levels without changing non-HDL-C in comparison with long-chain SFAs [66]. Treatment with CO also did not show an effect on triglyceride and cholesterol level, despite containing long-chain SFAs [34]. All four studies showed improvements in one or more domains of cognitive function post-treatment. Therefore, MCT consumption may lead to improvements in cognitive function without adversely impacting on cardiometabolic risk factors.
This systematic review has its value in being one of the very few and most recent systematic reviews conducted on the impact of MCT in cognitive function in AD patients. The use of our keyword terms identified all studies included in previous systematic reviews [18, 26], as well as additional studies published thereafter. Compared to previous systematic reviews our review has several strengths. First, it has the most up-to-date clinical data, with database search up until December 2022. Second, individual domains of cognitive function were considered for more comprehensive understandings of the effect of MCT on specific regions of the brain. Third, it provided subgroup analysis on the influence of APOE genotypes on the extent of effectiveness of MCT. Fourth, cardiometabolic risk and potential adverse effects upon MCT consumption were examined. Fifth, utilization of pre-post change in quantitative analysis to account for potential differences between groups, Lastly, we did not include pilot studies as their primary objective was to test the feasibility and prospects of the study; the methodology employed is typically unrefined, and results discussed focus on methodological quality to benefit future RCTs [67]. Inclusion of pilot and case report studies can decrease the quality level of the analysis, which we avoided in our systematic review.
However, this systematic review is not without limitations. First, only ten studies were eligible for inclusion despite a comprehensive search in five databases and citation screening. This is largely attributed to the limited number of available interventions, and some trials identified had no data and hence excluded. Second, single-arm studies were included in the review. However, these were not included in the quantitative analysis. Third, there was heterogeneity in cognitive assessments. While MMSE, MoCA, and ADAS-Cog are shown to closely cross-correlate with each other [68], correlations between subgroup tests are still to be examined. Fourth, different MCT formulations and dosages were used between studies. According to Vandenberghe et al. [69], the ketogenic effect of MCT depends on the percentage of C8 and C10. The ketogenic effect of C8 is two-fold that of C10 [70]. Fatty acids with carbon chain lengths≤8 can cross the inner mitochondria membrane in the absence of carnitine palmitoyl transferase I. [71]. Moreover, C8 appears to experience β-oxidation in astrocytes more easily than C10, hence more readily induces ketogenesis [72]. This could suggest that MCT may have different effects on blood lipid profile due to difference in C8 and C10 levels. Moreover, 20 g of C8 produced a significantly stronger ketogenic response than 10 g of C8 [73]. Therefore, the dose at which greatest ketogenic response could be achieved without introducing risk should be explored. Fifth, studies varied in patient characteristics (stage of disease). Disease state of AD patients is postulated to influence the effectiveness of MCT, and early diagnosis and treatment may increase response to therapy [74]. Lastly, some studies did not control for APOE genotype. APOE ɛ4 status was found as a potential effect modifier [27, 40, 41]. All these resulted in discrepancies in findings.
CONCLUSION
This systematic review and meta-analysis provide the most up-to-date consolidation and analysis of studies on the effect of MCT in treating cognitive impairment associated with AD. Meta-analysis of RCTs showed significant positive effect of MCT on general cognitive function, but not in the individual cognitive domains (i.e., memory, language, attention). Future interventions should provide more homogenized study designs, cognitive assessment methods and better characterized patient populations in order to arrive at a conclusion supported by stronger evidence. Finally, the relationship between effect modifiers and MCT should be considered to increase the precision of effect estimation.
ACKNOWLEDGMENTS
The authors have no acknowledgments to report.
FUNDING
The authors have no funding to report.
CONFLICT OF INTEREST
The authors have no conflict of interest to report.
DATA STATEMENT
The data has not been previously presented orally or by poster at scientific meetings.
SUPPLEMENTARY MATERIAL
[1] The supplementary material is available in the electronic version of this article: https://dx.doi.org/10.3233/JAD-230406.
REFERENCES
[1] | McKhann GM , Knopman DS , Chertkow H , Hyman BT , Jack CR Jr. , Kawas CH , Klunk WE , Koroshetz WJ , Manly JJ , Mayeux R , Mohs RC , Morris JC , Rossor MN , Scheltens P , Carrillo MC , Thies B , Weintraub S , Phelps CH ((2011) ) The diagnosis of dementia due to Alzheimer’s disease: Recommendations from the National Institute on Aging-Alzheimer’s Association workgroups on diagnostic guidelines for Alzheimer’s disease. Alzheimers Dement 7: , 263–269. |
[2] | Lindsay J , Laurin D , Verreault R , Hébert R , Helliwell B , Hill GB , McDowell I ((2002) ) Risk factors forAlzheimer’s disease: A prospective analysis from the Canadian Study of Health and Aging. Am J Epidemiol 156: , 445–453. |
[3] | Tarawneh R , Holtzman DM ((2012) ) The clinical problem of symptomatic Alzheimer disease and mild cognitive impairment. Cold Spring Harb Perspect Med 2: , a006148. |
[4] | Jia L , Du Y , Chu L , Zhang Z , Li F , Lyu D , Li Y , Zhu M , Jiao H , Song Y ((2020) ) Prevalence, risk factors, and management of dementia and mild cognitive impairment in adults aged 60 years or older in China: A cross-sectional study. Lancet Public Health 5: , e661–e671. |
[5] | Jongsiriyanyong S , Limpawattana P ((2018) ) Mild cognitive impairment in clinical practice: A review article. Am J Alzheimers Dis Other Demen 33: , 500–507. |
[6] | Flannery PJ , Trushina E ((2019) ) Mitochondrial dynamics and transport in Alzheimer’s disease. Mol Cell Neurosci 98: , 109–120. |
[7] | Abolhassani N , Leon J , Sheng Z , Oka S , Hamasaki H , Iwaki T , Nakabeppu Y ((2017) ) Molecular pathophysiology of impaired glucose metabolism, mitochondrial dysfunction, and oxidative DNA damage in Alzheimer’s disease brain. Mech Ageing Dev 161: , 95–104. |
[8] | Koepsell H ((2020) ) Glucose transporters in brain in health and disease. Pflugers Arch 472: , 1299–1343. |
[9] | Aoyama K , Nakaki T ((2013) ) Impaired glutathione synthesis in neurodegeneration. Int J Mol Sci 14: , 21021–21044. |
[10] | Cunnane SC , Courchesne-Loyer A , Vandenberghe C , St-Pierre V , Fortier M , Hennebelle M , Croteau E , Bocti C , Fulop T , Castellano CA ((2016) ) Can ketones help rescue brain fuel supply in later life? Implications for cognitive health during aging and the treatment of Alzheimer’s disease. Front Mol Neurosci 9: , 53. |
[11] | Pierre K , Pellerin L ((2005) ) Monocarboxylate transporters in the central nervous system: Distribution, regulation and function. J Neurochem 94: , 1–14. |
[12] | Page KA , Williamson A , Yu N , McNay EC , Dzuira J , McCrimmon RJ , Sherwin RS ((2009) ) Medium-chain fatty acids improve cognitive function in intensively treated type 1 diabetic patients and support} synaptic transmission during acute hypoglycemia. Diabetes 58: , 1237–1244. |
[13] | Schönfeld P , Wojtczak L ((2016) ) Short- and medium-chain fatty acids in energy metabolism: The cellular perspective. J Lipid Res 57: , 943–954. |
[14] | Castellano CA , Nugent S , Paquet N , Tremblay S , Bocti C , Lacombe G , Imbeault H , Turcotte É , Fulop T , Cunnane SC ((2015) ) Lower brain 18F-fluorodeoxyglucose uptake but normal 11C-acetoacetate metabolism in mild Alzheimer’sdisease dementia. J Alzheimers Dis 43: , 1343–1353. |
[15] | Croteau E , Castellano CA , Fortier M , Bocti C , Fulop T , Paquet N , Cunnane SC ((2018) ) A cross-sectional comparison of brain glucose and ketone metabolism in cognitively healthy older adults, mild cognitive impairment and early Alzheimer’s disease. Exp Gerontol 107: , 18–26. |
[16] | Miller VJ , Villamena FA , Volek JS ((2018) ) Nutritional ketosis and mitohormesis: Potential implications for mitochondrial function and human health. J Nutr Metab 2018: , 5157645. |
[17] | Chatterjee P , Fernando M , Fernando B , Dias CB , Shah T , Silva R , Williams S , Pedrini S , Hillebrandt H , Goozee K , Barin E , Sohrabi HR , Garg M , Cunnane S , Martins RN ((2020) ) Potential of coconut oil and medium chain triglycerides in the prevention and treatment of Alzheimer’s disease. Mech Ageing Dev 186: , 111209. |
[18] | Castro CB , Dias CB , Hillebrandt H , Sohrabi HR , Chatterjee P , Shah TM , Fuller SJ , Garg ML , Martins RN ((2023) ) Medium-chain fatty acids for the prevention or treatment of Alzheimer’s disease: A systematic review and meta-analysis. Nutr Rev, 10.1093/nutrit/nuac104. |
[19] | Fukao T , Lopaschuk GD , Mitchell GA ((2004) ) Pathways and control of ketone body metabolism: On the fringe of lipid biochemistry. Prostaglandins Leukot Essent Fatty Acids 70: , 243–251. |
[20] | Roopashree PG , Shetty SS , Suchetha Kumari N ((2021) ) Effect of medium chain fatty acid in human health and disease. J Funct Foods 87: , 104724. |
[21] | Augustin K , Khabbush A , Williams S , Eaton S , Orford M , Cross JH , Heales SJR , Walker MC , Williams RSB ((2018) ) Mechanisms of action for the medium-chain triglyceride ketogenic diet in neurological and metabolic disorders. Lancet Neurol 17: , 84–93. |
[22] | de la Rubia Ortí JE , García-Pardo MP , Drehmer E , Sancho Cantus D , Julián Rochina M , Aguilar MA , HuYang I ((2018) ) Improvement of main cognitive functions in patients with Alzheimer’s disease after treatment withcoconut oil enriched Mediterranean diet: A pilot study. J Alzheimers Dis 65: , 577–587. |
[23] | Ota M , Matsuo J , Ishida I , Takano H , Yokoi Y , Hori H , Yoshida S , Ashida K , Nakamura K , Takahashi T , Kunugi H ((2019) ) Effects of a medium-chain triglyceride-based ketogenic formula on cognitive function in patients with mild-to-moderate Alzheimer’s disease. Neurosci Lett 690: , 232–236. |
[24] | Mensink RP , Katan MB ((1992) ) Effect of dietary fatty acids on serum lipids and lipoproteins. A meta-analysis of 27 trials. Arterioscler Thromb 12: , 911–919. |
[25] | Coon DW , Edgerly ES ((1999) ) The personal and social consequences of Alzheimer disease. Genet Test 3: , 29–36. |
[26] | Avgerinos KI , Egan JM , Mattson MP , Kapogiannis D ((2020) ) Medium chain triglycerides induce mild ketosis and may improve cognition in Alzheimer’s disease. A systematic review and meta-analysis of human studies. Ageing Res Rev 58: , 101001. |
[27] | Henderson ST , Vogel JL , Barr LJ , Garvin F , Jones JJ , Costantini LC ((2009) ) Study of the ketogenic agent AC-1202 in mild to moderate Alzheimer’s disease: A randomized, double-blind, placebo-controlled, multicenter trial. Nutr Metab (Lond) 6: , 31. |
[28] | Dettori JR ((2011) ) Loss to follow-up. Evid Based Spine Care J 2: , 7–10. |
[29] | Langan C , Sarode DP , Russ TC , Shenkin SD , Carson A , Maclullich AMJ ((2017) ) Psychiatric symptomatology after delirium: A systematic review. Psychogeriatrics 17: , 327–335. |
[30] | Borenstein M , Hedges LV , Higgins JP , Rothstein HR ((2010) ) A basic introduction to fixed-effect and random-effects models for meta-analysis. Res Synth Methods 1: , 97–111. |
[31] | Deeks JJ , Higgins JPT , Altman DG ((2011) ) Chapter 9: Analysing data and undertaking meta-analyses. In. Cochrane Handbook for Systematic Reviews of Interventions (5.1.0). |
[32] | Cohen J ((1969) ) Statistical power analysis for the behavioral sciences (1st ed.), Academic Press, New York. |
[33] | Cohen J ((1994) ) The earth is round (p < 0.05). Am Psychol 49: , 997–1003. |
[34] | Gandotra S , Kour J , Van der Waag A ((2014) ) Efficacy of adjunctive extra virgin coconut oil use in moderate to severe Alzheimer’s disease. Int J Sch Cog Psychol 1: , 2. |
[35] | Juby AG , Blackburn TE , Mager DR ((2022) ) Use of medium chain triglyceride (MCT) oil in subjects with Alzheimer’s disease: A randomized, double-blind, placebo-controlled, crossover study, with an open-label extension. Alzheimers Dement (N Y) 8: , e12259. |
[36] | Balsis S , Benge JF , Lowe DA , Geraci L , Doody RS ((2015) ) How do scoreson the ADAS-Cog, MMSE, and CDR-SOB correspond? Clin Neuropsychol 29: , 1002–1009. |
[37] | De la Rubia Ortí JE , Sánchez Álvarez C , Selvi Sabater P , Bueno Cayo AM , Sancho Castillo S , Rochina S , Hu Yang I ((2017) ) Influencia del aceite de coco en enfermos de alzhéimer a nivel cognitivo. Nutr Hosp 34: , 352–356. |
[38] | Hu Yang I , Rubia Ortí JEdl , Selvi Sabater P , Sancho Castillo S , Rochina MJ , Manresa Ramón N , Montoya-Castilla I ((2015) ) Aceite de coco: Tratamiento alternativo no farmacológico frente a la enfermedad deAlzheimer. Nutr Hosp 32: , 2822–2827. |
[39] | Fortier M , Castellano CA , Croteau E , Langlois F , Bocti C , St-Pierre V , Vandenberghe C , Bernier M , Roy M , Descoteaux M , Whittingstall K , Lepage M , Turcotte É E , Fulop T , Cunnane SC ((2021) ) A ketogenic drink improvescognition in mild cognitive impairment: Results of a 6-month RCT. Alzheimers Dement 17: , 543–552. |
[40] | Reger MA , Henderson ST , Hale C , Cholerton B , Baker LD , Watson GS , Hyde K , Chapman D , Craft S ((2004) ) Effects of beta-hydroxybutyrate on cognition in memory-impaired adults. Neurobiol Aging 25: , 311–314. |
[41] | Xu Q , Zhang Y , Zhang X , Liu L , Zhou B , Mo R , Li Y , Li H , Li F , Tao Y , Liu Y , Xue C ((2020) ) Medium-chain triglycerides improved cognition and lipid metabolomics in mild to moderate Alzheimer’s disease patients with APOE4– /– : A double-blind, randomized, placebo-controlled crossover trial. Clin Nutr 39: , 2092–2105. |
[42] | Henderson ST , Morimoto BH , Cummings JL , Farlow MR , Walker J ((2020) ) A placebo-controlled, parallel-group, randomized clinical trial of AC-1204 in mild-to-moderate Alzheimer’s disease. J Alzheimers Dis 75: , 547–557. |
[43] | Xu S , Huang X , Gong Y , Sun J ((2021) ) Association between tooth loss rate and risk of mild cognitive impairment in older adults: A population-based longitudinal study. Aging (Albany NY) 13: , 21599–21609. |
[44] | Chételat G , Villemagne VL , Bourgeat P , Pike KE , Jones G , Ames D , Ellis KA , Szoeke C , Martins RN , O’Keefe GJ , Salvado O , Masters CL , Rowe CC , Australian Imaging Biomarkers and Lifestyle Research Group ((2010) ) Relationship between atrophy and β-amyloid deposition in Alzheimer disease. Ann Neurol 67: , 317–324. |
[45] | Scheltens P , De Strooper B , Kivipelto M , Holstege H , Chételat G , Teunissen CE , Cummings J , van der Flier WM ((2021) ) Alzheimer’s disease. Lancet 397: , 1577–1590. |
[46] | Bansal A , Kirschner M , Zu L , Cai D , Zhang L ((2019) ) Coconut oil decreases expression of amyloid precursor protein (APP) and secretion of amyloid peptides through inhibition of ADP-ribosylation factor 1 (ARF1). Brain Res 1704: , 78–84. |
[47] | Mirzaei F , Khazaei M , Komaki A , Amiri I , Jalili C ((2018) ) Virgin coconut oil (VCO) by normalizing NLRP3 inflammasome showed potential neuroprotective effects in amyloid-β induced toxicity and high-fat diet fed rat. Food Chem Toxicol 118: , 68–83. |
[48] | Studzinski CM , MacKay WA , Beckett TL , Henderson ST , Murphy MP , Sullivan PG , Burnham WM ((2008) ) Induction of ketosis may improve mitochondrial function and decrease steady-state amyloid-beta precursor protein (APP) levels in the aged dog. Brain Res 1226: , 209–217. |
[49] | Alghamdi BSA ((2018) ) Possible prophylactic anti-excitotoxic and anti-oxidant effects of virgin coconut oil on aluminium chloride-induced Alzheimer’s in rat models. J Integr Neurosci 17: , 593–607. |
[50] | Nafar F , Clarke JP , Mearow KM ((2017) ) Coconut oil protects cortical neurons from amyloid beta toxicity by enhancing signaling of cell survival pathways. Neurochem Int 105: , 64–79. |
[51] | Nafar F , Mearow KM ((2014) ) Coconut oil attenuates the effects of amyloid-β on cortical neurons in vitro. J Alzheimers Dis 39: , 233–237. |
[52] | Croteau E , Castellano CA , Richard MA , Fortier M , Nugent S , Lepage M , Duchesne S , Whittingstall K , Turcotte ÉE , Bocti C , Fülöp T , Cunnane SC ((2018) ) Ketogenic medium chain triglycerides increase brain energymetabolism in Alzheimer’s disease. J Alzheimers Dis 64: , 551–561. |
[53] | Kim J , Basak JM , Holtzman DM ((2009) ) The role of apolipoprotein E in Alzheimer’s disease. Neuron 63: , 287–303. |
[54] | Brandon JA , Farmer BC , Williams HC , Johnson LA ((2018) ) APOE and Alzheimer’s disease: Neuroimaging of metabolic and cerebrovascular dysfunction. Front Aging Neurosci 10: , 180. |
[55] | Craft S , Asthana S , Schellenberg G , Baker L , Cherrier M , Boyt AA , Martins RN , Raskind M , Peskind E , Plymate S ((2000) ) Insulin effects on glucose metabolism, memory, and plasma amyloid precursor protein in Alzheimer’s disease differ according to apolipoprotein-E genotype. Ann N Y Acad Sci 903: , 222–228. |
[56] | Reger MA , Watson GS , Frey WH 2nd , Baker LD , Cholerton B , Keeling ML , Belongia DA , Fishel MA , Plymate SR , Schellenberg GD , Cherrier MM , Craft S ((2006) ) Effects of intranasal insulin on cognition in memory-impaired older adults: Modulation by APOE genotype. Neurobiol Aging 27: , 451–458. |
[57] | Risner ME , Saunders AM , Altman JF , Ormandy GC , Craft S , Foley IM , Zvartau-Hind ME , Hosford DA , Roses AD ((2006) ) Efficacy of rosiglitazone in a genetically defined population with mild-to-moderate Alzheimer’s disease. Pharmacogenomics J 6: , 246–254. |
[58] | Gibson GE , Haroutunian V , Zhang H , Park LC , Shi Q , Lesser M , Mohs RC , Sheu RK , Blass JP ((2000) ) Mitochondrial damage in Alzheimer’s disease varies with apolipoprotein E genotype. Ann Neurol 48: , 297–303. |
[59] | Liang WS , Reiman EM , Valla J , Dunckley T , Beach TG , Grover A , Niedzielko TL , Schneider LE , Mastroeni D , Caselli R , Kukull W , Morris JC , Hulette CM , Schmechel D , Rogers J , Stephan DA ((2008) ) Alzheimer’s disease is associated with reduced expression of energy metabolism genes in posterior cingulate neurons. Proc Natl Acad Sci U S A 105: , 4441–4446. |
[60] | Maki KC , Dicklin MR , Kirkpatrick CF ((2021) ) Saturated fats and cardiovascular health: Current evidence and controversies. J Clin Lipidol 15: , 765–772. |
[61] | Joshi S , Kaushik V , Gode V , Mhaskar S ((2020) ) Coconut oil and immunity: What do we really know about it so far? J Assoc Physicians India 68: , 67–72. |
[62] | DeLany JP , Windhauser MM , Champagne CM , Bray GA ((2000) ) Differential oxidation of individual dietary fatty acids in humans. Am J Clin Nutr 72: , 905–911. |
[63] | Swift LL , Hill JO , Peters JC , Greene HL ((1990) ) Medium-chain fatty acids: Evidence for incorporation into chylomicron triglycerides in humans. Am J Clin Nutr 52: , 834–836. |
[64] | Courchesne-Loyer A , Fortier M , Tremblay-Mercier J , Chouinard-Watkins R , Roy M , Nugent S , Castellano CA , Cunnane SC ((2013) ) Stimulation of mild, sustained ketonemia by medium-chain triacylglycerols in healthy humans: Estimated potential contribution to brain energy metabolism. Nutrition 29: , 635–640. |
[65] | Traul KA , Driedger A , Ingle DL , Nakhasi D ((2000) ) Review of the toxicologic properties of medium-chain triglycerides. Food Chem Toxicol 38: , 79–98. |
[66] | Panth N , Abbott KA , Dias CB , Wynne K , Garg ML ((2018) ) Differential effects of medium- and long-chain saturated fatty acids on blood lipid profile: A systematic review and meta-analysis. Am J Clin Nutr 108: , 675–687. |
[67] | Chan CL , Leyrat C , Eldridge SM ((2017) ) Quality of reporting of pilot and feasibility cluster randomised trials: A systematic review. BMJ Open 7: , e016970. |
[68] | Wei YC , Chen CK , Lin C , Chen PY , Hsu PC , Lin CP , Shyu YC , Huang WY ((2022) ) Normative data of Mini-Mental State Examination, Montreal Cognitive Assessment, and Alzheimer’s Disease Assessment Scale-Cognitive subscale of community-dwelling older adults in Taiwan. Dement Geriatr Cogn Disord 51: , 365–376. |
[69] | Vandenberghe C , St-Pierre V , Pierotti T , Fortier M , Castellano C-A , Cunnane SC ((2017) ) Tricaprylin alone increases plasma ketone response more than coconut oil or other medium-chain triglycerides: An acute crossover study in healthy adults. Curr Dev Nutr 1: , e000257. |
[70] | St-Pierre V , Vandenberghe C , Lowry CM , Fortier M , Castellano CA , Wagner R , Cunnane SC ((2019) ) Plasma ketone and medium chain fatty acid response in humans consuming different medium chain triglycerides during a metabolic study day. Front Nutr 6: , 46. |
[71] | Khabbush A , Orford M , Tsai YC , Rutherford T , O’Donnell M , Eaton S , Heales SJR ((2017) ) Neuronal decanoic acid oxidation is markedly lower than that of octanoic acid: A mechanistic insight into the medium-chain triglyceride ketogenic diet. Epilepsia 58: , 1423–1429. |
[72] | Thevenet J , De Marchi U , Domingo JS , Christinat N , Bultot L , Lefebvre G , Sakamoto K , Descombes P , Masoodi M , Wiederkehr A ((2016) ) Medium-chain fatty acids inhibit mitochondrial metabolism in astrocytes promoting astrocyte-neuron lactate and ketone body shuttle systems. FASEB J 30: , 1913–1926. |
[73] | Vandenberghe C , St-Pierre V , Fortier M , Castellano CA , Cuenoud B , Cunnane SC ((2020) ) Medium chain triglycerides modulate the ketogenic effect of a metabolic switch. Front Nutr 7: , 3. |
[74] | Small GW ((2000) ) Early diagnosis of Alzheimer’s disease: Update on combining genetic and brain-imaging measures. Dialogues Clin Neurosci 2: , 241–246. |