Relationship of Muscle Apolipoprotein E Expression with Markers of Cellular Stress, Metabolism, and Blood Biomarkers in Cognitively Healthy and Impaired Older Adults
Abstract
Background:
Individuals with mild cognitive impairment (MCI) have reduced lipid-stimulated mitochondrial respiration in skeletal muscle. A major risk factor for Alzheimer’s disease (AD), the apolipoprotein E4 (APOE4) allele, is implicated in lipid metabolism and is associated with metabolic and oxidative stress that can result from dysfunctional mitochondria. Heat shock protein 72 (Hsp72) is protective against these stressors and is elevated in the AD brain.
Objective:
Our goal was to characterize skeletal muscle ApoE and Hsp72 protein expression in APOE4 carriers in relationship to cognitive status, muscle mitochondrial respiration and AD biomarkers.
Methods:
We analyzed previously collected skeletal muscle tissue from 24 APOE4 carriers (60y+) who were cognitively healthy (CH, n = 9) or MCI (n = 15). We measured ApoE and Hsp72 protein levels in muscle and phosphorylated tau181 (pTau181) levels in plasma, and leveraged previously collected data on APOE genotype, mitochondrial respiration during lipid oxidation, and VO2 max.
Results:
Muscle ApoE (p = 0.013) and plasma pTau181 levels (p < 0.001) were higher in MCI APOE4 carriers. Muscle ApoE positively correlated with plasma pTau181 in all APOE4 carriers (R2 = 0.338, p = 0.003). Hsp72 expression negatively correlated with ADP (R2 = 0.775, p = <0.001) and succinate-stimulated respiration (R2 = 0.405, p = 0.003) in skeletal muscle of MCI APOE4 carriers. Plasma pTau181 negatively tracked with VO2 max in all APOE4 carriers (R2 = 0.389, p = 0.003). Analyses were controlled for age.
Conclusion:
This work supports a relationship between cellular stress in skeletal muscle and cognitive status in APOE4 carriers.
INTRODUCTION
Approximately 16% of Americans age 65 years and older have mild cognitive impairment (MCI), and it is estimated that half of these cases are due to Alzheimer’s disease (AD), the most common form of dementia [1]. Impaired energy metabolism in the brain, characterized by reduced cerebral glucose metabolism [2], is a hallmark pathologic feature of AD. Metabolic dysfunction also manifests systemically in individuals with AD as reduced cardiorespiratory fitness [3], hypertriglyceridemia [4] and hyperglycemia [4].
Apolipoprotein E (ApoE) is a lipid transport protein, with various alleles modifying AD risk. When compared to non-carriers, APOE4 carriers display more pronounced impairments in glucose meta-bolism in various brain regions [5], are more susceptible to metabolic syndrome [6], and are less responsive to interventions that target energy metabolism to improve cognitive function [7, 8]. Evidence that APOE4 carrier status differentially affects both central and peripheral metabolism suggests that the underlying pathogenesis of AD varies by genotype and that whole-body metabolism may be more important to AD pathogenesis in APOE4 carriers. These data support the notion that APOE genotype-specific effects on metabolism deserve further investigation.
Skeletal muscle mitochondrial function is critical for whole body oxygen consumption [9] and glucose and fatty acid oxidation [10]. In the absence of AD medication treatment, individuals with MCI have reduced mitochondrial respiration in skeletal muscle [11]. Data in preclinical rodent models further suggests that APOE4 modifies skeletal muscle mitochondrial activity by increasing the expression of proteins implicated in fatty acid transport and oxidation [12].
ApoE and heat shock protein (Hsp72) are two proteins implicated in mitochondrial dysfunction and AD. Hsp72 is a highly-inducible, stress-responsive chaperone protein that has been found to be elevated in the temporal cortex of individuals with AD [13, 14]. In addition to its well-known roles in protein folding, this protein is responsive to and protective against declines in both ATP production [15] and oxidative stress [16, 17] that can result from mitochondrial dysfunction. Given that APOE4 is associated with impairments in mitochondrial activity [18] and the ability to handle oxidative stress [19], Hsp72 may be critical to mitigating cellular stress in APOE4 carriers.
Using skeletal muscle tissue collected from older adults who were cognitively healthy (CH) or MCI and at a genetic risk for AD (APOE4 carriers), we investigated the hypothesis that ApoE and Hsp72 expression are elevated in skeletal muscle of APOE4 carriers with MCI compared to those who are CH. We also determined if the expression of these proteins are inversely correlated with lipid-stimulated muscle mitochondrial respiration and positively correlated with plasma AD neuropathology biomarkers. We show that muscle ApoE and the plasma AD biomarker, phosphorylated tau181 (pTau181), are elevated in individuals with MCI and that plasma pTau181 is positively correlated with muscle ApoE and negatively correlated with VO2 max.
MATERIALS AND METHODS
Skeletal muscle and plasma
We leveraged a subset of vastus lateralis biopsy tissue and blood plasma banked from a previously completed study approved by the University of Kansas Medical Center’s Institutional Review Board (IRB #140787) [11]. Written informed consent was obtained from all participants. We analyzed tissue collected from a total of 24 APOE4 carriers who were either cognitively healthy (n = 9) or were diagnosed with MCI (n = 15). Diagnostic inclusion criteria included no prior memory complaints (CH older adults), or MCI diagnosed by a clinician and verified with medical records. In the CH group, there were 7 E3/E4 and 2 E4/E4 participants. In the MCI group, there were 11 E3/E4 and 4 E4/E4 participants. All participants were 60 years or older.
Western blot
Western blot analysis was performed on muscle tissue from CH (n = 8) and MCI (n = 15) APOE4 carriers. One CH participant had insufficient tissue available for western blot. Frozen muscle was powdered and lysed with a TissueLyser II bead homogenizer in buffer (50 mM HEPES, 12 mM sodium pyrophosphate, 100 mM NaF, 10 mM EDTA, 400μl each of phosphatase inhibitor cocktails 1 and 2, and 1% Triton X-100). Protein concentration was measured using a BCA protein assay. Proteins were separated using SDS-PAGE prior to transferring proteins to a PVDF membrane. Proteins were stained with antibodies directed against ApoE (Abcam, Cambridge, MA; ab52607) and Hsp72 (Enzo Life Sciences, Farmingdale, NY; C92F3A-5) and protein loading was corrected using Ponceau S Solution (Abcam, Cambridge, MA; ab270042). Densitometry was used to quantify protein bands and total protein using Image Lab software (Bio-Rad Laboratories, Hercules, CA).
Mitochondrial respiration and cardiorespiratory fitness
We leveraged mitochondrial O2 flux data and cardiorespiratory fitness data that was collected previously [11]. Briefly, vastus lateralis skeletal muscle tissue obtained at the time of biopsy was dissected to remove connective tissue and ∼30 mg was placed into ice cold buffer X as previously described [11]. Muscle fiber bundles were teased from the 30 mg of muscle and permeabilized for 30 min in 30μg/mL of saponin prior to being washed in buffer Z (105 mM K-MES, 30 mM KCl, 10 mM K2HPO4, 5 mM MgCl2-6H2O, and 0.5% w/w fatty acid-free BSA; pH 7.1) plus 0.5 M EGTA. O2 consumption rates in the presence of 4 mM ADP to assess state 3 respiration through complex I and 10 mM succinate to assess state 3 S respiration through complex II were measured in the presence of 0.018 mM palmitoylcarnitine (lipid), 0.01 mM blebbistatin, 0.02 mM palmitoyl CoA, 0.5 mM malate, and 5 mM carnitine in permeabilized muscle fiber bundles utilizing Buffer Z (plus 0.5M EGTA and 20 mM creatine monohydrate) on an Oroboros Oxygraph-2k system (Innsbruck, Austria) within 2-3 h of biopsy. Mitochondrial respiration values were normalized to dry muscle weight (pmol/s/mg dry weight per mL).
To assess cardiorespiratory fitness, a graded exercise test (GXT) was performed using a modified Bruce protocol [20]. Participants began by walking on a treadmill while speed and incline were gradually increased every 2 min as previously described [21]. Before and during the test, Respiratory Exchange Ratio (RER) was assessed using a non-rebreathing mask to capture oxygen and carbon dioxide levels. Cardiac rhythm was continuously monitored using a 12-lead electrocardiograph and at the end of each 2-min stage, blood pressure and rating of perceived exertion (RPE) were obtained. The test ended when participants reached volitional fatigue or met the absolute test termination criteria (RER> = 1.1, RPE 17, plateau in VO2 defined as 150 mL change from the last 60 s of the previous stage, and 90% HRmax). Maximal oxygen uptake was calculated relative to whole body mass (mL/kg/min). For the “VO2 max” analyses, we included individuals who met criteria for a “maximum” test (3/4 absolute test termination criteria met rather than just volitional fatigue) per ACSM guidelines [22].
Plasma biomarkers
Phlebotomy was performed at the KU Clinical and Translational Science Unit following an overnight fast. Whole blood was stored for APOE genotyping and further processed to generate plasma as previously described [11]. For this study, pTau181 was measured in plasma on a Simoa HD-X (Quanterix, Billerica, MA) according to the manufacturer’s instructions. Data on amyloid-β 42 (Aβ42), amyloid-β 40 (Aβ40), neurofilament light (NFL), and glial fibrillary acidic protein (GFAP) measured previously on the Simoa HD-X using the Neurology 4-Plex E kit were also statistically analyzed in relation to our new outcomes of interest [11].
Statistics
Mean group differences for continuous variables were assessed using ANOVA. Differences in nominal variables were assessed using a Chi-Square test. Linear associations between continuous variables were assessed using Pearson Correlation. All analyses were adjusted for age. Results were considered significant at p≤0.05.
RESULTS
Demographics
Individuals did not significantly differ based on demographic characteristics such as age, sex, education, or body mass index (Supplementary Table 1). Within the MCI group, the mean Mini-Mental State Examination score was 25 and 7 (46.7%) participants were taking the AD medication donepezil. There was no significant difference in lean mass between diagnostic groups.
Fig. 1
Apolipoprotein E (ApoE) expression in skeletal muscle and plasma phosphorylated tau181 (pTau181) expression are elevated in apolipoprotein ɛ4 (APOE4) carriers with mild cognitive impairment (MCI) and are positively correlated in all APOE4 carriers. Mean muscle ApoE±standard error measured by Western blot in relationship to diagnostic status (A). Plasma pTau181±standard error measured by Simoa-HDX immunoassay in relationship to diagnostic status (B). ApoE muscle content in relationship to plasma pTau181 levels (C). CH, cognitively healthy older adults; MCI, mild cognitive impairment. CH APOE4 carriers (n = 8-9), MCI APOE4 carriers (n = 14-15). *p < 0.001.
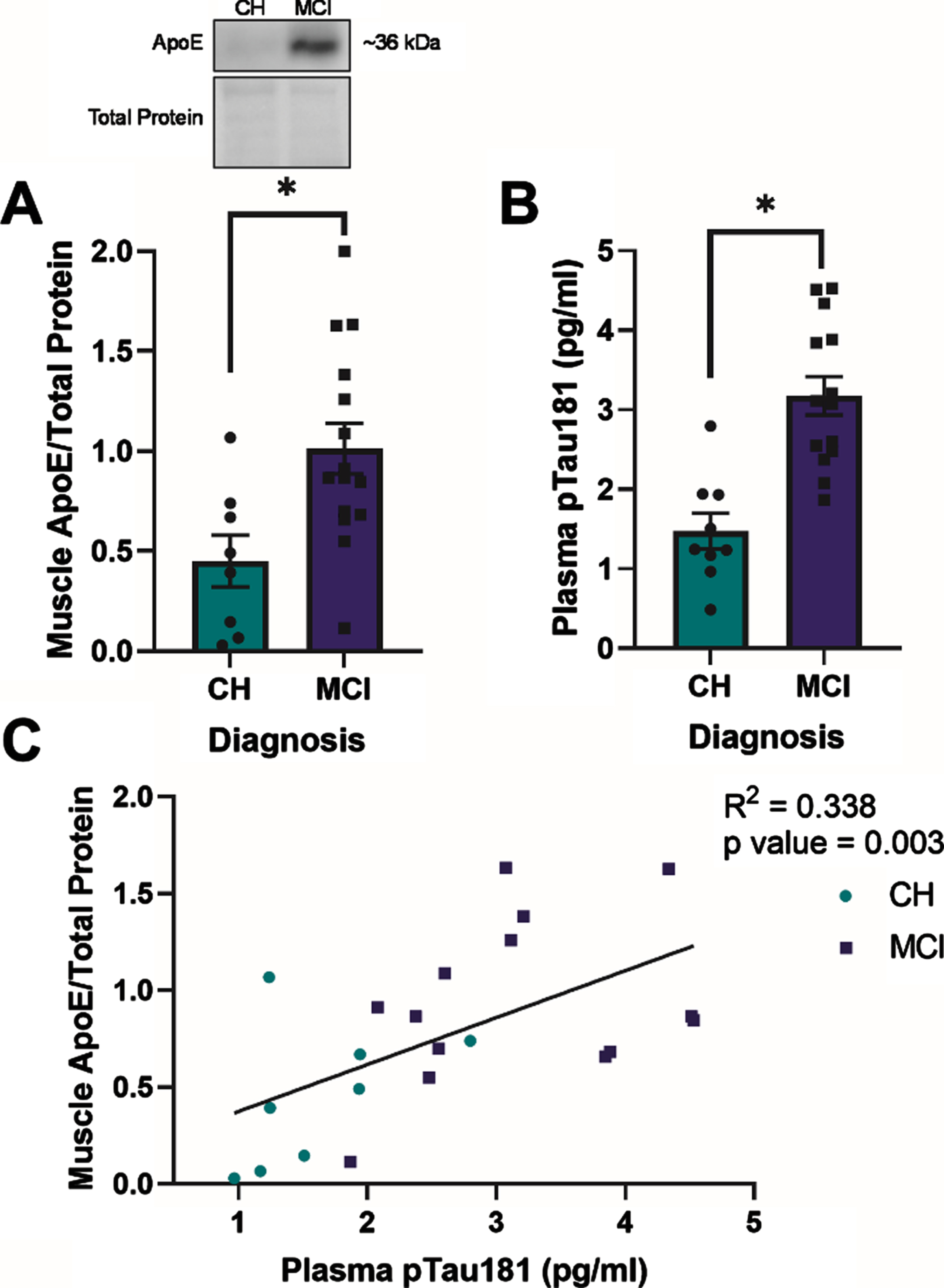
Apolipoprotein E expression in skeletal muscle and relationship with AD neuropathology blood biomarkers
ApoE expression in skeletal muscle (p = 0.013) and plasma pTau181 expression (p < 0.001) were higher in APOE4 carriers with MCI compared to those who were CH (Fig. 1A, B). In all APOE4 carriers, there was a positive correlation between muscle ApoE protein content and plasma pTau181 levels (R2 = 0.338, p = 0.003) (Fig. 1C). Muscle ApoE content also correlated with plasma GFAP (R2 = 0.175, p = 0.007) and plasma NFL (R2 = 0.270, p = 0.009) levels in all participants (Supplementary Figure 1A, B). There was no relationship between muscle ApoE content and plasma Aβ42 :40 (p > 0.05, Supplementary Figure 1C). Although we observed diagnostic differences in ApoE protein levels between groups, ApoE protein content did not correlate with lipid-stimulated mitochondrial respiration in the presence of ADP or succinate in either group (p > 0.05, data not shown).
Fig. 2
Heat shock protein 72 (Hsp72) expression negatively correlates with lipid-stimulated mitochondrial respiration in skeletal muscle of apolipoprotein ɛ4 (APOE4) carriers with mild cognitive impairment (MCI). Mean muscle Hsp72±standard error measured by Western blot in relationship to diagnostic status (A). Muscle Hsp72 in relationship to muscle State 3 (ADP) O2 flux (B) or State 3S (Succinate) O2 flux (C) measured by Oroboros. CH, cognitively healthy older adults; MCI, mild cognitive impairment. CH APOE4 carriers (n = 8), MCI APOE4 carriers (n = 15).
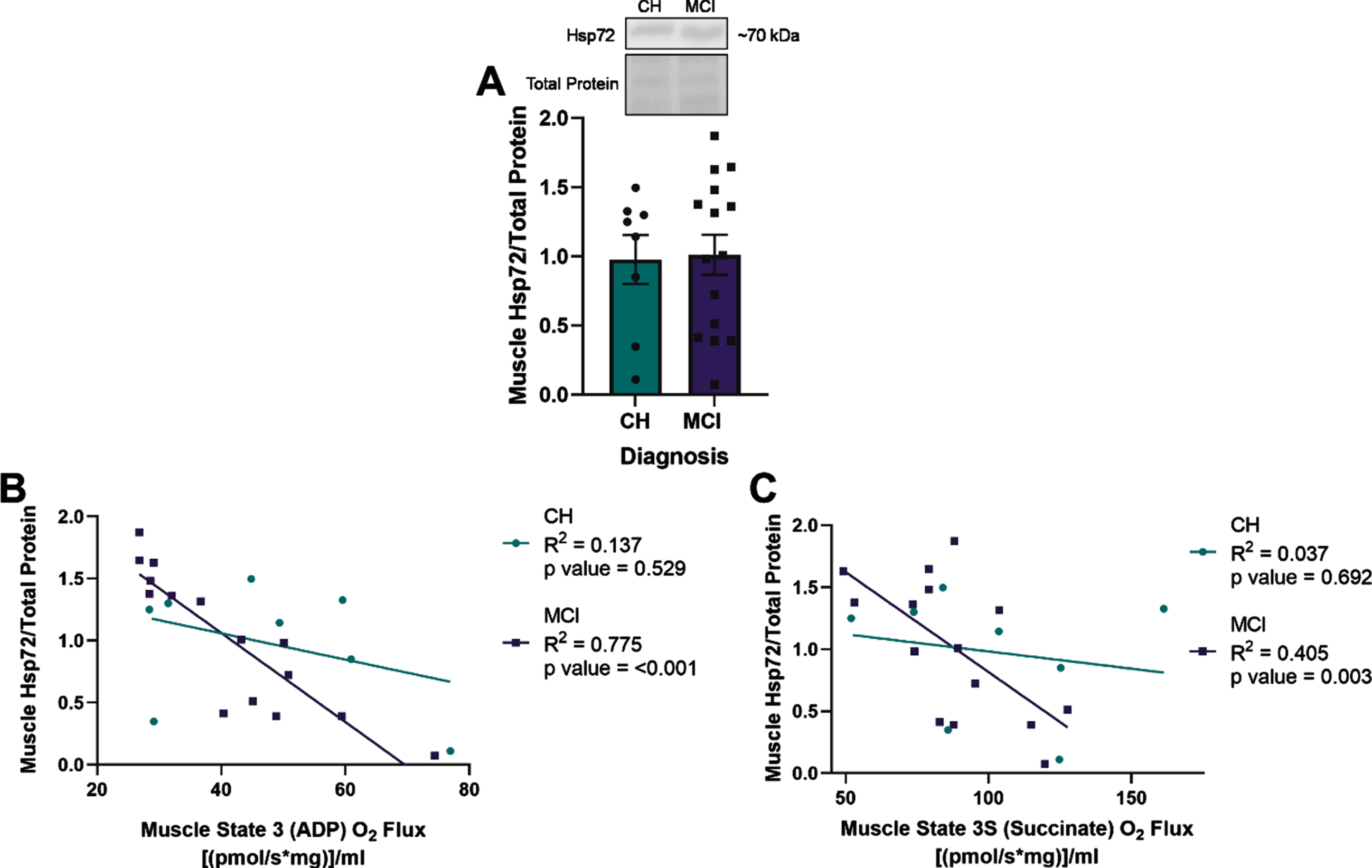
Heat shock response and relationship with mitochondrial respiration in skeletal muscle
ADP- and succinate-stimulated mitochondrial respiration were non-significantly reduced in APOE4 carriers with MCI compared to those who were CH (data not shown). There was no significant difference in muscle Hsp72 expression between diagnostic groups (Fig. 2A). However, we observed that Hsp72 expression negatively correlated with ADP (R2 = 0.775, p = <0.001) and succinate (R2 = 0.405, p = 0.003) stimulated mitochondrial respiration in the presence of palmitoyl-carnitine in skeletal muscle of APOE4 carriers with MCI, but not in those were CH (Fig. 2A, B). Hsp72 did not correlate with plasma AD neuropathology biomarkers (p > 0.05, Supplementary Figure 2).
AD neuropathology blood biomarkers and relationship with fitness
Cardiorespiratory fitness (VO2 max) did not significantly differ by diagnostic group in APOE4 carriers (Fig. 3A). However, plasma pTau181 (R2 = 0.389, p = 0.003, Fig. 3B) and GFAP (Supplementary Table 2) negatively correlated with VO2 max in all APOE4 carriers. Within diagnostic groups, plasma GFAP and NFL negatively correlated with VO2 max in APOE4 carriers who were cognitively healthy, but not in those with MCI (Supplementary Table 2). There was no relationship between Aβ42 :40 ratio and VO2 max.
Fig. 3
Plasma phosphorylated tau181 (pTau181) negatively correlates with VO2 max in all apolipoprotein ɛ4 (APOE4) carriers. VO2 max in relationship to diagnostic status (A). Plasma pTau181 measured by Simoa-HDX immunoassay in relationship to VO2 max in all APOE4 carriers (B). CH, cognitively healthy older adults; MCI, mild cognitive impairment. CH APOE4 carriers (n = 7), MCI APOE4 carriers (n = 14-15).
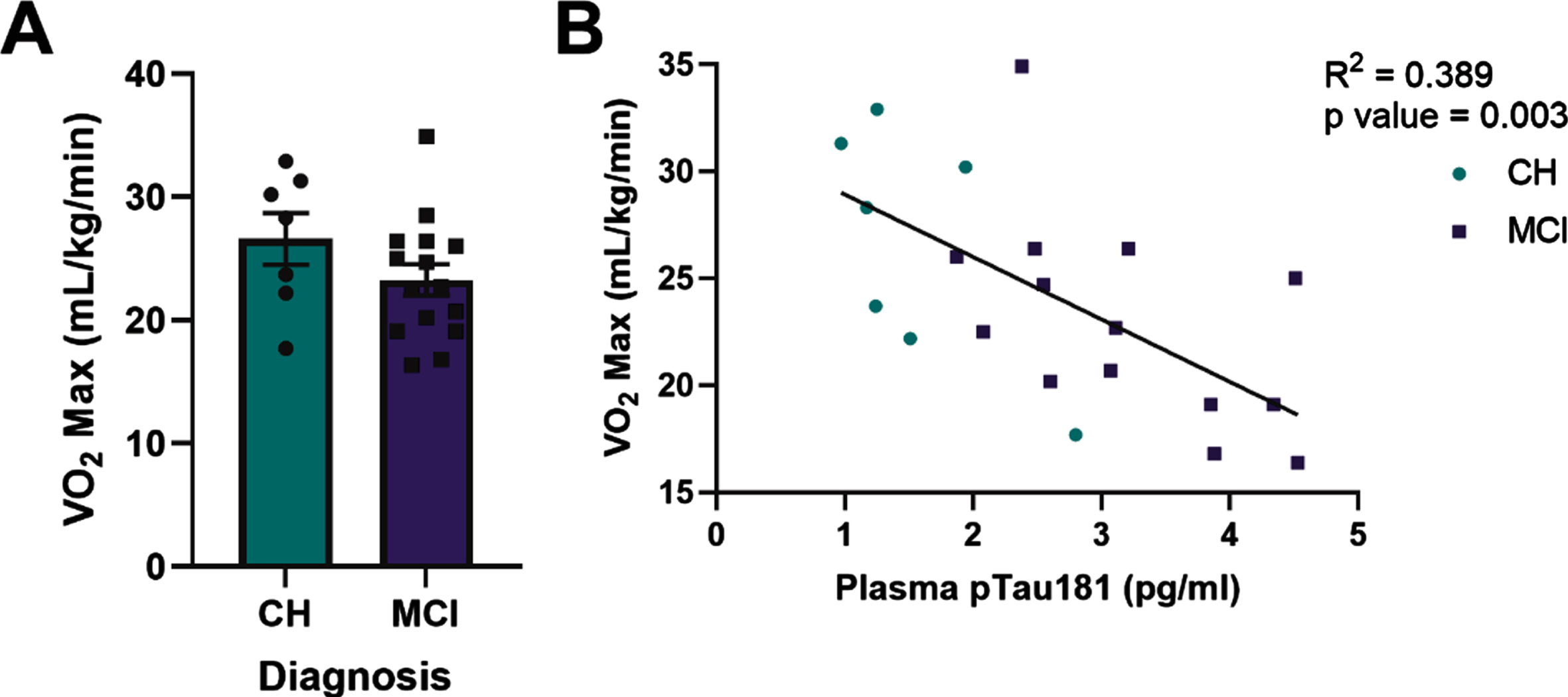
DISCUSSION
Research over the past few decades supports the notion that AD is a systemic disease [23]. Evidence that skeletal muscle mitochondrial respiration is reduced in the early stages of AD-related cognitive decline [11] extends previous studies that show mitochondrial and metabolic dysfunction in AD platelets and fibroblasts [24], and suggests that systemic metabolic tissues may influence or reflect AD progression. Skeletal muscle comprises 40–50% of total body mass and is a critical component for maintenance of physical function and metabolism of aging. In this study, we show for the first time that muscle ApoE levels are increased in APOE4 carriers with MCI compared to those who are CH and that Hsp72 levels negatively correlate with mitochondrial respiration in the muscle of APOE4 carriers with MCI, but not in those who are CH. We further show that the plasma AD biomarker, pTau181 negatively tracks with cardiorespiratory fitness in all APOE4 carriers. By demonstrating skeletal muscle changes in proteins implicated in responding to metabolic and oxidative stress in APOE4 carriers, we contribute novel information to the existing literature on systemic alterations in AD.
Recent animal studies have focused on the role of APOE4 in modulating systemic metabolism [12, 25, 26]. In addition to impaired spatial learning [25], mice expressing human APOE4 have impaired glucose clearance at lower body weights [25], increased plasma insulin [26], elevated levels of fasting plasma non-esterified fatty acids [12], and altered skeletal muscle expression of proteins related to mitochondrial energy metabolism [12]. Similarly, human APOE4 carriers have higher plasma triglyceride levels in the fasted and post-prandial state in addition to elevated post-prandial glucose [27]. Although the role of ApoE4 protein levels in modulating systemic metabolic changes is unclear, human APOE4 carriers have altered plasma levels of ApoE compared to non-carriers [28]. Further, the expression of ApoE4 fragments in Neuro-2a cells reduces the expression of Mitotracker red, a marker of mitochondrial function and integrity, in a dose-dependent manner [18]. We therefore were not surprised to find differences in ApoE protein levels in MCI compared to CH individuals [11].
As mentioned, muscle ApoE protein is elevated in APOE4 carriers with MCI compared to those who are CH. While increased levels of muscle ApoE may still contribute to reduced muscle mitochondrial respiration observed in MCI, ApoE protein levels did not correlate with ADP-stimulated or succinate-stimulated mitochondrial respiration utilizing lipids as a substrate in either group. This lack of relationship may be due to our limited sample size, medication use [11], or other factors. Preclinical models have shown that APOE4 alters the mobilization and utilization of fatty acids by adipose and skeletal muscle tissue [12, 26]. APOE4 transgenic mice preferentially mobilize fats from adipose tissue and increase the expression of proteins needed to oxidize fats in skeletal muscle [12]. Excess flux of lipids into muscle mitochondria and generation of reactive oxygen species may therefore be another mechanism by which mitochondrial respiration is impaired in MCI. APOE4 is associated with excess oxidative stress [29], which is handled by cells through various mechanisms, including heat shock proteins. Hsp72 may mitigate reductions in mitochondrial function in some MCI individuals who have elevated expression of ApoE in skeletal muscle.
Hsp72 is a chaperone protein whose expression is activated in response to cellular insults including misfolded proteins, oxidative stress, and reduced ATP availability [15, 16]. Hsp72 protein is elevated in the temporal cortex of individuals with AD [13, 14], suggesting activation of this system in response to AD-related cellular stress. While no studies have measured muscle Hsp72 content in skeletal muscle of individuals with AD, overexpression of Hsp72 in mouse skeletal muscle improves whole-body VO2, muscle mitochondrial-content and -respiration [30], while mice lacking Hsp72 display reduced oxygen consumption and fatty acid oxidation in muscle [31]. Since this protein is activated in the AD brain and because mitochondrial dysfunction can stimulate the heat shock response [15, 17], we hypothesized that Hsp72 would be elevated in MCI muscle compared to CH. The lack of difference in Hsp72 muscle levels between CH and MCI APOE4 carriers may be due to confounding effects of AD medications [11] or the availability of compensatory heat shock proteins [16] to deal with mitochondrial stress in some individuals. Detection of group differences in the expression of Hsp72 may also be limited by a state of chronic adaptation and compensation in these human samples. Compensatory mechanisms may also explain the absence of a statistically significant correlation between muscle Hsp72 and plasma AD biomarkers. Despite the lack of between-group differences in Hsp72 levels, the negative correlation between Hsp72 and lipid-stimulated mitochondrial respiration in skeletal muscle of APOE4 carriers with MCI supports a role for Hsp72 in responding to mitochondrial dysfunction during cognitive decline. In a cognitively healthy state, there may be compensatory stress-responsive mechanisms other than Hsp72 available to deal with impaired mitochondrial respiration. In contrast, MCI may represent a state of increased reliance on the Hsp72 chaperone system to respond to mitochondrial stress due to a failure of other pathways. Further studies are needed to determine if systemic activation of Hsp72 in APOE4 carriers with low mitochondrial respiration and MCI is protective against declines in mitochondrial activity and if these changes are representative of or influence changes in the brain.
Increasing expression of Hsp72 in response to declining mitochondrial respiration in skeletal muscle of APOE4 carriers with MCI may also represent increased oxidative stress, since poorly functioning mitochondria produce excess reactive oxygen species that can damage a cell by oxidizing membrane lipids, proteins, and DNA [32]. We therefore hypothesized that proteins associated with metabolic/oxidative stress in muscle would be related to metabolic/oxidative stress related pathology in the brain. Oxidative stress increases the phosphorylation of tau [32], although the extent to which metabolic and oxidative stress in peripheral tissues reflects tau phosphorylation in the brain is not clear. We hypothesized that plasma pTau181, a biomarker for tau hyperphosphorylation in the brain, would track with Hsp72 expression in skeletal muscle of APOE4 carriers. As expected, plasma pTau181 expression was higher in MCI compared to CH APOE4 carriers, consistent with data showing that tau accumulation tracks with cognitive decline [33]. It is also known that ApoE4 enhances tau phosphorylation [34]. Although muscle Hsp72 did not correlate with plasma pTau181 in APOE4 carriers, the negative correlation between plasma pTau181 levels and VO2 max in all APOE4 carriers supports a potential connection between brain neuropathology and whole-body metabolism. The importance of Hsp72 localization [35] may underlie the lack of association between whole-muscle cell Hsp72 measured in our study and plasma pTau181.
Although the interpretation of our results is limited by its cross-sectional design and small sample size, the strengths of this study should be noted. This study is one of few to utilize human skeletal muscle tissue to assess differences related to cognitive status in systemic tissues. This data is paired with rich AD biomarker data that reflects expected differences between diagnostic groups. Furthermore, we demonstrate diagnostic dependent relationships in a highly metabolic and abundant tissue that can be used to help further our understanding of how whole-body metabolism is related to cognitive function in APOE4 carriers. As this was an exploratory study, we were limited by the existing sample size. Future studies are needed to confirm these results and determine the implications of these findings.
The effects of APOE4 are not limited to the brain. APOE4 increases the risk of metabolic syndrome [36] and diseases characterized by peripheral metabolic dysfunction such as obesity and type 2 diabetes, are also risk factors for AD [37]. Therefore, understanding the systemic metabolic effects of APOE4 is crucial to elucidating the mechanisms by which carrying an APOE4 allele contributes to cognitive decline. Our work reveals diagnostic dependent differences in skeletal muscle between individuals who are CH or have been diagnosed with MCI but both carry the APOE4 genotype. This raises the potential for skeletal muscle to be used as an early, minimally invasive biomarker for neuropathologic and cognitive changes. Furthermore, our work adds to the growing body of literature demonstrating systemic changes in AD.
ACKNOWLEDGMENTS
We would like to thank our research participants who generously gave their time and effort to make this study possible.
FUNDING
This work was supported by the National Institute on Aging through R21AG056062 (JPT), R01AG062548 (JKM), P30 AG072973 (RHS), P30 AG035982 (RHS) and a Biomedical Research Training Program Award from the University of Kansas Medical Center (CNJ). Additional support is provided by the Margaret “Peg” McLaughlin and Lydia A. Walker Opportunity Fund.
CONFLICT OF INTEREST
The authors have no conflict of interest to report.
DATA AVAILABILITY
The dataset used in the current study has been uploaded to the Harvard Dataverse repository and will be made publicly available at https://doi.org/10.7910/DVN/VDBMPPfollowingpublication.
SUPPLEMENTARY MATERIAL
[1] The supplementary material is available in the electronic version of this article: https://dx.doi.org/10.3233/JAD-221192.
REFERENCES
[1] | ((2022) ) 2022 Alzheimer’s disease facts and figures. Alzheimers Dement 18: , 700–789. |
[2] | Neth BJ , Craft S ((2017) ) Insulin resistance and Alzheimer’s disease: Bioenergetic linkages. Front Aging Neurosci 9: , 345. |
[3] | Burns JM , Cronk BB , Anderson HS , Donnelly JE , Thomas GP , Harsha A , Brooks WM , Swerdlow RH ((2008) ) Cardiorespiratory fitness and brain atrophy in early Alzheimer disease. Neurology 71: , 210–216. |
[4] | Razay G , Vreugdenhil A , Wilcock G ((2007) ) The metabolic syndrome and Alzheimer disease. Arch Neurol 64: , 93–96. |
[5] | Mosconi L , Nacmias B , Sorbi S , De Cristofaro MT , Fayazz M , Tedde A , Bracco L , Herholz K , Pupi A ((2004) ) Brain metabolic decreases related to the dose of the ApoE e4 allele in Alzheimer’s disease. J Neurol Neurosurg Psychiatry 75: , 370–376. |
[6] | Sima A , Iordan A , Stancu C ((2007) ) Apolipoprotein E polymorphism–a risk factor for metabolic syndrome. Clin Chem Lab Med 45: , 1149–1153. |
[7] | Craft S , Asthana S , Schellenberg G , Baker L , Cherrier M , Boyt AA , Martins RN , Raskind M , Peskind E , Plymate S ((2000) ) Insulin effects on glucose metabolism, memory, and plasma amyloid precursor protein in Alzheimer’s disease differ according to apolipoprotein-E genotype. Ann N Y Acad Sci 903: , 222–228. |
[8] | Huang TL , Zandi PP , Tucker KL , Fitzpatrick AL , Kuller LH , Fried LP , Burke GL , Carlson MC ((2005) ) Benefits of fatty fish on dementia risk are stronger for those without APOE ɛ4. Neurology 65: , 1409–1414. |
[9] | Ivy JL , Costill DL , Maxwell BD ((1980) ) Skeletal muscle determinants of maximum aerobic power in man. Eur J Appl Physiol Occup Physiol 44: , 1–8. |
[10] | Merz KE , Thurmond DC ((2020) ) Role of skeletal muscle in insulin resistance and glucose uptake. Compr Physiol 10: , 785–809. |
[11] | Morris JK , McCoin CS , Fuller KN , John CS , Wilkins HM , Green ZD , Wang X , Sharma P , Burns JM , Vidoni ED , Mahnken JD , Shankar K , Swerdlow RH , Thyfault JP ((2021) ) Mild cognitive impairment and donepezil impact mitochondrial respiratory capacity in skeletal muscle. Function (Oxf) 2: , zqab045. |
[12] | Huebbe P , Dose J , Schloesser A , Campbell G , Glüer CC , Gupta Y , Ibrahim S , Minihane AM , Baines JF , Nebel A , Rimbach G ((2015) ) Apolipoprotein E (APOE) genotype regulates body weight and fatty acid utilization-Studies in gene-targeted replacement mice. Mol Nutr Food Res 59: , 334–343. |
[13] | Koren J , 3rd, Jinwal UK , Lee DC , Jones JR , Shults CL , Johnson AG , Anderson LJ , Dickey CA ((2009) ) Chaperone signalling complexes in Alzheimer’s disease. J Cell Mol Med 13: , 619–630. |
[14] | Yoo BC , Seidl R , Cairns N , Lubec G ((1999) ) Heat-shock protein 70 levels in brain of patients with Down syndrome and Alzheimer’s disease. J Neural Transm Suppl 57: , 315–322. |
[15] | Van Why SK , Mann AS , Thulin G , Zhu XH , Kashgarian M , Siegel NJ ((1994) ) Activation of heat-shock transcription factor by graded reductions in renal ATP, in vivo, in the rat. J Clin Invest 94: , 1518–1523. |
[16] | Kregel KC ((2002) ) Heat shock proteins: Modifying factors in physiological stress responses and acquired thermotolerance. J Appl Physiol (1985) 92: , 2177–2186. |
[17] | Barrett MJ , Alones V , Wang KX , Phan L , Swerdlow RH ((2004) ) Mitochondria-derived oxidative stress induces a heat shock protein response. J Neurosci Res 78: , 420–429. |
[18] | Chang S , ran Ma T , Miranda RD , Balestra ME , Mahley RW , Huang Y ((2005) ) Lipid- and receptor-binding regions of apolipoprotein E4 fragments act in concert to cause mitochondrial dysfunction and neurotoxicity. Proc Natl Acad Sci U S A 102: , 18694–18699. |
[19] | Miyata M , Smith JD ((1996) ) Apolipoprotein E allele-specific antioxidant activity and effects on cytotoxicity by oxidative insults and beta-amyloid peptides. Nat Genet 14: , 55–61. |
[20] | Billinger SA , Vidoni ED , Greer CS , Graves RS , Mattlage AE , Burns JM ((2014) ) Cardiopulmonary exercise testing is well tolerated in people with Alzheimer-related cognitive impairment. Arch Phys Med Rehabil 95: , 1714–1718. |
[21] | Morris JK , Vidoni ED , Johnson DK , Van Sciver A , Mahnken JD , Honea RA , Wilkins HM , Brooks WM , Billinger SA , Swerdlow RH , Burns JM ((2017) ) Aerobic exercise for Alzheimer’s disease: A randomized controlled pilot trial. PLoS One 12: , e0170547. |
[22] | Liguori G (2022) ACSM’s Guidelines for Exercise Testing and Prescription, 11 ed. Wolters Kluwer Health. |
[23] | Morris JK , Honea RA , Vidoni ED , Swerdlow RH , Burns JM ((2014) ) Is Alzheimer’s disease a systemic disease? Biochim Biophys Acta 1842: , 1340–1349. |
[24] | Swerdlow RH ((2012) ) Mitochondria and cell bioenergetics: Increasingly recognized components and a possible etiologic cause of Alzheimer’s disease. Antioxid Redox Signal 16: , 1434–1455. |
[25] | Jones NS , Watson KQ , Rebeck GW ((2019) ) Metabolic disturbances of a high-fat diet are dependent on APOE genotype and sex. eNeuro 6: , ENEURO.0267-19.2019. |
[26] | Arbones-Mainar JM , Johnson LA , Torres-Perez E , Garcia AE , Perez-Diaz S , Raber J , Maeda N ((2016) ) Metabolic shifts toward fatty-acid usage and increased thermogenesis are associated with impaired adipogenesis in mice expressing human APOE4. Int J Obes (Lond) 40: , 1574–1581. |
[27] | Dart A , Sherrard B , Simpson H ((1997) ) Influence of apo E phenotype on postprandial triglyceride and glucose responses in subjects with and without coronary heart disease. Atherosclerosis 130: , 161–170. |
[28] | Martínez-Morillo E , Hansson O , Atagi Y , Bu G , Minthon L , Diamandis EP , Nielsen HM ((2014) ) Total apolipoprotein E levels andspecific isoform composition in cerebrospinal fluid and plasma fromAlzheimer’s disease patients and controls. Acta Neuropathol 127: , 633–643. |
[29] | Orr AL , Kim C , Jimenez-Morales D , Newton BW , Johnson JR , Krogan NJ , Swaney DL , Mahley RW ((2019) ) Neuronal apolipoprotein E4 expression results in proteome-wide alterations and compromises bioenergetic capacity by disrupting mitochondrial function. J Alzheimers Dis 68: , 991–1011. |
[30] | Henstridge DC , Bruce CR , Drew BG , Tory K , Kolonics A , Estevez E , Chung J , Watson N , Gardner T , Lee-Young RS , Connor T , Watt MJ , Carpenter K , Hargreaves M , McGee SL , Hevener AL , Febbraio MA ((2014) ) Activating HSP72 in rodent skeletal muscle increases mitochondrial number and oxidative capacity and decreases insulin resistance. Diabetes 63: , 1881–1894. |
[31] | Drew BG , Ribas V , Le JA , Henstridge DC , Phun J , Zhou Z , Soleymani T , Daraei P , Sitz D , Vergnes L , Wanagat J , Reue K , Febbraio MA , Hevener AL ((2014) ) HSP72 is a mitochondrial stress sensor critical for Parkin action, oxidative metabolism, and insulin sensitivity in skeletal muscle. Diabetes 63: , 1488–1505. |
[32] | Mondragón-Rodríguez S , Perry G , Zhu X , Moreira PI , Acevedo-Aquino MC , Williams S ((2013) ) Phosphorylation of tau proteinas the link between oxidative stress, mitochondrial dysfunction, andconnectivity failure: Implications for Alzheimer’s disease. Oxid Med Cell Longev 2013: , 940603. |
[33] | Gomes LC , Benedetto G , Scorrano L ((2011) ) During autophagy mitochondria elongate, are spared from degradation and sustain cell viability. Nat Cell Biol 13: , 589–598. |
[34] | Brecht WJ , Harris FM , Chang S , Tesseur I , Yu GQ , Xu Q , Dee Fish J , Wyss-Coray T , Buttini M , Mucke L , Mahley RW , Huang Y ((2004) ) Neuron-specific apolipoprotein e4 proteolysis is associated with increased tau phosphorylation in brains of transgenic mice. J Neurosci 24: , 2527–2534. |
[35] | Ellis S , Killender M , Anderson RL ((2000) ) Heat-induced alterations in the localization of HSP72 and HSP73 as measured by indirect immunohistochemistry and immunogold electron microscopy. J Histochem Cytochem 48: , 321–332. |
[36] | Torres-Perez E , Ledesma M , Garcia-Sobreviela MP , Leon-Latre M , Arbones-Mainar JM ((2016) ) Apolipoprotein E4 association with metabolic syndrome depends on body fatness. Atherosclerosis 245: , 35–42. |
[37] | Profenno LA , Porsteinsson AP , Faraone SV ((2010) ) Meta-analysis of Alzheimer’s disease risk with obesity, diabetes, and related disorders. Biol Psychiatry 67: , 505–512. |