Effect of Acetylcholinesterase Inhibitors on Cerebral Perfusion and Cognition: A Systematic Review
Abstract
Background:
Perfusion imaging has the potential to identify neurodegenerative disorders in a preclinical stage. However, to correctly interpret perfusion-derived parameters, the impact of perfusion modifiers should be evaluated.
Objective:
In this systematic review, the impact of acute and chronic intake of four acetylcholinesterase inhibitors (AChEIs) on cerebral perfusion in adults was investigated: physostigmine, donepezil, galantamine, and rivastigmine.
Results:
Chronic AChEI treatment results in an increase of cerebral perfusion in treatment-responsive patients with Alzheimer’s disease, dementia with Lewy bodies, and Parkinson’s disease dementia in the frontal, parietal, temporal, and occipital lobes, as well as the cingulate gyrus. These effects appear to be temporary, dose-related, and consistent across populations and different AChEI types. On the contrary, further perfusion decline was reported in patients not receiving AChEIs or not responding to the treatment.
Conclusion:
AChEIs appear to be a potential perfusion modifier in neurodegenerative patients. More research focused on quantitative perfusion in both patients with and without a cholinergic deficit is needed to draw conclusions on whether AChEI intake should be considered when analyzing perfusion data.
INTRODUCTION
Dementia is an undeniable concern for aging societies, with a predicted doubling of cases within the next quarter of the century, accompanied by an enormous personal and financial burden on patients, family, and society [1]. Dementia patients are expected to benefit from therapy that intervenes as early as possible [2]. Unfortunately, diagnostic uncertainty currently entails a delay of about one year from symptom onset to diagnosis, preventing early treatment [3–5]. On top of that, research shows dementia-related cerebral changes up to twenty years before the start of the first symptoms [6]. Therefore, evaluation of clinical endpoints to start treatment might not be adequate if degeneration is no longer salvageable at that stage.
With this in mind, quantifiable and easily accessible biomarkers could play an important role in the early detection, monitoring of disease progression and the assessment of treatment response [7]. A growing arsenal of biomarkers, such as perfusion imaging, provides new insights into dementia pathology and etiology [6, 8]. Perfusion imaging techniques have been available for more than 20 years, but widespread use in routine clinical practice has never been achieved. This is partially due to the large between- and within-subject variability in perfusion due to physiology, lifestyle and medication use, which might confound pathology at very early stages [9].
To interpret and quantify perfusion-derived parameters, the impact of those perfusion modifiers should be evaluated, ensuring the presence of true disease-related changes. Recently, Clement et al. (2018) enlisted and evaluated the impact of non-medication-related perfusion modifiers on cerebral perfusion and proposed a standard operating procedure to take those into account. However, perfusion changes caused by medication were not yet included [9].
Therefore, this systematic review aims to investigate the impact of acute and chronic intake of acetylcholinesterase inhibitors (AChEIs) on global and regional cerebral perfusion in patients with mild cognitive impairment (MCI), Alzheimer’s disease (AD), and healthy controls. AChEIs inhibit the breakdown of acetylcholine, which is known to play an important role in the modulation of regional cerebral perfusion (rCBF). The purpose is to evaluate the perfusion modifying effects of AChEIs, we refer to the review of Versijpt et al. [10] for an in-depth overview of the cognitive effects of AChEIs.
METHODS
A systematic review was conducted, after registration on PROSPERO (Registration number: CRD42020158625) [11]. The review was completed according to the Preferred Reporting Items for Systematic Reviews and Meta-Analysis (PRISMA) [12].
Eligibility criteria
Studies evaluating perfusion changes in patients taking AChEIs were included. The main inclusion and exclusion criteria are described in Table 1.
Table 1
In- and exclusion criteria applied to the full texts of the published and unpublished reports
Category | Inclusion criteria | Exclusion criteria |
Population | Adults, both healthy individuals as patients with AD or MCI. | Children and adolescents younger than 18 years, animal research and studies involving only a subset of relevant participants. |
Intervention | Licensed acetylcholinesterase inhibitors. Acute and chronic intake, as well as the termination of intake (withdrawal effects) | studies involving only a subset of relevant participants, if results cannot be assessed separately and studies assessing perfusion during cognitive tasks |
Comparator | Placebo, baseline measurement or other acetylcholinesterase inhibitors | |
Outcome | Global and/or regional cerebral perfusion in rest (micro- and macrovascular level). | |
Study types | All types of studies will be included |
Search strategy
An extensive, electronic literature search was conducted using the Cochrane Central Register of Controlled Trials, MEDLINE via the PubMed interface, EMBASE via the Embase interface, and Web of Science. The search strategy (Supplementary Material 1) included the following categories: cerebral perfusion imaging, AChEIs and adults. Keywords and MeSH terms relating to these categories were used to optimize the search strategy. All reports published up to October 4, 2022 were considered. To identify additional published, unpublished, and ongoing trials, the US National Institutes of Health Ongoing Trials Register, the ClinicalTrials.gov (http://www.clinicaltrials.gov; searched March 21, 2022) and the World Health Organization International Clinical Trials Registry Platform (WHO ICTRP) (http://apps.who.int/trialsearch) were searched (last search date: March 21, 2022). Additionally, experts and authors in the field were contacted to provide unpublished results. Finally, the reference lists of the identified studies and the Science Citation Index Cited Reference were explored for forward tracking of included studies.
Study selection and inclusion criteria
The titles and abstracts of all published and unpublished reports were evaluated for eligibility by two investigators independently, using Rayyan QCRI (Qatar Computing Research Institute, Doha, Qatar). Observer differences were resolved by consensus. Next, the full text of the remaining reports was evaluated for eligibility. No publication year, language, study design or type of perfusion measurement technique restrictions were applied.
Data extraction and risk of bias analysis
The following details of the included studies were extracted by a trained investigator, using a pilot-tested, review-specific data-extraction form: report and study design, participants eligibility, group-specific intervention, perfusion measuring methods, outcome, and risk of bias. The primary outcome was global and regional cerebral perfusion changes, caused by acute or chronic AChEIs treatment, and withdrawal after treatment termination, compared to baseline or placebo treatment, for each specific AChEI (i.e., physostigmine, donepezil, rivastigmine, galantamine). The secondary outcome was the relationship between these effects and clinical treatment response.
Risk of bias (ROB) was assessed using the ‘Risk of Bias in Non-Randomised Studies –of interventions’ (ROBINS-I) for non-randomized and observational studies [13, 14] and implemented in the data-extraction form. Each study was assessed for potential bias, as low, moderate, serious, or critical risk (Supplementary Material 2). No articles were excluded based on the ROB.
Synthesis and analysis
A qualitative analysis was performed by evaluating the results of each report in a structured manner in accordance with the review of Clement et al. [9]. This systematic review includes both AChEIs elicited perfusion changes, and their effect on cognition.
The narrative synthesis is ordered in different levels: 1) intake frequency (acute versus chronic intake); 2) study time point (baseline versus follow-up); and 3) rCBF change per brain region. Additionally, the cognitive response, the role of treatment responsiveness, duration and dosage was also assessed for chronic AChEIs intake.
RESULTS
A total of 1,254 studies were identified in the initial literature search. A flow diagram of the study selection process is shown in Fig. 1. An overview of study characteristics is provided in Fig. 2.
Fig. 1
The PRISMA flow diagram of the selection of studies included in this systematic review. WoS, Web Of Science, nreports, number of reports.
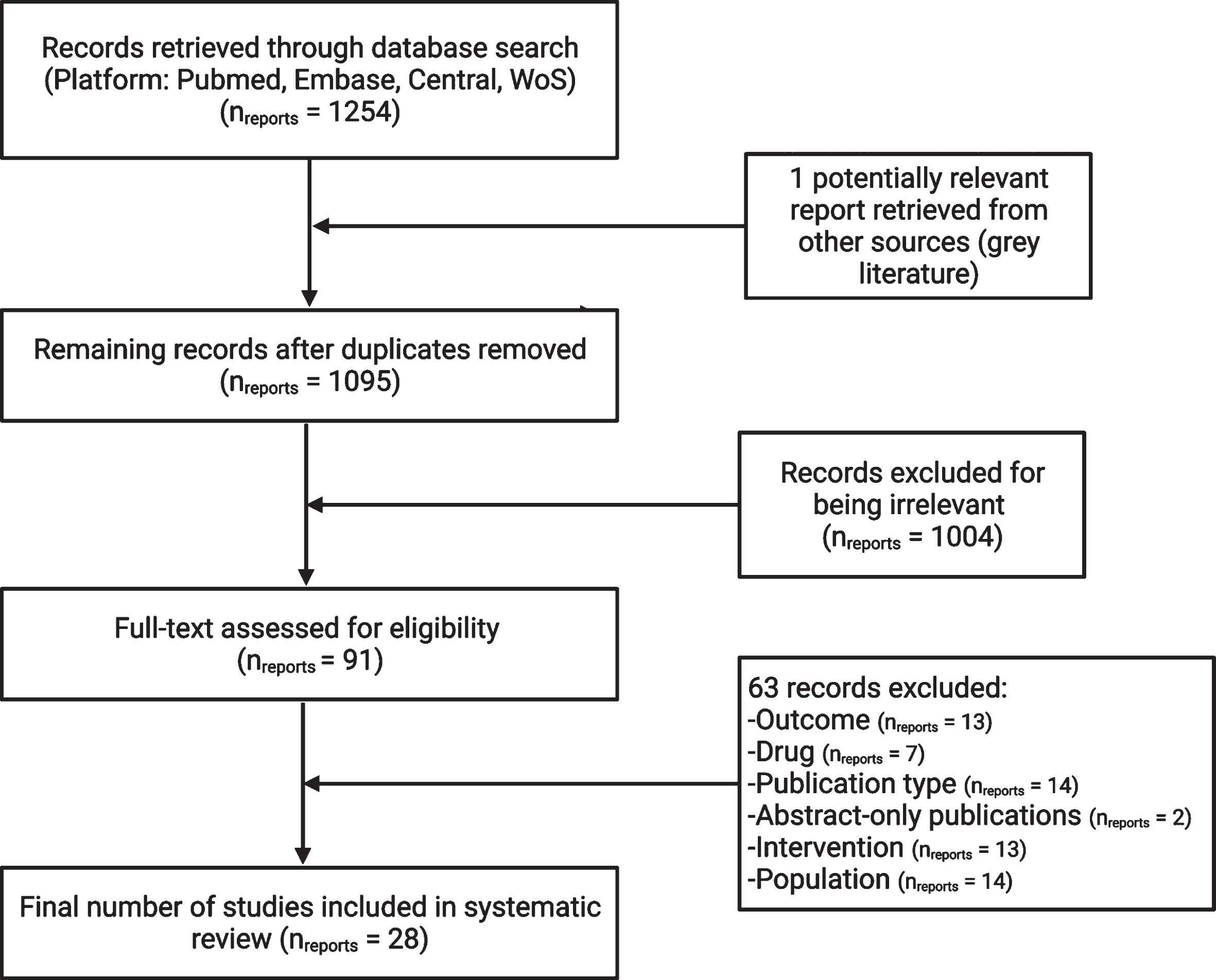
Fig. 2
Visual representation of characteristics of the 28 included studies. A total of 485 participants were identified, 3 imaging techniques and 4 drugs have been used to evaluate perfusion. Perfusion was assessed, either after a one-off intake of a single dose or after a long-term intake of AChEIs. AD, Alzheimer’s disease; MCI, mild cognitive impairment; ASL, arterial spin labeling; PET, positron emission tomography; SPECT, single-photon emission computerized tomography; n, number.
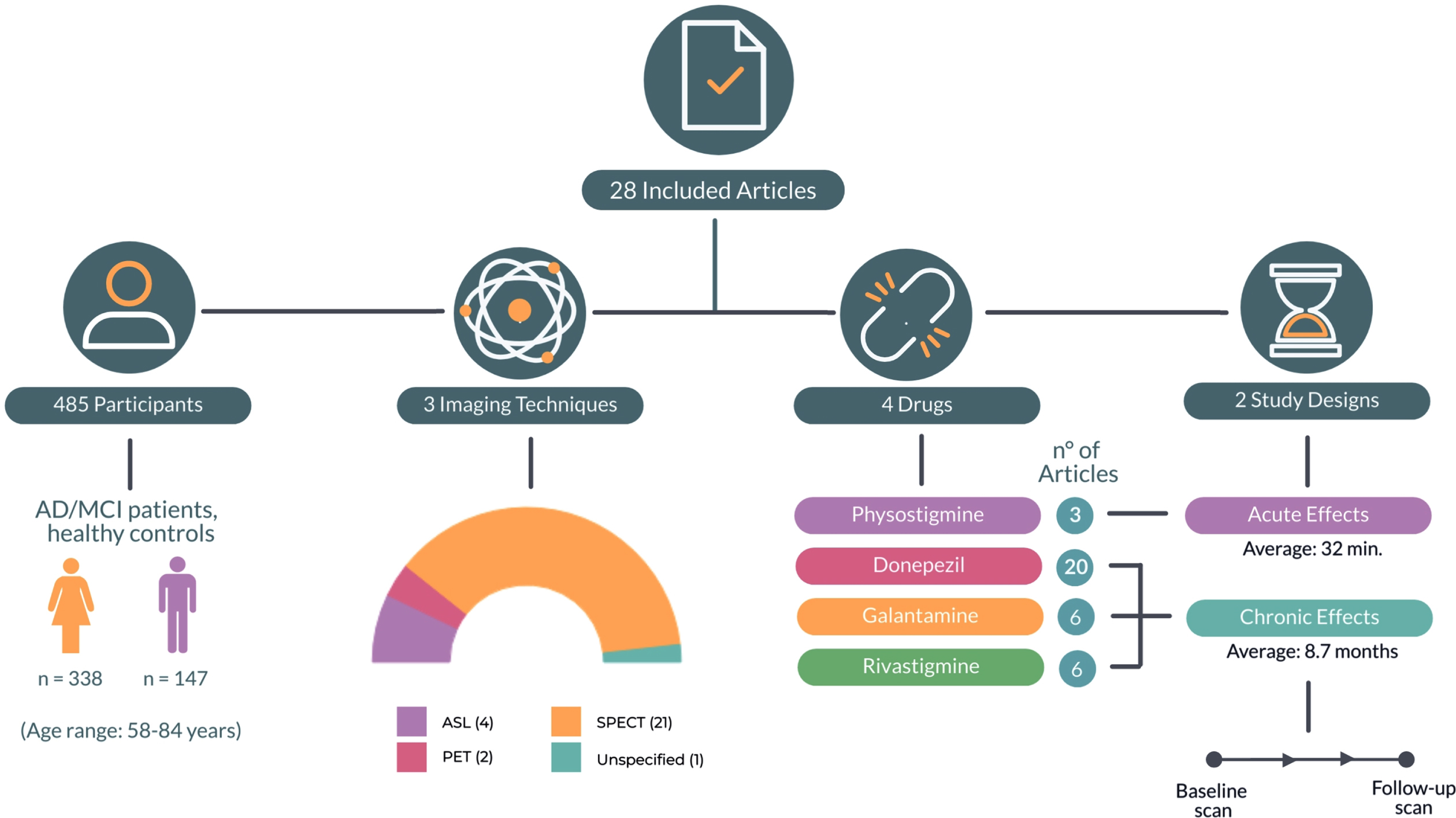
Perfusion was mainly assessed, either after an on-off intake of a single dose (number of reports (nreports) = 3, 10.7%) [15–17] of AChEI, representing the acute effects on perfusion, or after long-term AChEIs intake (nreports = 25, 89.3%) [18–42], investigating the chronic effects. No articles on withdrawal were found. When assessing acute effects, the impact of physostigmine on cerebral perfusion was investigated, 10 min to 2 h after treatment (average follow-up (FU): 32 min) [15–17]. The chronic effects of donepezil (nreports = 20) [18–22, 28–42], rivastigmine (nreports = 6) [19, 21, 24–27], and galantamine (nreports = 6) [18–23], were investigated with an average waiting time of 8.7 months (ranging between three and 18 months). 21 studies [18, 20, 21, 23–36, 38, 40–42] investigated and compared the cerebral perfusion effects of a single drug, whereas 4 studies [19–22] compared the effects of different AChEIs by using multiple treatment groups. Only results with a significance level of 0.05 were reported.
Three imaging techniques have been utilized to evaluate cerebral perfusion: arterial spin labeling magnetic resonance imaging (ASL MRI) (nreports = 4) [19, 28, 29, 32], positron emission tomography (PET) (nreports = 2) [17, 23], and technetium-99m hexamethyl propylenamine oxime single photon emission tomography (SPECT) (nreports = 21) [16, 18, 20–22, 24–27, 29, 31, 33–42]. The imaging technique was not specified in one publications [15].
The risk of bias of the included studies was high due to pre-selection of the study population or the lack of analysis methods for the control of (baseline) confounding factors.
Acute perfusion modifying effects of AChEIs
Three studies investigated the effects of physostigmine on cerebral perfusion in patients with AD [15–17] and in healthy individuals [16]. Each participant was scanned before and after the administration of a single dose of physostigmine. Physostigmine induced a small, but significant, perfusion increase in the frontal cortex. A significant, larger perfusion increase in the parietal and temporal cortices was seen in AD patients [15–17], but not in healthy individuals [16]. One study reported an increase in the occipital cortex in AD patients, and in the cerebellum, the striatum, and the thalamus [17].
Effects of chronic intake of acetylcholinesterase inhibitors
Twenty-five studies investigated the effects of chronic AChEI intake on rCBF in healthy individuals (npatients = 138) [19–21, 23, 26, 27, 29, 36] and AD patients (npatients = 455) [18–42]. Perfusion was measured both at baseline and after a FU period.
Baseline cerebral perfusion
At baseline, a significant regional hypoperfusion in the temporoparietal cortices was observed, extending to the bilateral posterior cingulate gyri in AD patients compared to healthy individuals. Additional frontal lobe involvement was reported in more advanced disease stages [19, 20, 22, 24, 30, 34, 40, 41].
Cerebral perfusion after chronic intake of AChEIs
Perfusion changes were reported in the frontal cortex (nreports = 19/25, 18 ↑, 1 ↓), parietal cortex (nreports = 7/25, 5 ↑, 2 ↓), temporal cortex (nreports = 12/25, 9 ↑, 3 ↓), occipital cortex (nreports = 10/25, 9 ↑, 1 ↓), anterior cingulate cortex (ACC) (nreports = 5/25, 3 ↑, 2 ↓), posterior cingulate cortex (PCC) (nreports = 8/25, 6 ↑, 2 ↓), thalamus (nreports = 2/25, 2 ↑), and cerebellum (nreports = 1/25, 1 ↑). A summary of these results is displayed in Fig. 3.
Fig. 3
Overview of the percentage of articles that found a significant (p < 0.05) regional cerebral perfusion change after long-term acetylcholinesterase-inhibitor treatment in patients with Alzheimer’s disease. ACC, anterior cingulate cortex; PCC, posterior cingulate cortex.
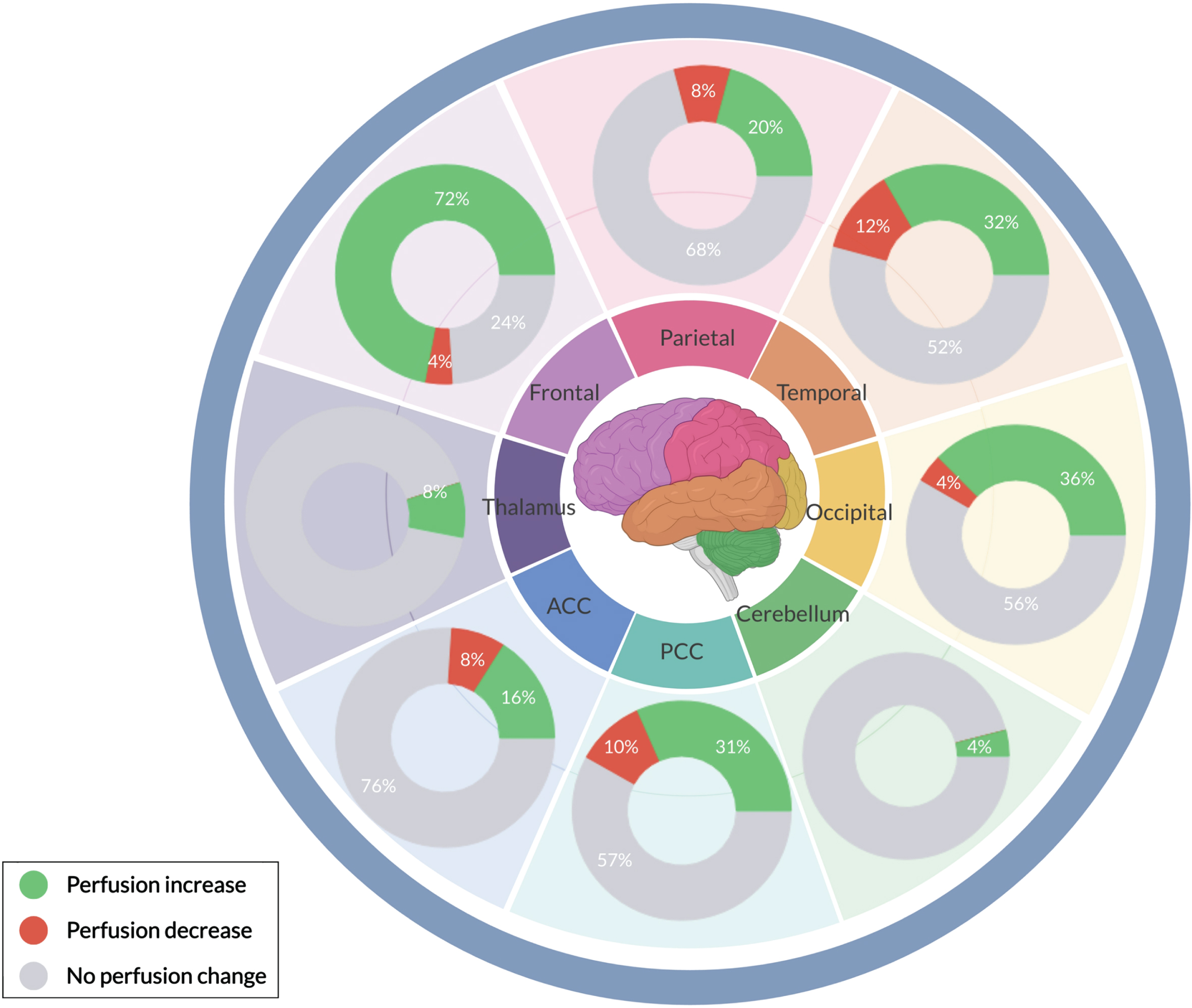
Frontal cortex
18 (72% of articles discussing chronic AChEI intake (Achronic)) [19–25, 27–29, 32–36, 38, 40, 41] reported an rCBF increase, more specifically, in the inferior frontal, orbital, subcallosal and rectal gyri, and the paracentral lobule. Only one study (4%) [31] reported a decrease in the frontal cortex rCBF after AChEI treatment. Six studies (24%) observed no perfusion changes [18, 26, 30, 37, 39, 42].
Parietal cortex
Five (20% of Achronic) [24, 26, 29, 39, 40] reported an increase and two (8%) a decrease [22, 41] in the parietal cortex, while 18 did not observe any changes in cerebral perfusion (68%) [18–21, 23, 25, 27, 28, 30–38, 42].
Temporal cortex
One third of the studies (32% of Achronic) demonstrated that long-term AChEI treatment increases the temporal lobe perfusion [20, 24–26, 29, 31, 38, 40, 41], while half of the studies reported no perfusion changes (52%) [18, 19, 22, 23, 27, 28, 30, 32–37]. Only three studies (12%) reported a perfusion decrease in the temporal cortex [21, 35, 37].
Occipital lobe
The majority of the studies did not observe any changes in the occipital perfusion (56% of Achronic) [18, 19, 21–25, 27, 28, 33–35, 38, 40]. However, nine studies (36%) reported an increase [20, 21, 29, 31, 36, 37, 39–41] and one (4%) reported a decrease in the occipital perfusion [42].
Regional perfusion
rCBF increases in the ACC and PCC were observed in respectively four (16% of Achronic) [21–23, 34] and six studies (31%) [19, 21–23, 28, 32]. On the contrary, perfusion decreases were reported in both the ACC and PCC in two reports (10%) [21, 41]. No changes in the ACC and PCC were reported in respectively 15 (76%) [18–20, 24, 25, 27–29, 31–33, 35, 36, 38, 40] and 11 (57%) studies [20, 24, 25, 27, 29, 31, 33, 35, 36, 38, 40]. Finally, in two studies (8%) a perfusion increase was observed in the thalamus [17, 21] and in one (4%) in the cerebellum [31].
In contrast, in patients without AChEI treatment, a deterioration of the rCBF in the frontal, parietal and occipital lobes, as well as in the cingulate gyrus was observed, representing the natural course of the disease [33, 35]. No perfusion changes were observed in healthy individuals after chronic AChEI intake [19, 23].
Treatment responsiveness
Six studies classified the included Alzheimer’s disease patients into responders and non-responders [26, 30, 37, 39, 42, 43] based on the Mini-Mental State Examination (MMSE) score evolution. Within the studies, cognitive treatment response was defined as an increase of ≥1 in MMSE scores after at least six months of therapy.
During baseline testing, no significant differences in MMSE scores nor perfusion between both subgroups were reported [35, 37]. After at least six months of AChEI treatment, the mean increase in MSSE score in responders was 2.05±0.45, and a significant (p < 0.05) perfusion increase in the frontal lobe [26, 35, 37, 39, 44], the parietal lobe [26, 35, 37, 42, 43], the temporal lobe [26, 35, 37, 42, 43] and in the cingulate gyrus [30, 37] were observed (Table 2). In non-responders, however, the MMSE score decreased by 3.85±0.7 points and a widespread perfusion decrease was noted.
Table 2
Summary of significant (p < 0.05) cerebral perfusion changes in cognitive (non-)responders after acetylcholinesterase-inhibitor treatment in patients with Alzheimer’s disease. Cognitive treatment response was defined as an increase of≥1 in MMSE scores after at least six months of therapy. A significant perfusion increase was observed in most of the responders, whereas an overall significant perfusion decrease was reported in clinical non-responders, illustrating a potential relation between perfusion (change) and cognition (change). *p < 0.01. n°, number of participants. Only qualitative perfusion data could be obtained
Response | n° | Author | Brain Region | |||||
Frontal | Parietal | Temporal | Occipital | ACC | PCC | |||
Responder | 36 | Iizuka et al. [30] | ⇑ | |||||
25 | Nobili et al. [35] | |||||||
55 | Shimizu et al. [21] | ⇑ | ||||||
23 | Tepmongkol et al. [39] | ⇑ | ⇑ | ⇑ | ||||
18 | Venneri et al. [26] | ⇑ | ⇑ | ⇑ | ||||
29 | Yoshida et al. [42] | ⇑ | ⇑ | |||||
Non-Responder | 36 | Iizuka et al. [30] | ||||||
25 | Nobili et al. [35] | ⇓ | ⇓ | ⇓ | ⇓ | |||
55 | Shimizu et al. [21] | ⇑ | ⇓ | ⇓ | ⇓ | |||
23 | Tepmongkol et al. [39] | ⇓ | ||||||
18 | Venneri et al. [26] | ⇓ | ⇓ | ⇓ | ⇓ | |||
29 | Yoshida et al. [42] | ⇓ | ⇓ | ⇓ |
⇑=Significant perfusion increase compared to baseline (p < 0.05). ⇓=Significant perfusion decrease compared to baseline (p < 0.05) White box, No significant perfusion change compared to baseline.
Treatment duration
The influence of treatment duration on cerebral perfusion changes was evaluated in patients with AD at baseline, after short-term (3–6 months) and long-term treatment (12–18 months) in three studies (Table 3) [18, 30, 40, 42]). At baseline, the patients showed a global perfusion decrease compared to healthy individuals [18]. After 3–6 months of treatment, either no perfusion changes were observed [18, 30] or either an increase in cerebral perfusion was reported, mainly in the frontal [40], temporal [40], and parietal lobe [40], as well as the PCC [30]. After 12–18 months of treatment, two out of three studies found a decrease in cerebral perfusion in the temporal lobe [18] and PCC [30]. The pattern of hypoperfusion was even more remarkable than at baseline [30, 40, 42]. However, in one study, perfusion after treatment still appeared to be slightly increased compared to baseline [40]. These results were in line with the MMSE scores, ameliorating during the first three to six months, after which cognitive decline resumed [40].
Table 3
Summary of the significant (p < 0.05) cerebral perfusion changes after short-term (0–12 months) and long-term (≥12 months) acetylcholinesterase-inhibitor treatment in patients with Alzheimer’s disease, dementia with Lewy bodies, and Parkinson’s disease dementia. ST, short-term; LT, long-term; Du, duration; ACC, anterior cingulate cortex; PCC, posterior cingulate cortex; D, donepezil; G, galantamine; n°, number of participants. Only qualitative perfusion data could be obtained
ST versus LT | Author | Drug | Du | n° | Brain Region | |||||
Frontal | Parietal | Temporal | Occipital | ACC | PCC | |||||
Short-term | Ushuijima et al. [40] | D | 3 | 17 | ⇑ | ⇑ | ⇑ | |||
Iizuka et al. [30] | D | 6 | 36 | ⇑ | ||||||
Araki et al. [18] | D | 11 | 11 | ⇓ | ||||||
G | 11 | 13 | ||||||||
Long-term | Ushuijima et al. [40] | D | 12 | 17 | ⇑ | ⇑ | ⇑ | |||
Iizuka et al. [30] | D | 18 | 39 | ⇓ | ||||||
Araki et al. [18] | D | 13 | 11 | |||||||
G | 13 | 13 | ⇓ |
⇑=Significant perfusion increase compared to baseline (p < 0.05) ⇓=Significant perfusion decrease compared to baseline (p < 0.05) White box, No significant perfusion change compared to baseline.
Dosage
Only one study [45] specifically examined the effects of the dosage of donepezil. Initially, the (mild) AD patients were treated with 5 mg donepezil/day. After showing disease progression, the dosage was increased to 10 mg/day. This dosage increase appeared to result in a perfusion increase in the left and right frontal lobe, and a small portion of the right parietal lobe. On the other hand, significant decreases in perfusion were observed in the left frontal lobe, a minimal portion of the right frontal lobe, and a portion of the right parietal lobe [45].
Different types of AChEIs
Only two studies compared the impact of different AChEIs on cerebral perfusion [21, 46]. One study enrolled eighteen patients with Parkinson’s disease dementia, of which eleven treated with rivastigmine (6 mg/d, twice daily), and seven with donepezil (10 mg/d, daily). No significant differences in the regional perfusion pattern were observed after six months of treatment between the two groups [46]. Additionally, a 3-arm 12-month clinical trial, including donepezil, rivastigmine, and galantamine, was carried out in 55 patients with AD. Similarly, no significant perfusion differences were found between the groups [21].
DISCUSSION
This systematic review suggests an increase in cerebral perfusion after both short- and long-term intake of AChEIs, in the frontal, parietal, temporal, and occipital lobes, as well as the cingulate gyrus in patients with Alzheimer’s disease, dementia with Lewy bodies, and Parkinson’s disease dementia, but not in healthy individuals. These effects appear to be dose-related, related to clinical treatment response, and consistent across patient populations and types of AChEIs.
The exact mechanisms underlying these rCBF changes are not yet fully understood. However, increasing evidence indicates that the inhibition of acetylcholine breakdown partially correct the cholinergic deficit and contributes to localized arterial dilatation, thus increasing perfusion. This is referred to as the cholinergic-vascular hypothesis [47, 48]. Therefore, AChEIs not only boost cholinergic neurotransmission and compensate for the loss of functioning brain cells as illustrated in Fig. 4, but also appear to alter the vascular tone [16]. However, for the included studies, it is difficult to disentangle the direct effects of AChEIs on CBF from the indirect effects that might be caused by AChEIs-induced changes in neuronal activity in AD patients. We refer to the articles of Claassen et al. [47] and Van Beek et al. [48] for a more detailed overview of the role of cholinergic innervation in AD.
Fig. 4
Mechanism of action of acetylcholinesterase inhibitors. A cholinergic neuron and the three targets of AChEIs: acetylcholinesterase, butyrylcholinesterase (BuChE), and nicotinic cholinergic receptors. Acetylcholinesterase breaks down acetylcholine (ACh) presynaptically, postsynaptically, as well as extracellularly. All four AChEIs block this enzyme, and this causes ACh levels to increase. BuChE, which is found in glial cells that are located near neurons, is a second enzyme that breaks down ACh. Both rivastigmine and physostigmine inhibit this enzyme. Galantamine also allosterically modulates pre- and postsynaptic nicotinic receptors, which play a key role in focusing attention and memory.
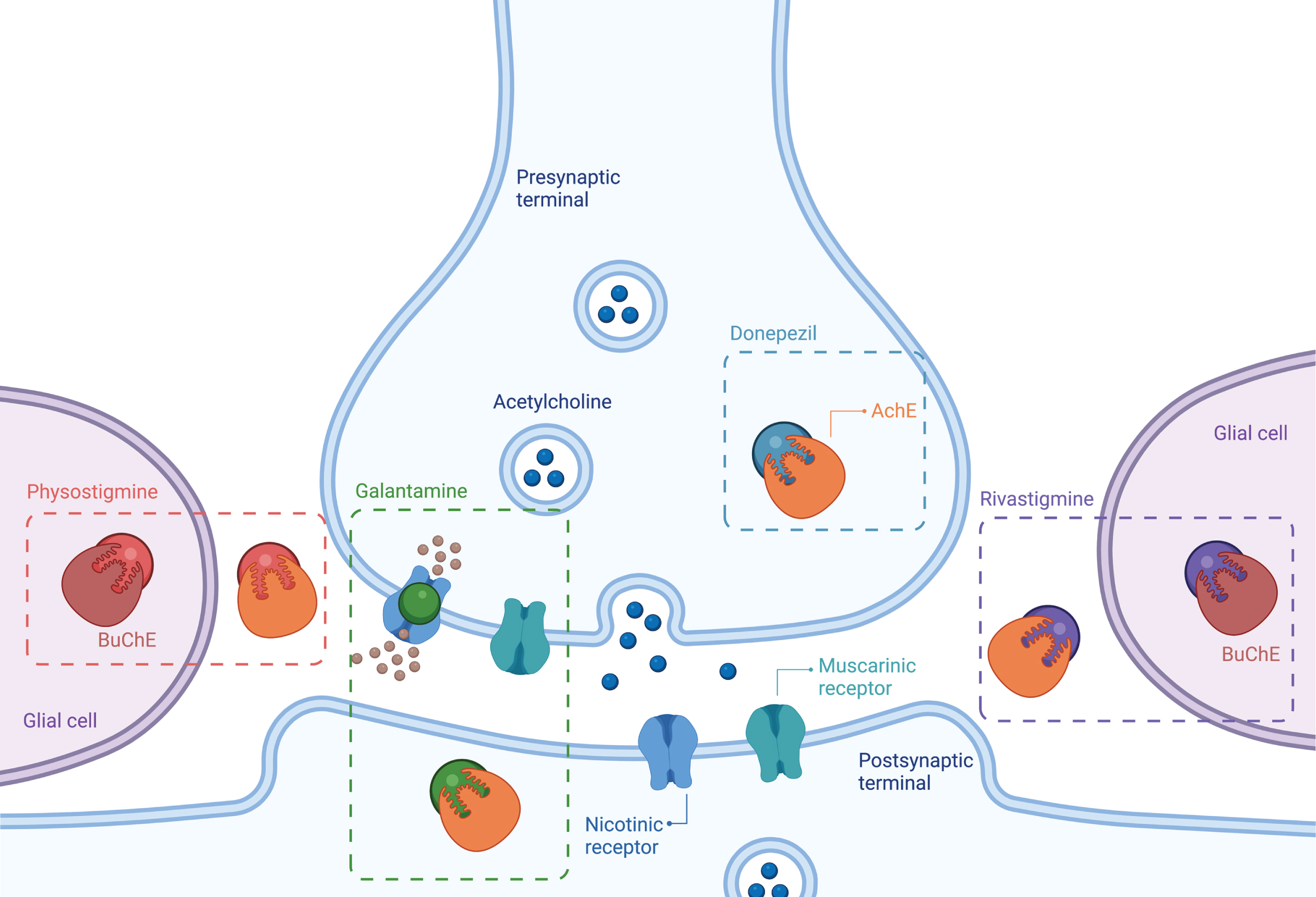
Both the effects of acute and chronic AChEI intake have been studied. However, as the clinical relevance of acute intake is limited to the treatment of glaucoma and anticholinergic toxicity, the majority of investigations focuses on chronic AChEI intake. 67.6% percent of the studies investigating the long-term effect of AChEIs reported a significant increase after treatment in the frontal lobes. To a lesser extent, similar increases were observed in the temporal (41.2%), parietal (23.5%), and occipital (30%) lobe and in the (20%) cingulate gyrus (ACC: 14%; PCC: 28%). In contrast, a widespread perfusion decrease was observed in non-treated dementia patients, representing the natural course of the disease. Cholinergic neurons are widely distributed throughout the brain, explaining the global character of these effects [49]). Moreover, the preferential frontal response could be related to the denser representation of cholinergic projections from the brainstem to this region, or to the fact that the frontal cortex is relatively spared in the early stages of AD [21].
Some studies also indicate that patients who clinically respond to AChEIs show either an improvement or stabilization of perfusion in the above-mentioned regions, whereas non-responders show a progressive decline. These observations may indicate a relation between rCBF and clinical treatment response [50]. Moreover, several studies suggest a role for perfusion measurements in the early evaluation of treatment responsiveness [30, 37, 42]. However, large-scale post hoc analyses of rCBF as a predictor for treatment response is lacking [51]. Treatment responsiveness is usually limited to the evaluation of cognitive functioning, while other important outcome parameters, such as daily functioning, were not considered [50].
Some studies suggest that the perfusion increase after AChEI treatment is only temporary [18, 30, 40]. More specifically, an increase in perfusion and cognitive functioning was reported after six months of treatment, which disappeared again after eighteen months. These temporary effects explain the modest clinical efficacy of AChEIs against cognitive decline and behavioral symptoms of dementia, only for a limited period of time. This is not surprising as AChEIs are incapable of arresting neuronal degeneration, but temporarily delay clinical progression by improving perfusion and cholinergic signaling. The observed decrease in cognitive functioning and perfusion after long-term treatment might reflect the irreversibility of the nerve degeneration [40].
Additionally, AChEI dosage appears to impact the effects on perfusion. Lower doses of donepezil (5 mg/d) tend to be less beneficial in patients with moderate to severe AD. However, these patients, who are presumed to have a greater loss of cholinergic function, can still achieve therapeutic benefits from higher doses (> 10 mg/d) of AChEIs. Therefore, higher doses could be considered when lower doses show no clinical improvement [52].
This systematic review has been performed rigorously following a predefined protocol; however, several limitations impede drawing conclusions. The main limitation arises from the methodological heterogeneity of the included studies. More specifically, the variety of the included patient population characteristics, poly drug use, the interventional dosage, duration of follow-up, imaging modalities, and outcome measures, complicate comparison across studies. In addition, current evidence is limited to methodological studies with a high risk of bias, while randomized controlled trials are lacking. Furthermore, the extracted study results are generally limited to qualitative perfusion information in bigger brain regions, after a relatively short observation period in specific populations. Moreover, there has never been a comparison with a control placebo group. Therefore, we cannot assess how much of the outcomes may be due to placebo effect. No firm conclusions can be drawn about the effects of AChEIs in populations without a perfusion deficit, since only two studies investigated the effects of AChEIs in healthy individuals. All remaining perfusion information of healthy individuals was used as a control group to interpret perfusion changes in the patient populations. The lack of quantitative perfusion information conceals the magnitude of these effects on perfusion, and hampers conducting a meta-analysis. Also, lobular perfusion was investigated in most available studies, while information on perfusion changes in smaller cerebral regions is lacking. Additionally, there is no evidence of the long-term perfusion effects of AChEIs, as the majority of the studies were limited to 12 months of observation. Finally, the study population consisted of AD patients, treated with donepezil, rendering the conclusions not generalizable to other patient populations. Although it is difficult to overcome all these limitations, there is a need to set up well-designed studies focusing on local, quantitative perfusion measurements in different study populations, using different AChEI types and dosages.
Despite these limitations, current evidence indicates that the intake of AChEIs influences cerebral perfusion, thus confounding perfusion measurements. Therefore, AChEIs should be considered as a perfusion modifier, and be taken into account when interpreting or analyzing perfusion images [9]. However, additional research is required to assess the true impact of AChEIs, compared to important perfusion modifiers such as exercise, age, and sleep, mainly in terms of effect size [9].
Conclusion
This systematic review provides an overview of the reported effects of AChEIs on cerebral perfusion. Overall, a perfusion increase was observed following chronic AChEI treatment in treatment-responsive patients with Alzheimer’s disease, dementia with Lewy bodies, and Parkinson’s disease dementia in the frontal, parietal, temporal, and occipital lobes, as well as in the cingulate gyrus. On the contrary, further perfusion decline was reported in patients not receiving AChEIs or not responding to the treatment. These effects appear to be temporary, dose-related, and consistent across populations and different AChEI types. More research focused on quantitative perfusion information in both patients and healthy individuals is required to draw conclusions about whether AChEI intake should be considered when analyzing perfusion data.
ACKNOWLEDGMENTS
The authors wish to express their gratitude to Dr. Nele Pauwels from the Knowledge Centre for Health Ghent (KCGG), Ghent University and Ghent University Hospital, for her advisory role in the development of the systematic review protocol and search strategy.
FUNDING
This research did not receive any specific grant from funding agencies in the public, commercial, or not-for-profit sectors.
CONFLICT OF INTEREST
The authors have no conflict of interest to report.
DATA AVAILABILITY
The data supporting the findings of this study are available within the article and its supplementary material.
SUPPLEMENTARY MATERIAL
[1] The supplementary material is available in the electronic version of this article: https://dx.doi.org/10.3233/JAD-221125.
REFERENCES
[1] | Fox C , Lafortune L , Boustani M , Brayne C ((2013) ) The pros and cons of early diagnosis in dementia. Br J Gen Pract 63: , e510–e512. |
[2] | Johnson NA , Jahng G-H , Weiner MW , Miller BL , Chui HC , Jagust WJ , Gorno-Tempini ML , Schuff N ((2005) ) Pattern of cerebral hypoperfusion in Alzheimer disease and mild cognitive impairment measured with arterial spin-labeling MR imaging: Initial experience. Radiology 234: , 851–859. |
[3] | Bradford A , Kunik ME , Schulz P , Williams SP , Singh H ((2009) ) Missed and delayed diagnosis of dementia in primary care: Prevalence and contributing factors. Alzheimer Dis Assoc Disord 23: , 306–314. |
[4] | de Miranda LFJR , Matoso de R O , Rodrigues MV , de Lima TOL , Nascimento AF , Carvalho FC , Moreira de DR M , Fernandes JC , de Paula JJ , Magno LAV , Caramelli P , de Moraes EN ((2011) ) Factors influencing possible delay in the diagnosis of Alzheimer’s disease: Findings from a tertiary Public University Hospital. Dement Neuropsychol 5: , 328–331. |
[5] | Helvik A-S , Engedal K , Saltytė Benth J , Selbæk G ((2018) ) Time from symptom debut to dementiaassessment by the specialist healthcare service in Norway. Dement Geriatr Cogn Dis Extra 8: , 117–127. |
[6] | Hays CC , Zlatar ZZ , Wierenga CE ((2016) ) The utility of cerebral blood flow as a biomarker of preclinical Alzheimer’s disease. Cell Mol Neurobiol 36: , 167–179. |
[7] | Horgan D , Nobili F , Teunissen C , Grimmer T , Mitrecic D , Ris L , Pirtosek Z , Bernini C , Federico A , Blackburn D , Logroscino G , Scarmeas N ((2020) ) Biomarker testing: Piercing the fog of Alzheimer’s and related dementia. Biomedicine Hub 5: , 1–22. |
[8] | Isaacs JD , Boenink M ((2020) ) Biomarkers for dementia: Too soon for routine clinical use. Lancet Neurol 19: , 884–885. |
[9] | Clement P , Mutsaerts H-J , Václavu L , Ghariq E , Pizzini FB , Smits M , Acou M , Jovicich J , Vanninen R , Kononen M , Wiest R , Rostrup E , Bastos-Leite AJ , Larsson E-M , Achten E ((2018) ) Variability of physiological brainperfusion in healthy subjects - A systematic review of modifiers. Considerations for multi-center ASL studies. J Cereb Blood Flow Metab 38: , 1418–1437. |
[10] | Versijpt J ((2014) ) Effectiveness and cost-effectiveness of the pharmacological treatment of Alzheimer’s disease and vascular dementia. J Alzheimers Dis 42: (Suppl 3), S19–25. |
[11] | Moher D , Shamseer L , Clarke M , Ghersi D , Liberati A , Petticrew M , Shekelle P , Stewart LA ((2015) ) Preferred Reporting Items for Systematic Review and Meta-Analysis Protocols (PRISMA-P) 2015 statement. Syst Rev 4: , 1. |
[12] | Page MJ , McKenzie JE , Bossuyt PM , Boutron I , Hoffmann TC , Mulrow CD , Shamseer L , Tetzlaff JM , Akl EA , Brennan SE , Chou R , Glanville J , Grimshaw JM , Hróbjartsson A , Lalu MM , Li T , Loder EW , Mayo-Wilson E , McDonald S , McGuinness LA , Stewart LA , Thomas J , Tricco AC , Welch VA , Whiting P , Moher D ((2021) ) The PRISMA 2020 statement: An updated guideline for reporting systematic reviews. BMJ 372: , n71. |
[13] | Sterne JA , Hernán MA , Reeves BC , Savović J , Berkman ND , Viswanathan M , Henry D , Altman DG , Ansari MT , Boutron I , Carpenter JR , Chan A-W , Churchill R , Deeks JJ , Hróbjartsson A , Kirkham J , Jüni P , Loke YK , Pigott TD , Ramsay CR , Regidor D , Rothstein HR , Sandhu L , Santaguida PL , Schünemann HJ , Shea B , Shrier I , Tugwell P , Turner L , Valentine JC , Waddington H , Waters E , Wells GA , Whiting PF , Higgins JP ((2016) ) ROBINS-I: Atool for assessing risk of bias in non-randomised studies of interventions. BMJ 355: , i4919. |
[14] | Higgins JPT , Savović J , Page MJ , Elbers RG , Sterne JAC (2019) Assessing risk of bias in a randomized trial. Cochrane Handbook for Systematic Reviews of Interventions, pp. 205-228. |
[15] | Gustafson L ((1993) ) Physostigmine and tetrahydroaminoacridine treatment of Alzheimer’s disease. Acta Neurol Scand Suppl 149: , 39–41. |
[16] | Geaney DP , Soper N , Shepstone BJ , Cowen PJ ((1990) ) Effect of central cholinergic stimulation on regional cerebral blood flow in Alzheimer disease. Lancet 335: , 1484–1487. |
[17] | Blin J , Ivanoiu A , Coppens A , De Volder A , Labar D , Michel C , Laterre EC ((1997) ) Cholinergic neurotransmission has different effects on cerebral glucose consumption and blood flow in young normals, aged normals, and Alzheimer’s disease patients. Neuroimage 6: , 335–343. |
[18] | Araki Y , Huruichi M , Nokura H ((2017) ) [p1-401]: Association between adherence of Alzheimer’s disease medication and 3d-ssp decrease images. Alzheimers Dement 13: , P427. |
[19] | Chaudhary S , Scouten A , Schwindt G , Janik R , Lee W , Sled JG , Black SE , Stefanovic B ((2013) ) Hemodynamic effects of cholinesterase inhibition in mild Alzheimer’s disease. J Magn Reson Imaging 38: , 26–35. |
[20] | Cho H , Kwon J-H , Seo H-J , Kim J-S ((2010) ) The short-term effect of acetylcholinesterase inhibitor on the regional cerebral blood flow of Alzheimer’s disease. Arch Gerontol Geriatr 50: , 222–226. |
[21] | Shimizu S , Kanetaka H , Hirose D , Sakurai H , Hanyu H ((2015) ) Differential effects of acetylcholinesterase inhibitors on clinical responses and cerebral blood flow changes in patients with Alzheimer’s disease: A 12-month, randomized, and open-label trial. Dement Geriatr Cogn Dis Extra 5: , 135–146. |
[22] | Shirayama Y , Takahashi M , Oda Y , Yoshino K , Sato K , Okubo T , Iyo M ((2019) ) rCBF and cognitive impairment changes assessed by SPECT and ADAS-cog in late-onset Alzheimer’s disease after 18 months of treatment with the cholinesterase inhibitors donepezil or galantamine. Brain Imaging Behav 13: , 75–86. |
[23] | Litvinenko IV , Odinak MM , Mogil’naya VI , Emelin AY ((2008) ) Efficacy and safety of galantamine (reminyl) for dementia in patients with Parkinson’s disease (an open controlled trial). Neurosci Behav Physiol 38: , 937–945. |
[24] | Ettorre E , Bersani FS , Colella F , Servello A , Minichino A , Megna V , Liberatore M , Biondi M , Marigliano V ((2015) ) Cerebral perfusional effects of 1-year rivastigmine treatment in Alzheimer disease: A case report. Riv Psichiatr 50: , 188–191. |
[25] | Lojkowska W , Ryglewicz D , Jedrzejczak T , Minc S , Jakubowska T , Jarosz H , Bochynska A ((2003) ) The effect of cholinesterase inhibitors on the regional blood flow in patients with Alzheimer’s disease and vascular dementia. J Neurol Sci 216: , 119–126. |
[26] | Venneri A , Shanks MF , Staff RT , Pestell SJ , Forbes KE , Gemmell HG , Murray AD ((2002) ) Cerebral blood flow and cognitive responses to rivastigmine treatment in Alzheimer’s disease. Neuroreport 13: , 83–87. |
[27] | Cerci SS , Tamam Y , Kaya H , Yildiz M , Arslan S ((2007) ) Effect of rivastigmine on regional cerebral blood flow in Alzheimer’s disease. Adv Ther 24: , 611–621. |
[28] | Antuono P , Jones J , Wu Z , McRea T , Franczak M , Ward DB , Li SJ ((2009) ) IC-P-168: Detection of changes in cerebralblood flow perfusion following aricept ® treatment in mild Alzheimer subjects. Alzheimers Dement 5: , P66–P66. |
[29] | Fong TG , Inouye SK , Dai W , Press DZ , Alsop DC ((2011) ) Association cortex hypoperfusion in mild dementia with Lewy bodies: A potential indicator of cholinergic dysfunction? Brain Imaging Behav 5: , 25–35. |
[30] | Iizuka T , Kameyama M ((2017) ) Cholinergic enhancement increases regional cerebral blood flow to the posterior cingulate cortex in mild Alzheimer’s disease. Geriatr Gerontol Int 17: , 951–958. |
[31] | Kanaya K , Abe S , Sakai M , Fujii H , Koizumi K , Iwamoto T ((2012) ) Efficacy of a high dosage of donepezil for Alzheimer’s disease as examined by single-photon emission computed tomography imaging. Psychogeriatrics 12: , 172–178. |
[32] | Li W , Antuono PG , Xie C , Chen G , Jones JL , Ward BD , Franczak MB , Goveas JS , Li S-J ((2012) ) Changes in regional cerebral blood flow and functional connectivity in the cholinergic pathway associated with cognitive performance in subjects with mild Alzheimer’s disease after 12-week donepezil treatment. Neuroimage 60: , 1083–1091. |
[33] | Nakano S , Asada T , Matsuda H , Uno M , Takasaki M ((2001) ) Donepezil hydrochloride preserves regional cerebral blood flow in patients with Alzheimer’s disease. J Nucl Med 42: , 1441–1445. |
[34] | Niwa H , Koumoto C , Shiga T , Takeuchi J , Mishima S , Segawa T , Atsumi T , Shimizu C , Koike T , Yoshioka N ((2006) ) Clinical analysis of cognitive function in diabetic patients by MMSE and SPECT. Diabetes Res Clin Pract 72: , 142–147. |
[35] | Nobili F , Vitali P , Canfora M , Girtler N , De Leo C , Mariani G , Pupi A , Rodriguez G ((2002) ) Effects of long-term Donepezil therapy on rCBF of Alzheimer’s patients. Clin Neurophysiol 113: , 1241–1248. |
[36] | Rodriguez G , Vitali P , De Leo C , De Carli F , Girtler N , Nobili F ((2002) ) Quantitative EEG changes in Alzheimer patients during long-term donepezil therapy. Neuropsychobiology 46: , 49–56. |
[37] | Shimizu S , Hanyu H , Iwamoto T , Koizumi K , Abe K ((2006) ) SPECT follow-up study of cerebral blood flow changes during Donepezil therapy in patients with Alzheimer’s disease. J Neuroimaging 16: , 16–23. |
[38] | Tateno M , Kobayashi S , Utsumi K , Morii H , Fujii K ((2008) ) Quantitative analysis of the effects of donepezil on regional cerebral blood flow in Alzheimer’s disease by using an automated program, 3DSRT. Neuroradiology 50: , 723–727. |
[39] | Tepmongkol S , Hemrungrojn S , Dupont P , Tunvirachaisakul C , Aniwattanapong D , Likitjareon Y , Supasitthumrong T , Tawankanjanachot I , Siritranon N , Chuchuen P , Natsawang B , Tangwongchai S ((2019) ) Early prediction of donepezil cognitive response in Alzheimer’s disease by brain perfusion single photon emissiontomography. Brain Imaging Behav 13: , 1665–1673. |
[40] | Ushuijima Y , Okuyama C , Mori S , Kubota T , Nakai T , Nishimura T ((2006) ) Regional cerebral blood flow in Alzheimer’s disease: Comparison between short and long-term donepezil therapy. Ann Nucl Med 20: , 425–429. |
[41] | Warren S , Hier DB , Pavel D ((1998) ) Visual form of Alzheimer’s disease and its response to anticholinesterase therapy. J Neuroimaging 8: , 249–252. |
[42] | Yoshida T , Ha-Kawa S , Yoshimura M , Nobuhara K , Kinoshita T , Sawada S ((2007) ) Effectiveness of treatment with donepezil hydrochloride and changes in regional cerebral blood flow in patients with Alzheimer’s disease. Ann Nucl Med 21: , 257–265. |
[43] | Nobili F , Copello F , Buffoni F , Vitali P , Girtler N , Bordoni C , Safaie-Semnani E , Mariani G , Rodriguez G ((2001) ) Regional cerebral blood flow and prognostic evaluation in Alzheimer’s disease. Dement Geriatr Cogn Disord 12: , 89–97. |
[44] | Yoshida Y , Sugiyama T , Utsunomiya K , Ogura Y , Ikeda T ((2010) ) A pilot study for the effects of donepezil therapy on cerebral and optic nerve head blood flow, visual field defect in normal-tension glaucoma. J Ocul Pharmacol Ther 26: , 187–192. |
[45] | Kaasinen V , Någren K , Järvenpää T , Roivainen A , Yu M , Oikonen V , Kurki T , Rinne JO ((2002) ) Regional effects ofdonepezil and rivastigmine on cortical acetylcholinesterase activityin Alzheimer’s disease. J Clin Psychopharmacol 22: , 615–620. |
[46] | Ceravolo R , Volterrani D , Frosini D , Bernardini S , Rossi C , Logi C , Manca G , Kiferle L , Mariani G , Murri L , Bonuccelli U ((2006) ) Brain perfusion effects of cholinesterase inhibitors in Parkinson’s disease with dementia. J Neural Transm 113: , 1787–1790. |
[47] | Claassen JAHR , Jansen RWMM ((2006) ) Cholinergically mediated augmentation of cerebral perfusion in Alzheimer’s disease and related cognitive disorders: The cholinergic-vascular hypothesis. J Gerontol A Biol Sci Med Sci 61: , 267–271. |
[48] | Van Beek AHEA , Claassen JAHR ((2011) ) The cerebrovascular role of the cholinergic neural system in Alzheimer’s disease. Behav Brain Res 221: , 537–542. |
[49] | Saykin AJ , Wishart HA , Rabin LA , Flashman LA , McHugh TL , Mamourian AC , Santulli RB ((2004) ) Cholinergic enhancement of frontal lobe activity in mild cognitive impairment. Brain 127: , 1574–1583. |
[50] | Adler G , Brassen S , Chwalek K , Dieter B , Teufel M ((2004) ) Prediction of treatment response to rivastigmine in Alzheimer’s dementia. J Neurol Neurosurg Psychiatry 75: , 292–294. |
[51] | Lemstra AW , Richard E , van Gool WA ((2007) ) Cholinesterase inhibitors in dementia: Yes, no, or maybe? Age Ageing 36: , 625–627. |
[52] | Farlow MR , Salloway S , Tariot PN , Yardley J , Moline ML , Wang Q , Brand-Schieber E , Zou H , Hsu T , Satlin A ((2010) ) Effectiveness and tolerability of high-dose (23 mg/d) versus standard-dose (10 mg/d) donepezil in moderate to severe Alzheimer’s disease: A 24-week, randomized, double-blind study. Clin Ther 32: , 1234–1251. |