Clinical Approach of Low-Dose Whole-Brain Ionizing Radiation Treatment in Alzheimer’s Disease Dementia Patients1
Abstract
Our research team recently published two relevant papers. In one study, we have seen the acute effect of low-dose ionizing irradiation (LDIR) did not reduce the amyloid-β (Aβ) protein concentration in brain tissue, yet significantly improved synaptic degeneration and neuronal loss in the hippocampus and cerebral cortex. Surprisingly, in another study, we could see late effect that the LDIR-treated mice showed significantly improved learning and memory skills compared with those in the sham group. In addition, Aβ concentrations were significantly decreased in brain tissue. Furthermore, the pro-inflammatory cytokine tumor necrosis factor-α was decreased and the anti-inflammatory cytokine transforming growth factor-β was increased in the brain tissue of 5xFAD mice treated with LDIR. Definitive clinical results for the safety and efficacy of LDIR have not yet been published and, despite the promising outcomes reported during preclinical studies, LDIR can only be applied to patients with Alzheimer’s disease dementia when clinical results are made available. In addition, in the case of LDIR, additional large-scale clinical studies are necessary to determine the severity of Alzheimer’s disease dementia, indications for LDIR, the total dose to be irradiated, fraction size, and intervals of LDIR treatment. The purpose of this review is to summarize the mechanism of LDIR based on existing preclinical results in a way that is useful for conducting subsequent clinical research.
INTRODUCTON
For a century, ionizing radiation has played an important role in treating not only solid cancers but also hematologic cancers. Recently, the possibility of deploying radiation treatment for benign diseases has been suggested and the range of radiation therapy indications is expected to be expanded [1]. Due to the growing aging population, the number of patients with degenerative brain diseases has significantly increased; however, a cure remains unavailable [2]. Recently, the results of preclinical studies have shown that low-dose irradiation (LDIR), a nonpharmacological treatment method, may be a new treatment option for patients with Alzheimer’s disease dementia (AD) [3, 4].
Generally, high-dose radiation causes inflamma-tion and cancer in the body. In particular, palliative whole-brain radiation therapy in patients with meta-static brain tumors reportedly results in diminished memory and cognitive function [5]. Consequently, the application of brain radiation therapy in patients with AD was not considered as a viable treatment option. However, if the radiation dose administered to the hippocampus during whole-brain radiotherapy for patients with brain metastasis were to be reduced below a certain level (median dose <9 Gy), the decline in the patient’s cognitive function and memory would be less significant [6]. In 2016, a decrease in the amyloid-β (Aβ) protein concentration after hemibrain irradiation was reported at Beaumont Hospital [7]. Further, brain radiation therapy was shown to reduce tau-associated neurofibrillary tangles as well as Aβ in 3xTg-AD mice [8].
Based on these preclinical results, our hospital research team is conducting a phase II study (NCT04203121) to examine the efficacy of low-dose brain irradiation in patients with early or mid-stage AD, which is also being investigated by other insti-tutions including the Virginia Commonwealth Uni-versity (NCT02769000), University of Geneva (NCT03352258), and Beaumont Hospital (NCT02359864).
The first two reports about the mode of action for the neuroprotective effects of LDIR in our research laboratory have been made available to date [9, 10] and suggest this method could be a nonpharmacological treatment option for AD patients. In addition, analyzing changes over time after LDIR would help to establish a protocol for confirming treatment re-sponse in future clinical studies.
DETERMINING WHETHER LDIR DIRECTLY OR INDIRECTLY REDUCES THE Aβ PROTEIN CONCENTRATION IN AD
The classical effect of high-dose low linear energy transfer X-ray irradiation is the formation of hydroxyl radicals through radiolysis of water. The hydroxyl radical is primarily responsible for causing double strand breaks in DNA leading to cell death. One study has reported no effect of high dose radiation on Aβ1–42 fibrils or the formation of these fibrils in vitro [11].
In the first experiment, LDIR [9 Gy/5 fractions (fx); biologically effective dose; BED2 = 17.1 Gy2 ] was administered to whole brains of 4-month-old mice, who were euthanized after four days to examine the tissue; at this point, a reduction in the Aβ protein concentration was not observed [9]. The absence of effect of LDIR four days after treatment suggests on the contrary that the radiations do not directly impact amyloid but that it is an indirect effect which may take time to be effective. In addition, synaptic degeneration, neuronal loss, and neuroinflammation were significantly suppressed in the hippocampus and cerebral cortex compared with the group not treated with LDIR. To determine the cytoprotective effects against Aβ toxic substances, SH-SY5Y neu-ronal cells were treated with Aβ1–42 (2μM), followed by LDIR (1 Gy). Based on the MTT assay and DNA-fragmentation test results, LDIR successfully protected nerve cells. Moreover, the production of proinflammatory molecules and the activation of the nuclear factor-kappa B (NF-κB) pathway was suppressed in BV-2 microglial cells after LDIR (Fig. 1).
Fig. 1
Schematic diagram of intra cranial change according to timing after LDIR.
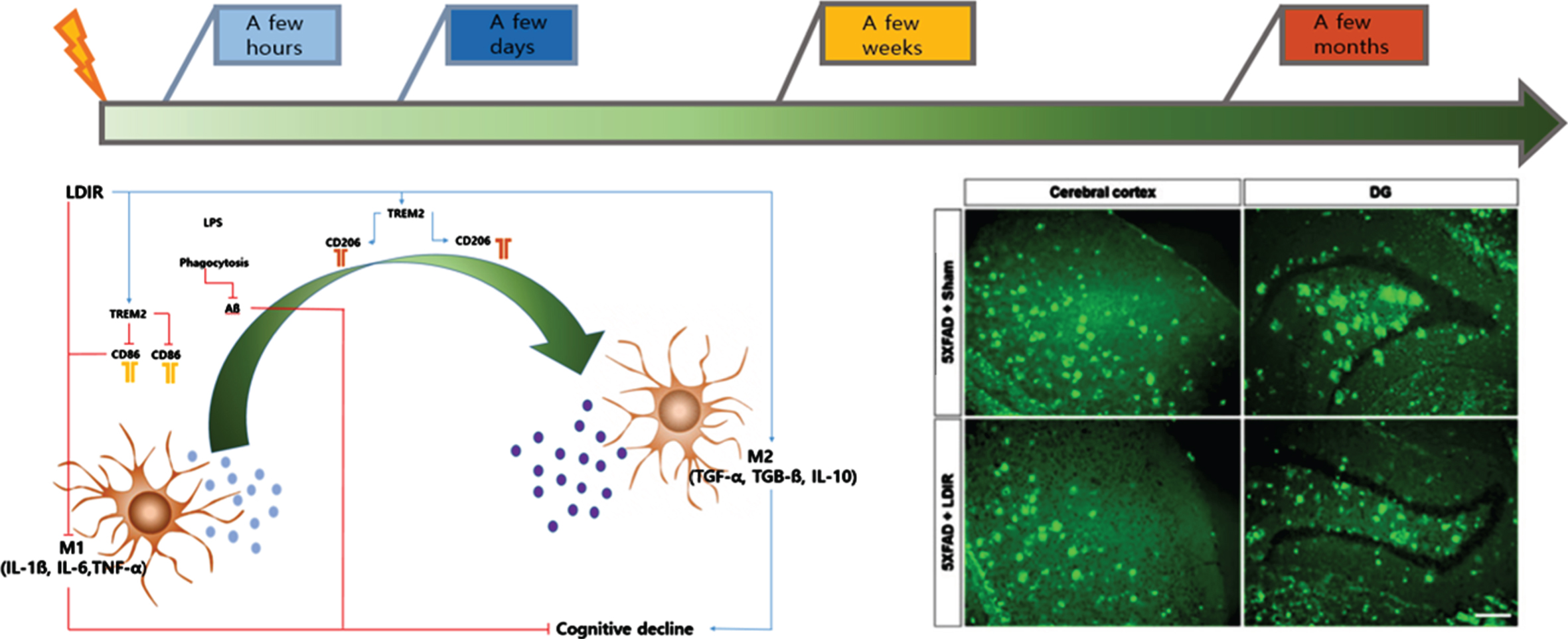
In our second paper, whole-brain LDIR was administered to 6-month-old 5xFAD mice [10]. After eight weeks, cognitive tests were performed, while the brain tissues were examined at 10 weeks after the mice were sacrificed (Fig. 2).
Fig. 2
Effect of LDIR on Aβ deposition in the subiculum and cerebral cortex of 5XFAD mice. A) Schematic diagram of the experimental procedure. 5XFAD mice were exposed to sham or LMDIR (9 Gy in five fractions), and then sacrificed 4 days after the final irradiation. B) Histochemical staining for thioflavin S was performed to identify amyloid plaques in the cerebral cortex and hippocampal dentate gyrus (DG) of the sham- and LDIR-treated 5XFAD mice (scale bar = 250 m). C) Aβ-positive areas in the cerebral cortex and dentate gyrus of the sham- and LDIR-treated 5XFAD mice were quantified and plotted as a percentage. D) Representative of swimming paths was recorded on videotape during the test session of the Morris water maze. E) Latency to escape time of the mice was measured. Repeated-measures two-way ANOVA with Fisher’s post hoc test compared to 5XFAD+Sham mice. F) Measurement of quadrant time to spend in the hidden platform. Data are presented as mean SD (n = 10 mice in sham-treated 5XFAD mice and n = 14 mice in LDIR-treated 5XFAD mice).
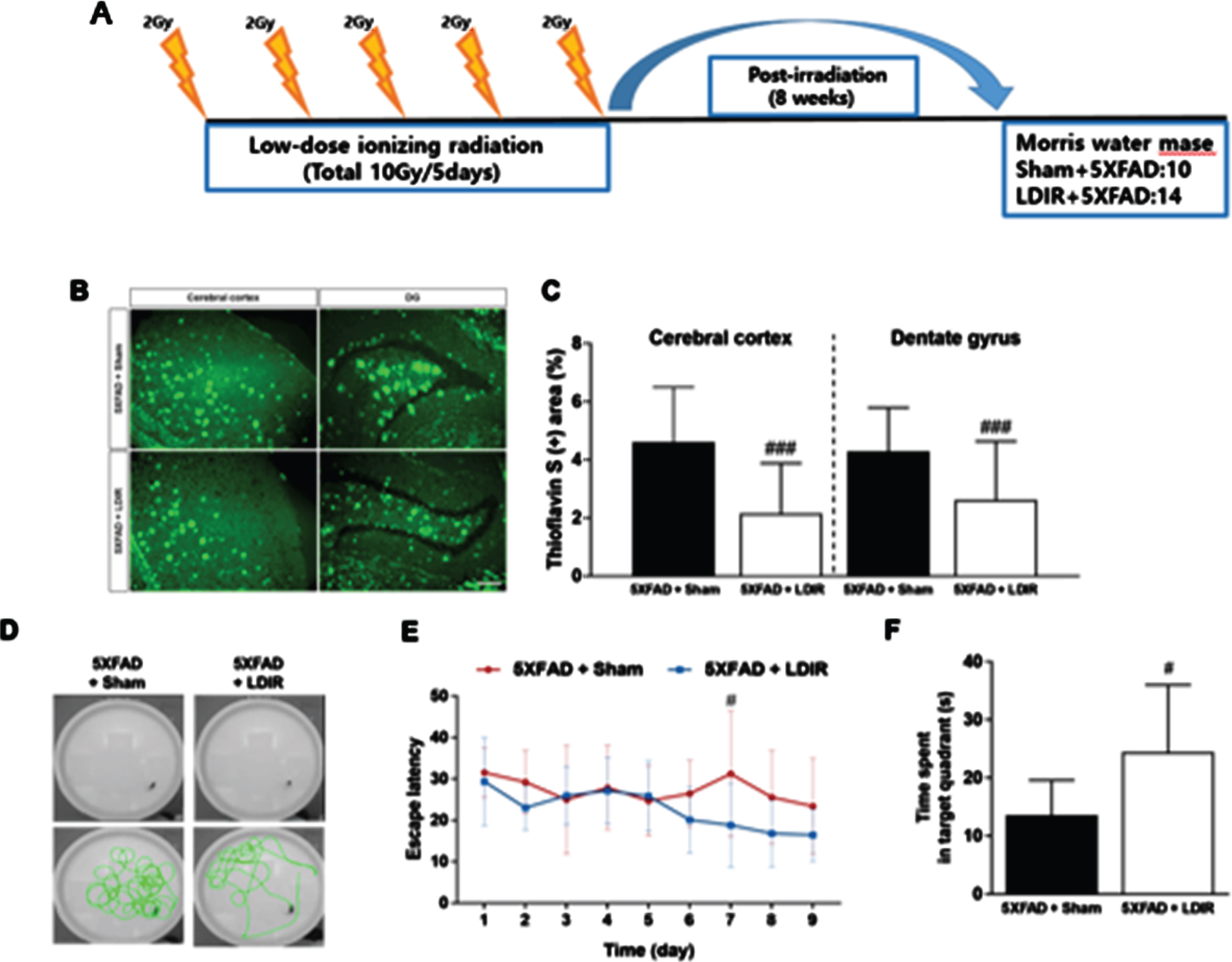
In cognitive tests, the LDIR-treated mice showed significantly improved learning and memory skills compared with the sham group. In addition, Aβ was significantly decreased in brain tissue. Furthermore, the proinflammatory cytokine tumor necrosis factor-α (TNF-α) was decreased and the anti-inflammatory cytokine transforming growth factor-β (TGF-β) was increased in the brain tissue of 5xFAD mice treated with LDIR. After LPS-stimulated BV-2 microglial cells were treated with LDIR (1 Gy), the phenotype of these cells converted to the M2 phenotype by M1. Moreover, the proinflammatory cytokine markers [TNF-α, interleukin (IL)-1, and IL-6] were decreased and anti-inflammatory markers such as M2 cytokine markers (TGF-β, TGF-α, and IL-10) were increased. Furthermore, LDIR significantly increased TREM2 expression in the membranes of BV-2 microglial cells (Fig. 1).
In summary, the therapeutic effect is concerned by the indirect impact of immune cell phenotype regulation along with inhibition of the NF-κB pathway and anti-inflammatory cytokines rather than the direct effect of LDIR reducing Aβ protein levels and tau-associated neurofibrillary tangles [12].
LDIR REGULATES THE PHENOTYPE OF MICROGLIAL CELLS
Microglial cells play an important role in the phagocytosis of neuronal debris and synapse pruning to maintain homeostasis in the brain microenvironment [13, 14]. In a recent report, the regulation of microglial cells was suggested as a potential treatment method for AD [15]. Based on results obtained by our research team, immediately after LDIR, microglial cells in the Tg mouse brain showed red-uced microgliosis and neuronal loss, indicating a neuroprotective effect of LDIR. When the M2 type was replaced, Aβ plaque levels were decreased due to an increase in Aβ phagocytosis, while synaptic pruning and synaptic plasticity activation may eventually lead to increased cognitive function [10].
Meanwhile, the long-term effects of LDIR have been reported in another study; specifically, the number of microglial cells was increased at four weeks after irradiation with 10 Gy/5 fx and further increased after eight weeks [3].
In this experiment, neurogenesis was not observed in the brain tissue immediately after LDIR in a 4-month-old dementia mice model, while neurogenesis remained unconfirmed even after nine weeks. However, the possibility of neurogenesis in 6-month-old dementia mice could not be excluded.
Summarizing the results of the above mentioned experiments, rather than decreasing Aβ directly with LDIR, inhibition for the production of pro-inflam-matory molecules and the activation of the NF-κB pathway were associated with reduction of Aβ toxicity immediately after LDIR. These results indicate the neuroprotective effects are due to the suppression of Aβ and, by controlling the phagocytosis of abnormal proteins in brain tissue, synapse formation, and phenotype of microglia involved in synaptic pruning, an improvement in cognitive function and a decrease in the Aβ protein concentration after at least eight weeks were observed.
IDENTIFYING THE BEST STAGE OF AD IN WHICH TO PURSUE LOW-DOSE RADIATION THERAPY
The research on LDIR has shown the triggering of indirect effects is the main mechanism of action, such as the inhibition of proinflammatory cytokines, increased anti-inflammatory cytokine release, and microglia phenotype regulation caused by the inhibition of the NF-kβ pathway, rather than the direct degradation of Aβ proteins. Therefore, the stage of AD in which neurodegeneration has progressed only minimally may be considered the best time to perform LDIR [16] (Fig. 3).
Fig. 3
Schematic representation of changes in cognitive, metabolic, structural and molecular pathogenetic parameters in relation to estimated years to symptomatic onset of dominantly inherited AD [17].
![Schematic representation of changes in cognitive, metabolic, structural and molecular pathogenetic parameters in relation to estimated years to symptomatic onset of dominantly inherited AD [17].](https://ip.ios.semcs.net:443/media/jad/2021/80-3/jad-80-3-jad210042/jad-80-jad210042-g003.jpg)
As shown in Table 1, the four study subjects were primarily in the early stages of dementia. However, based on the study results, LDIR was performed in a prodromal stage in high-risk patients before serious neurological damage, during asymptomatic periods, and during mild cognitive impairment, which is expected to delay the onset of symptoms in patients with AD and dementia and contribute to breakthrough development in the treatment of AD.
Table 1
Clinical trials using LDIR for the treatment of Alzheimer’s Disease
NCT No./ Institution | Inclusion criteria | RT dose | Primary outcome | Secondary outcome |
02359864/Beaumont Hospital | N:30 | A:10Gy/5fx | Safety & Toxicity | PET, |
MMSE; 10–20 | B: 20Gy/10fx | Neurocognitive test, | ||
Age:>55 y | 12 months after RT | |||
02769000/University of Virginia Commonwealth | N:30 | A: 10Gy/5fx | Safety & Toxicity | Neurocognitive test, |
MMSE; not record prodromal-moderate stage | B: 20Gy/10fx | 12 months after RT | ||
Age:>55 y | ||||
03352258/University of Geneva | N:20 | A: No RT | Safety & Toxicity | Neurocognitive test, |
MMSE; not record | B: 10Gy/5fx | 12 months after RT | ||
Age: 18–80 y | ||||
04203121/ Our Studies | N:10 | A: 9Gy/5fx | Safety & Toxicity | MRI, PET, |
KMMSE:10–24 | B: 5.4Gy/3fx | Neurocognitive test, | ||
Age: 50–90 y | 12 months after RT |
RT, Radiation Treatment; MMSE, Mini-Mental Status Examination; KMMSE, Korean version Mini-Mental Status Examination.
Unfortunately, we could not confirm neurogenesis after LDIR in two experiments, but it is difficult to completely eliminate the possibility of neurogenesis when we can clearly observe an increase in cognitive function at eight weeks after low-dose brain irradiation. Since clinical research is ongoing, it is not possible to confirm the improvement of cognitive function, and LDIR has been reported to improve cognitive function in the animal model (5XFAD model).
Therefore, it can be suggested that it is even effective to administer radiation therapy in the prodromal stage or early stage where irreversible neurodegeneration is relatively minor due to nerve damage caused by Aβ proteins and tau-associated neurofibrillary tangles.
DETERMINATION OF TOTAL RADIATION DOSE, FRACTIONATION SIZE, AND INTERFRACTIONAL INTERVAL
Total dose
According to the preclinical results published in 2016 by Marples et al., the effects of 10 Gy/5 fx were investigated in the right side of the brain of dementia mice [3]. The number of Aβ plaques in the treated right hemisphere decreased by 72%as compared with in the left hemisphere that did not receive radiation treatment; this dose was suggested as a cost-effective regimen.
In a previous study, tracheobronchial amyloidosis was treated with radiation; a 78%decrease of Aβ was reported when 20 Gy/10 fx was administered [17]. Considering cognitive dysfunction or late complications, setting the total dose to 10 Gy/5 fx for the purpose of sparing the hippocampus is reasonable [18]. The total dose of four clinical trials currently being performed varies BED2 = 20 to 40 Gy2 a in the whole brain. The total radiation dose used in this investigator’s clinical study was lower than those in the other three studies.
The 9 Gy/5 fx dose was determined based on a mean dose of 9 Gy entering the hippocampus, and Aβ reduction was expected not to be lower than that seen with the existing 10 Gy/5 fx dose, with no difference in cognitive function improvement [19]. In contrast, a 5.4 Gy/3 fx dose was deployed in an experimental group. In a 2016 study, a total of 20 mGy (20 cGy) was administered to AD patient for several months over five cranial computed tomography scans [20]. Then, the experimental group was used to determine whether a reduced dose could be used successfully in low-dose radiation treatment. Eventually, the radiation dose required for the treatment of AD patients may be determined based on ongoing clinical research results. In solid cancer treatment, various treatment doses are used depending on the treatment purpose and cell type. Further clinical and preclinical research to elucidate the optimal total dose for AD is warranted.
Fractionation number, fraction size, and interfractional interval of ionizing radiation
In recent years, hypofractionated radiation treatment regimens such as stereotactic radiosurgery and stereotactic body radiation therapy have been widely adopted for the treatment of solid cancers. These radiation techniques can be very convenient strategies because their treatment effects can be maximized by irradiating the treatment site with a large fraction size of radiation once or several times in a short period of time as compared with during conventional radiation treatment, thus shortening the treatment period for the patient. However, many disadvantages also exist that can lead to the onset of acute or late complications. Furthermore, when radiation therapy is performed for benign diseases such as degenerative neurological diseases, the total dose of radiation is important; however, the fraction size and the number of fractionations are also significant points of consideration.
Bistolfi et al. recommended administering a total of 20 Gy once a week at the time of irradiation for six months to one year (50 cGy×40–60 weeks or 100 cGy×20–30 weeks) [21]. In clinical studies currently underway, the fraction size is 180 to 200 cGy, which is larger than that proposed by Bistolfi et al., and the treatment period is also very short. According to a Beaumont Hospital study, even using the same BED dose, the number of Aβ plaques was decreased more significantly with a smaller fraction-size regimen.
However, at 10 Gy/10 fx BED2 = 15 Gy2, 10 Gy/5 fx BED2 = 20 Gy2, the total dose was 10 Gy, which caused a difference in Aβ reduction. However, differences in cognitive function were not reported between regimens. Because patients are concerned about nerve damage and late complications, it is recommended that the radiation fraction size not exceed 200 cGy.
In particular, many preclinical results as well as clinical results of radiotherapy for arthritis showed cell proliferation and anti-inflammatory effects were obtained with less than 100 cGy; however, clinical results for AD are not yet available [22].
Aβ reduction is reported to be the largest at a total radiation dose of 20 Gy (BED2 = 40 Gy2); however, whether Aβ reduction causes cognitive improvement and shows a significant difference on amyloid positron-emission tomography needs to be confirmed in future clinical studies. In addition, although whole-brain radiation therapy is being performed with the same dose in existing clinical studies, the use of hippocampus-sparing intensity-modulated radiotherapy in the future is possible by adjusting the radiation dose administered to the cerebral cortex and hippocampus in consideration of late complications in the hippocampus.
The interfractional interval between radiation treatments is also very valuable considering the repopulation of normal cells. In several studies, LDIR was conducted in patients with degenerative arthritis who did not respond to drugs. In these studies, the LDIR regimen was 1 Gy/6 fx for two weeks [23] and 0.5 Gy twice weekly for four weeks repeated once after eight weeks[24]. As indicated in the above study, the intervals of radiotherapy vary. All reported clinical studies of AD recommend daily radiotherapy five times a week. For redistribution and repopulation of normal neurons, an additional research protocol is needed to wide the interval of radiation treatment. Therefore, we can consider the long-term interval between fractions for AD patients as being effective like palliative radiation for uncontrollable arthritis.
CONCLUSIONS
To date, four clinical studies on the role of LDIR in whole-brain radiation therapy for patients with early or mid-stage AD have been reported; however, definitive clinical results have not yet been published. As such, despite the promising results of these preclinical studies, radiotherapy can be applied to patients with AD only when clinical results are made available. In addition, in the case of low-dose radiation therapy, additional large-scale clinical studies are needed to determine the severity of AD, indications for radiation therapy, the total dose to be irradiated, fraction size, and intervals of radiation therapy.
If low-dose radiation therapy is used to treat AD, it is expected to become a breakthrough treatment method at a relatively low cost and short treatment period compared to the cost of drug development and treatment and treatment period. This treatment could play a significant role in reducing social and national medical costs and improving the lives of AD patients and their caregivers as well as of patients with other diseases.
ACKNOWLEDGMENTS
This work was supported by the National Res-earch Foundation of Korea (NRF) grant funded by the Korea government (MSIT) (NRF-2016R1D1A1B03935770 to W.K.C) and Medical Science Res-earch Institute grant, Kyung Hee University Hospital at Kangdong in 2018 to W.K.C.
Authors’ disclosures available online (https://www.j-alz.com/manuscript-disclosures/21-0042).
REFERENCES
[1] | Juniku N , Micke O , Seegenschmiedt MH , Muecke R ((2019) ) Radiotherapy for painful benign skeletal disorders: Results of a retrospective clinical quality assessment. Strahlenther Onkol 195: , 1068–1073. |
[2] | Liu X , Hou D , Lin F , Luo J , Xie J , Wang Y , Tian Y ((2019) ) The role of neurovascular unit damage in the occurrence and development of Alzheimer’s disease. Rev Neurosci 30: , 477–484. |
[3] | Marples B , Mcgee M , Callan S , Bowen SE , Thibodeau BJ , Michael DB , Wilson GD , Maddens ME , Fontanesi J , Martinez AA ((2016) ) Cranial irradiation significantly reduces beta amyloid plaques in the brain and improves cognition in a murine model of Alzheimer’s disease (AD). Radiother Oncol 118: , 43–51. |
[4] | Ceyzeriat K , Tournier BB , Millet P , Frisoni GB , Garibotto V , Zilli T ((2020) ) Low-dose radiation therapy: A new treatment strategy for Alzheimer’s disease? J Alzheimers Dis 74: , 411–419. |
[5] | Laack NN , Brown PD ((2004) ) Cognitive sequelae of brain radiation in adults. Semin Oncol 31: , 702–713. |
[6] | Gondi V , Pugh SL , Tome WA , Caine C , Corn B , Kanner A , Rowley H , Kundapur V , DeNittis A , Greenspoon JN , Konski AA , Bauman GS , Shah S , Shi W , Wendland M , Kachnic L , Mehta MP ((2014) ) Preservation of memory with conformal avoidance of the hippocampal neural stem-cell compartment during whole-brain radiotherapy for brain metastases (RTOG 0933): A phase II multi-institutional trial. J Clin Oncol 32: , 3810–3816. |
[7] | Wilson GD , Marples B ((2016) ) A new use for an old treatment: Radiation therapy and Alzheimer’s disease. Radiat Res 185: , 443–448. |
[8] | Wilson GD , Wilson TG , Hanna A , Fontanesi G , Kulchycki J , Buelow K , Pruetz BL , Michael DB , Chinnaiyan P , Maddens ME , Martinez AA , Fontanesi J ((2020) ) Low dose brain irradiation reduces amyloid-beta and tau in 3xTg-AD mice. J Alzheimers Dis 75: , 15–21. |
[9] | Kim S , Nam Y , Kim C , Lee H , Hong S , Kim HS , Shin SJ , Park YH , Mai HN , Oh SM , Kim KS , Yoo DH , Chung WK , Chung H , Moon M ((2020) ) Neuroprotective and anti-inflammatory effects of low-moderate dose ionizing radiation in models of Alzheimer’s disease. Int J Mol Sci 21: , 3678. |
[10] | Kim S , Chung H , Mai HN , Nam Y , Shin SJ , Park YH , Chung MJ , Lee JK , Rhee HY , Jahng GH , Kim Y , Lim YJ , Kong M , Moon M , Chung WK ((2020) ) Low-dose ionizing radiation modulates microglia phenotypes in the models of Alzheimer’s disease. Int J Mol Sci 21: , 4532. |
[11] | Patrias LM , Klaver AC , Coffey MP , Finke JM , Digambaranath JL , Dang L , Martinez AA , Loeffler DA ((2011) ) Effects of external beam radiation on in vitro formation of Abeta1-42 fibrils and preformed fibrils. Radiat Res 175: , 375–381. |
[12] | Garibotto V , Frisoni GB , Zilli T ((2016) ) Re: Cranial irradiation significantly reduces beta amyloid plaques in the brain and improves cognition in a murine model of Alzheimer’s disease (AD). Radiother Oncol 118: , 577–578. |
[13] | Block ML , Zecca L , Hong JS ((2007) ) Microglia-mediated neurotoxicity: Uncovering the molecular mechanisms. Nat Rev Neurosci 8: , 57–69. |
[14] | Davis BM , Salinas-Navarro M , Cordeiro MF , Moons L , De Groef L ((2017) ) Characterizing microglia activation: A spatial statistics approach to maximize information extraction. Sci Rep 7: , 1576. |
[15] | Yang RR , Wang H , Wen J , Ma K , Chen DJ , Chen Z , Huang C ((2019) ) Regulation of microglial process elongation, a featured characteristic of microglial plasticity. Pharmacol Res 139: , 286–297. |
[16] | Masters CL , Bateman R , Blennow K , Rowe CC , Sperling RA , Cummings JL ((2015) ) Alzheimer’s disease. Nat Rev Dis Primers 1: , 15056. |
[17] | Kurrus JA , Hayes JK , Hoidal JR , Menendez MM , Elstad MR ((1998) ) Radiation therapy for tracheobronchial amyloidosis. Chest 114: , 1489–1492. |
[18] | Nakahara Y , Takagi Y , Okuma Y , Hosomi Y , Okamura T , Shibuya M , Masuda N ((2015) ) Neurotoxicity due to prophylactic cranial irradiation for small-cell lung cancer: A retrospective analysis. Mol Clin Oncol 3: , 1048–1052. |
[19] | Brown PD , Gondi V , Pugh S , Tome WA , Wefel JS , Armstrong TS , Bovi JA , Robinson C , Konski A , Khuntia D , Grosshans D , Benzinger TLS , Bruner D , Gilbert MR , Roberge D , Kundapur V , Devisetty K , Shah S , Usuki K , Anderson BM , Stea B , Yoon H , Li J , Laack NN , Kruser TJ , Chmura SJ , Shi WY , Deshmukh S , Mehta MP , Kachnic LA ((2020) ) Hippocampal avoidance during whole-brain radiotherapy plus memantine for patients with brain metastases: Phase III Trial NRG Oncology CC001. J Clin Oncol 38: , 1019–1029. |
[20] | Cuttler JM , Moore ER , Hosfeld VD , Nadolski DL ((2016) ) Treatment of Alzheimer disease with CT scans: A case report. Dose Response 14: , 1559325816640073. |
[21] | Bistolfi F ((2008) ) Localized amyloidosis and Alzheimer’s disease: The rationale for weekly long-term low dose amyloid-based fractionated radiotherapy. Neuroradiol J 21: , 683–692. |
[22] | Gueguen Y , Bontemps A , Ebrahimian TG ((2019) ) Adaptive responses to low doses of radiation or chemicals: Their cellular and molecular mechanisms. Cell Mol Life Sci 76: , 1255–1273. |
[23] | Van den Ende CHM , Minten MJM , Leseman-Hoogenboom MM , van den Hoogen FHJ , Den Broeder AA , Mahler EAM , Poortmans PMP ((2020) ) Long-term efficacy of low-dose radiation therapy on symptoms in patients with knee and hand osteoarthritis: Follow-up results of two parallel randomised, sham-controlled trials. Lancet Rheumatol 2: , E42–E49. |
[24] | Rogers S , Eberle B , Vogt DR , Meier E , Moser L , Ordonez SG , Desborough S , Riesterer O , Takacs I , Hasler P , Bodis S ((2020) ) Prospective evaluation of changes in pain levels, quality of life and functionality after low dose radiotherapy for epicondylitis, plantar fasciitis, and finger osteoarthritis. Front Med 7: , 195. |