Elevated Norepinephrine Metabolism Gauges Alzheimer’s Disease-Related Pathology and Memory Decline
Abstract
The noradrenergic (NE) locus coeruleus (LC) is vulnerable to hyperphosphorylated tau, and dysregulated NE-metabolism is linked to greater tau and disease progression. We investigated whether elevated NE-metabolism alone predicts memory decline or whether concomitant presence of tau and amyloid-β is required. Among 114 memory clinic participants, time trends (max. six years) showed dose-response declines in learning across groups with elevated NE-metabolite 3-methoxy-4-hydroxyphenylethyleneglycol (MHPG) with no, one, or two Alzheimer’s disease biomarkers; and no decline in the low MHPG group. Elevated MHPG is required and sufficient to detect learning declines, supporting a pathophysiologic model including the LC-NE system contributing to initial Alzheimer’s disease-related processes.
INTRODUCTION
Autopsy studies reported that the locus coeruleus (LC), the major site for norepinephrine (NE) synthesis, is one of the first brain regions to accumulate hyperphosphorylated tau pathology [1, 2]. The role of the LC in the late-life phenotypes of Alzheimer’s disease (AD) remains unclear, but recent work com-bining in vitro, animal experiments, and postmortem human AD brains showed that higher DOPEGAL (3,4-dihydroxyphenylglycolaldehyde), a metabolite of NE measured in LC cells, was associated with greater tau aggregation, propagation and cognitive deficits [3]. Correspondingly, in humans, fewer rem-aining number of neurons in an atrophied LC were associated with increased NE-activity in the medial frontal cortex [4–6], as measured with the metabolite 3-methoxy-4-hydroxyphenylethyleneglycol (MHPG), another NE-metabolite downstream to DOPEGAL. Higher MHPG in the cerebrospinal fluid (CSF) has been linked to increases in disease severity, higher tau pathology and cognitive impairment [6, 7]. However, it remains unclear whether cognitive impairment requires elevated MHPG or rather results from the coinciding pathological hallmarks of AD, amyloid-β (Aβ) and tau.
We aimed to examine the relationship between hyperphosphorylated tau, Aβ, and MHPG, measured in CSF. In addition, we investigated whether memory decline shows a dose-response relationship depending on whether MHPG is elevated and whether MHPG is accompanied by AD biomarkers. Ultimately, these results will facilitate our understanding of the LC-NE system in the pathophysiological cascade of AD and whether NE-related markers can serve as indicator for disease progression.
METHODS
Participants
Participants (n=114) were included from a convenience cohort from the memory clinic of the Maastricht University Medical Center (MUMC), diagnosed with subjective cognitive decline (SCD, n=60), mild cognitive impairment (MCI, n=35) or probable AD dementia (n=19). Diagnoses were made by experienced physicians based on the core clinical criteria for MCI [8] and AD dementia [9]. Criteria for SCD included self-reported presence of subjective cognitive complaints and no impairments on cognitive tests (impairment is defined as a score below –1.5SD of the age-, sex-, and education-adjusted mean). Participants receiving a SCD diagnosis could have a CDR-rating >0 if cognitive tests were considered normal. Exclusion criteria were: major neurological disease, clinical diagnosis of other neurodegenerative disorders, recent transient ischemic attack or cerebrovascular accident, history of psychiatric disorders, and alcohol or drug abuse [7]. All participants provided consent, study protocols were approved by the Medical Ethics Committee of the MUMC and conform the World Medical Association Declaration of Helsinki.
CSF analyses and genetics
CSF was collected via a lumbar puncture and biochemical analysis of CSF Aβ1–42, p-tau181p, and MHPG was done blinded to diagnostic information and following standardized protocol [7]. APOE genotyping was determined using polymerase chain reaction.
Neuropsychological assessment
The cognitive assessment included the Clinical Dementia Rating (CDR) scale, Mini-Mental State Examination (MMSE), categorical verbal fluency task, letter-digit substitution test (LDST), and the verbal 15-word learning task (WLT, learning and delayed recall). Participants were invited for annual follow-up visits over a six-year period. Following our previous analyses, we focused on the WLT components in this study [7].
Statistical analyses
Statistical analyses were conducted in R version 3.5.1. We assessed the relationship between MHPG, CSF-p-tau or Aβ with linear regression (adjusting for age, sex, education, CDR-score, and APOE). Reported estimates are standardized. Commonality analysis quantified the explained variance accounted for by respective predictor variables and floodlight analyses determined the range at which Aβ modified the relationship between MHPG and p-tau. To determine whether MHPG by itself or in the presence of worse AD-pathology was associated with memory decline, we created four groups using median-split for MHPG, p-tau and Aβ (higher CSF p-tau or lower CSF Aβ are referred to as elevated): “elevated MHPG, no elevated AD-biomarkers (MHPG+)”, “elevated MHPG with one AD-biomarker elevated (MHPG+1)”, “elevated MHPG with two AD-biomarkers elevated (MHPG+2)” or “low MHPG (MHPG-)”. A median split was chosen to ensure sufficient statistical power for further analyses (per group minimum n=20).
These groups were fed as a predictor into a linear mixed effects model with random intercepts for each subject and random slopes for time, with either WLT learning or recall as outcome and adjusting for age, sex, education, APOE, and interactions with time. Time-trends and group contrasts were estimated using marginal means with false discovery rate-adjustment. Statistical threshold was set at p<0.05.
RESULTS
Mean age of the sample was 62.97 year, with almost half of the individuals carrying at least one APOE ɛ4 allele (48.25%) and the majority having a CDR score≥0.5 (n=106, 92.98%, Table 1). Participants were followed for an average of 2.16 years, with 80 participants having two, n=52 three, n=27 four, and n=8 five timepoints (total 281 observations).
Table 1
Demographics of the MHPG/Biomarker groups
Entire sample | MHPG- | MHPG+ | MHPG+1 | MHPG+2 | F-test/χ2 | |
(n=114) | (n=45) | (n=21) | (n=21) | (n=27) | ||
Age (y) | 62.97 (9.04) | 60.93 (9.26) | 59.57 (6.65) | 63.19 (9.90) | 68.85# (6.95) | 6.35*** |
Sex (female, n (%)) | 33 (28.95%) | 14 (31%) | 4 (19%) | 2 (9%) | 13 (48%)⊕ | 9.79* |
Education (level) | 3.89 (1.93) | 3.67 (1.98) | 3.66 (2.08) | 4.09 (1.76) | 4.30 (1.90) | 0.77 |
APOE ɛ4 (n, (%)) | 55 (48.25%) | 24 (53%) | 5 (24%) | 8 (38%) | 18 (67%)$ Ω | 10.02* |
Diagnostic groups (n) | 60 SCD (53%) | 23 SCD (51%) | 16 SCD (76%) | 16 SCD (76%) | 5 SCD (19%) | 31.1*** |
35 MCI (31%) | 15 MCI (33%) | 5 MCI (24%) | 5 MCI (24%) | 10 MCI (37%) | ||
19 AD (16%) | 7 AD (16%) | – | – | 12 AD (44%) | ||
MMSE (score) | 27.56 (2.52) | 27.56† (2.84) | 28.67 (1.20) | 28.15 (1.46) | 26.26† (2.82) | 4.51** |
Baseline WLT learning (words) | 38.02 (11.97) | 37.84 (10.97) | 46.48 (11.34) | 42.10 (8.25) | 28.56# $ (10.16) | 13.14*** |
Baseline WLT recall (words) | 6.79 (4.04) | 7.13 (3.80) | 8.71 (3.69) | 8.33 (2.97) | 3.52# (3.63) | 10.86*** |
CSF Aβ (pg/ml) | 923.08 (360.79) | 951.60 (342.84) | 1203.71 (193.07) | 1053.33 (356.96) | 555.96# $ (146.43) | 23.11** |
CSF p-tau (pg/ml) | 62.24 (33.64) | 55.98 (30.64) | 41.10 (6.95) | 48.24 (9.71) | 100.00# ∧ (33.93) | 26.61*** |
MHPG (nmol/l) | 38.10 (9.01) | 29.86 (4.25) | 42.05 (5.00) | 43.76 (7.84) | 44.93+ (6.83) | 52.09*** |
Follow-up time (y) | 2.16 (1.34) | 2.26 (1.41) | 2.35 (1.46) | 2.00 (1.24) | 1.80 (1.05) | 1.11 |
Number of observations | 281 | 129 | 54 | 45 | 53 | 1.37 |
Mean and standard deviations of demographics of the entire cohort and the four subgroups. Group differences were tested with ANOVA (followed by pairwise t-tests) or chi-square test. *p<0.05; **p<0.01; ***p<0.001;#MHPG+2 >MHPG-=MHPG+=MHPG+1;$MHPG-<MHPG+∧MHPG->MHPG+ ⊕MHPG+2 >MHPG+1=MHPG+;ΩMHPG+2 >MHPG+†MHPG+2 <MHPG+=MHPG+1;+MHPG-<MHPG+ MHPG1+ MHPG2+and MHPG+ <MHGP2+. Linear mixed effects models were adjusted for age, sex, education, and APOE because of these group differences. Education: education level (8 levels), this coding system is based on Verhage (1964) and the Standard Classification of Education of the Dutch Central Bureau of Statistics (CBS, 2014). It is equivalent to the International Standard Classification of Education (UNESCO, 1997). There was one missing value for the MMSE total score in the MHPG+1 group.
MHPG was not associated with Aβ (β=–0.07, t(108)=–0.93, p=0.35, 95% CI[–0.23, 0.09]), but positively associated with higher p-tau (β=0.25, t(108)=3.12, p=0.002, 95% CI [0.09, 0.41]), and this effect was modified by Aβ (β=–1.08, t(106)=–2.60, p=0.011, 95% CI[–1.89,–0.26], R2=0.45 (adjusted R2=0.41), Fig. 1A). A large proportion of explained variance of p-tau is shared among MHPG, Aβ, and its interaction (21.9%), but MHPG contributed the largest unique effect in explained variance (14.88%), apart from the covariates (Fig. 1B). Floodlight analyses indicated that the relationship between p-tau and MHPG was significant at a range that includes CSF-Aβ values considered to be normal (<938pg/ml (clinical cutoff: 500pg/ml), Fig. 1A and Supplementary Figure 1).
Fig. 1
Associations between MHPG, Aβ, and p-tau and their relevance to memory decline. A) Interaction between CSF MHPG and CSF Aβ on CSF p-tau showing that a significant positive relationship between MHPG and p-tau occurs at Aβ-values <938pg/ml (derived from floodlight analyses; see Supplementary Figure 1). Analyses were done continuously but are grouped for visualization purposes; B) Variance decomposition of unique and shared contributions in explaining CSF p-tau of the model in A; C) Linear mixed effects model demonstrating that participants in the MHPG- group decline significantly less than the other three groups on the WLT learning over max. 6 years.
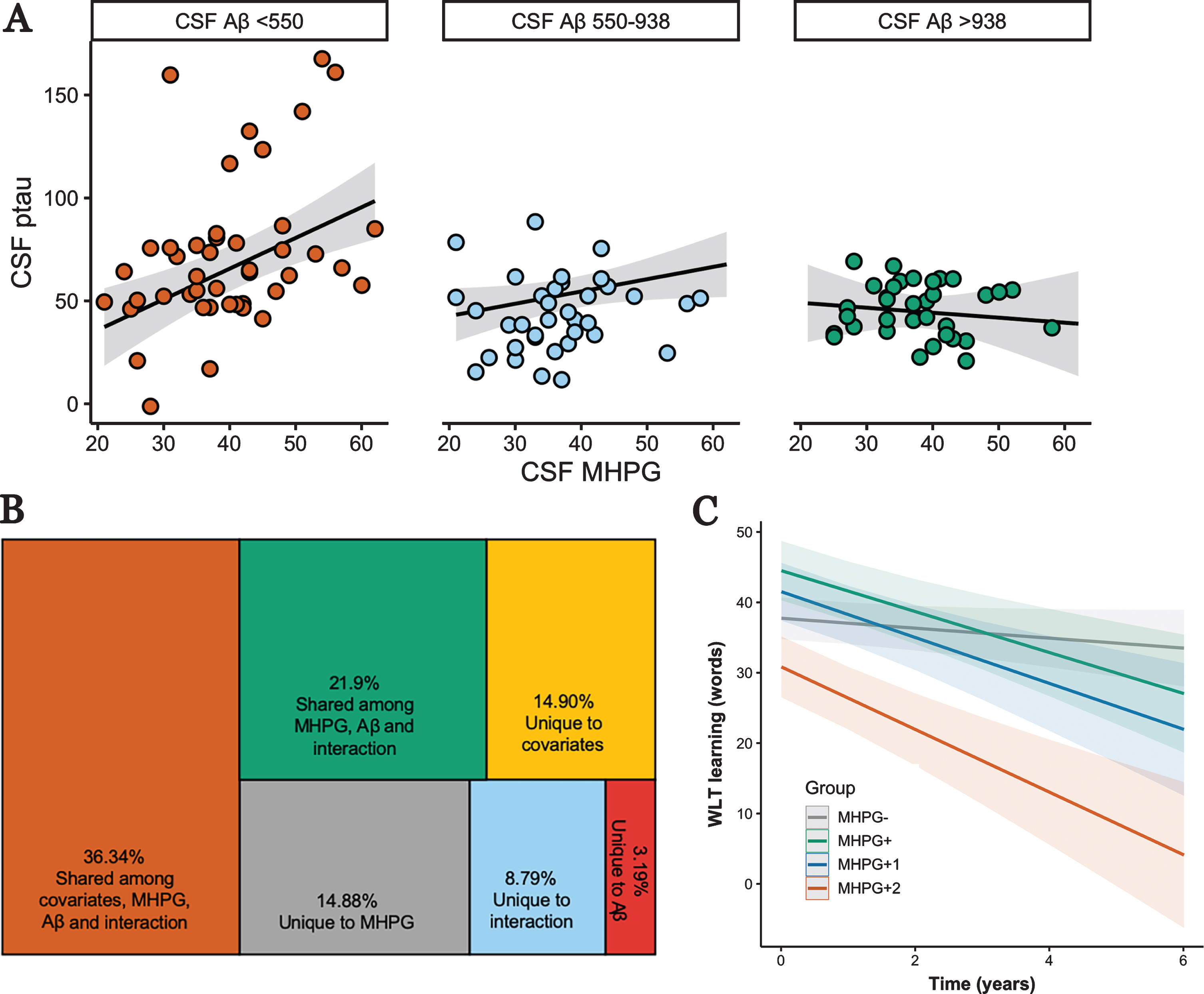
We then examined whether there is a dose-response relationship between elevated MHPG and having none, one, or two elevated AD-biomarkers in predicting memory decline (see Supplemental Material for relationships between each CSF marker and learning over time). To that end we created groups based on the median of the respective biomarker (Table 1). We assume missing at random given that the number of observations was not different among the groups (F (3,277) =2.01, p=0.12). For the WLT learning, est-imated time trends within each group revealed a dose-response effect: MHPG- (–1.12 words/year, 95% CI[–2.35, 0.12])<MHPG+ (–3.43 words/year, 95% CI [–5.36, –1.50])<MHPG+1 (–3.59 words/year, 95% CI [–5.84,–1.34])<MHPG+2 (–4.28 words/year, 95% CI [–6.58, –1.98]). These time trends differed significantly between the MHPG- group and the other groups (F (3,266) =6.29, p<0.001; MHPG- versus MHPG+: t(45.1)=2.80, pFDR=0.02; MHPG- versus MHPG+1: t(67.7)=2.62, pFDR=0.02; MHPG- versus MHPG+2: t(62)=3.10, pFDR=0.018; Fig.1C). Even though, the MHPG+2 group consisted of a higher proportion of AD patients, results remained similar when additionally adjusting for diagnosis. No other contrasts were significant (all ps>0.68). Adding total CDR-score as a covariate did not change these results. For the delayed recall, we observed no differences in the slopes between the groups (F (3,266) =0.50, p=0.68). To further understand the role of MHPG and AD-biomarkers in the MHPG- group, we separated the MHPG- group posthoc into three groups based on the same median cut-points (MHPG- (n=17; 45 observations); MHPG- with one elevated biomarker (n=13; 44 observations) and MHPG with two elevated biomarkers (n=15; 40 observations)), but observed no group differences among these three groups in predicting decline on the WLT learning (F (2,116,) =1.82, p=0.16) or delayed recall (F (2,116) =0.62, p=0.54).
DISCUSSION
In this study, higher NE-metabolism was associated with higher CSF p-tau at Aβ values above the clinical cut-off. In addition, elevated MHPG was associated with declines in learning over a 6-year period, and memory decline was steeper in the presence of higher p-tau or Aβ pathology. Interestingly, AD pathology in the context of lower MHPG was not associated with significant memory decline. Based on these findings, we speculate that NE hypermetabolism by itself is a sufficient and required mechanisms related to decline in learning. Mounting postmortem and animal work suggests that the LC contributes to progression of pathology and symptomatology of AD [2, 3, 10, 11]. Our observations are consistent with a pathophysiology of AD in which changes in the LC-NE system may precede initial accumulation of tau pathology and subtle memory decline [12, 13], prior to significant deposition of Aβ. However, serial observations and experiments manipulating NE metabolism will be required to verify this pathophysiological sequence. Elevated NE metabolism may hold promise as early indicator of disease progression, at a stage where brain changes may be reversible and treatments be more efficacious.
Elevated MHPG with or without AD biomarkers was related to decline in learning, but the groups did not differ with respect to decline on delayed recall. Animal and human studies indicated that activation of the LC-NE system modulates all stages of memory processing, encoding, consolidation, and retrieval [14, 15]. In our data, all groups showed decline in recall performance. Recall scores are known to reflect short-term decline, whereas learning scores are more sensitive to long-term decline and have more clinical meaningfulness [16]. Examining relationships among NE-metabolism, AD-biomarkers and cognitive performance along the entire disease trajectory can shed light on the temporal dynamics of cognitive processes affected by LC alterations.
This study has strengths, including the combination of multiple CSF markers and longitudinal cognitive data, but, unfortunately, the modest sample size did not allow us to further split up the groups based on other thresholds, search for more complex interactions or consider nonlinear learning functions in a latent growth curve framework [17]. Replication in larger cohorts, ideally with longitudinal datapoints, will be necessary to investigate effect modifications, influence of aminergic mediation use, generalizability to other populations including those with lower proportions of APOE ɛ4 carriers, as well as temporal ordering of all markers and the evolution of LC hypermetabolism as the disease progresses. The diffusion of MHPG into spinal cord tissue has challenged its interpretation as a pure indicator of NE metabolism [18] (but see [19–22]). Future studies should consider including CSF NE and plasma MHPG as additional measures, but similar associations can be expected given that CSF MHPG measures correlate very highly with plasma measures [19–22]. Finally, while animal work indicates that NE metabolism may be directly linked to formation of LC tau [3], it is challenging to examine this in vivo as we do not yet have a method that is specifically sensitive to LC tau pretangle material.
ACKNOWLEDGMENTS
This work was supported by a standard grant of Alzheimer Nederland [#15007, HILJ]. Dr. Jacobs receives funding from the National Institute on Aging [R01 AG062559, HILJ]. Dr. Van Egroo is supported by an excellence grant from WBI.World [SOR/2020/479197].
Authors’ disclosures available online (https://www.j-alz.com/manuscript-disclosures/20-1411r1)
SUPPLEMENTARY MATERIAL
[1] The supplementary material is available in the electronic version of this article: https://dx.doi.org/10.3233/JAD-201411
REFERENCES
[1] | Braak H , Thal DR , Ghebremedhin E , Del Tredici K ((2011) ) Stages of the pathologic process in Alzheimer disease: Age categories from 1 to 100 years. J Neuropathol Exp Neurol 70: , 960–969. |
[2] | Ehrenberg AJ , Nguy AK , Theofilas P , Dunlop S , Suemoto CK , Di Lorenzo Alho AT , Leite RP , Diehl Rodriguez R , Mejia MB , Rub U , Farfel JM , de Lucena Ferretti-Rebustini RE , Nascimento CF , Nitrini R , Pasquallucci CA , Jacob-Filho W , Miller B , Seeley WW , Heinsen H , Grinberg LT ((2017) ) Quantifying the accretion of hyperphosphorylated tau in the locus coeruleus and dorsal raphe nucleus: The pathological building blocks of early Alzheimer’s disease. Neuropathol Appl Neurobiol 43: , 393–408. |
[3] | Kang SS , Liu X , Ahn EH , Xiang J , Manfredsson FP , Yang X , Luo HR , Liles LC , Weinshenker D , Ye K ((2020) ) Norepinephrine metabolite DOPEGAL activates AEP and pathological Tau aggregation in locus coeruleus. J Clin Invest 130: , 422–437. |
[4] | Hoogendijk WJ , Feenstra MG , Botterblom MH , Gilhuis J , Sommer IE , Kamphorst W , Eikelenboom P , Swaab DF ((1999) ) Increased activity of surviving locus ceruleus neurons in Alzheimer’s disease. Ann Neurol 45: , 82–91. |
[5] | Raskind MA , Peskind ER , Holmes C , Goldstein DS ((1999) ) Patterns of cerebrospinal fluid catechols support increased central noradrenergic responsiveness in aging and Alzheimer’s disease. Biol Psychiatry 46: , 756–765. |
[6] | Sheline YI , Miller K , Bardgett ME , Csernansky JG ((1998) ) Higher cerebrospinal fluid MHPG in subjects with dementia of the Alzheimer type. Relationship with cognitive dysfunction. Am J Geriatr Psychiatry 6: , 155–161. |
[7] | Jacobs HIL , Riphagen JM , Ramakers I , Verhey FRJ ((2019) ) Alzheimer’s disease pathology: Pathways between central norepinephrine activity, memory, and neuropsychiatric symptoms. Mol Psychiatry, doi: 10.1038/s41380-019-0437-x |
[8] | Albert MS , DeKosky ST , Dickson D , Dubois B , Feldman HH , Fox NC , Gamst A , Holtzman DM , Jagust WJ , Petersen RC , Snyder PJ , Carrillo MC , Thies B , Phelps CH ((2011) ) The diagnosis of mild cognitive impairment due to Alzheimer’s disease: Recommendations from the National Institute on Aging-Alzheimer’s Association workgroups on diagnostic guidelines for Alzheimer’s disease. Alzheimers Dement 7: , 270–279. |
[9] | McKhann GM , Knopman DS , Chertkow H , Hyman BT , Jack CR Jr. , Kawas CH , Klunk WE , Koroshetz WJ , Manly JJ , Mayeux R , Mohs RC , Morris JC , Rossor MN , Scheltens P , Carrillo MC , Thies B , Weintraub S , Phelps CH ((2011) ) The diagnosis of dementia due to Alzheimer’s disease: Recommendations from the National Institute on Aging-Alzheimer’s Association workgroups on diagnostic guidelines for Alzheimer’s disease. Alzheimers Dement 7: , 263–269. |
[10] | Chalermpalanupap T , Schroeder JP , Rorabaugh JM , Liles LC , Lah JJ , Levey AI , Weinshenker D ((2018) ) Locus coeruleus ablation exacerbates cognitive deficits, neuropathology, and lethality in P301S tau transgenic mice. J Neurosci 38: , 74–92. |
[11] | Theofilas P , Ehrenberg AJ , Dunlop S , Di Lorenzo Alho AT , Nguy A , Leite REP , Rodriguez RD , Mejia MB , Suemoto CK , Ferretti-Rebustini REL , Polichiso L , Nascimento CF , Seeley WW , Nitrini R , Pasqualucci CA , Jacob Filho W , Rueb U , Neuhaus J , Heinsen H , Grinberg LT ((2017) ) Locus coeruleus volume and cell population changes during Alzheimer’s disease progression: A stereological study in human postmortem brains with potential implication for early-stage biomarker discovery. Alzheimers Dement 13: , 236–246. |
[12] | Braak H , Del Tredici K ((2016) ) Potential pathways of abnormal tau and alpha-synuclein dissemination in sporadic Alzheimer’s and Parkinson’s diseases. Cold Spring Harb Perspect Biol 8: , a023630. |
[13] | Weinshenker D ((2018) ) Long road to ruin: Noradrenergic dysfunction in neurodegenerative disease. Trends Neurosci 41: , 211–223. |
[14] | Hansen N ((2017) ) The longevity of hippocampus-dependent memory is orchestrated by the locus coeruleus-noradrenergic system. Neural Plast 2017: , 2727602. |
[15] | Jacobs HI , Priovoulos N , Poser BA , Pagen LH , Ivanov D , Verhey FR , Uludag K ((2020) ) Dynamic behavior of the locus coeruleus during arousal-related memory processing in a multi-modal 7T fMRI paradigm. Elife 9: , e52059. |
[16] | Mormino EC , Papp KV , Rentz DM , Donohue MC , Amariglio R , Quiroz YT , Chhatwal J , Marshall GA , Donovan N , Jackson J , Gatchel JR , Hanseeuw BJ , Schultz AP , Aisen PS , Johnson KA , Sperling RA ((2017) ) Early and late change on the preclinical Alzheimer’s cognitive composite in clinically normal older individuals with elevated amyloid beta. Alzheimers Dement 13: , 1004–1012. |
[17] | Dahl MJ , Mather M , Duzel S , Bodammer NC , Lindenberger U , Kuhn S , Werkle-Bergner M ((2019) ) Rostral locus coeruleus integrity is associated with better memory performance in older adults. Nat Hum Behav 3: , 1203–1214. |
[18] | Eisenhofer G , Kopin IJ , Goldstein DS ((2004) ) Catecholamine metabolism: A contemporary view with implications for physiology and medicine. Pharmacol Rev 56: , 331–349. |
[19] | Elsworth JD , Redmond DE Jr. , Roth RH ((1982) ) Plasma and cerebrospinal fluid 3-methoxy-4-hydroxyphenylethylene glycol (MHPG) as indices of brain norepinephrine metabolism in primates. Brain Res 235: , 115–124. |
[20] | Janssens J , Vermeiren Y , Fransen E , Aerts T , Van Dam D , Engelborghs S , De Deyn PP ((2018) ) Cerebrospinal fluid and serum MHPG improve Alzheimer’s disease versus dementia with Lewy bodies differential diagnosis. Alzheimers Dement (Amst) 10: , 172–181. |
[21] | Kopin IJ , Gordon EK , Jimerson DC , Polinsky RJ ((1983) ) Relation between plasma and cerebrospinal fluid levels of 3-methoxy-4-hydroxyphenylglycol. Science 219: , 73–75. |
[22] | Raskind MA , Peskind ER , Halter JB , Jimerson DC ((1984) ) Norepinephrine and MHPG levels in CSF and plasma in Alzheimer’s disease. Arch Gen Psychiatry 41: , 343–346. |