Peripheral Markers of Neurovascular Unit Integrity and Amyloid-β in the Brains of Menopausal Women
Abstract
Background:
The identification of blood-borne biomarkers for the diagnosis and prognosis of Alzheimer’s disease and related dementias is more feasible at the population level than obtaining cerebrospinal fluid or neuroimaging markers.
Objective:
This study determined the association of blood microvesicles, derived from cells of the neurovascular unit, with brain amyloid-β deposition in menopausal women.
Methods:
A subset of women from the Kronos Early Estrogen Prevention Study underwent brain amyloid-β positron emission tomography three years following cessation of study treatment with placebo (PL, n = 29), transdermal 17β-estradiol (tE2; n = 21), or oral conjugated equine estrogen (oCEE; n = 17). Isolated peripheral venous blood microvesicles were analyzed by digital flow cytometry using fluorophore conjugated antibodies directed toward total tau, amyloid-β 1–42 (Aβ1–42), neuron specific class III β-tubulin (Tuj1), microglia ionized calcium -binding adaptor molecule 1(Iba1), glial fibrillary acid protein (GFAP), and low density lipoprotein receptor-related protein1 (LRP1). Principal components analysis reduced the dimensionality of these selected six markers to two principal components (PCs). Proportional odds ordinal logistic regression analysis was used with amyloid-β deposition regressed on these PCs.
Results:
Only the number of microvesicles positive for Aβ1–42 differed statistically among prior treatment groups (median [IQR]: 6.06 [2.11, 12.55] in PL; 2.49 [0.73, 3.59] in tE2; and 4.96 [0.83, 10.31] in oCEE; p = 0.032). The joint association between the 2 PCs and brain amyloid-β deposition was significant (p = 0.045).
Conclusion:
Six selected markers expressing peripheral blood microvesicles derived from cells of the neurovascular unit, when summarized into two principal components, were associated with brain amyloid-β deposition.
INTRODUCTION
The development of diagnostic and prognostic blood-borne biomarkers for Alzheimer’s disease (AD) pathology and dementia would be more feasible and more cost-effective at the population level compared to cerebrospinal fluid or neuroimaging measures. Studies suggest that it may now be possible to measure the hallmark characteristics of AD, amyloid-β and tau, in the blood [1–8]. However, previous studies have not considered that the ability to measure these proteins in blood not only reflects changes in the activation or function of neurons and glial cells, but also may reflect changes in cerebral microvasculature of the blood-brain barrier. The concept of the neurovascular unit composed of cerebrovascular endothelial cells, glia, and neurons conveys the dynamic interaction among these cellular elements in affecting brain function and repair in relationship to cerebral ischemia, brain injury due to trauma or irradiation, and neurological diseases such as multiple sclerosis and AD [9–15]. An integrative approach which includes the collective analysis of blood-borne markers of both AD pathology and the neurovascular unit may provide insight into early changes in neurovascular cellular activation and function that may precede development of cognitive impairment.
Injured and activated neurovascular cells shed two distinct major populations of biologically-active extracellular vesicles into the blood: 1) Exosomes (shed from endosomes) and 2) Microvesicles (MV; shed from cell membranes). Both are involved in cell-cell communication and the specific exchange of bioactive molecules in pathophysiological processes. Several studies highlighted the importance of these vesicles and their bioactive molecules to identify and understand the early pathogenesis of AD, and the potential for their use as a diagnostic or prognostic biomarker [16–20]. The concentration and content of bioactive molecules of MV in body fluids depend upon their cellular origin and the pathophysiological processes that trigger their production. Thus, the absolute and relative population of MV and their content of bioactive molecules may reflect early or late pathophysiological processes in the brain [16–21]. Therefore, this study was designed to assess the ability to measure MV derived from cells of the neurovascular unit in peripheral blood as potential markers of amyloid-β deposition in the brain. This analysis was performed on blood from a cohort of middle-aged, post-menopausal women who participated in the ancillary study of Kronos Early Estrogen Prevention Study (KEEPS) for measures of brain amyloid-β by positron emission tomography [22].
METHODS
Study participants
Women enrolled in the KEEPS (NCT000154180) ancillary neuroimaging study at Mayo Clinic were eligible for this study. In brief, women were between 42–58 years old and within 6 months to 3 years past their last menses at the time of enrollment for the initial trial. Women were excluded if they had a coronary artery calcium score of > 50 Agatston Units (AU), smoked over 10 cigarettes per day, body mass index (BMI) > 35 kg/m2, a history of cardiovascular disease, LDL cholesterol > 190 mg/dl, triglycerides > 400 mg/dl, diagnosis of diabetes, uncontrolled hypertension (systolic blood pressure > 150 mmHg and/or diastolic blood pressure > 95 mm Hg) and current or recent (6 months) use of cholesterol lowering medications (statins, fibrate, or > 500 mg/day niacin). Women were randomized for four years to: oral conjugated equine estrogens (oCEE; Premarin, 0.45 mg/day); transdermal 17β-estradiol (tE2, Climera, 50μg/day; or placebo (PL) pills and patch. Progesterone was given orally (Prometrium; micronized progesterone, 200 mg/day) for the first 12 days each month to both active treatment groups [23]. The study was approved by the Mayo Clinic Institutional Review Board and all women gave written informed consent.
Neuroimaging
Neuroimaging for the current study was conducted from December 2012 through July 2014 and included the subset of women enrolled in KEEPS at the Mayo Clinic Rochester, MN who agreed to continue the study three years after the end of the trial. Exclusion criteria for the imaging studies were contraindications for safety and neurologic disorders such as brain tumors, multiple sclerosis, neurodevelopmental abnormalities, or treatments (e.g., systemic chemotherapy) that would affect the brain structure.
Positron emission tomography (PET) with Pittsburgh compound-B (PiB) was acquired using a PET/CT scanner (DRX; GE Healthcare) operating in 3D mode three years after the end of hormone therapies. An average of 596 MBq (range, 292–729 MBq) 11C-PiB was used. Following the injection of11C-PiB, a 40 min 11C-PiB uptake period, a 20 minPiB scan consisting of four 5 min dynamic frames was obtained. In addition, MRI examinations were performed at 3 Tesla (GE Healthcare). A 3-D high resolution magnetization prepared rapid gradient echo was obtained for anatomical segmentation and labeling. Standardized uptake value ratios (SUVr) were calculated from the PiB PET images as previously described [24]. Briefly, using an automated image processing pipeline, PET image volumes were registered to each subject’s own 3-dimensional T1-weighted MRI using SPM12. Regional cortical uptake of PiB was determined using the Mayo Clinic Adult Lifespan Template (MCALT_ADIR122) [25]. The global cortical PiB retention standardized uptake value ratio (SUVr) was obtained from the bilateral parietal (including posterior cingulate and precuneus), temporal, prefrontal, orbitofrontal, and anterior cingulate regions referenced to the cerebellar crus region.
Cognitive function
A battery of neuropsychological tests was administered within three weeks of the neuroimaging examinations in the Research Psychometrics Resource Laboratory within the Division of Neurocognitive Disorders in the Department of Psychiatry and Psychology at Mayo Clinic. Cognitive performance was investigated in four domains: 1) learning and memory (California Verbal Learning Test, New York University Paragraphs, and Benton Visual Retention Test); 2) auditory attention and working memory (Wechsler Memory Scale-III Letter-Number Sequencing and Digit Span subtests); 3) visual attention and perceptual speed (Trail Making Test part A, Color and Word trials of the Stroop test, and Wechsler Adult Intelligence Scale-III Digit Symbol Coding subtest); and 4) speeded language/fluency (phonemic [F, A, S] and category [animals, fruits, vegetables]) and flexibility (Trail Making Test part B, and Color-Word Interference Trial of the Stroop). The individual test scores were summarized within general domains representing global cognitive function as previously described [26].
Blood collection and analysis
Women were asked to refrain from aspirin two weeks prior to blood collection. Fasting venous blood was collected in tubes containing a mixture of thrombin inhibitor (1μM hirudin) plus coagulation factor Xa inhibitor (10μM soybean trypsin inhibitor) within a week of the brain imaging as previously described [27, 28]. Total cholesterol, low-density lipoprotein cholesterol (LDL-C), and high-density lipoprotein cholesterol (HDL-C), triglycerides, blood glucose and 17β-estradiol were measured by the Mayo Clinic Department of Laboratory Medicine and Pathology (Rochester, MN). Apolipoprotein E (APOE) genotyping was performed after randomization [26].
Blood-borne microvesicles were isolated from platelet-free plasma by high-speed centrifugation (20,000 g for 30 min) and characterized by digital flow cytometer using fluorophore conjugated antibodies directed toward cells of the neurovascular unit (Table 1): total tau, amyloid-β 1–42 (Aβ1–42), neuron specific class III β-tubulin (Tuj1), microglia ionized calcium-binding adaptor molecule-1(Iab-1), glial fibrillary acid protein (GFAP), and low density lipoprotein receptor-related protein1 (LRP1) as described previously [28].
Table 1
Fluorophore conjugated antibodies used in the analyses of microvesicles in plasma collected from postmenopausal women
Markers used for the cells of neurovascular unit |
Alzheimer Disease: Total tau Amyloid-β 1–42 (Aβ1–42) |
Cerebrovascular endothelial cells: Low density lipoprotein receptor-related protein 1(LRP1), Amyloid-β clearance receptor |
Astrocytes: Glial fibrillary acidic protein (GFAP) |
Microglia: Ionized calcium-binding adaptor molecule 1 (Iba1) |
Neurons: Neuron specific class III beta-tubulin (Tuj-1) |
Statistical analysis
Continuous variables were described with medians and interquartile ranges (IQR). Differences among groups defined by previous hormone treatment were assessed using the proportional odds (PO) ordinal logistic model applied to the ranked versions of the continuous variables. Its two degree-of-freedom likelihood ratio test for any group difference provides a generalization of the Kruskal-Wallis test but also provides a more consistent framework for pairwise treatment comparisons, as derived by Wald tests on the individual parameter estimates. To limit multiple comparisons, pairwise group contrasts were performed only when the overall test of association for treatment was significant. The marginal relationship of each individual blood-borne biomarker with the PET SUVr measure of amyloid-β deposition was examined using Spearman’s rank correlation analyses. Because the sample size was insufficient to support a multivariable analysis of these relationships, data reduction was performed via principal components (PC) analysis with the goal of reducing the dimensionality of the six brain cellular markers to the fewest number of PCs that could explain the majority of its variation. Multivariable PO regression analysis was then used to regress amyloid-β load on the selected PCs. A joint test of the association of all PCs with amyloid-β was constructed using a multiple degree-of-freedom likelihood ratio χ2 test (LRT). Partial tests for association were reported on each individual PC only when the PCs were statistically significant as a group. The PC-based regression analysis was then repeated for secondary outcomes, including white matter hyperintensity, hippocampal volume, and global cognitive score.
RESULTS
Amyloid PET imaging was available in 67 women from the follow-up KEEPS study conducted three years after completion of the trial; median (IQR) age at time of imaging was 60.4 (58.7, 61.7) years. Phenotypic and clinical characteristics of these women are shown in Table 2. As reported previously for the study sample that included one additional participant, these characteristics were mostly similar among the previous hormone treatment groups. The exceptions were increased presence of the APOE ɛ4 variant and decreased levels of amyloid-β load, after controlling for age, in women assigned to the tE2 group. [22]
Table 2
Baseline phenotypic and clinical characteristics of the study population
Characteristic | N | Total (n = 67) |
Age at follow-up study, y | 67 | 60.4 (58.7, 61.8) |
Time since menopause at follow-up study, y | 67 | 8.4 (7.9, 9.2) |
Education: | 67 | |
High school or less | 7 (10.4%) | |
Some college/technical/vocational school | 27 (40.3%) | |
College graduate or higher | 33 (49.3%) | |
Smoking status: | 67 | |
Never | 46 (68.7%) | |
Former | 19 (28.4%) | |
Current | 2 (3.0%) | |
Body mass index, kg/m2 | 67 | 27.4 (24.1, 31.4) |
Waist circumference, cm | 67 | 89.0 (80.5, 97.8) |
Systolic blood pressure, mm Hg | 67 | 127.3 (116.3, 135.3) |
Diastolic blood pressure, mm Hg | 67 | 77.3 (72.3, 82.0) |
Fasting glucose, mg/dL | 66 | 94.0 (90.0, 100.0) |
Total cholesterol, mg/dL | 66 | 204.5 (189.0, 227.0) |
HDL (high density lipoprotein) cholesterol, mg/dL | 66 | 62.0 (54.0, 73.0) |
LDL (low density lipoprotein) cholesterol, mg/dL | 66 | 120.6 (106.2, 139.2) |
Triglycerides, mg/dL | 66 | 92.5 (71.0, 115.0) |
hs-C reactive protein, mg/dL | 66 | 0.14 (0.07, 0.30) |
APOE ɛ4 carrier, n (%) | 67 | 18 (26.9%) |
Results are reported as median (25th, 75th percentile), except for education, smoking status and APOE ɛ4 which are reported as counts (percentages). N is the number of non-missing values.
The total number of amyloid-β associated MV in peripheral blood was significantly different across treatment groups (overall p = 0.032), with lower levels in women who were treated with tE2 compared with placebo (p = 0.009; Table 3, Fig. 1). There were no differences in the concentration of blood-borne MV from blood-brain barrier endothelium, neurons, and microglia across hormone treatment groups (Table 3, Fig. 1). Of the six biomarker measures, only the number of astrocyte-derived MV (GFAP) showed a nominally significant correlation with PiB SUVr in unadjusted analysis (Spearman rs = 0.27, p = 0.026). Overall distributions of amyloid-β load, as measured by PiB SUVr, and other imaging and cognitive measures are illustrated in Fig. 2. Cognitive assessments were available for 62 of the 67 women, of whom global cognitive scores were comparable across treatment groups (median [IQR]: 0.30 [–2.24, 1.45] in PL; 0.45 [–1.77, 2.29] in tE2 and –0.87 [–2.04, 1.76] in oCEE; p = 0.908).
Table 3
Peripheral blood microvesicles positive for amyloid-β, tau, and antigens for cells of neurovascular unit, by prior treatment group
Variable | Comparisons by Treatment | |||
PL (n = 29) | tE2 (n = 21) | oCEE (n = 17) | p | |
Aβ1–42 | 6.06 (2.11, 12.55) | 2.49 (0.73, 3.59)* | 4.96 (0.83, 10.31) | 0.032 |
Tau | 0.42 (0.19, 0.77) | 0.38 (0.20, 0.77) | 0.66 (0.20, 1.56) | 0.696 |
GFAP | 4.87 (3.30, 7.30) | 5.95 (4.83, 6.98) | 5.54 (3.11, 7.78) | 0.370 |
Tuj1 | 5.51 (3.75, 10.47) | 6.46 (5.03, 11.94) | 5.06 (4.07, 6.34) | 0.186 |
LRP1 | 2.08 (1.60, 6.44) | 3.36 (1.34, 6.05) | 4.67 (2.88, 9.12) | 0.292 |
Iba1 | 0.19 (0.10, 0.32) | 0.13 (0.07, 0.20) | 0.19 (0.10, 0.96) | 0.474 |
Results are reported as median (25th, 75th percentile). Likelihood ratio tests from proportional odds ordinal logistic semiparametric models were used to identify any between-group differences. When significant, pairwise treatment comparisons were additionally assessed using Wald tests on individual parameter estimates. *Pairwise treatment comparison versus placebo was statistically significant (p < 0.05). PL, placebo; tE2, transdermal 17β-estradiol; CEE, oral conjugated equine estrogen; for definitions of the biomarker variables, refer to Table 1.
Fig. 1
Distribution of peripheral blood microvesicles positive for markers for cells of the neurovascular unit, and amyloid-β and tau by treatment group.
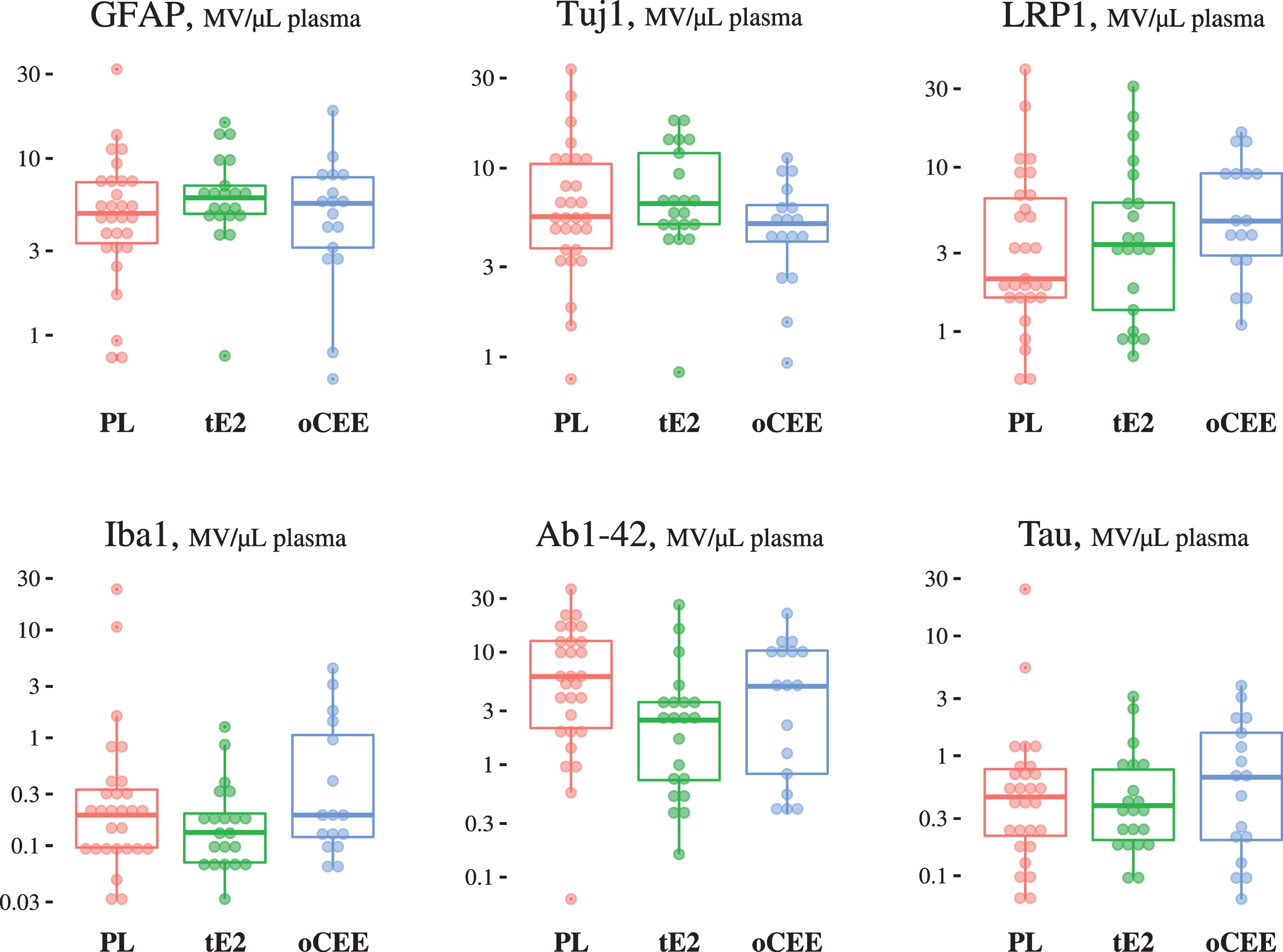
Fig. 2
Data distribution of amyloid-β load, white matter hyperintensity, hippocampal volume, and global cognitive score.
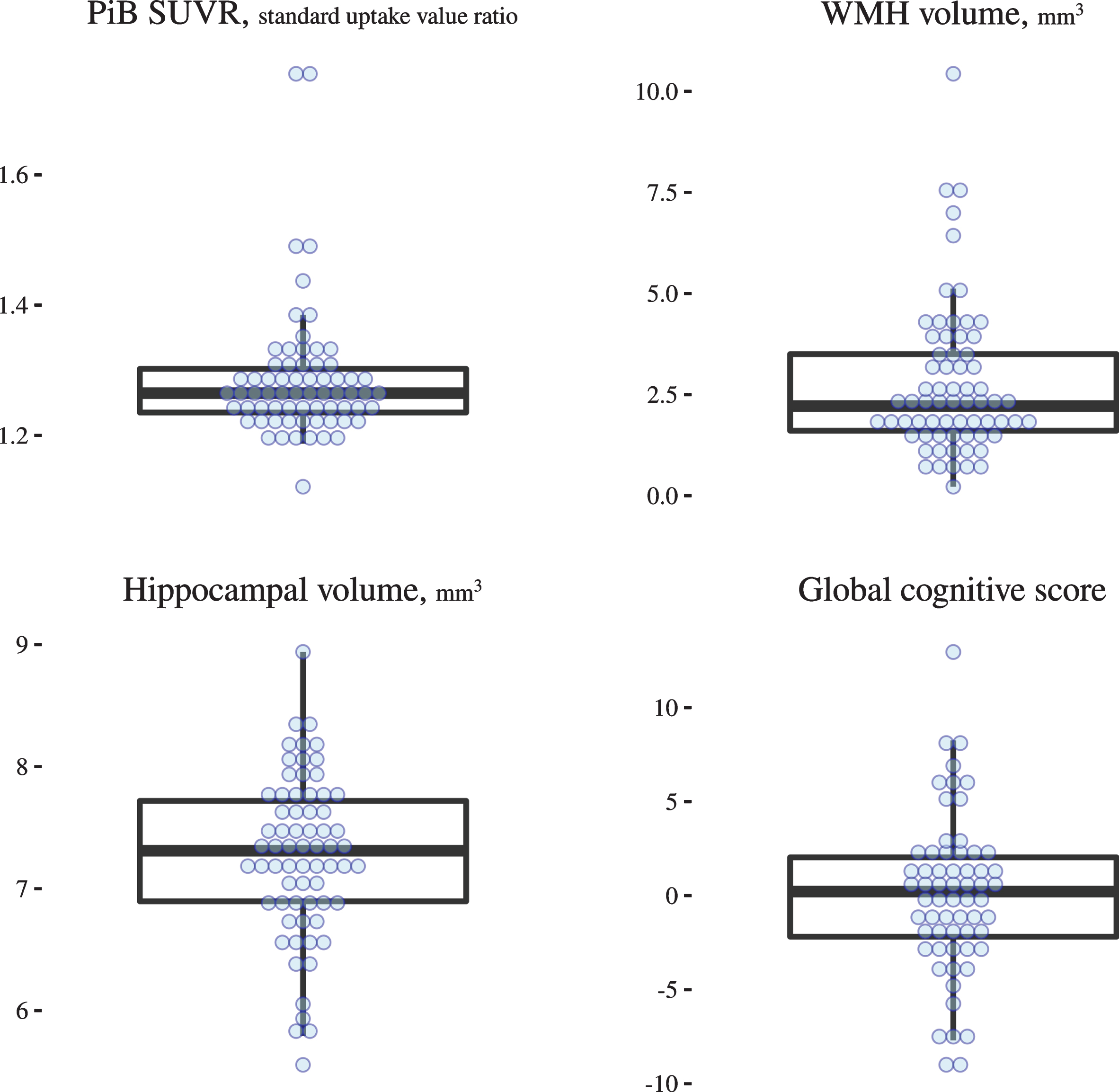
Principal components (PC) analysis was used to reduce the dimensionality of the six biomarkers to two PCs: the first PC is a contrast between microglial-positive MV and an average of astrocyte- and neuron-derived MV, while the second PC is a weighted average of all brain cellular markers except astrocyte-derived MV (Table 4). Together these first two principal components explain 58% of the total variation in the six original biomarkers. In a regression analysis, the joint test for association of the two PCs with the level of PiB SUVr was statistically significant (Global p = 0.045; Table 5). Partial tests revealed significant association of the first PC (p = 0.034; Table 5), but not the second PC (p = 0.143; Table 5), with PiB SUVr. There was no joint significant effect of the PCs on the secondary outcomes of white matter hyperintensities, hippocampal volume or global cognitive score (Table 5).
Table 4
Principal components of the six markers of the neurovascular unit scoring of individual PCs
Microvesicle Measure | PC#1 | PC#2 |
Astrocyte-derived (GFAP) | 0.68 | 0.07 |
Neuron-derived (Tuj1) | 0.59 | 0.41 |
Amyloid-β clearance receptor (LRP1) | –0.08 | 0.42 |
Microglia-derived (Iba-1) | –0.40 | 0.41 |
Amyloid-β positive (Aβ1–42) | –0.14 | 0.26 |
Tau positive (tau) | –0.08 | 0.65 |
Proportion | 31.8% | 26.0% |
Cumulative | 31.8% | 57.8% |
Principal components analysis was used to reduce the dimensionality of the 6 brain markers to 2 principal components (PC). Based on the loadings above, the first PC is basically a contrast between amyloid-β positive and an average of astrocyte- and neuron-derived, while the second PC is a weighted average of all brain markers except astrocyte-derived MV. Together these first 2 PCs explain 58% of the variation in the original 6 markers.
Table 5
Joint and partial effects of the 2 principal components on amyloid-β load
Dependent Variable‡ | Independent Variable | Test of Association | |
Amyloid-β load | PCs #1-2 | Global | χ2= 6.2, 2 df, p = 0.045 |
PC #1 | Partial | χ2 = 4.5, 1 df, p = 0.034 | |
PC #2 | Partial | χ2 = 2.1, 1 df, p = 0.143 | |
White matter hyperintensities | PCs #1-2 | Global | χ2= 1.6, 2 df, p = 0.443 |
Hippocampal volume | PCs #1-2 | Global | χ2= 3.0, 2 df, p = 0.218 |
Global cognition score | PCs #1-2 | Global | χ2= 0.5, 2 df, p = 0.785 |
‡Proportional odds ordinal logistic regression was used to separately predict each dependent variable using the 2 principal components. The global test of association for both PCs jointly are from a likelihood ratio χ2 test with 2 degrees of freedom (df), while partial tests of association for each PC (reported only when global test was significant) are from Wald χ2 tests with 1 df.
DISCUSSION
This study provides proof of concept that MV-carrying surface amyloid-β (a hallmark AD pathology) and MV-derived from cells of the neurovascular unit can be detected in peripheral blood. When analyzed separately, only MV derived from astrocytes was univariately associated with amyloid PET among these postmenopausal women. Collectively, after reducing the six biomarkers to two principal components, both the global and partial tests of these principal components were marginally associated with PiB SUVr, suggesting a potential relationship. Although the association of astrocyte-derived MV with amyloid PET was relatively weak (rs = 0.27), their contribution to the weighted averages in the PC analysis supports their contribution in the neurovascular unit to the overall relationship with amyloid-β. It is possible that changes in the neurovascular unit represent early pathological processes associated with amyloid-β pathology of AD that may precede functional changes in cognitively unimpaired women. The global association of the markers of the neurovascular unit with amyloid-β on PET load supports the functional contribution of the cells of the neurovascular unit to development of AD pathology.
The detection of markers of activated astroglia and neurons by way of shed MV into peripheral blood is consistent with the “two-hit vascular hypothesis” of AD that proposes that brain microvascular damage is an initial insult through which dysfunction of the blood-brain barrier or hypoperfusion of the brain leads to secondary neuronal injury and primes the brain for accumulation of amyloid-β [29]. Indeed, neurovascular injury can initiate a cascade of molecular and cellular events leading to neurodegeneration, cognitive impairment, and AD dementia [30]. The vascular remodeling and inflammatory responses of astrocytes and microglia to the leakage through the blood-brain barrier of neurotoxic proteins could promote neuronal injury by forming or exacerbating neurofibrillary tangles, white matter damage, decreased dendritic spine density, and amyloid-β accumulation [29]. This vascular remodeling seems to be specific for amyloid-β accumulation, as other components of the neurovascular unit did not associate with white matter hyperintensities, or hippocampal volumes, and seems to precede changes in cognition, as the association of the PC with cognitive tests scores was not significant.
While promising, confirmation of these results using a larger and more diverse cohort or in a longitudinal assessment of women of the KEEPS will be required in order to develop these markers into a simple blood test to identify persons at risk for amyloid-β deposition in the brain. The methodology to isolate MV used in this study has been standardized and validated in our laboratory [28]. However, consensus for collection techniques to reduce the impact of preclinical variables for larger studies of diverse groups of men and women is needed [20, 31]. To correct the current data set for other phenotypic variables of participants would not provide useful information as multivariable models require the sample size to be sufficiently large to support the number of predictor variables with accurate and reliable estimates. Furthermore, to understand the contribution of various other phenotypic characteristics on development of MV and the rate of accumulation of amyloid-β requires longitudinal assessments as previously noted, for example, the association of thrombogenic MV associated with development of white matter hyperintensities over a period of four years [32]. Unfortunately, assessments of MV derived from the neurovascular unit were not part of the data set collected at baseline in KEEPS. Other future studies could consider other markers of capillary integrity such as mural cells, including pericytes and vascular smooth muscle cells.
ACKNOWLEDGMENTS
This study was funded by grants from the NIH R21 NS66147 and RF1 AG57547 to Kejal Kantarci, HL90639 and P50 AG44170 to Virginia M. Miller, the Aurora Foundation to the Kronos Longevity Research Institute, UL1 RR0241501, and the Mayo Foundation. 1From the National Center for Research Resources (NCRR), a component of the National Institutes of Health (NIH), and the NIH Roadmap for Medical Research. Contents of this paper are solely the responsibility of the authors and do not necessarily represent the official view of NCRR or NIH.
Authors’ disclosures available online (https://www.j-alz.com/manuscript-disclosures/20-1410r1).
REFERENCES
[1] | Dage JL , Wennberg AMV , Airey DC , Hagen CE , Knopman DS , Machulda MM , Roberts RO , Jack CR Jr. , Petersen RC , Mielke MM ((2016) ) Levels of tau protein in plasma are associated with neurodegeneration and cognitive function in a population-based elderly cohort. Alzheimers Dement 12: , 1226–1234. |
[2] | Mielke MM , Hagen CE , Wennberg AMV , Airey DC , Savica R , Knopman DS , Machulda MM , Roberts RO , Jack CR Jr. , Petersen RC , Dage JL ((2017) ) Association of plasma total tau level with cognitive decline and risk of mild cognitive impairment or dementia in the Mayo Clinic Study on Aging. JAMA Neurol 74: , 1073–1080. |
[3] | Ovod V , Ramsey KN , Mawuenyega KG , Bollinger JG , Hicks T , Schneider T , Sullivan M , Paumier K , Holtzman DM , Morris JC , Benzinger T , Fagan AM , Patterson BW , Bateman RJ ((2017) ) Amyloid beta concentrations and stable isotope labeling kinetics of human plasma specific to central nervous system amyloidosis. Alzheimers Dement 13: , 841–849. |
[4] | Mielke MM , Hagen CE , Xu J , Chai X , Vemuri P , Lowe VJ , Airey DC , Knopman DS , Roberts RO , Machulda MM , Jack CR Jr. , Petersen RC , Dage JL ((2018) ) Plasma phospho-tau181 increases with Alzheimer’s disease clinical severity and is associated with tau- and amyloid-positron emission tomography. Alzheimers Dement 14: , 989–997. |
[5] | Nakamura A , Kaneko N , Villemagne VL , Kato T , Doecke J , Dore V , Fowler C , Li QX , Martins R , Rowe C , Tomita T , Matsuzaki K , Ishii K , Ishii K , Arahata Y , Iwamoto S , Ito K , Tanaka K , Masters CL , Yanagisawa K ((2018) ) High performance plasma amyloid-beta biomarkers for Alzheimer’s disease. Nature 554: , 249–254. |
[6] | Vergallo A , Megret L , Lista S , Cavedo E , Zetterberg H , Blennow K , Vanmechelen E , De Vos A , Habert MO , Potier MC , Dubois B , Neri C , Hampel H , group IN-ps, Alzheimer Precision Medicine I ((2019) ) Plasma amyloid beta 40/42 ratio predicts cerebral amyloidosis in cognitively normal individuals at risk for Alzheimer’s disease. Alzheimers Dement 15: , 764–775. |
[7] | Karikari TK , Pascoal TA , Ashton NJ , Janelidze S , Benedet AL , Rodriguez JL , Chamoun M , Savard M , Kang MS , Therriault J , Scholl M , Massarweh G , Soucy JP , Hoglund K , Brinkmalm G , Mattsson N , Palmqvist S , Gauthier S , Stomrud E , Zetterberg H , Hansson O , Rosa-Neto P , Blennow K ((2020) ) Blood phosphorylated tau 181 as a biomarker for Alzheimer’s disease: A diagnostic performance and prediction modelling study using data from four prospective cohorts. Lancet Neurol 19: , 422–433. |
[8] | Thijssen EH , La Joie R , Wolf A , Strom A , Wang P , Iaccarino L , Bourakova V , Cobigo Y , Heuer H , Spina S , VandeVrede L , Chai X , Proctor NK , Airey DC , Shcherbinin S , Duggan Evans C , Sims JR , Zetterberg H , Blennow K , Karydas AM , Teunissen CE , Kramer JH , Grinberg LT , Seeley WW , Rosen H , Boeve BF , Miller BL , Rabinovici GD , Dage JL , Rojas JC , Boxer AL , Advancing Research and Treatment for Frontotemporal Lobar Degeneration (ARTFL) investigators ((2020) ) Diagnostic value of plasma phosphorylated tau181 in Alzheimer’s disease and frontotemporal lobar degeneration. Nat Med 26: , 387–397. |
[9] | Humpel C , Marksteiner J ((2005) ) Cerebrovascular damage as a cause for Alzheimer’s disease. Curr Neurovasc Res 2: , 341–347. |
[10] | Bushnell CD , Hurn P , Colton C , Miller VM , del Zoppo G , Elkind MS , Stern B , Herrington D , Ford-Lynch G , Gorelick P , James A , Brown CM , Choi E , Bray P , Newby LK , Goldstein LB , Simpkins J ((2006) ) Advancing the study of stroke in women: Summary and recommendations for future research from an NINDS-Sponsored Multidisciplinary Working Group. Stroke 37: , 2387–2399. |
[11] | del Zoppo GJ , Milner R ((2006) ) Integrin-matrix interactions in the cerebral microvasculature. Arterioscler Thromb Vasc Biol 26: , 1966–1975. |
[12] | Popa-Wagner A , Buga AM , Popescu B , Muresanu D ((2015) ) Vascular cognitive impairment, dementia, aging and energy demand. A vicious cycle.S. J Neural Transm (Vienna) 122: (Suppl 1), 47–54. |
[13] | Gorelick PB , Scuteri A , Black SE , Decarli C , Greenberg SM , Iadecola C , Launer LJ , Laurent S , Lopez OL , Nyenhuis D , Petersen RC , Schneider JA , Tzourio C , Arnett DK , Bennett DA , Chui HC , Higashida RT , Lindquist R , Nilsson PM , Roman GC , Sellke FW , Seshadri S ((2011) ) Vascular contributions to cognitive impairment and dementia: A statement for healthcare professionals from the american heart association/american stroke association. Stroke 42: , 2672–2713. |
[14] | Geraldes R , Esiri MM , DeLuca GC , Palace J ((2017) ) Age-related small vessel disease: A potential contributor to neurodegeneration in multiple sclerosis. Brain Pathol 27: , 707–722. |
[15] | Ungvari Z , Tarantini S , Hertelendy P , Valcarcel-Ares MN , Fulop GA , Logan S , Kiss T , Farkas E , Csiszar A , Yabluchanskiy A ((2017) ) Cerebromicrovascular dysfunction predicts cognitive decline and gait abnormalities in a mouse model of whole brain irradiation-induced accelerated brain senescence. Geroscience 39: , 33–42. |
[16] | Joshi P , Benussi L , Furlan R , Ghidoni R , Verderio C ((2015) ) Extracellular vesicles in Alzheimer’s disease: Friends or foes? Focus on abeta-vesicle interaction. Int J Mol Sci 16: , 4800–4813. |
[17] | Coleman BM , Hill AF ((2015) ) Extracellular vesicles–Their role in the packaging and spread of misfolded proteins associated with neurodegenerative diseases. Semin Cell Dev Biol 40: , 89–96. |
[18] | Porro C , Trotta T , Panaro MA ((2015) ) Microvesicles in the brain: Biomarker, messenger or mediator? J Neuroimmunol 288: , 70–78. |
[19] | Goetzl EJ , Boxer A , Schwartz JB , Abner EL , Petersen RC , Miller BL , Kapogiannis D ((2015) ) Altered lysosomal proteins in neural-derived plasma exosomes in preclinical Alzheimer disease. Neurology 85: , 40–47. |
[20] | Badhwar A , Haqqani AS ((2020) ) Biomarker potential of brain-secreted extracellular vesicles in blood in Alzheimer’s disease. Alzheimers Dement (Amst) 12: , e12001. |
[21] | Rajendran L , Bali J , Barr MM , Court FA , Kramer-Albers EM , Picou F , Raposo G , van der Vos KE , van Niel G , Wang J , Breakefield XO ((2014) ) Emerging roles of extracellular vesicles in the nervous system. J Neurosci 34: , 15482–15489. |
[22] | Kantarci K , Lowe VJ , Lesnick TG , Tosakulwong N , Bailey KR , Fields JA , Shuster LT , Zuk SM , Senjem ML , Mielke MM , Gleason C , Jack CR , Rocca WA , Miller VM ((2016) ) Early postmenopausal transdermal 17beta-estradiol therapy and amyloid-beta deposition. J Alzheimers Dis 53: , 547–556. |
[23] | Harman SM , Brinton EA , Cedars M , Lobo R , Manson JE , Merriam GR , Miller VM , Naftolin F , Santoro N ((2005) ) KEEPS: The Kronos Early Estrogen Prevention Study. Climacteric 8: , 3–12. |
[24] | Jack CR Jr. , Lowe VJ , Senjem ML , Weigand SD , Kemp BJ , Shiung MM , Knopman DS , Boeve BF , Klunk WE , Mathis CA , Petersen RC ((2008) ) 11C PiB and structural MRI provide complementary information in imaging of Alzheimer’s disease and amnestic mild cognitive impairment. Brain 131: , 665–680. |
[25] | Schwarz C , JL G , Ward C , Vemuri P , Senjem M , Wiste H , Peterson R , Knopman D , Jack C ((2017) ) The Mayo Clinic Adult Lfespan Template: Better quantification across the lifespan. Alzheimers Dement 13: , P792. |
[26] | Gleason CE , Dowling NM , Wharton W , Manson JE , Miller VM , Atwood CS , Brinton EA , Cedars MI , Lobo RA , Merriam GR , Neal-Perry G , Santoro NF , Taylor HS , Black DM , Budoff MJ , Hodis HN , Naftolin F , Harman SM , Asthana S ((2015) ) Effects of hormone therapy on cognition and mood in recently postmenopausal women: Findings from the randomized, controlled KEEPS-Cognitive and Affective Study. PLoS Med 12: , e1001833. |
[27] | Jayachandran M , Litwiller RD , Lahr BD , Bailey KR , Owen WG , Mulvagh SL , Heit JA , Hodis HN , Harman SM , Miller VM ((2011) ) Alterations in platelet function and cell-derived microvesicles in recently menopausal women: Relationship to metabolic syndrome and atherogenic risk. J Cardiovasc Transl Res 4: , 811–822. |
[28] | Jayachandran M , Miller VM , Heit JA , Owen WG ((2012) ) Methodology for isolation, identification and characterization of microvesicles in peripheral blood. J Immunol Methods 375: , 207–214. |
[29] | Zlokovic BV ((2011) ) Neurovascular pathways to neurodegeneration in Alzheimer’s disease and other disorders. Nat Rev Neurosci 12: , 723–738. |
[30] | Nelson AR , Sweeney MD , Sagare AP , Zlokovic BV ((2016) ) Neurovascular dysfunction and neurodegeneration in dementia and Alzheimer’s disease. Biochim Biophys Acta 1862: , 887–900. |
[31] | Hansson O , Mikulskis A , Fagan AM , Teunissen C , Zetterberg H , Vanderstichele H , Molinuevo JL , Shaw LM , Vandijck M , Verbeek MM , Savage M , Mattsson N , Lewczuk P , Batrla R , Rutz S , Dean RA , Blennow K ((2018) ) The impact of preanalytical variables on measuring cerebrospinal fluid biomarkers for Alzheimer’s disease diagnosis: A review. Alzheimers Dement 14: , 1313–1333. |
[32] | Raz L , Jayachandran M , Tosakulwong N , Lesnick TG , Wille SM , Murphy MC , Senjem ML , Gunter JL , Vemuri P , Jack CR Jr. , Miller VM , Kantarci K ((2013) ) Thrombogenic microvesicles and white matter hyperintensities in postmenopausal women. Neurology 80: , 911–918. |