Sexual Dimorphism in the 3xTg-AD Mouse Model and Its Impact on Pre-Clinical Research
Abstract
Female sex is a leading risk factor for developing Alzheimer’s disease (AD). Sexual dimorphism in AD is gaining attention as clinical data show that women are not only more likely to develop AD but also to experience worse pathology and faster cognitive decline. Pre-clinical AD research in animal models often neglects to address sexual dimorphism in evaluation of behavioral or molecular characteristics and outcomes. This can compromise its translation to a clinical setting. The triple-transgenic AD mouse model (3xTg-AD) is a commonly used but unique AD model because it exhibits both amyloid and tau pathology, essential features of the human AD phenotype. Mounting evidence has revealed important sexually dimorphic characteristics of this animal model that have yet to be reviewed and thus, are often overlooked in studies using the 3xTg-AD model. In this review we conduct a thorough analysis of reports of sexual dimorphism in the 3xTg-AD model including findings of molecular, behavioral, and longevity-related sex differences in original research articles through August 2020. Importantly, we find results to be inconsistent, and that strain source and differing methodologies are major contributors to lack of consensus regarding traits of each sex. We first touch on the nature of sexual dimorphism in clinical AD, followed by a brief summary of sexual dimorphism in other major AD murine models before discussing the 3xTg-AD model in depth. We conclude by offering four suggestions to help unify pre-clinical mouse model AD research inspired by the NIH expectations for considering sex as a biological variable.
INTRODUCTION
Sexual dimorphism has recently come into focus in the Alzheimer’s disease (AD) community as clinical data show that women are not only more likely to develop AD but also to experience more rapid cognitive decline [1–3]. This finding is even more pronounced in those who are carriers of the ɛ4 allele of apolipoprotein E (APOE4), the strongest and most prevalent known genetic risk factor for AD [4, 5]. Reasoning behind sexual dimorphism in AD suggests that it is due to intrinsic risk factors like genetics, sex hormones, and differing inflammatory responses, in addition to the longer life expectancy of females [1]. Moreover, females are documented to respond better than males to two acetylcholinesterase inhibitors (donepezil and rivastigmine), 2 out of 4 of the only currently FDA-approved drugs for treatment of AD symptoms [6, 7]. Despite this evidence of sex-ual dimorphism in AD, there is a lack of sex-stratified data from clinical trials of AD as well as in pre-clin-ical and basic research using animal models [7–9].
Scrutiny over the utility of animal models for therapeutic discovery in AD has intensified over the last decade [10, 11]. One animal model, the triple transgenic AD (3xTg-AD) mouse, has been widely used for almost 20 years because it is one of the only two mouse models in which interventions against both human amyloid and tau pathology can be studied simultaneously [12]. The 3xTg-AD mouse overexpresses three human transgenes throughout the central nervous system th-at contribute to the AD-like phenotype of this model. Two of these transgenes cause early-onset AD in pa-tients, namely the amyloid precursor protein with the Swedish familial double mutation (APPKM670/671NL) and the presenilin 1 with a substitution mutation (PS1M146V). Together, these mutations accelerate production of pathological Aβ and deposition into amyloid plaques [12, 13]. The third transgene is mic-rotubule associated protein tau (MAPT) with a P301L mutation that causes increased aggregation of hyperphosphorylated tau into neurofibrillary tau tangles [14]. While this is the major AD mouse model in which both human amyloid and tau pathology can be studied simultaneously, it is important to note that the MAPT mutation is a familial determinant for ear-ly-onset frontotemporal dementia [15]. We have sum-marized the evidence confirming that male and female 3xTg-AD mice are sexually dimorphic in both the presentation of AD molecular pathology and in behavioral studies examining short-term recognition and spatial memory. Surprisingly, we could not identify any well-powered studies that compared total transgene expression levels between sexes.
Both the amyloid and tau pathologies are required for a confirmed diagnosis of AD; however, they are not the only factors that contribute to neurodegeneration and memory loss; neuroinflammation, cell death, and widespread metabolic changes are concomitant [16, 17]. Concerns regarding the consistency of the data obtained from this animal model have recently arisen and have yet to be reviewed, including colony drift and considerable sexually dimorphic presentation of AD pathology and cognitive deficits [18, 19]. Understanding sexually dimorphic responses to preclinical therapeutic interventions will elucidate mechanisms of both the disease and the interventions. Despite the noted sexual dimorphism of this mouse model, there are a limited number of studies that use both sexes (Fig. 1), and even fewer that re-port results separately by sex or directly compared results between males and females. Preclinical animal models should be considered on the basis of their ability to recapitulate both the universal hallmarks of AD as well as the sexually dimorphic nature of AD [20]. In this review, we evaluate the literature regarding sexual dimorphism in molecular and behavioral presentations of disease progression in the 3xTg-AD mouse model along with sexually dimorphic responses to preclinical therapeutic interventions.
Fig. 1
Graph of 613 original publications using 3xTg-AD mice by sex over time.
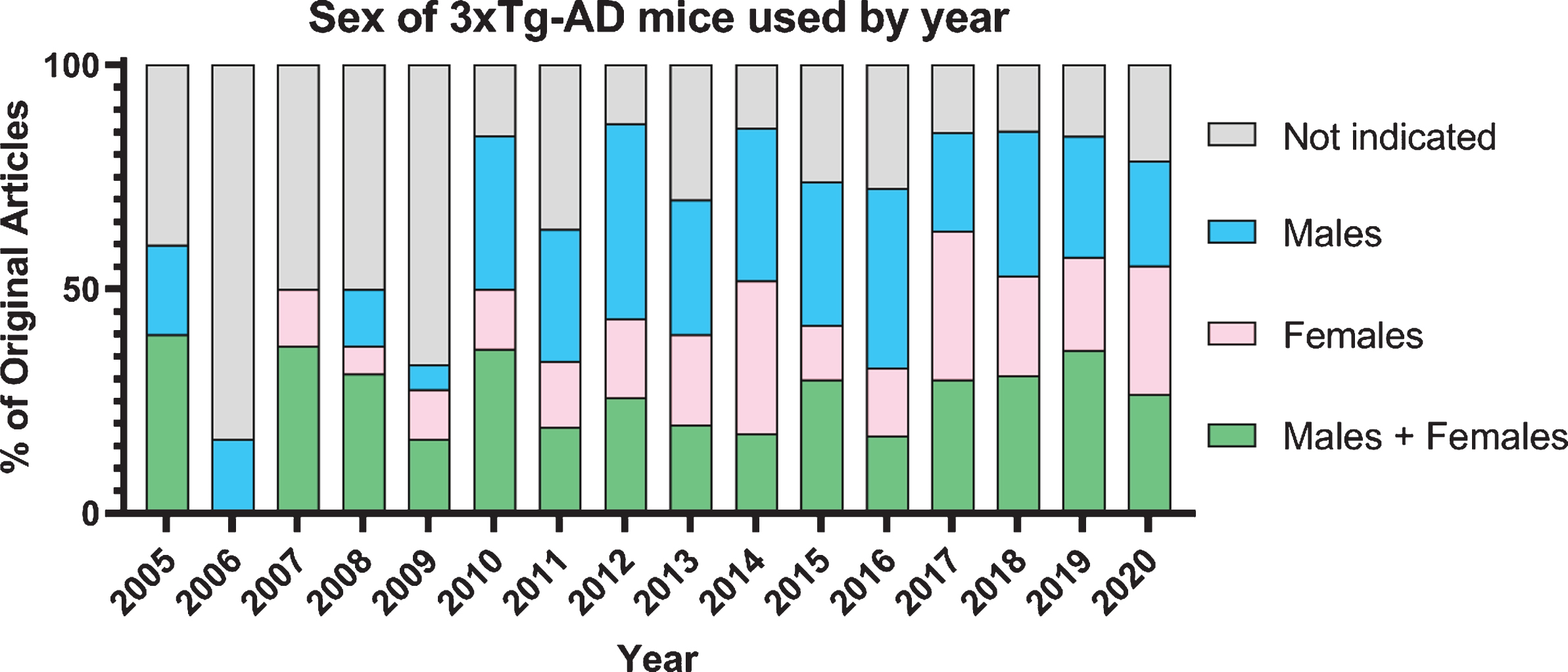
SEXUAL DIMORPHISM IN THE CLINICAL PRESENTATION OF ALZHEIMER’S DISEASE PATIENTS
Although the mechanisms behind sexual dimorp-hism in AD are the subject of ongoing investigations, it has been well-documented that AD disproportionately affects women, at a ratio of approximately two to one [21]. One explanation for the higher prevalence in females may be the link to APOE4, an allele variant believed to affect mechanisms associated with clearance of amyloid-β (Aβ), hyperphosphorylation of tau, microglial activation, and synaptic plasticity. APOE4 is strongly correlated to risk for sporadic and late-onset familial AD, and this correlation is more prominent in women than men [4]. Studies in human subjects have shown that female APOE4 carriers have worse performance in memory tasks and more rapid cognitive decline than men, even in non-AD cohorts [22]. It has also been suggested that APOE4 allele carriers respond differently with respect to safety and efficacy in clinical trials [23]. Clinically, sex differences in Aβ plaque burden are still being studied, with some trials reporting an increase in women [24, 25], while others have found no sex differences in Aβ deposition [26]. Studies comparing tau between males and females are also finding a greater burden in women. Oveisgharan et al. analyzed postmortem brains from AD patients and report significantly higher densities of tau tangles in women [27]. Another study, in a cohort of individuals at risk of developing AD based on Aβ load, tau deposits (as measured by positron emission tomography; PET) in the entorhinal cortex appeared earlier in women compared to men, further supporting the notion that sex influences AD risk [28]. Taken together, this recent literature points towards a trend of worse pathology in women.
Behind these discrepancies between sexes in AD pathology are several inherently sex-based differences including sex hormones, epigenetics, immune response, and lifespan. One hypothesis for the higher prevalence of AD in women lies in the drastic changes in sex hormones women experience as they traverse menopause, resulting in a depletion of estrogen post-menopause [29]. In addition to the reported neuroprotective aspects of estrogen [30], female estrogen and male androgen act upon gene expression through epigenetic modifications such as methylation and acetylation [31]. These sex hormone-based epigenetic effects may influence susceptibility to certain disease or possibly response to drug therapies. However, their impact in AD pathology remains largely elusive.
Studies have shown significant differences in immune system activation between the sexes, specifically in innate and adaptive immunity [32]. Importantly, immune response has been implicated as a critical factor in AD development, with inflammatory cytokines, chemokines, and gliosis increased in AD patients [33]. Autoimmunity differs between sexes in clinical presentation and prevalence, with women representing almost 80% of all known cases of autoimmunity in the US [34]. Furthermore, men and women show different responses to vaccines, as measured by antibody response [35]. For example, females, compared to males, had a better humoral response to influenza vaccines and hepatitis vaccines, but a worse response to vaccines for measles and yellow fever. The mechanisms behind these sex differences are still under investigation but are thought to be a combination of differences in genetics and gonadal hormones. This is of interest to AD research as vaccines against Aβ are under investigation in clinical trials [36, 37].
Lifespan is also a confounding variable. Women, on average, live longer than men, increasing the probability of developing AD. However, longitudinal and epidemiological studies that have controlled for this have concluded a higher lifetime risk for women compared to men [38]. These factors are, of course, not mutually exclusive and influence each other significantly, which makes distinguishing a precise mechanism behind sexual dimorphism in AD unrealistic but makes sex-based stratifications all the more important.
Sex differences in clinical trial outcomes are often overlooked and underreported, making it difficult to determine how sex differences influence AD and possibly other neurodegenerative diseases. In fact, there was a strong male bias in clinical trials until it was required by law in 1993, that female enrollment must be included in phase III clinical trials [39]. There are widespread reports of differences in therapeutic response between sexes, yet stratification of results remains limited [40]. Among 54 clinical trials for the AD therapeutics cholinesterase inhibitors (donepezil, rivastigmine, and galantamine) and the N-methyl-D-aspartate receptor agonist memantine, 48 did not report data separately by sex [41]. This lack of stratification in clinical data may be problematic since sex differences have been reported in the Mini-Mental State Examination [42], verbal memory tests [43], cholinergic systems [44], and drug metabolism [45]. A similar lack of stratification is found in preclinical studies, as described below.
SEXUAL DIMORPHISM IN THE 3xTg-AD MOUSE MODEL
Overall, sex differences are underreported in pre-clinical AD research as most studies do not equally re-cruit animals from each sex, especially not in numbers large enough to reach statistical power [7]. Sexual dimorphism has been reported in many of the most commonly used AD mouse models, which employ various familial mutations in human amyloid-beta protein precursor (AβPP or APP) and presenilin 1 (PS1). Reports include increased Aβ plaque burden in females of the APP/PS1 [46–49], TASTPM [50], and Tg2576 [1, 51, 52] mouse models, and sex differences in tau phosphorylation in the P301L mouse [53, 54].
Sex differences in behavior have also been noted in various AD models including stress response in 5xFAD [55, 56], impaired reference memory in female Tg2576 [52], and impaired spatial learning in female TgCRND8 mice [57]. However, the 3xTg-AD mouse has been particularly noted for the mod-el’s variable sex differences, which have yet to be reviewed [19]. These sex differences in AD mouse models often do not correlate, and sometimes even contradict differences seen clinically, which could hinder the development of AD treatments if they are not accounted for.
Sexual dimorphism in AD pathology
Accumulation of Aβ in serum and brain tissue is reported to occur earlier and progress at an accelerated rate in female 3xTg-AD mice in the majority of studies that compare sex (Table 1) [58–70]. A significant difference in Aβ pathology in this mouse model proves problematic for the pre-clinical screening of Aβ-targeted therapies, since the age of onset and subsequent Aβ plaque accumulation both differ between males and females. The Mufson laboratory reported Aβ immunoreactive-plaque deposition in the subiculum of females at an age of 8-9 months and males at 11-12 months [59, 62]. Hebda-Bauer et al. saw AβPP/Aβ immunoreactivity in the hippocampus of both males and females at 3 months, yet also reported greater immunoreactivity in females [70]. Stimmell et al. and Yang et al. also found significantly greater Aβ (6E10) immunopositive-plaque deposition in the hippocampus of female mice at 6 months and 12 months, respectively [58, 71]. Creighton et al. report nearly 4-fold greater Aβ42 deposition in the hippocampus, as measured by ELISA, in female compared to male 3xTg-AD [66]. Omori et al. report Aβ42 levels approximately 20-fold higher in females than males using ELISA [69]. The LaFerla laboratory, which described the generation of the 3xTg-AD model, found no sex differences in whole brain Aβ40 and Aβ42 at 2, 6, 12, and 15 months of age [72]. We identified only one other study that measured cortical Aβ40 and Aβ42 at 6 months and observed no difference between sexes [73]. Notably, no study has reported a significantly greater amount in Aβ levels in male 3xTg-AD mice compared to females. Recently, Russo-Savage et al. measured greater levels of human APP in the somatosensory cortex of females at 3 months old using western blot [74]. However, this was only evaluated between a single mouse from each sex and no analysis was performed on AβPP processing enzymes or Aβ production.
Table 1
Summary of sex differences in molecular and behavioral studies using the 3xTg-AD model
Age (mo) | Molecular summary | Behavioral summary | Author | Year |
4, 12, 18 | F ↓ NLGN1 | F ↔ M performance in Y-maze, | Dufort-Gervais et al. [115] | 2020 |
SOR, and MWM | ||||
12 | F ↑ Aβ42 | M ↑ impairment in OR; | Creighton et al. [66] | 2019 |
F ↔ M impairment in OLM | ||||
6 | F ↑ Aβ | F ↑ impairment in spatial reorientation | Stimmell et al. [58] | 2019 |
12 | F ↑ Aβ and p-tau | F ↑ impairment in MWM | Yang et al. [71] | 2018 |
9 | F ↑ Aβ40 and Aβ42 | Omori et al. [69] | 2017 | |
12 and 18 | F ↑ Aβ40 and Aβ42 | Vandal et al. [68] | 2015 | |
2, 6, 12, 15 | M ↑ impairment in RAM | Stevens et al. [92] | 2015 | |
6 | M ↑ impairment in CFC; F ↔ M performance in NOR and Y-maze | Stover et al. [88] | 2015 | |
4 | F ↑ impairment in PM, | Cañete et al. [91] | 2015 | |
HB, DLB, TM, and ACT | ||||
12 and 15 | F ↑ impairment in MWM, M ↑ inhibition in CT and OF | Blazquez et al. [89] | 2014 | |
3-4 | F ↑ Aβ | Hebda-Bauer et al. [70] | 2013 | |
12 and 18 | F ↑ Aβ40 and Aβ42 | Bories et al. [67] | 2012 | |
2-3, 13–15, 18–20 | F ↑ Aβ | Perez et al. [59] | 2011 | |
6 | F ↑ Aβ40 and Aβ42 | Gimenez-Llort et al. [61] | 2010 | |
12 to 14 | F ↑ Aβ | F ↑ impairment in Y-maze | Carroll et al. [60] | 2010 |
8-9 and 18–20 | F ↑ Aβ and p-tau | Oh et al. [62] | 2010 | |
2, 3, 4, 6, 9, 12 | F ↑ Aβ | Rodriguez et al. [63] | 2008 | |
9, 16, 23 | F ↑ Aβ40 and Aβ42; | Hirata-Fukae et al. [64] | 2008 | |
F ↔ M tau | ||||
2, 4, 6, 9, 12, 15 | F ↔ M Aβ and p-tau | F ↔ M performance in MWM, | Clinton et al. [72] | 2007 |
IA, NOR at 2, 4, 12 months; | ||||
F ↑ impairment in MWM and IA at 6 and 9 months | ||||
10 | F ↑ Aβ; M ↑ tau | F ↔ M performance in MWM and OF | Nelson et al. [65] | 2007 |
↑= increase, ↓= decrease, ↔= no change or difference. F, female; M, male; Aβ, amyloid-β; p-tau, phosphorylated tau; NLGN1, Neuroligin-1; ACT, spontaneous circadian motor activity test; CFC, cued fear conditioning; CT, corner test; DLB, dark-light box; HB, Boissier’s hole-board test; IA, inhibitory avoidance; MWM, Morris water maze; NOR, novel object recognition; OF, open field; OLM, object location memory; PM, elevated plus maze; RAM, radial arm maze; SOR, spatial object recognition; TM, tunnel-maze.
Sexually dimorphic tau burden has received less attention and studies that have addressed it produced variable and inconclusive results [61, 62, 64, 65, 71]. Gimenez-Llort et al. did not report sex-based differences in PHF-tau immunostaining in hippocampus and amygdala [61]. Oh et al. on the other hand, used four different immunohistochemical stains for different tau residues (MC1, AT8, AT180, and PHF-1) and found greater tau staining in 18- to 20-month old females compared to males [62]. Additionally, Yang et al. report increased phospho-tau (T231) positive cells in the hippocampus of 12-month-old females [71]. In two of the studies that reported greater Aβ burden in females, the authors did not observe a correlation between sex and tau burden [61, 64]. Nelson et al. reported less hippocampal and amygdala tau in 5-month-old females relative to males using HT7 immunostaining, although Aβ ELISA uncovered Aβ40 to be three-fold greater in females than males [65].
Sexual dimorphism in physiological responses
Kapadia et al. report worse autoimmunity in male 3xTg-AD mouse model, including reduced CD+T-splenocytes, higher serum autoantibody levels, and greater splenomegaly in 6-month-old males compared to females [73]. They hypothesize that early autoimmunity in the males may protect them from plaque deposition and go on to suggest that the observed sex-related immune differences might be linked to differences in behavior. Of note, no sex differences were observed in cortical tau, Aβ, or BDNF levels in these mice. An investigation of immunoendocrine aging in 3xTg-AD mice reported a worse neuroimmunoendocrine network in males, involving lower splenic lymphocyte chemotaxis and proliferation and lower thymic natural killer cell activity [75, 76].
Adding further complexity to the observed pathological sex differences in 3xTg-AD mice are the effects of sex and stress hormones. Studies using ovariectomized (OVX) 3xTg-AD mice report earlier and accelerated AD pathology and impaired learning compared to sham-operated mice, while 17β-estra-diol treatment was enough to prevent the accelerated decline [77–79]. Gonadectomized (GDX) male 3xTg-AD mice also show increased Aβ and increased memory-related behavioral deficits, which are attenuated with testosterone treatment [80–82]. Together, these studies suggest that androgen and estrogen pathways may influence regulation of Aβ and tau pat-hology in 3xTg-AD mice. Furthermore, studies have found sex-specific corticosteroid responses in 3xTg-AD mice. Clinton et al. report that 9-month-old 3xTg-AD females, but not males, have an elevated corticosterone response following stressful tasks, which consequently affects their performance in memory-related tasks [83]. A recent study corroborates this finding, reporting increased corticosterone response to restraint stress in 4-month-old females compared to males and wild type females, proposing that this increased stress response may precede and contribute to AD pathology in these mice [84]. Given that chr-onic stress is a risk factor for AD [85], these studies suggest that sex-specific stress responses may help explain sex differences in AD pathology. Influences of the neuroendocrine system on AD pathology are of great importance in the context of AD sexual dimorphism and should be considered in pre-clinical research.
Despite worse pathology described by many of the studies discussed here, female 3xTg-AD mice typically outlive their male counterparts. Kane et al. measured survival of 3xTg-AD mice and found females to live an average of 130 days longer than males [86]. This study also described males to have higher frailty index scores than females and concluded frailty index to be an adequate predictor of health span in this model. Rae and Brown tested lifespans of a number of AD mouse models and found female 3xTg-AD mice had an average lifespan of 744 days while males lived an average of 469 days [87], suggesting that lifespan should be taken into account when predicting health span of a particular mouse model. According to their findings, age-related disorders in mice should consider sex differences in life expectancy, so that the relative age of female mice in the 3xTg-AD model is “younger” than males given the females longer life expectancy. This, however, contradicts studies that note worse molecular and cognitive responses in females compared to males.
Sexual dimorphism in behavior
Non-memory-related behavioral discrepancies between 3xTg-AD males and females are not robust and are often influenced by the background strain. At 6.5 months of age, one study reported that 3xTg-AD females displayed higher average velocity than males in the Barnes maze test [88]. These authors also performed fear conditioning and demonstrated that 6.5-month-old 3xTg-AD females spend more time freezing than males. However, this was true for the wild type strain as well. Additionally, Blázquez et al. reported that 3xTg-AD males and their wild type strain exhibit higher behavioral inhibition/fearfulness than females as measured in both the Corner and in the open field (OF) tests at 12 and 15 months of age [89]. In contrast, Fertan et al. showed that female 3xTg-AD mice, regardless of treatment, had longer latency to fall times in the rotarod task compared to males and this was independent of the background strain [90].
Novel object recognition (NOR) tasks assessing working memory appear to be weak discriminators of possible sexual dimorphism for recognition memory as Clinton et al. did not detect any evidence of sexual dimorphism at 2, 6, 9, and 12 months of age in either short-term (3 h) or long term (24 h) intertrial interval (ITI) NOR paradigms [72]. Creighton et al. detected slightly greater impairments in males than females with an ITI of 5 min which disappeared when groups were tested using a 90 min ITI [66]. Importantly, the NOR apparatus used in this study had clear walls, allowing the mice to use spatial cues which may have affected these results.
Multiple studies assessing spatial memory obs-erved that females perform overall worse in the Mor-ris water maze (MWM) task assessing spatial memory. This is supposedly linked to the often-advanced amyloid pathology of female 3xTg-AD mice. Young (4–6 mo) and old (12–15 mo) females show poorer place learning acquisition than males in the MWM and the older female mice also displayed impaired reference memory during platform removal [71, 72, 89, 91]. 12–14 month-old females are shown to perform worse than males in the Y-maze task assessing working spatial memory [60]. In a novel test of spatial memory, the spatial reorientation task, only at 6 months of age were deficits noticeable between female 3xTg-AD mice and non-Tg controls. This deficit was not observed in male mice at 3, 6, nor 12 months of age and also not observed in fem-ale mice at 3 months of age. This study also demon-strated lesser staining for Aβ in the dorsal CA1 re-gion of the hippocampus in males compared to females [58].
In contrast, Stover et al. found that 6.5-month-old females performed better during both the acquisition phase and the reversal phase of the Barnes maze task, making fewer errors and exhibiting a lesser latency to goal time [88]. Additionally, in the radial arm maze (testing working and reference spatial memory), 3xTg-AD male mice commit more errors than female mice beginning at 2 months of age. Importantly, in this task, separate cohorts of increasingly older mice did not perform worse than younger mice and there were no noticeable sex differences in the background strain, B6129SF2/J [92].
Sexual dimorphism in therapeutic response
Lastly, we identified only two studies that were sufficiently powered (n = 10–19 /treatment/sex) to detect sex differences in response to a preclinical therapeutic intervention. In 2016, Sawmiller et al. reported that the naturally occurring flavenoid, diosmin, reduced ce-rebral Aβ oligomers only in female 3xTg-AD mice while it improved markers of phospho-tau and neu-roinflammation in both sexes [93]. Additionally, diosmin was shown to improve memory in both sexes of the 3xTg-AD model in the fear conditioning task with no reports of sexual dimorphism in the B6129SF2/J background strain. In 2019, Fertan et al. showed that a novel Indolamine 2,3-dioxygenase (IDO) inhibitor, DWG-1036, exerted sex-dependent side effects and behavioral changes [90]. They reported that DWG-1036 caused excessive weight loss in females of both WT strain (B6129SF2/J) and the 3xTg-AD strain but not in males. In the trace fear conditioning task of working memory, Fertan et al. observed improved memory (measured by increased duration of freezing) only in DWG-1036-treated 3xTg-AD males. DWG-1036 inhibits IDO, an essential enzyme in kynurenine synthesis pathway which is modulated by sex hormone levels [94]. Interestingly, publicly available studies of anti-amyloid antibody therapies, which are the most recent clinical therapeutic candidates for AD, have mostly been conducted in only female 3xTg-AD mice [95–104]. The only exceptions to this do not present sex-stratified data or comment on sexual dimorphism in AD or the 3xTg-AD model [105–108].
SUMMARY
Sexual dimorphisms are commonly observed in patients and pre-clinical models yet are often ove-rlooked when critically interpreting results. In hum-ans, the incidence, pathology, and presentation of AD differs strikingly between the sexes, yet sex-str-atification of data is often performed as an afte-rthought. In smaller Phase I and II trials, this may potentially veil both sexually dimorphic improvement and exacerbation of disease condition. In an-imal models of AD, sex differences in Aβ plaque load, immune response, lifespan, memory, and non-memory-related behavior are observed. However, few studies report, or have the power to report, these differences.
The literature reviewed here evaluates the heterogeneity in neuropathology, lifespan, and memory- and non-memory-related behavioral performance reported between sexes of the 3xTg-AD mouse model. In some cases, this sexual dimorphism mimics clinical observations; however in others, the AD-like phenotypes are opposite. There are multiple potential reasons for this large variability, one of which includes the source and maintenance of the mouse colony. Our review of the literature indicated that there are two major sources where researchers pro-cured their 3xTg-AD models: purchased from Jackson Laboratory (JAX) and the Mutant Mouse Resource and Research Center (MMRRC) or donated from the originating colony at the laboratory of Dr. LaFerla. We did not observe a correlation between severity of pathology and source of the 3xTg-AD mice used in these studies. In 2014, the developing laboratory communicated to JAX that male 3xTg-AD mice “may not exhibit the phenotypic traits initially described” [109]. This finding, along with the dimorphisms highlighted above, warrants copy number, transgene expression validation, and baseline behavioral analysis in future applications of this model. These measures are rudimentary yet integral to yielding informative and reproducible outcomes. Additionally, male mice from both the 3xTg-AD and background strain exhibit increased potential for aggressiveness that may warrant housing in isolation which has known effects on immune and endocrine signaling as well as non-memory and memory-related behavior [110]. This should be considered when deciding how to house the animals depending on what types of data are being collected.
Another possible cause of the heterogeneity is methodological variations in the biochemical analyses. For example, there are at least four common methods to measure amyloid load in the brain including western blotting, ELISA, Luminex, and immunofluorescence. Adding a further layer of complexity is the wide array of antibodies available for Aβ detection. Many studies analyzed Aβ deposition by immunohistochemistry using antibodies that react to varying regions of Aβ or AβPP including abnormal isoforms and precursor forms of Aβ [59, 62, 70, 71]. Even among antibodies specific for the Aβ40 or Aβ42 end residues, there are multiple products available. Behavior protocols vary widely between groups as well.
Some studies justify their use of one sex over the other, citing reasons including that females are reported to develop amyloid plaques earlier than males, or that females have a more homogenous pathology than male 3xTg-AD mice. The main reason cited for using only males in many of studies is to avoid the metabolic, hormonal, and behavioral changes that accompany the female estrous cycle. Studies that do utilize both sexes do not always compare sexes separately due to low power. Of the 613 original research articles published since the creation of the 3xTg-AD mouse model in 2003, 23% of the articles do not indicate the sex of the animal used in the study. This causes problems when comparing or recreating these studies, especially given the large differences between sexes seen in some of the studies discussed above [66, 69].
In order to rectify and unify our research efforts moving forward, preclinical and clinical studies need to be well powered so that independent statistical analyses on males and females can be performed within each treatment group. Although this may increase the duration and cost (monetary and in animal lives) of a study, it will help account for the variability observed when analyzing results and improve translatability of the findings. The NIA has acknowledged the need for more unified and transparent communication of AD research by creating an easily searchable, peer-reviewed database to submit published and unpublished data from preclinical studies, the Alzheimer’s Disease Preclinical Efficacy Database (AlzPED) [111]. Moving forward, it is highly encouraged to utilize this database both when designing a study and when sharing results. It will help lay the groundwork to synthesize emerging reports of sexual dimorphism, including less studied areas of the AD landscape like synaptic transmission [112, 113], ultrastructural analysis of plaque and tangle morphology [62], and disruptions in circadian rhythm [114].
When choosing and applying mouse models to preclinical research, we make four recommendations that aid consideration of sex as a biological variable (SABV) in accordance with NIH expectations: 1) a thorough understanding of the published characterizations of the model, 2) highly powered studies where males and females can be analyzed independently within each treatment group, 3) independent validation of transgene expression, and 4) at least partial validation of previously published molecular and behavioral hallmarks to be assessed, including baseline behavior and biometrics. Incorporating these four recommendations into future animal model research will aid the continuity between animal model studies and improve the quality of their findings, leading to a stronger understanding of how and which therapeutic approaches hold translational potential.
DISCLOSURE STATEMENT
Authors’ disclosures available online (https://www.j-alz.com/manuscript-disclosures/20-1014r2).
REFERENCES
[1] | Fisher DW , Bennett DA , Dong H ((2018) ) Sexual dimorphism in predisposition to Alzheimer’s disease. Neurobiol Aging 70: , 308–324. |
[2] | Mielke MM , Vemuri P , Rocca WA ((2014) ) Clinical epidemiology of Alzheimer’s disease: Assessing sex and gender differences. Clin Epidemiol 6: , 37–48. |
[3] | Arnold M , Nho K , Kueider-Paisley A , Massaro T , Huynh K , Brauner B , MahmoudianDehkordi S , Louie G , Moseley MA , Thompson JW , John-Williams LS , Tenenbaum JD , Blach C , Chang R , Brinton RD , Baillie R , Han X , Trojanowski JQ , Shaw LM , Martins R , Weiner MW , Trushina E , Toledo JB , Meikle PJ , Bennett DA , Krumsiek J , Doraiswamy PM , Saykin AJ , Kaddurah-Daouk R , Kastenmüller G ((2020) ) Sex and APOE ɛ4 genotype modify the Alzheimer’s disease serum metabolome. Nat Commun 11: , 1148. |
[4] | Altmann A , Tian L , Henderson VW , Greicius MD ; Al-zheimer’s Disease Neuroimaging Initiative Investigators ((2014) ) Sex modifies the APOE-related risk of developing Alzheimer’s disease. Ann Neurol 75: , 563–573. |
[5] | Riedel BC , Thompson PM , Brinton RD ((2016) ) Age, APOE and sex: Triad of risk of Alzheimer’s disease. J Steroid Biochem Mol Biol 160: , 134–147. |
[6] | Scacchi R , Gambina G , Broggio E , Corbo RM ((2014) ) Sex and ESR1 genotype may influence the response to treatment with donepezil and rivastigmine in patients with Alzheimer’s disease. Int J Geriatr Psychiatry 29: , 610–615. |
[7] | Honarpisheh P , McCullough LD ((2019) ) Sex as a biological variable in the pathology and pharmacology of neurodegenerative and neurovascular diseases. Br J Pharmacol 176: , 4173–4192. |
[8] | Mehta D , Jackson R , Paul G , Shi J , Sabbagh M ((2017) ) Why do trials for Alzheimer’s disease drugs keep failing? A discontinued drug perspective for 2010-2015. Expert Opin Investig Drugs 26: , 735–739. |
[9] | Ferretti MT , Iulita MF , Cavedo E , Chiesa PA , Schumacher Dimech A , Santuccione Chadha A , Baracchi F , Girouard H , Misoch S , Giacobini E , Depypere H , Hampel H ; Women’s Brain Project and the Alzheimer Precision Medicine Initiative ((2018) ) Sex differences in Alzheimer disease —the gateway to precision medicine. Nat Rev Neurol 14: , 457–469. |
[10] | Götz J , Bodea LG , Goedert M ((2018) ) Rodent models for Alzheimer disease. Nat Rev Neurosci 19: , 583–598. |
[11] | King A ((2018) ) The search for better animal models of Alzheimer’s disease. Nature 559: , S13–S15. |
[12] | Oddo S , Caccamo A , Shepherd JD , Murphy MP , Golde TE , Kayed R , Metherate R , Mattson MP , Akbari Y , LaFerla FM ((2003) ) Triple-transgenic model of Alzheimer’s disease with plaques and tangles: Intracellular Abeta and synaptic dysfunction. Neuron 39: , 409–421. |
[13] | Oddo S , Caccamo A , Kitazawa M , Tseng BP , LaFerla FM ((2003) ) Amyloid deposition precedes tangle formation in a triple transgenic model of Alzheimer’s disease. Neurobiol Aging 24: , 1063–1070. |
[14] | Barghorn S , Zheng-Fischhöfer Q , Ackmann M , Biernat J , von Bergen M , Mandelkow EM , Mandelkow E ((2000) ) Structure, microtubule interactions, and paired helical filament aggregation by tau mutants of frontotemporal dementias. Biochemistry 39: , 11714–11721. |
[15] | Borrego-Écija S , Morgado J , Palencia-Madrid L , Grau-Rivera O , Reñé R , Hernández I , Almenar C , Balasa M , Antonell A , Molinuevo JL , Lladó A , Martínez de Pancorbo M , Gelpi E , Sánchez-Valle R ((2017) ) Frontotemporal dementia caused by the P301L mutation in the MAPT gene: Clinicopathological features of 13 cases from the same geographical origin in Barcelona, Spain. Dement Geriatr Cogn Disord 44: , 213–221. |
[16] | DeTure MA , Dickson DW ((2019) ) The neuropathological diagnosis of Alzheimer’s disease. Mol Neurodegener 14: , 32. |
[17] | Hou Y , Dan X , Babbar M , Wei Y , Hasselbalch SG , Croteau DL , Bohr VA ((2019) ) Ageing as a risk factor for neurodegenerative disease. Nat Rev Neurol 15: , 565–581. |
[18] | Belfiore R , Rodin A , Ferreira E , Velazquez R , Branca C , Caccamo A , Oddo S ((2019) ) Temporal and regional progression of Alzheimer’s disease-like pathology in 3xTg-AD mice. Aging Cell 18: , e12873. |
[19] | Jankowsky JL , Zheng H ((2017) ) Practical considerations for choosing a mouse model of Alzheimer’s disease. Mol Neurodegener 12: , 89. |
[20] | Mazure CM , Swendsen J ((2016) ) Sex differences in Alzheimer’s disease and other dementias. Lancet Neurol 15: , 451–452. |
[21] | ((2019) ) 2019 Alzheimer’s disease facts and figures. Alzheimers Dement 15: , 321–387. |
[22] | Ungar L , Altmann A , Greicius MD ((2014) ) Apolipoprotein E, gender, and Alzheimer’s disease: An overlooked, but potent and promising interaction. Brain Imaging Behav 8: , 262–273. |
[23] | Farlow MR ((2010) ) Should the ApoE genotype be a covariate for clinical trials in Alzheimer disease? Alzheimers Res Ther 2: , 15. |
[24] | Mosconi L , Berti V , Quinn C , McHugh P , Petrongolo G , Varsavsky I , Osorio RS , Pupi A , Vallabhajosula S , Isaacson RS , de Leon MJ , Brinton RD ((2017) ) Sex differences in Alzheimer risk. Neurology 89: , 1382–1390. |
[25] | Barnes LL , Wilson RS , Bienias JL , Schneider JA , Evans DA , Bennett DA ((2005) ) Sex differences in the clinical manifestations of Alzheimer disease pathology. Arch Gen Psychiatry 62: , 685–691. |
[26] | Buckley RF , Mormino EC , Amariglio RE , Properzi MJ , Rabin JS , Lim YY , Papp KV , Jacobs HIL , Burnham S , Hanseeuw BJ , Doré V , Dobson A , Masters CL , Waller M , Rowe CC , Maruff P , Donohue MC , Rentz DM , Kirn D , Hedden T , Chhatwal J , Schultz AP , Johnson KA , Villemagne VL , Sperling RA ; Alzheimer’s Disease Neuroimaging Initiative; Australian Imaging, Biomarker and Lifestyle study of ageing, Harvard Aging Brain Study ((2018) ) Sex, amyloid, and APOE ɛ4 and risk of cognitive decline in preclinical Alzheimer’s disease: Findings from three well-characterized cohorts. Alzheimers Dement 14: , 1193–1203. |
[27] | Oveisgharan S , Arvanitakis Z , Yu L , Farfel J , Schneider JA , Bennett DA ((2018) ) Sex differences in Alzheimer’s disease and common neuropathologies of aging. Acta Neuropathol 136: , 887–900. |
[28] | Buckley RF , Mormino EC , Rabin JS , Hohman TJ , Landau S , Hanseeuw BJ , Jacobs HIL , Papp KV , Amariglio RE , Properzi MJ , Schultz AP , Kirn D , Scott MR , Hedden T , Farrell M , Price J , Chhatwal J , Rentz DM , Villemagne VL , Johnson KA , Sperling RA ((2019) ) Sex differences in the association of global amyloid and regional tau deposition measured by positron emission tomography in clinically normal older adults. JAMA Neurol 76: , 542–551. |
[29] | Scheyer O , Rahman A , Hristov H , Berkowitz C , Isaacson RS , Brinton RD , Mosconi L ((2018) ) Female sex and Alzheimer’s risk: The menopause connection. J Prev Alzheimers Dis 5: , 225–230. |
[30] | Brann DW , Dhandapani K , Wakade C , Mahesh VB , Khan MM ((2007) ) Neurotrophic and neuroprotective actions of estrogen: Basic mechanisms and clinical implications. Steroids 72: , 381–405. |
[31] | McCarthy MM , Auger AP , Bale TL , De Vries GJ , Dunn GA , Forger NG , Murray EK , Nugent BM , Schwarz JM , Wilson ME ((2009) ) The epigenetics of sex differences in the brain. J Neurosci 29: , 12815–12823. |
[32] | Klein SL , Flanagan KL ((2016) ) Sex differences in immune responses. Nat Rev Immunol 16: , 626–638. |
[33] | Frost GR , Jonas LA , Li YM ((2019) ) Friend, foe or both? Immune activity in Alzheimer’s disease. Front Aging Neurosci 11: , 337. |
[34] | Fairweather D , Frisancho-Kiss S , Rose NR ((2008) ) Sex differences in autoimmune disease from a pathological perspective. Am J Pathol 173: , 600–609. |
[35] | Cook IF ((2008) ) Sexual dimorphism of humoral immunity with human vaccines. Vaccine 26: , 3551–3555. |
[36] | Wang CY , Wang PN , Chiu MJ , Finstad CL , Lin F , Lynn S , Tai YH , De Fang X , Zhao K , Hung CH , Tseng Y , Peng WJ , Wang J , Yu CC , Kuo BS , Frohna PA ((2017) ) UB-311, a novel UBITh® amyloid β peptide vaccine for mild Alzheimer’s disease. Alzheimers Dement (N Y) 3: , 262–272. |
[37] | Lambracht-Washington D , Rosenberg RN ((2013) ) Adv-ances in the development of vaccines for Alzheimer’s disease. Discov Med 15: , 319–326. |
[38] | Seshadri S , Wolf PA , Beiser A , Au R , McNulty K , White R , D’Agostino RB ((1997) ) Lifetime risk of dementia and Alzheimer’s disease: The impact of mortality on risk estimates in the Framingham Study. Neurology 49: , 1498–1504. |
[39] | S.1 - 103rd Congress (1993-1994): National Institutes of Health Revitalization Act of 1993. |
[40] | Franconi F , Campesi I , Colombo D , Antonini P ((2019) ) Sex-gender variable: Methodological recommendations for increasing scientific value of clinical studies. Cells 8: , 476. |
[41] | Canevelli M , Quarata F , Remiddi F , Lucchini F , Lacorte E , Vanacore N , Bruno G , Cesari M ((2017) ) Sex and gender differences in the treatment of Alzheimer’s disease: A systematic review of randomized controlled trials. Pharmacol Res 115: , 218–223. |
[42] | Pradier C , Sakarovitch C , Le Duff F , Layese R , Metelkina A , Anthony S , Tifratene K , Robert P ((2014) ) The mini mental state examination at the time of Alzheimer’s disease and related disorders diagnosis, according to age, education, gender and place of residence: A cross-sectional study among the French National Alzheimer database. PLoS One 9: , e103630. |
[43] | Sundermann EE , Maki P , Biegon A , Lipton RB , Mielke MM , Machulda M , Bondi MW ; Alzheimer’s Disease Neuroimaging Initiative ((2019) ) Sex-specific norms for verbal memory tests may improve diagnostic accuracy of amnestic MCI. Neurology 93: , e1881–e1889. |
[44] | Giacobini E , Pepeu G ((2018) ) Sex and gender differences in the brain cholinergic system and in the response to therapy of Alzheimer disease with cholinesterase inhibitors. Curr Alzheimer Res 15: , 1077–1084. |
[45] | Waxman DJ , Holloway MG ((2009) ) Sex differences in the expression of hepatic drug metabolizing enzymes. Mol Pharmacol 76: , 215–228. |
[46] | Wang J , Tanila H , Puoliväli J , Kadish I , van Groen T ((2003) ) Gender differences in the amount and deposition of amyloidβ in APPswe and PS1 double transgenic mice. Neurobiol Dis 14: , 318–327. |
[47] | Pistell PJ , Zhu M , Ingram DK ((2008) ) Acquisition of conditioned taste aversion is impaired in the amyloid precursor protein/presenilin 1 mouse model of Alzheimer’s disease. Neuroscience 152: , 594–600. |
[48] | Ordóñez-Gutiérrez L , Antón M , Wandosell F ((2015) ) Peripheral amyloid levels present gender differences associated with aging in AβPP/PS1 mice. J Alzheimers Dis 44: , 1063–1068. |
[49] | Ordoñez-Gutierrez L , Fernandez-Perez I , Herrera JL , Anton M , Benito-Cuesta I , Wandosell F ((2016) ) AβPP/PS1 transgenic mice show sex differences in the cerebellum associated with aging. J Alzheimers Dis 54: , 645–656. |
[50] | Howlett DR , Richardson JC , Austin A , Parsons AA , Bate ST , Davies DC , Gonzalez MI ((2004) ) Cognitive correlates of Abeta deposition in male and female mice bearing amyloid precursor protein and presenilin-1 mutant transgenes. Brain Res 1017: , 130–136. |
[51] | Callahan MJ , Lipinski WJ , Bian F , Durham RA , Pack A , Walker LC ((2001) ) Augmented senile plaque load in aged female β-Amyloid precursor protein-transgenic mice. Am J Pathol 158: , 1173–1177. |
[52] | Schmid S , Rammes G , Blobner M , Kellermann K , Bratke S , Fendl D , Kaichuan Z , Schneider G , Jungwirth B ((2019) ) Cognitive decline in Tg2576 mice shows sex-specific differences and correlates with cerebral amyloid-beta. Behav Brain Res 359: , 408–417. |
[53] | Sun Y , Guo Y , Feng X , Jia M , Ai N , Dong Y , Zheng Y , Fu L , Yu B , Zhang H , Wu J , Yu X , Wu H , Kong W ((2020) ) The behavioural and neuropathologic sexual dimorphism and absence of MIP-3α in tau P301S mouse model of Alzheimer’s disease. J Neuroinflammation 17: , 72. |
[54] | Buccarello L , Grignaschi G , Castaldo AM , Di Giancamillo A , Domeneghini C , Melcangi RC , Borsello T ((2017) ) Sex impact on tau-aggregation and postsynaptic protein levels in the P301L mouse model of tauopathy. J Alzheimers Dis 56: , 1279–1292. |
[55] | Devi L , Alldred MJ , Ginsberg SD , Ohno M ((2010) ) Sex- and brain region-specific acceleration of β-amyloidogenesis following behavioral stress in a mouse model of Alzheimer’s disease. Mol Brain 3: , 34. |
[56] | Roddick KM , Schellinck HM , Brown RE ((2014) ) Olfactory delayed matching to sample performance in mice: Sex differences in the 5XFAD mouse model of Alzheimer’s disease. Behav Brain Res 270: , 165–170. |
[57] | Granger MW , Franko B , Taylor MW , Messier C , George-Hyslop PS , Bennett SA ((2016) ) A TgCRND8 mouse model of Alzheimer’s disease exhibits sexual dimorphisms in behavioral indices of cognitive reserve. J Alzheimers Dis 51: , 757–773. |
[58] | Stimmell AC , Baglietto-Vargas D , Moseley SC , Lapointe V , Thompson LM , LaFerla FM , McNaughton BL , Wilber AA ((2019) ) Impaired spatial reorientation in the 3xTg-AD mouse model of Alzheimer’s disease. Sci Rep 9: , 1311. |
[59] | Perez SE , He B , Muhammad N , Oh KJ , Fahnestock M , Ikonomovic MD , Mufson EJ ((2011) ) Cholinotrophic basal forebrain system alterations in 3xTg-AD transgenic mice. Neurobiol Dis 41: , 338–352. |
[60] | Carroll JC , Rosario ER , Kreimer S , Villamagna A , Gentzschein E , Stanczyk FZ , Pike CJ ((2010) ) Sex differences in β-amyloid accumulation in 3xTg-AD mice: Role of neonatal sex steroid hormone exposure. Brain Res 1366: , 233–245. |
[61] | Giménez-Llort L , García Y , Buccieri K , Revilla S , Suñol C , Cristofol R , Sanfeliu C ((2010) ) Gender-specific neuroimmunoendocrine response to treadmill exercise in 3xTg-AD mice. Int J Alzheimers Dis 2010: , e128354. |
[62] | Oh KJ , Perez SE , Lagalwar S , Vana L , Binder L , Mufson EJ ((2010) ) Staging of Alzheimer’s pathology in triple transgenic mice: A light and electron microscopic analysis. Int J Alzheimers Dis 2010: , e780102. |
[63] | Rodríguez JJ , Jones VC , Tabuchi M , Allan SM , Knight EM , LaFerla FM , Oddo S , Verkhratsky A ((2008) ) Impaired adult neurogenesis in the dentate gyrus of a triple transgenic mouse model of Alzheimer’s disease. PLoS One 3: , e2935. |
[64] | Hirata-Fukae C , Li HF , Hoe HS , Gray AJ , Minami SS , Hamada K , Niikura T , Hua F , Tsukagoshi-Nagai H , Horikoshi-Sakuraba Y , Mughal M , Rebeck GW , LaFerla FM , Mattson MP , Iwata N , Saido TC , Klein WL , Duff KE , Aisen PS , Matsuoka Y ((2008) ) Females exhibit more extensive amyloid, but not tau, pathology in an Alzheimer transgenic model. Brain Res 1216: , 92–103. |
[65] | Nelson RL , Guo Z , Halagappa VM , Pearson M , Gray AJ , Matsuoka Y , Brown M , Martin B , Iyun T , Maudsley S , Clark RF , Mattson MP ((2007) ) Prophylactic treatment with paroxetine ameliorates behavioral deficits and retards the development of amyloid and tau pathologies in 3xTgAD mice. Exp Neurol 205: , 166–176. |
[66] | Creighton SD , Mendell AL , Palmer D , Kalisch BE , MacLusky NJ , Prado VF , Prado MAM , Winters BD ((2019) ) Dissociable cognitive impairments in two strains of transgenic Alzheimer’s disease mice revealed by a battery of object-based tests. Sci Rep 9: , 57. |
[67] | Bories C , Guitton MJ , Julien C , Tremblay C , Vandal M , Msaid M , De Koninck Y , Calon F ((2012) ) Sex-dependent alterations in social behaviour and cortical synaptic activity coincide at different ages in a model of Alzheimer’s disease. PLoS One 7: , e46111. |
[68] | Vandal M , White PJ , Chevrier G , Tremblay C , St-Amour I , Planel E , Marette A , Calon F ((2015) ) Age-dependent impairment of glucose tolerance in the 3xTg-AD mouse model of Alzheimer’s disease. FASEB J 29: , 4273–4284. |
[69] | Omori C , Motodate R , Shiraki Y , Chiba K , Sobu Y , Kimura A , Nakaya T , Kondo H , Kurumiya S , Tanaka T , Yamamoto K , Nakajima M , Suzuki T , Hata S ((2017) ) Facilitation of brain mitochondrial activity by 5-aminolevulinic acid in a mouse model of Alzheimer’s disease. Nutr Neurosci 20: , 538–546. |
[70] | Hebda-Bauer EK , Simmons TA , Sugg A , Ural E , Stewart JA , Beals JL , Wei Q , Watson SJ , Akil H ((2013) ) 3xTg-AD mice exhibit an activated central stress axis during early-stage pathology. J Alzheimers Dis 33: , 407–422. |
[71] | Yang JT , Wang ZJ , Cai HY , Yuan L , Hu MM , Wu MN , Qi JS ((2018) ) Sex differences in neuropathology and cognitive behavior in APP/PS1/tau triple-transgenic mouse model of Alzheimer’s disease. Neurosci Bull 34: , 736–746. |
[72] | Clinton LK , Billings LM , Green KN , Caccamo A , Ngo J , Oddo S , McGaugh JL , LaFerla FM ((2007) ) Age-dependent sexual dimorphism in cognition and stress response in the 3xTg-AD mice. Neurobiol Dis 28: , 76–82. |
[73] | Kapadia M , Mian MF , Michalski B , Azam AB , Ma D , Salwierz P , Christopher A , Rosa E , Zovkic IB , Forsythe P , Fahnestock M , Sakic B ((2018) ) Sex-dependent differences in spontaneous autoimmunity in adult 3xTg-AD mice. J Alzheimers Dis 63: , 1191–1205. |
[74] | Russo-Savage L , Rao VKS , Eipper BA , Mains RE ((2020) ) Role of Kalirin and mouse strain in retention of spatial memory training in an Alzheimer’s disease model mouse line. Neurobiol Aging 95: , 69–80. |
[75] | Arranz L , De Castro NM , Baeza I , Giménez-Llort L , De la Fuente M ((2011) ) Effect of environmental enrichment on the immunoendocrine aging of male and female triple-transgenic 3xTg-AD mice for Alzheimer’s disease. J Alzheimers Dis 25: , 727–737. |
[76] | Giménez-Llort L , Arranz L , Maté I , la Fuente MD ((2008) ) Gender-specific neuroimmunoendocrine aging in a triple-transgenic 3×Tg-AD mouse model for Alzheimer’s disease and its relation with longevity. Neuroimmunomodulation 15: , 331–343. |
[77] | Carroll JC , Pike CJ ((2008) ) Selective estrogen receptor modulators differentially regulate Alzheimer-like changes in female 3xTg-AD mice. Endocrinology 149: , 2607–2611. |
[78] | Carroll JC , Rosario ER , Chang L , Stanczyk FZ , Oddo S , LaFerla FM , Pike CJ ((2007) ) Progesterone and estrogen regulate Alzheimer-like neuropathology in female 3xTg-AD mice. J Neurosci 27: , 13357–13365. |
[79] | Carroll JC , Rosario ER , Villamagna A , Pike CJ ((2010) ) Continuous and cyclic progesterone differentially interact with estradiol in the regulation of Alzheimer-like pathology in female 3×Transgenic-Alzheimer’s disease mice. Endocrinology 151: , 2713–2722. |
[80] | Rosario ER , Carroll JC , Oddo S , LaFerla FM , Pike CJ ((2006) ) Androgens regulate the development of neuropathology in a triple transgenic mouse model of Alz-heimer’s disease. J Neurosci 26: , 13384–13389. |
[81] | Rosario ER , Carroll J , Pike CJ ((2010) ) Testosterone regulation of Alzheimer-like neuropathology in male 3xTg-AD mice involves both estrogen and androgen pathways. Brain Res 1359: , 281–290. |
[82] | Rosario ER , Carroll JC , Pike CJ ((2012) ) Evaluation of the effects of testosterone and luteinizing hormone on regulation of β-amyloid in male 3xTg-AD mice. Brain Res 1466: , 137–145. |
[83] | Clinton LK , Billings LM , Green KN , Caccamo A , Ngo J , Oddo S , McGaugh JL , LaFerla FM ((2007) ) Age-dependent sexual dimorphism in cognition and stress response in the 3xTg-AD mice. Neurobiol Dis 28: , 76–82. |
[84] | Nguyen ET , Selmanovic D , Maltry M , Morano R , Franco-Villanueva A , Estrada CM , Solomon MB ((2020) ) Endocrine stress responsivity and social memory in 3xTg-AD female and male mice: A tale of two experiments. Horm Behav 126: , 104852. |
[85] | Machado A , Herrera AJ , de Pablos RM , Espinosa-Oliva AM , Sarmiento M , Ayala A , Venero JL , Santiago M , Villarán RF , Delgado-Cortés MJ , Argüelles S , Cano J ((2014) ) Chronic stress as a risk factor for Alzheimer’s disease. Rev Neurosci 25: , 785–804. |
[86] | Kane AE , Shin S , Wong AA , Fertan E , Faustova NS , Howlett SE , Brown RE ((2018) ) Sex differences in healthspan predict lifespan in the 3xTg-AD mouse model of Alzheimer’s disease. Front Aging Neurosci 10: , 172. |
[87] | Rae EA , Brown RE ((2015) ) The problem of genotype and sex differences in life expectancy in transgenic AD mice. Neurosci Biobehav Rev 57: , 238–251. |
[88] | Stover KR , Campbell MA , Van Winssen CM , Brown RE ((2015) ) Early detection of cognitive deficits in the 3xTg-AD mouse model of Alzheimer’s disease. Behav Brain Res 289: , 29–38. |
[89] | Blázquez G , Cañete T , Tobeña A , Giménez-Llort L , Fernández-Teruel A ((2014) ) Cognitive and emotional profiles of aged Alzheimer’s disease (3×TgAD) mice: Effects of environmental enrichment and sexual dimorphism. Behav Brain Res 268: , 185–201. |
[90] | Fertan E , Wong AA , Vienneau NA , Brown RE ((2019) ) Age and sex differences in motivation and spatial working memory in 3xTg-AD mice in the Hebb-Williams maze. Behav Brain Res 370: , 111937. |
[91] | Cañete T , Blázquez G , Tobeña A , Giménez-Llort L , Fernandez-Teruel A ((2015) ) Cognitive and emotional alterations in young Alzheimer’s disease (3xTgAD) mice: Effects of neonatal handling stimulation and sexual dimorphism. Behav Brain Res 281: , 156–171. |
[92] | Stevens LM , Brown RE ((2015) ) Reference and working memory deficits in the 3xTg-AD mouse between 2 and 15-months of age: A cross-sectional study. Behav Brain Res 278: , 496–505. |
[93] | Sawmiller D , Habib A , Li S , Darlington D , Hou H , Tian J , Shytle RD , Smith A , Giunta B , Mori T , Tan J ((2016) ) Diosmin reduces cerebral Aβ levels, tau hyperphosphorylation, neuroinflammation, and cognitive impairment in the 3xTg-AD mice. J Neuroimmunol 299: , 98–106. |
[94] | Baratta AM , Buck SA , Buchla AD , Fabian CB , Chen S , Mong JA , Pocivavsek A ((2018) ) Sex differences in hippocampal memory and kynurenic acid formation following acute sleep deprivation in rats. Sci Rep 8: , 6963. |
[95] | Rasool S , Martinez-Coria H , Wu JW , LaFerla F , Glabe CG ((2013) ) Systemic vaccination with anti-oligomeric monoclonal antibodies improves cognitive function by reducing Aβ deposition and tau pathology in 3xTg-AD mice. J Neurochem 126: , 473–482. |
[96] | Montoliu-Gaya L , Esquerda-Canals G , Bronsoms S , Villegas S ((2017) ) Production of an anti-Aβ antibody fragment in Pichia pastoris and in vitro and in vivo validation of its therapeutic effect. PLoS One 12: , e0181480. |
[97] | Herline K , Prelli F , Mehta P , MacMurray C , Goñi F , Wisniewski T ((2018) ) Immunotherapy to improve cognition and reduce pathological species in an Alzheimer’s disease mouse model. Alzheimers Res Ther 10: , 54. |
[98] | Montoliu-Gaya L , Güell-Bosch J , Esquerda-Canals G , Roda AR , Serra-Mir G , Lope-Piedrafita S , Sánchez-Quesada JL , Villegas S ((2018) ) Differential effects of apoE and apoJ mimetic peptides on the action of an anti-Aβ scFv in 3xTg-AD mice. Biochem Pharmacol 155: , 380–392. |
[99] | Rosenberg RN , Fu M , Lambracht-Washington D ((2018) ) Active full-length DNA Aβ42 immunization in 3xTg-AD mice reduces not only amyloid deposition but also tau pathology. Alzheimers Res Ther 10: , 115. |
[100] | Acero G , Garay C , Venegas D , Ortega E , Gevorkian G ((2020) ) Novel monoclonal antibody 3B8 specifically recognizes pyroglutamate-modified amyloid β 3-42 peptide in brain of AD patients and 3xTg-AD transgenic mice. Neurosci Lett 724: , 134876. |
[101] | Güell-Bosch J , Lope-Piedrafita S , Esquerda-Canals G , Montoliu-Gaya L , Villegas S ((2020) ) Progression of Alzheimer’s disease and effect of scFv-h3D6 immunotherapy in the 3xTg-AD mouse model: An in vivo longitudinal study using magnetic resonance imaging and spectroscopy. NMR Biomed 33: , e4263. |
[102] | Esquerda-Canals G , Roda AR , Martí-Clúa J , Montoliu-Gaya L , Rivera-Hernández G , Villegas S ((2019) ) Treatment with scFv-h3D6 prevented neuronal loss and improved spatial memory in young 3xTg-AD mice by reducing the intracellular Amyloid-β burden. J Alzheimers Dis 70: , 1069–1091. |
[103] | Roda AR , Montoliu-Gaya L , Serra-Mir G , Villegas S ((2020) ) Both amyloid-β peptide and tau protein are affected by an anti-amyloid-β antibody fragment in elderly 3xTg-AD mice. Int J Mol Sci 21: , 6630. |
[104] | Esquerda-Canals G , Marti J , Rivera-Hernández G , Giménez-Llort L , Villegas S ((2013) ) Loss of deep cerebellar nuclei neurons in the 3xTg-AD mice and protection by an anti-amyloid β antibody fragment. MAbs 5: , 660–664. |
[105] | Oddo S , Billings L , Kesslak JP , Cribbs DH , LaFerla FM ((2004) ) Aβ immunotherapy leads to clearance of early, but not late, hyperphosphorylated tau aggregates via the proteasome. Neuron 43: , 321–332. |
[106] | Mamikonyan G , Necula M , Mkrtichyan M , Ghochikyan A , Petrushina I , Movsesyan N , Mina E , Kiyatkin A , Glabe CG , Cribbs DH , Agadjanyan MG ((2007) ) Anti-Aβ1-11 antibody binds to different β-amyloid species, inhibits fibril formation, and disaggregates preformed fibrils but not the most toxic oligomers. J Biol Chem 282: , 22376–22386. |
[107] | Rasool S , Martinez-Coria H , Milton S , Glabe CG ((2013) ) Nonhuman amyloid oligomer epitope reduces Alzheimer’s-like neuropathology in 3xTg-AD transgenic mice. Mol Neurobiol 48: , 931–940. |
[108] | Kazim SF , Chuang SC , Zhao W , Wong RKS , Bianchi R , Iqbal K ((2017) ) Early-onset network hyperexcitability in presymptomatic Alzheimer’s disease transgenic mice is suppressed by passive immunization with anti-human APP/Aβ antibody and by mGluR5 blockade. Front Aging Neurosci 9: , 71. |
[109] | jax.org, B6;129-Tg(APPSwe,tauP301L)1Lfa Psen1tm1Mpm/Mmjax. |
[110] | Kappel S , Hawkins P , Mendl MT ((2017) ) To group or not to group? Good practice for housing male laboratory mice. Animals (Basel) 7: , 88. |
[111] | AlzPED | Alzheimer’s Disease Preclinical Efficacy Database, https://alzped.nia.nih.gov/, Accessed on August 18, 2020. |
[112] | Alia A , Roßner S ((2018) ) Gender, GABAergic dysfunction and AD. Aging (Albany NY) 10: , 3636–3637. |
[113] | Jiao SS , Bu XL , Liu YH , Zhu C , Wang QH , Shen LL , Liu CH , Wang YR , Yao XQ , Wang YJ ((2016) ) Sex dimorphism profile of Alzheimer’s disease-type pathologies in an APP/PS1 mouse model. Neurotox Res 29: , 256–266. |
[114] | Sterniczuk R , Dyck RH , Laferla FM , Antle MC ((2010) ) Characterization of the 3xTg-AD mouse model of Alzheimer’s disease: Part 1. Circadian changes. Brain Res 1348: , 139–148. |
[115] | Dufort-Gervais J , Provost C , Charbonneau L , Norris CM , Calon F , Mongrain V , Brouillette J ((2020) ) Neuroligin-1 is altered in the hippocampus of Alzheimer’s disease patients and mouse models, and modulates the toxicity of amyloid-beta oligomers. Sci Rep 10: , 6956. |