APOE4 Status is Related to Differences in Memory-Related Brain Function in Asymptomatic Older Adults with Family History of Alzheimer’s Disease: Baseline Analysis of the PREVENT-AD Task Functional MRI Dataset
Abstract
Background:
Episodic memory decline is one of the earliest symptoms of late-onset Alzheimer’s disease (AD). Older adults with the apolipoprotein E ɛ4 (+APOE4) genetic risk factor for AD may exhibit altered patterns of memory-related brain activity years prior to initial symptom onset.
Objective:
Here we report the baseline episodic memory task functional MRI results from the PRe-symptomatic EValuation of Experimental or Novel Treatments for Alzheimer’s Disease cohort in Montreal, Canada, in which 327 healthy older adults were scanned within 15 years of their parent’s conversion to AD.
Methods:
Volunteers were scanned as they encoded and retrieved object-location spatial source associations. The task was designed to discriminate between brain activity related to spatial source recollection and object-only (recognition) memory. We used multivariate partial least squares (PLS) to test the hypothesis that +APOE4 adults with family history of AD would exhibit altered patterns of brain activity in the recollection-related memory network, comprised of medial frontal, parietal, and medial temporal cortices, compared to APOE4 non-carriers (–APOE4). We also examined group differences in the correlation between event-related brain activity and memory performance.
Results:
We found group similarities in memory performance and in task-related brain activity in the recollection network, but differences in brain activity-behavior correlations in ventral occipito-temporal, medial temporal, and medial prefrontal cortices during episodic encoding.
Conclusion:
These findings are consistent with previous literature on the influence of APOE4 on brain activity and provide new perspective on potential gene-based differences in brain-behavior relationships in people with first-degree family history of AD.
INTRODUCTION
Late-onset sporadic Alzheimer’s disease (AD) accounts for an estimated 70% of dementia cases worldwide [1, 2]. Typically appearing after 65 years of age, this form of AD greatly increases in incidence beyond 85 years of age and has grown in prevalence with increasing life expectancy [3]. Despite the rising prevalence and burden of AD, there are currently no widely effective treatments to prevent or delay symptom progression [2]. Moreover, new AD drug trials fail at a rate of over 100 : 1 [4].
Given the current challenges of developing effective treatments for AD, increasing focus surrounds early identification, intervention, and prevention of AD in asymptomatic adults at higher risk of developing the disease [5]. Such risk factors include having an apolipoprotein E ɛ4 (APOE4) allele as well as first-degree family history (i.e., parent or sibling) of the disease [6]. The combination of these factors appears to have an additive effect on AD risk and may influence the age of symptom onset beyond the effects of APOE genotype alone [7]. Evidence further indicates that subtle neurological changes may precede symptom onset by as many as 20 years, during a period known as the ‘silent’ preclinical stage of AD [8, 9]. Therefore, studies have increasingly focused on searching for early neural biomarkers or cognitive indices that could help predict AD development—particularly in individuals at higher risk of AD—at a period in which neurocognitive systems remain relatively intact [10–12]. Such early risk identification would permit access to interventions aimed at preventing or delaying AD onset (e.g., physical and cognitive exercise, control of blood pressure and cardiovascular conditions, improved diet, etc.) before the disease has progressed, and may help decrease the social and economic burdens associated with AD [13–15].
Declines in memory of past personal events (i.e., episodic memory) represent one of the earliest symptoms of AD [16] and associate with preclinical or pre-symptomatic AD-related neuropathology [17]. Thus, the neural systems supporting episodic memory may be key candidate sites in which the first signs of AD-related neuropathology arise during the ‘silent’ phase of the disease [18, 19]. Consistent with this theory, differences in task-related brain activity in people with mild cognitive impairment and AD, compared to healthy older adults, appear to reflect declines in memory and attention processing [20, 21], and may index changes related to AD progression [22, 23]. Moreover, brain activity related to memory encoding in regions that subserve episodic memory, including hippocampal, parahippocampal, posterior parietal, and lateral prefrontal cortex, appears different in healthy aging, mild cognitive impairment, and AD [24]. Notably, although recollection of rich contextual details related to a past event tends to decline even in healthy aging [25, 26], recognition of previously encountered objects or events appears more severely impacted in pathological aging [27]. Episodic memory tasks that can differentiate the neural systems associated with familiarity, compared to recollection, may therefore be particularly helpful in identifying early signs of AD related neuropathology in healthy, at-risk adults.
Our past work demonstrated an association between episodic memory performance and changes in task-related functional magnetic resonance imaging (fMRI) detectable as early as middle age [28] and more pronounced in APOE4 carriers or those with family history of AD [29]. These distinct associations appear in the absence of measurable behavioral differences and may be greater for the encoding and retrieval of contextual details associated with a past event, compared to general sense of familiarity [28]. Examining brain-behavior relationships during episodic memory task performance may therefore provide new insights for identifying early AD markers. In particular, exploring differences in episodic memory-related brain function in older adults with and without risk factors such as an APOE4 allele and family history of AD may help better understand the impact of AD on memory systems [30–33].
The present study
Here we primarily aimed to report data from the functional neuroimaging task used in the PREVENT-AD program (https://douglas.research.mcgill.ca/stop-ad-centre), in a sample of older adults at higher risk of AD due to a parental or multiple-sibling family history. Previous studies on AD risk, particularly those investigating the contribution of genetic profile on cognitive symptoms, have not always considered family history despite evidence for its impact on AD development beyond genetic composure [7]. Failure to account for such combined gene-environment contributors may lead to an inaccurate estimate of APOE4 influence on AD and individual risk profiles [34]. Because our sample uniformly contained older adults with first-degree family history of AD, we aimed to examine the potential influence of APOE4 on episodic memory performance and related brain activity over and above the influence of family history.
Moreover, whereas previous studies have largely drawn on univariate analytical tools, comparatively few have examined the relationship between APOE4 and whole-brain functional changes. Thus, we further sought to examine the potential influence of carrying an APOE4 allele (i.e., +APOE4) on whole-brain activity during encoding and retrieval of objects (i.e., recognition) and their location (i.e., source recall), in cognitively healthy older adults with parental history of AD.
To achieve this, we performed partial least squares (PLS) analyses, a data-driven method to objectively assess patterns of whole-brain activity in APOE4 carriers versus non-carriers. Although traditional methods such as univariate contrast-based, voxel-wise approaches can also evaluate task-related differences in brain activity, PLS has several advantages. First, PLS-based approaches do not rely on pre-defined contrasts, revealing the most stable and robust effects using permutation testing and bootstrapping. In addition, PLS does not rely on the assumptions associated with linear modeling (e.g., normality of sample distribution, equal sample sizes). Finally, PLS offers a sensitive, data-driven multivariate approach that captures the complex relationships between whole-brain patterns of brain activity and exogenous factors (e.g., episodic memory performance) in a single mathematical step [35, 36]. Thus, PLS is the ideal method to examine memory-related patterns of brain activity and the relationship of such activity with performance in our sample of APOE4 carriers versus non-carriers.
We hypothesized that, relative to –APOE4, +APOE4 would display different patterns of brain activity unrestricted to the hippocampus or medial temporal lobe during episodic encoding and retrieval. We further anticipated that the difference in task-related brain responses between APOE4 carriers versus non-carriers would interact with object recognition and recall of spatial context. Specifically, given that source recollection performance and associated brain activity may change even in healthy aging [25, 26], we anticipated that differences in brain-behavior relationships would primarily reflect a deficit in object recognition in +APOE4 compared to –APOE4 individuals.
METHODS
Participants: PREVENT-AD Cohort
Participants were recruited for the longitudinal PRe-symptomatic EValuation of Experimental or Novel Treatments for Alzheimer’s Disease (PREVENT-AD) program, an observational cohort study of asymptomatic older adults with a parental or multiple-sibling family history of AD in Montreal, Canada [37]. We evaluated baseline data from 327 older adults (Mage = 63.40±5.24 years, 234 women) who were enrolled up to August 31, 2017 (i.e., data release 5.0) and participated in the task fMRI portion of the study (see below).
We excluded participants on the basis of confounding genetic factors (i.e., APOE2 carriers, n = 34; APOE44, n = 7; unavailable genotype, n = 3); having below-chance performance or fewer than eight trials per response type in the task fMRI protocol (n = 95); and poor fMRI image resolution (n = 32). Our final sample comprised 172 older adults (Mage = 63.42±5.12 years; 128 women), including 103 APOE33 (i.e., –APOE4; 76/103 = 74% women) and 69 APOE34 heterozygotes (i.e., +APOE4; 52/69 = 75% women).
Protocol
Enrolment criteria for the PREVENT-AD trial are described elsewhere [37]. Briefly, all participants had at least one parent or multiple siblings diagnosed with sporadic AD or a condition suggesting AD-like dementia within 15 years [38]. At baseline and during each subsequent follow-up assessment, participants performed neuropsychological tests as well as an object-location memory task in the scanner, described below. Here we focus on baseline analyses of the task-related fMRI based on APOE4 genotype. We describe longitudinal analyses of this task fMRI protocol in a forthcoming report. For more information on PREVENT-AD, see: douglas.qc.ca/page/prevent-alzheimer-the-centre.
Determination of family history of AD
If a documented expert medical history was not available, a brief questionnaire from the Cache County Study on Memory Health and Aging (Utah, USA) determined that a parent or multiple siblings: 1) had trouble with memory or concentration that was sufficiently severe to cause disability or loss of function; 2) had insidious onset or gradual progression of the condition that was not an obvious consequence of a stroke or other sudden insult.
APOE genotyping
Genetic characterization was completed via blood draw, as previously described [39]. DNA was isolated from 200μl of the blood sample using QIASymphony and the DNA Blood Mini QIA kit (Qiagen, Valencia, CA, USA). APOE gene variant was determined using pyrosequencing with PyroMark Q96 (Qiagen, Toronto, ON, Canada). For further detail on the methods used in the PREVENT-AD cohort, see Tremblay-Mercier et al. [40].
Neuropsychological testing
Neuropsychological assessments took approximately 40 minutes to administer and were completed prior to every testing session. The test battery included:
The Repeatable Battery for the Assessment of Neuropsychological Status (RBANS), a battery of neuropsychological assessments aiming to identify abnormal cognitive decline in older adults [41]. The RBANS provides scaled scores for five cognitive indices: immediate memory, visuospatial construct, language, attention, and delayed memory. We included these scaled scores, as well as the total score, in our analyses. Different versions of the RBANS were used in follow-up sessions to prevent practice effects.
The Alzheimer-Dementia Eight Scale (AD8), an eight-item screening tool. The AD8 items index memory, orientation, judgment, and function. A score of two or above suggests impaired cognitive function [42].
The Clinical Dementia Rating (CDR), a five-point scale used to characterize memory, orientation, judgment & problem solving, community affairs, home & hobbies, and personal care [43]. The information for each rating is obtained through a semi-structured interview of the patient and a reliable informant or collateral source (e.g., family member).
The Montreal Cognitive Assessment (MoCA), a brief cognitive screening tool sensitive to mild declines in cognitive function [44].
Task fMRI: Behavioral protocol
Participants were instructed to lie supine in a 3T Siemens Trio scanner (see below), while performing a source memory task programmed in E-Prime version 1.0 (Psychology Software Tools, Inc). During an initial encoding phase, participants were cued (10 s) to memorize a series of 48 colored line drawings of common objects from the BOSS database [45] in their spatial location (i.e., to the left or right of a central fixation cross). Each object was presented for 2000 ms followed by a variable inter-trial interval (ITI; durations of 2200, 4400, or 8800 ms; mean ITI = 5.13 s) to add jitter to the fMRI data collection [46]. Following the encoding phase, there was a 20 min delay during which participants received structural MRI scans.
Following the 20 min delay, a cue (10 s) alerted participants to the beginning of the retrieval phase. During retrieval, participants were presented with 96 colored drawings of common objects: 48 ‘old’ (i.e., previously encoded) stimuli and 48 novel objects, in randomized order. Each object was presented in the center of the screen for 3000 ms, with variable ITI (2200, 4400, or 8800 ms). All participants used a fiber-optic 4-button response box to make task-related responses, and had an opportunity to familiarize themselves with the response choices during a practice session prior to testing. For each retrieval object, participants made a forced-choice between four alternative answers: i) “The object is FAMILIAR but you don’t remember the location”; ii) “You remember the object and it was previously on the LEFT”; iii) “You remember the object and it was previously on the RIGHT”; and iv) “The object is NEW”. Thus (i) responses reflected object recognition, which may associate more closely with familiarity versus recollection-based retrieval processes, whereas (ii) and (iii) responses reflect associative recollection of object-location associations, and (iv) responses reflected either correct rejections of novel objects or failed retrieval (“misses”). Responding (i)–(iii) to new objects reflected false alarms.
fMRI data acquisition
Functional magnetic resonance images were acquired with a 3T Siemens Trio scanner using the standard 12-channel head coil, located at the Douglas Institute Brain Imaging Centre in Montreal, Canada. T1-weighted anatomical images were acquired after the encoding phase of the fMRI task using a 3D gradient echo MPRAGE sequence (TR = 2300 ms, TE = 2.98 ms, flip angle = 9°, 176 1 mm sagittal slices, 1×1×1 mm voxels, FOV = 256 mm). Blood oxygenated level dependent (BOLD) images were acquired using a single-shot T2*-weighted gradient echo-planar imaging (EPI) pulse sequence with TR = 2000 ms, TE = 30 ms, FOV = 256 mm. Brain volumes with 32 oblique slices of 4 mm thickness (with no slice gap) were acquired along the anterior-posterior commissural plane with in-plane resolution of 4×4 mm.
A mixed rapid event-related design was employed to collect task-related BOLD activation during performance of the memory task (see above). Visual task stimuli were generated on a computer and back-projected onto a screen in the scanner bore. The screen was visible to participants lying in the scanner via a mirror mounted within the standard head coil. Participants requiring correction for visual acuity wore plastic corrective glasses.
Data analysis
Preprocessing of fMRI data
We converted reconstructed images to NIfTI format and preprocessed them using in Statistical Parametric Mapping software version 12 (SPM12). Images from the first 10 s of scanning were discarded to allow equilibration of the magnetic field. All functional images were realigned to the first image and corrected for movement artifacts using a 6-parameter rigid body spatial transform and a PLS approach. Functional images were then spatially normalized to the MNI EPI-template using the “Old Normalize” method in SPM12 at 4×4×4 mm voxel resolution, and smoothed using an 8 mm full-width half-maximum (FWHM) isotropic Gaussian kernel. Participants with head motion exceeding 4 mm in the x, y, or z axis during encoding and retrieval were excluded from further analyses. Participants with movements that could not be sufficiently repaired, resulting in distorted brain images as judged by an examiner, were excluded from further analysis. To be included in further analyses, all participants were required to have a minimum of eight observations per event type (i.e., object recognition and source recollection).
Behavioral analyses
We performed behavioral data analyses on neuropsychological tests and episodic memory task performance using SPSS version 24 with a significance threshold of p = 0.05, Greenhouse-Geisser corrections for sphericity, and Bonferroni corrections for multiple comparisons, where applicable. Because evidence suggests meaningful sex/gender differences in the neural and behavioral correlates of episodic memory and cognition in aging [47–49], we also investigated self-reported sex as a factor in our analyses.
Neuropsychological tests
We tested for group differences in AD8, MoCA, CDR, and RBANS scores using multivariate analysis of variance (MANOVA), with genotype (APOE34, APOE33) and self-reported sex (male, female) as independent factors, and test scores as dependent variables.
Episodic memory task
We calculated mean accuracy and mean response time (RT; ms) for +APOE4 and –APOE4 individuals, as well as self-reported males and females, for all possible response types: correct object recognition (recognizing old objects but providing no or incorrect associative spatial context), correct associative spatial context recollection (correctly recalling object-location associations); source misattributions (incorrectly identifying spatial context of an old object; e.g., saying an object previously seen on the left was initially presented on the right), false alarms (incorrectly identifying new objects as old), misses (incorrectly identifying old objects as new), and correct rejections (correctly identifying new objects). These stimulus-response categories are presented in Table 1. We computed overall accuracy (i.e., hits) using the sum of correct associative spatial context recollection judgments and correct object recognitions, including source misattribution trials. We used d’, computed as overall standardized hit rate minus standardized false alarm rate, as a measure of sensitivity, and c, computed by multiplying the average of the standardized hit and false alarm rates by –1, to measure response bias [50]. We further estimated episodic memory performance by measuring the probability of source recall (pSource) and recognition (pRecog), calculated as follows:
(1) pSource = Z(sourcehits) –Z(sourcemisattributions)
(2) pRecog = Z(recognitionhits) –Z(falsealarms)
Table 1
Participant responses and their categorization based on the presented stimuli
Stimulus | Participant Response | |||
“Old, Left” | “Old, Right” | “Familiar” | “New” | |
Old, Left | Source Hit | Source Misattribution | Recognition | Miss |
Old, Right | Source Misattribution | Source Hit | Recognition | Miss |
New | False Alarm | False Alarm | False Alarm | Correct Rejection |
Similar to d’, these scores provide a relative measure of accuracy that takes into account both hits and false alarms while isolating responses based on source recall versus familiarity. We then used multivariate general linear models to evaluate between-group differences on hits, pSource and pRecog scores, and RT on hit, miss, and false alarm trials.
fMRI analyses
We used spatio-temporal PLS to conduct multivariate event-related fMRI analysis using PLSGUI software (https://www.rotman-baycrest.on.ca/index.php?section=84). We selected PLS due to its ability to identify whole-brain spatially and temporally distributed patterns of brain activity that differ across experimental conditions and/or relate to a specific behavioral measure [51; see elaboration of PLS advantages above]. We conducted two types of PLS analyses: 1) mean-centered task partial least squares (T-PLS) to identify group similarities and differences in event-related activity during successful object-location associative encoding and retrieval; and 2) behavioral PLS (B-PLS) to examine group similarities and differences in the correlations between event-related activity and performance, indexed by pSource and pRecog. Details on PLS have been published elsewhere [52, 53].
For both T-PLS and B-PLS, we averaged the event-related data for each participant across the entire time series and stacked these data by group (i.e., APOE4) and based on participants’ subsequent memory performance as follows: 1) encoding objects in which participants subsequently remembered object-location source associations (correct source recall; ENC-source); 2) encoding objects in which participants subsequently remembered only the object identified, but failed to recall spatial source information (source failure with object recognition; ENC-recog); 3) retrieval objects for which participants correctly recalled object-location source associations (RET-source); and iv) retrieval objects for which participants correctly recalled only the object identity, but failed to recall spatial source association (RET-recog). The stacked data matrix contained the fMRI data for each event onset (time lag = 0) with seven subsequent time lags, representing a total of 14 s of activation after event onset (TR = 2s * 7 = 14 s) for successfully encoded (ENC-source, ENC-recog) and successfully retrieved (RET-source and RET-recog) events. All participants analyzed had a minimum of eight correct events per event type. There was no signal at lag 0 because data were baseline corrected to the event onset. Therefore, signal in subsequent lags was expressed as percentage deviation from event onset.
Task PLS (T-PLS)
We mean centered the fMRI data column-wise during the encoding and retrieval phases of the episodic memory task, to evaluate whole-brain similarities and differences between –APOE4 and +APOE4 individuals in brain activity related to encoding and retrieval of object-location associations. PLS performs singular value decomposition on the stacked data matrix to express the cross-covariance between the fMRI data and each condition into a set of mutually orthogonal latent variables (LVs). The number of LVs produced is equivalent to the number of event types included in the analysis. Thus, this analysis yielded eight LVs (4 event-types * 2 groups). Each LV comprises: 1) a singular value reflecting the amount of covariance accounted for by the LV; 2) a design salience with a set of contrasts representing the relationship between tasks in each group and the pattern of brain activation; and 3) a singular image representing the numerical weights assigned to each voxel at each TR/time lag (i.e., the “brain salience” representing the contribution of a region at each TR), yielding a spatio-temporal pattern of whole-brain activity for the entire time series. Design saliences and brain weights can be either positive or negative, and indicate whether activity in the associated voxels are positively or negatively associated with the correlation profile: positive brain weights (depicted as warm-colored regions in the singular images) are positively correlated to positive design saliences, whereas negative brain weights (depicted as cool-colored regions in the singular images) are positively correlated with negative design saliences and vice-versa [52, 53]. Thus, the pattern of whole brain activity shown in the singular image is symmetrically associated with the contrast effect identified by the design salience plot.
Behavioral PLS
We used B-PLS to analyze whole-brain similarities and differences in brain activity directly correlated with pSource and pRecog during encoding and retrieval between +APOE4 and –APOE4 individuals. We stacked the behavioral vector containing pSource and pRecog in the same order as the fMRI data matrix (i.e., participant within group). As in the T-PLS, B-PLS performed singular value decomposition of the stacked cross-correlation matrix to yield eight LVs. However, rather than design saliences, B-PLS analysis yields: 1) a singular value, reflecting the amount of covariance explained by the LV; 2) a singular image consisting of positive and negative brain saliences (weights), and 3) a correlation profile depicting how participants’ retrieval accuracy (pSource or pRecog) correlates with the pattern of brain activity identified in the singular image. The correlation profile and brain saliences represent a symmetrical pairing of brain-behavior correlation patterns for each group to a pattern of brain activity, respectively. As with the T-PLS analysis, brain saliences can have positive or negative values, and reflect whether activity in a given voxel is positively or negatively associated with the correlation profile depicted. Thus, negative correlations on the correlation plot indicate a negative correlation between performance and positive brain weights (depicted as warm-colored regions in the singular image), but a positive correlation between performance and negative brain weights (depicted as cool-colored regions in the singular image). Conversely, positive correlations indicate a positive correlation between performance and positive brain weights, but a negative correlation between performance and negative brain weights.
We assessed the significance of each LV in the T-PLS and B-PLS through 1000 permutations involving resampling without replacement from the data matrix to reassign the order of event types within participant. We determined the stability of the brain saliences using 500 bootstrap samples for the standard errors of voxel saliences for each LV, sampling participants with replacement while maintaining the order of event types for all participants. We considered only voxels with brain saliences ≥3.28 times greater than the bootstrap standard error (approximately corresponding to p = 0.001) and a minimum spatial extent of 10 contiguous voxels as stable.
We computed temporal brain scores for each significant LV to determine the time lags with the strongest correlation profile. Temporal brain scores reflect how strongly each participant’s data reflected the pattern of brain activity expressed in the singular image in relation to its paired correlation profile, at each time lag. We report only peak coordinates from time lags at which the correlation profile was maximally differentiated within the temporal window sampled (lags 2–5; 4–10 s after event onset). We converted these peak coordinates to Talairach space using the icbm2tal transform [54] as implemented in GingerAle 2.3 [55]. Because our acquisition incompletely acquired the cerebellum, peak coordinates from this region are not reported. We used the Talairach and Tournoux atlas [56] to identify the Brodmann area (BA) localizations of significant activation sites.
RESULTS
After removing age outliers (i.e., individuals with age above or below 2SD from the mean), we analyzed data from 165 participants, including 98 –APOE4 and 67 +APOE4 individuals. Participant demographics and mean motion during fMRI are shown in Table 2. Evidence suggests that proximity to the age of AD diagnosis in a parent or sibling may predict the onset of dementia symptomatology [32]. We therefore included estimated years to AD symptom onset (EYO), calculated as the age of AD onset in the earliest affected family member subtracted from the participant’s current age, as part of these baseline analyses.
Table 2
Demographic background and mean fMRI motion (in mm) of participants included in behavioral analyses (n = 165) based on APOE4 group, represented as mean values±standard deviation
Years of Age | Age Range | n Women:Men | Years of Education | EYO | Motion | |
–APOE4 | 63.43±4.51* | 55.30–73.14 | 73 : 25 | 14.80±3.34 | 9.93±8.08 | 0.025±0.067 |
+APOE4 | 61.98±3.91 | 55.13–72.62 | 51 : 16 | 15.48±3.46 | 9.19±7.66 | 0.027±0.066 |
*p = 0.033.
We found that –APOE4 individuals were significantly older (t163 = 2.15, p = 0.033). We therefore included age as a covariate in our analyses of performance below.
Neuropsychological performance
Participant scores on the neuropsychological tests are presented in Table 3. Although groups were balanced in their sex distributions (χ2 = 0.057, p = 0.812), we found a significant correlation between sex and performance on the MoCA (rpb = 0.227, p = 0.003) and CDR (rpb = –0.183, p = 0.019). Thus, we included sex as a factor in our analyses of neuropsychological performance factors, with age as a covariate.
Table 3
Neuropsychological performance by A) APOE4 and B) sex, represented as mean values±standard deviation. Data missing from one participant who did not receive the MoCA (n = 164) and seven participants who did not receive the RBANS (n = 158)
A) | AD8 | CDR | MoCA | Immediate memory | Visuospatial construction | Language | Attention | Delayed memory | RBANS total |
–APOE4 | 0.12±0.41 | 0.015±0.09 | 27.68**±1.62 | 104.34±10.9 | 94.76±12.3 | 98.40±10.19 | 106.03±15.74 | 104.41±8.73 | 101.62±9.36 |
+APOE4 | 0.24±0.61 | 0.007±0.06 | 28.38±1.45 | 103.73±11.23 | 94.66±13.12 | 101.66±8.69 | 105.41±13.78 | 103.47±10.95 | 102.05±10.73 |
B) | |||||||||
Women | 0.15±0.45 | 0.004±0.05 | 28.17*±1.45 | 103.87±11.36 | 95.53±12.35 | 99.78±10.09 | 106.39±15.07 | 104.36±9.87 | 102.22±10.14 |
Men | 0.24±0.62 | 0.037±0.13 | 27.34±1.81 | 104.75±9.95 | 92.30±13.26 | 99.55±8.63 | 103.97±14.58 | 103.08±9.08 | 100.53±9.20 |
*p = 0.011; **p = 0.006.
MoCA total score
We found a significant main effect of APOE4 (F(1,159) = 10.07, p = 0.002, ηp2 = 0.06) and of sex (F(1,159) = 6.45, p = 0.011, ηp2 = 0.04), but no interaction between the two (Fig. 1). Follow up analyses revealed that +APOE4 individuals had significantly higher MoCA scores compared to –APOE4 individuals (t162 = 2.81, p = 0.006), and that women had significantly higher MoCA scores compared to men (t58.11 = 2.66, p = 0.01). Notably, nine –APOE4 individuals scored below the clinical cutoff of 26 compared to only two +APOE4 individuals, although this difference was not significant (χ2 = 1.31, p = 0.25). Conversely, the proportion of women who scored below 26 on the MoCA (5/119 = 4%) was significantly lower than the proportion of men (7/34 = 21%; χ2 = 7.77, p = 0.005).
Fig. 1
MoCA scores by (a) APOE4 carrier status and (b) self-reported sex. Shaded margins indicate 95% confidence intervals.
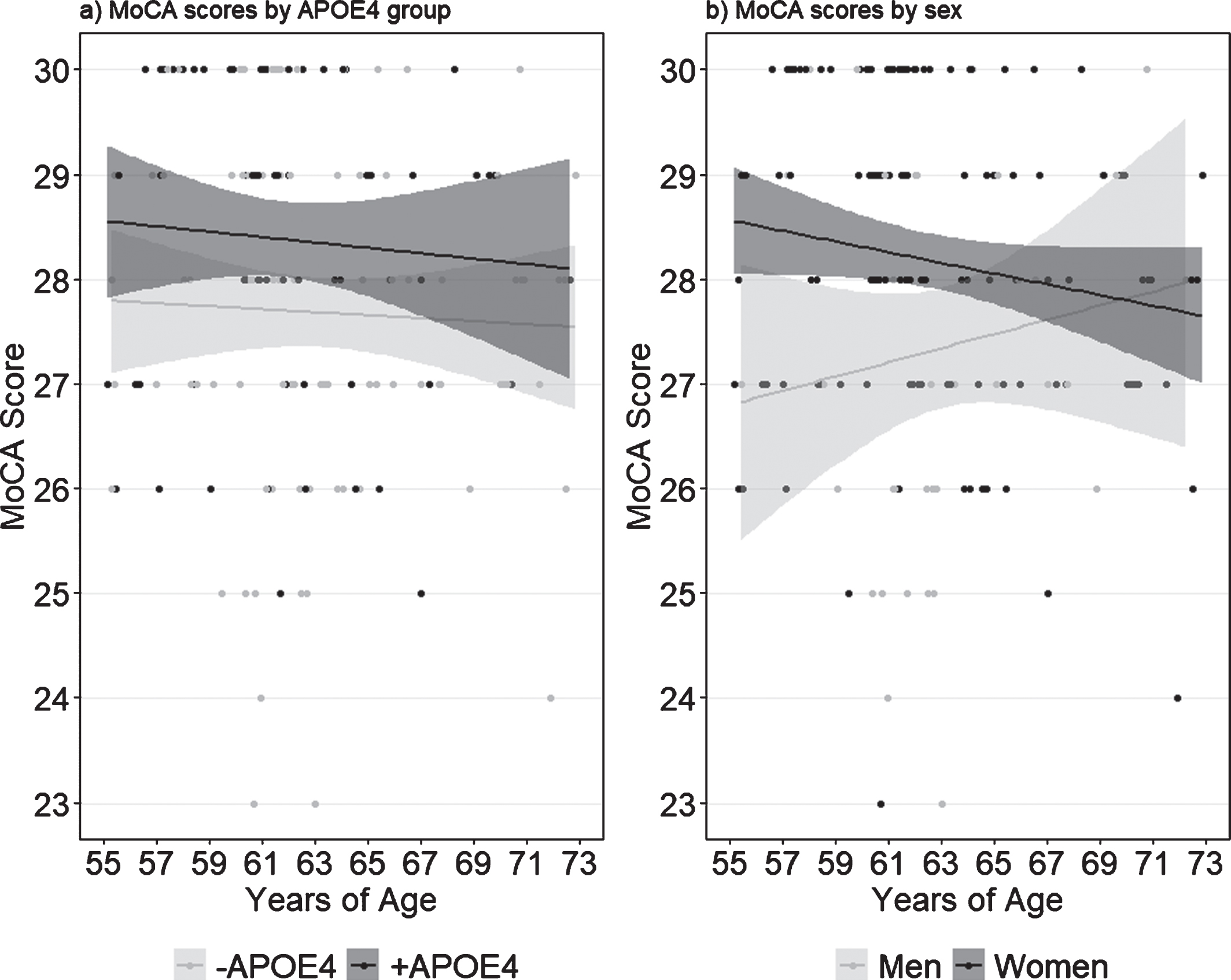
RBANS scores
Multivariate ANOVA on the RBANS subtests revealed no significant effect of APOE4 (Wilk’s λ = 0.971, F(6,148) = 0.744, p = 0.615), of sex (Wilk’s λ = 0.981, F(6,148) = 0.487, p = 0.817), or an interaction between the two (Wilk’s λ = 0.933, F(6,148) = 1.763, p = 0.11), on RBANS test scores.
AD8 and CDR total scores
We found a main effect of APOE4 (F(1,160) = 6.24, p = 0.014, ηp2 = 0.038; ns after removing sex as a factor), and an interaction between APOE4 and sex (F(1,160) = 4.92, p = 0.028, ηp2 = 0.030; ns after correcting for multiple comparisons) on AD8 total score. We also found a main effect of sex on CDR total score (F(1,160) = 5.21, p = 0.024, ηp2 = 0.032). This effect did not remain significant after correcting for multiple comparisons; we therefore performed no follow up analyses on CDR scores.
fMRI: Episodic memory task performance
Behavioral results from the object-location associative memory task are shown in Table 4. Multivariate ANOVA with APOE and sex as between subjects factors, and age as a covariate, revealed no significant group differences or interaction effects in measures of task accuracy (Wilk’s λ = 0.969, F(5,156) = 0.983, p = 0.43) or RT (Wilk’s λ = 0.972, F(7,147) = 0.607, p = 0.749) at baseline. Similarly, we found no effect of APOE (Wilk’s λ = 0.990, F(2,159) = 0.816, p = 0.44), of sex (Wilk’s λ = 0.982, F(2,159) = 1.466, p = 0.23), or an interaction between the two (Wilk’s λ = 1.0, F(2,159) = 0.01, p = 0.99) when examining pSource (i.e., correct associative spatial context recollection) and pRecog (i.e., correct object recognition) as performance outcomes (Fig. 2). Our analyses nevertheless revealed significant differences in RT based on response type (F(3.99,610.8) = 3.37, p = 0.01, ηp2 = 0.022; Fig. 3). In all groups, participants had significantly longer RT for recognition (i.e., source failure) and FA trials compared to source hits, source misattributions, misses, and correct rejections (t164≥6.45, p≤0.0001), and significantly faster RT for correct rejections compared to all other trials (t164≥4.00, p≤0.0001). Of trials presenting “old” (i.e., previously viewed) objects, participants had the fastest RT for source hits (t159≥8.98, p≤0.0001).
Table 4
Performance on the episodic memory task (n = 165). Errors are standard deviations
A) Mean accuracy metrics by response type and measures of signal detection theory | |||||||||||
Total hit rate | Source hits | Recognitions | Source misattributions | Misses | False alarms | Correct rejections | d’ | pSource | pRecog | c | |
–APOE4 | 0.84±0.11 | 0.54±0.15 | 0.18±0.12 | 0.13±0.07 | 0.16±0.11 | 0.16±0.12 | 0.84±0.14 | –0.12±1.43 | –0.05±1.39 | –0.14±1.28 | –0.09±.70 |
+APOE4 | 0.84±0.13 | 0.54±0.17 | 0.18±0.12 | 0.12±0.06 | 0.16±0.13 | 0.12±0.10 | 0.88±0.10 | 0.20±1.43 | 0.15±1.27 | 0.21±1.29 | 0.20±1.43 |
B) RT (s) by response type | |||||||||||
Source hits | Recognition hits | Source misattributions | Misses | False alarms | Correct rejections | ||||||
–APOE4 | 1.72±0.30 | 2.45±.59 | 2.07±0.42 | 2.04±0.49 | 2.50±.054 | 1.65±0.34 | |||||
+APOE4 | 1.73±0.29 | 2.44±.51 | 2.17±0.39 | 1.99±0.40 | 2.41±0.50 | 1.60±0.25 |
Fig. 2
Performance on the episodic memory task by APOE4 carrier status (a,c) and self-reported sex (b,d). Probability of source recall represents standardized source misattribution rate subtracted from standardized source hit rate. Probability of recognition represents standardized false alarm rate subtracted from standardized object recognition rate. Shaded margins indicate 95% confidence intervals.
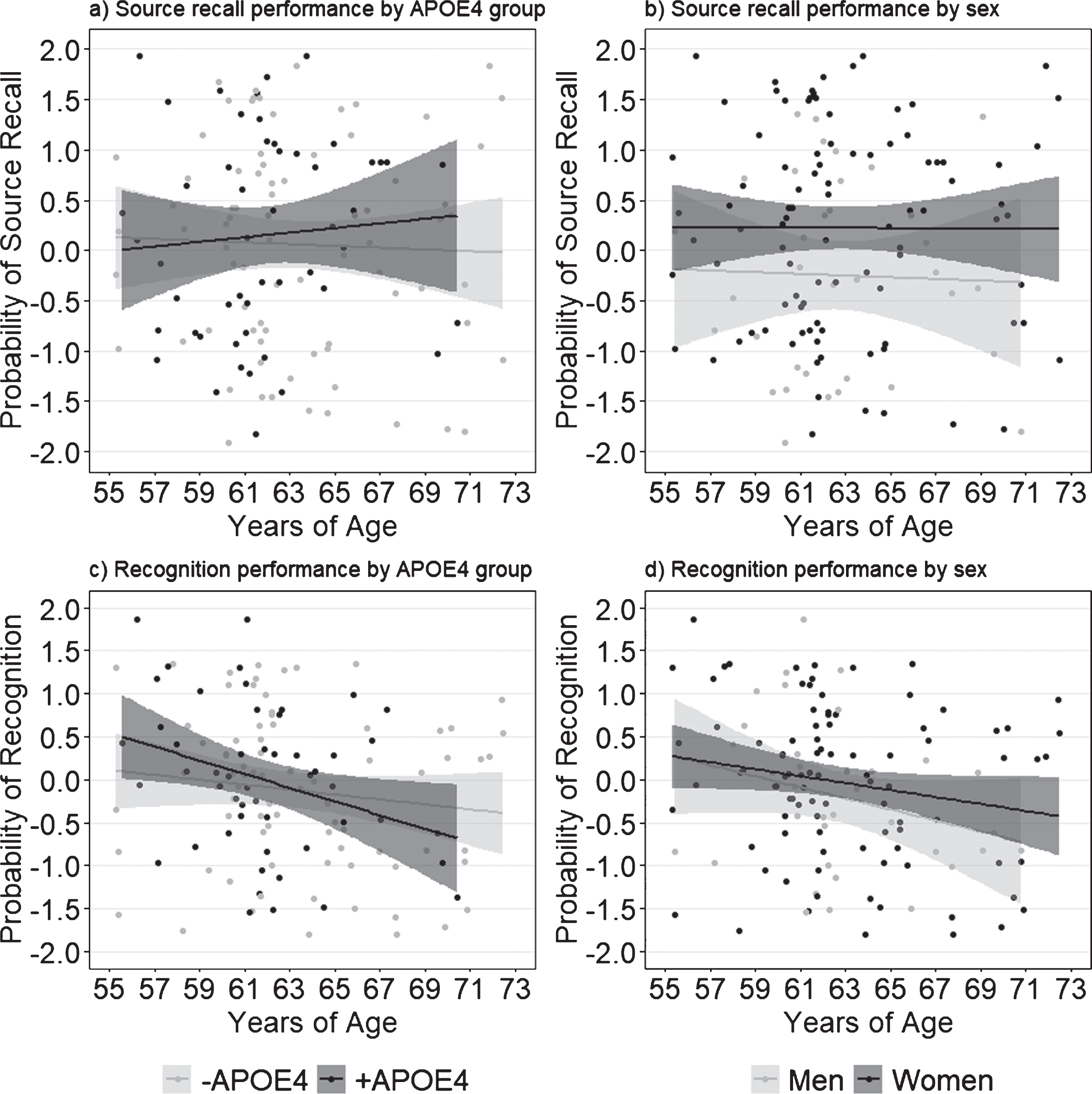
Fig. 3
Response times on the episodic memory task by response category. On average, participants in all groups responded fastest for correct rejections, followed by correct object-location source associations, and slowest for object recognitions and false alarms. Shaded margins indicate 95% confidence intervals.
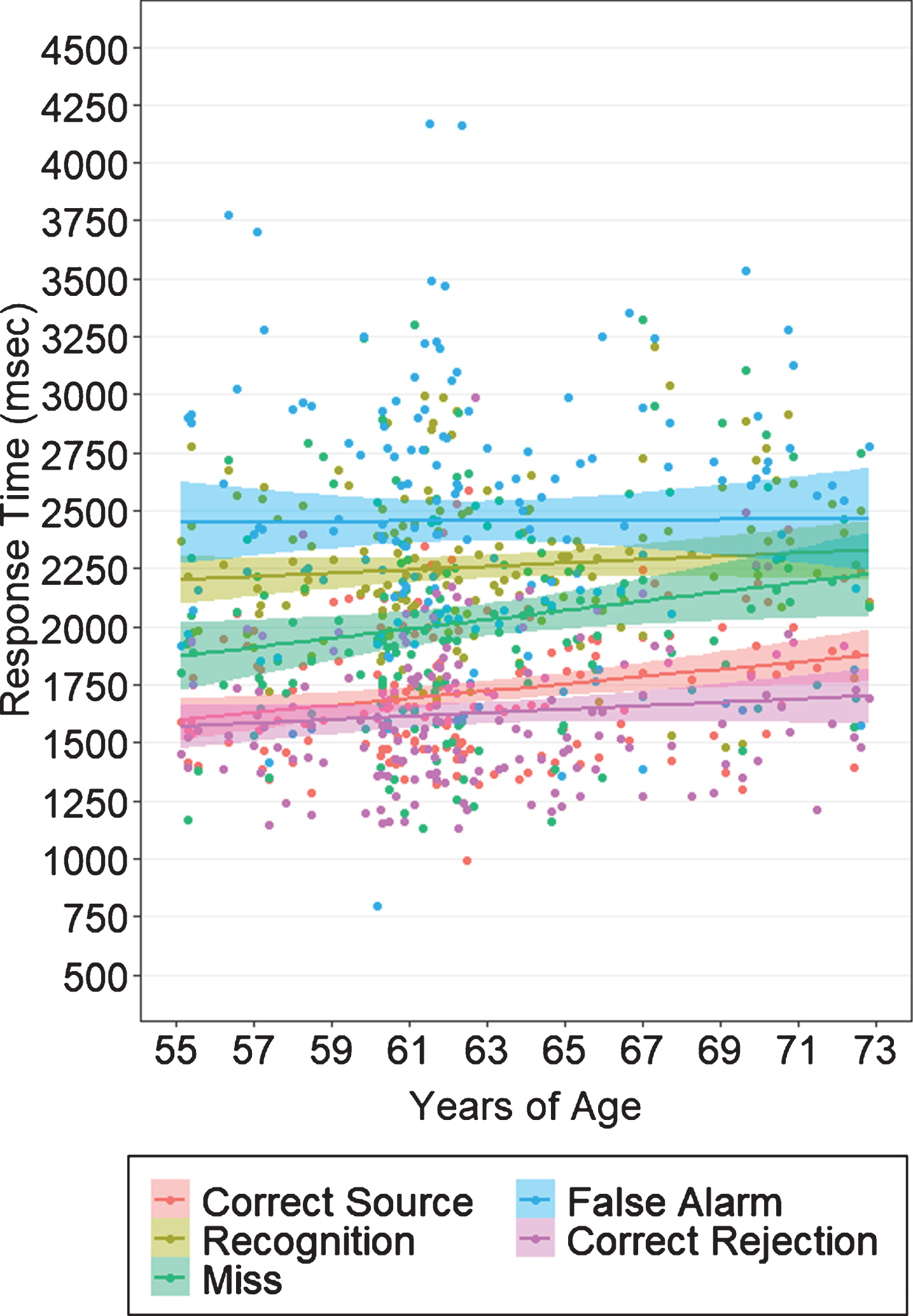
fMRI results
T-PLS
The PLS analysis yielded two significant LVs (p < 0.0001). The first significant LV accounted for 66.7% of the cross-block covariance and identified brain regions in which activity significantly differed during encoding, compared to retrieval, in both +APOE4 and –APOE4 groups (Fig. 4A). Table 5 lists the local maxima from LV 1. In both APOE4 groups, positively-weighted brain regions were more active during retrieval, compared to encoding, whereas the negatively-weighted brain regions were more active during encoding, compared to retrieval, across all groups. More activity was detected in the left ventromedial/orbitofrontal/anterior cingulate, and the left lateral middle temporal cortex during encoding, compared to retrieval. Conversely, during retrieval there was more activity in the bilateral claustrum, cingulate gyrus, inferior frontal gyrus, and precuneus, as well as the left middle frontal gyrus, compared to encoding.
Table 5
Local maxima revealed for LV1 of the T-PLS analysis. We report only lags 2–5, and clusters with a spatial threshold of at least 10 continuous voxels
Temporal lag | Bootstrap ratio | Spatial extent (voxels) | Talairach coordinates | Gyral location | Brodmann area | ||
X | Y | Z | |||||
Positive Salience Regions | |||||||
Right Hemisphere | |||||||
2,3,4,5 | 17.42 | 6781 | 25 | 21 | 3 | Claustrum | 45 |
2 | 10.23 | 225 | 13 | –56 | 17 | Posterior Cingulate | 23 |
5 | 9.29 | 162 | 2 | –74 | 48 | Precuneus | 7 |
4 | 8.06 | 63 | 10 | –6 | 4 | Thalamus | |
5 | 7.68 | 75 | 39 | –59 | 46 | Inferior Parietal Lobule/Precueus | 7 |
4 | 7.19 | 33 | 6 | –91 | –11 | Lingual Gyrus | 18 |
2 | 7.16 | 19 | 6 | 13 | 42 | Cingulate Gyrus | 32 |
2 | 7.01 | 32 | 35 | –83 | 29 | Superior Occipital Gyrus | 19 |
4 | 6.61 | 14 | 29 | 53 | 24 | Superior Frontal Gyrus | 10 |
Left Hemisphere | |||||||
4 | 12.69 | 2183 | –13 | –73 | 40 | Precuneus | 7 |
3 | 12.20 | 495 | –20 | –91 | –5 | Lingual Gyrus | 17 |
2,4 | 10.48 | 253 | –9 | 13 | 45 | Medial Frontal Gyrus | 32 |
2 | 10.12 | 94 | –39 | –79 | 29 | Superior Occipital Gyrus | 19 |
4 | 10.04 | 98 | –30 | 18 | –2 | Claustrum | |
2,3,4 | 8.79 | 123 | –46 | 14 | 34 | Middle Frontal Gyrus | 9 |
4 | 8.68 | 74 | –5 | –30 | 27 | Posterior Cingulate | 23 |
2 | 7.64 | 38 | –5 | –23 | 27 | Cingulate Gyrus | 23 |
4 | 7.29 | 25 | –16 | –8 | –4 | Lentiform Nucleus | Medial Globus |
Pallidus | |||||||
4 | 6.98 | 20 | –31 | –88 | 3 | Middle Occipital Gyrus | 18 |
3 | 6.63 | 9 | –1 | –24 | –41 | ||
Negative Salience Regions | |||||||
Right Hemisphere | |||||||
5 | –7.23 | 61 | 21 | –92 | 3 | Lingual Gyrus | 17 |
Left Hemisphere | |||||||
3,4 | –8.27 | 58 | –1 | 30 | –11 | Medial Frontal | 11 |
3 | –7.91 | 23 | –60 | –11 | –19 | Inferior Temporal Gyrus | 20 |
2,3 | –7.67 | 139 | –39 | –34 | 62 | Postcentral Gyrus | 2 |
2,5 | –7.48 | 80 | –35 | –27 | 66 | Precentral Gyrus | 4 |
5 | –7.15 | 28 | –4 | 26 | –15 | Medial Frontal Gyrus | 11 |
2 | –6.73 | 5 | –64 | –10 | 9 | Superior Temporal Gyrus | 22 |
5 | –6.34 | 12 | –46 | –84 | –4 | Inferior Occipital Gyrus | 19 |
LV2 of the T-PLS accounted for 13.78% of the cross-block covariance. The design salience plot and singular image presented in Fig. 4B indicates that this LV identified brain regions that were differentially activated during correct retrieval of object-location associations, compared to objects alone (i.e., source hits versus source misattributions and failures) in both APOE groups. The local maxima from this LV are presented in Table 6. This LV identifies mainly negatively-weighted brain regions and indicates that activity was greater in middle occipital, inferior parietal lobule, caudate, and temporal gyri, including the left parahippocampus and right hippocampus, during object-location associative retrieval, compared to object only retrieval. In contrast, activity was greater in the bilateral inferior frontal gyrus, cingulate gyrus, left middle frontal gyrus, and right inferior parietal lobule during object only retrieval, compared to object-location retrieval.
Fig. 4
Design salience plot and singular image representing brain activity patterns by condition for (a) LV1 and (b) LV2, revealed by the T-PLS analysis. LEFT: Error bars on design salience plots represent 95% confidence intervals. Positive brain scores indicate conditions in which activity was greater in positive brain salience regions (shown in red in the singular images) and vice versa. Negative brain scores indicate conditions in which activity was greater in negative brain salience regions (shown in red in the singular images) and vice versa. RIGHT: Singular images were thresholded at a bootrstrap ratio of±3.5, p < 0.001. Red brain regions represent positive brain saliences; blue regions represent negative brain saliences. Activations are presented on template images of the lateral and medial surfaces of the left and right hemispheres of the brain using Caret software (http://brainvis.wustl.edu/wiki/index.php/Caret:Download).
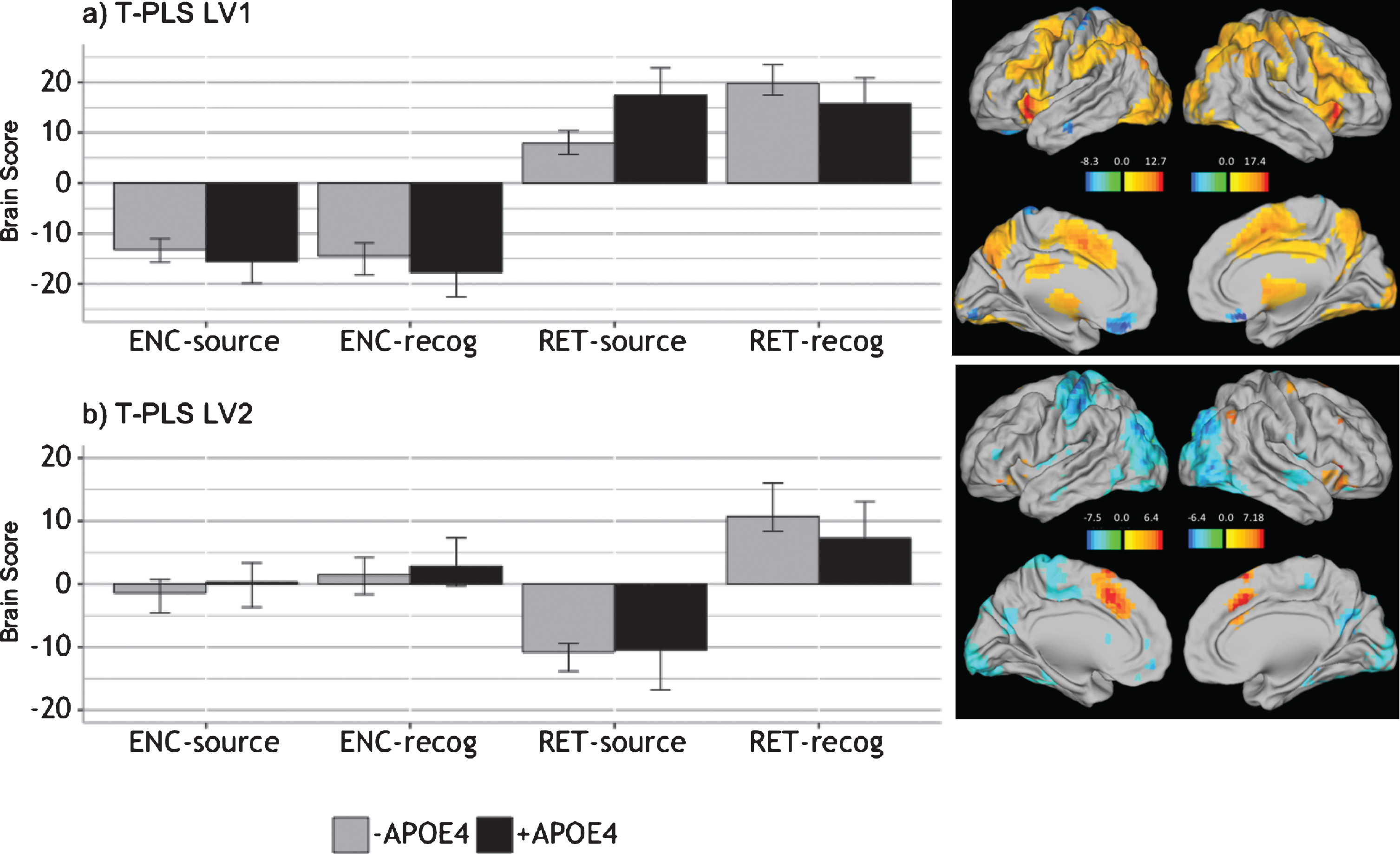
Table 6
Local maxima revealed for LV2 of the T-PLS analysis. We report only lags 2–5, and clusters with a spatial threshold of at least 10 continuous voxels
Temporal lag | Bootstrap ratio | Spatial extent (voxels) | Talairach coordinates | Gyral location | Brodmann area | ||
X | Y | Z | |||||
Positive Salience Regions | |||||||
Right Hemisphere | |||||||
4,5 | 7.18 | 306 | 2 | 17 | 42 | Cingulate Gyrus | 32 |
4, 5 | 5.87 | 127 | 40 | 21 | 0 | Inferior Frontal Gyrus | 47 |
5 | 4.75 | 39 | 46 | –62 | 42 | Inferior Parietal Lobule | 40 |
4,5 | 4.55 | 32 | 32 | –15 | 61 | Precentral Gyrus | 6 |
Left Hemisphere | |||||||
3,4 | 6.39 | 160 | –45 | 17 | 2 | Inferior Frontal Gyrus | 47 |
5 | 4.84 | 113 | –45 | 33 | –8 | Inferior Frontal Gyrus | 47 |
Negative Salience Regions | |||||||
Right Hemisphere | |||||||
3,4,5 | –6.37 | 127 | 14 | 23 | 18 | Caudate | |
2 | –5.96 | 192 | 28 | –77 | 37 | Precuneus | 19 |
2,3,4 | –5.37 | 82 | 51 | –8 | –6 | Superior Temporal Gyrus | 22 |
2 | –5.40 | 332 | 43 | –69 | –2 | Inferior Temporal Gyrus | 37 |
2 | –4.86 | 100 | 47 | –3 | 12 | Insula | 13 |
3 | –4.79 | 35 | 54 | –10 | 44 | Postcentral Gyrus | 3 |
4 | –4.78 | 24 | 32 | –35 | –6 | Hippocampus | |
2,5 | –4.05 | 53 | 29 | –30 | –20 | Parahippocampal Gyrus | 36,37 |
2 | –3.91 | 19 | 13 | –12 | 36 | Cingulate Gyrus | 24 |
Left Hemisphere | |||||||
2,3,4 | –7.54 | 953 | –35 | –30 | 62 | Precentral Gyrus | 4 |
3 | –7.34 | 2294 | –31 | –79 | 22 | Middle Occipital Gyrus | 19 |
2 | –6.62 | 1747 | –39 | –32 | 47 | Inferior Parietal Lobule | 40 |
3,5 | –6.23 | 175 | –20 | 24 | 13 | Caudate | |
4 | –5.99 | 493 | –23 | 32 | 3 | ||
3 | –5.43 | 70 | –60 | –12 | –5 | Middle Temporal Gyrus | 21 |
5 | –5.03 | 74 | 21 | –44 | 15 | 5 | |
5 | –4.85 | 35 | –17 | –42 | 65 | Postcentral Gyrus | 5 |
2,4,5 | –4.79 | 29 | –38 | –34 | –10 | Parahippocampal Gyrus | 36 |
2 | –4.50 | 95 | 29 | 32 | 4 | ||
2 | –4.32 | 16 | –16 | 43 | 12 | Medial Frontal Gyrus | 10 |
2 | –4.31 | 11 | –56 | –7 | –15 | Inferior Temporal Gyrus | 20 |
2,5 | –4.07 | 10 | –19 | –6 | –29 | Uncus | 36 |
5 | –4.01 | 10 | –31 | –73 | 0 | Lingual Gyrus | 18 |
2 | –3.94 | 22 | –12 | –21 | 13 | Thalamus |
B-PLS
After removing performance outliers based on pSource and pRecog, we analyzed data from 129 participants (78 –APOE4 and 51 +APOE4). The B-PLS yielded one significant LV (p = 0.008), which accounted for 23.82% of the cross-block covariance (Fig. 5). Table 7 lists the local maxima for positively- and negatively-weighted brain regions. This LV identified brain regions with group differences in the correlation between pRecog performance and encoding activity for objects which participants subsequently retrieved only object identity but failed to recall the spatial source information (ENC-recog). In addition, this LV identified brain regions in which activity during object-location source retrieval (RET-source) correlated with pSource performance in –APOE4 individuals. Specifically, in these individuals, activity in positively-weighted brain regions during ENC-recog events was negatively correlated with pRecog scores, and activity in these same regions during RET-source events was positively correlated with pSource scores. In addition, in –APOE4 individuals, activity in negatively-weighted brain regions during ENC-recog events was positively correlated with pRecog scores and activity in these regions during RET-source was negatively correlated with pSource scores. Positively-weighted brain regions included right thalamus, right supramarginal gyrus and left insula (Table 7). Negatively-weighted brain regions included bilateral occipito-temporal cortices, uncus, caudate, and anterior-medial PFC, as well as left parahippocampal gyrus. Therefore, in –APOE4 individuals, activity in a traditional episodic memory network (negatively-weighted brain regions) during encoding predicted subsequent object-only retrieval (i.e., source failures), and less activation in these regions at retrieval predicted better source performance.
Fig. 5
Correlations between brain activity and task performance by condition, revealed by the B-PLS analysis. LEFT: Bars represent brain-behavior correlations for each group, by condition. Positive correlations indicate conditions in which performance was positively associated with activity in positive brain salience regions (shown in red in the singular images) and vice-versa. Negative correlations indicate conditions in which performance was positively associated with activity in negative brain salience regions (shown in blue in the singular images) and vice-versa. Error bars represent 95% confidence intervals. RIGHT: The singular image thresholded at a bootstrap ratio of±3.5, p < 0.001. Red brain regions represent positive brain saliences; blue regions represent negative brain saliences. Activations are presented on template images of the lateral and medial surfaces of the left and right hemispheres of the brain using Caret software (http://brainvis.wustl.edu/wiki/index.php/Caret:Download).
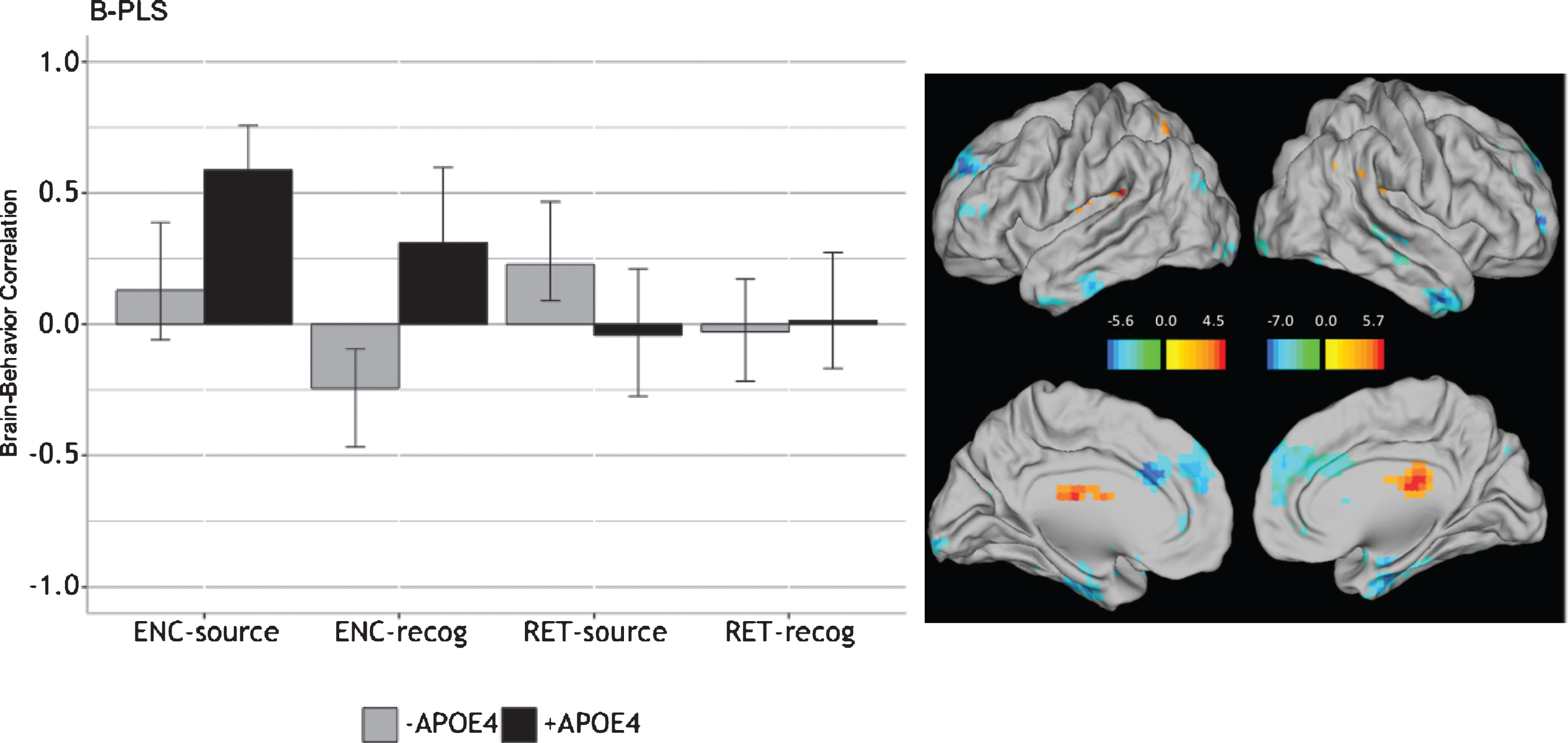
Table 7
Local maxima revealed by the B-PLS analysis. We report only lags 2–5, and clusters with a spatial threshold of at least 10 continuous voxels
Temporal lag | Bootstrap ratio | Spatial extent (voxels) | Talairach coordinates | Gyral location | Brodmann area | ||
X | Y | Z | |||||
Positive Salience Regions | |||||||
Right Hemisphere | |||||||
3, 5 | 5.69 | 63 | 58 | –53 | 29 | Supramarginal Gyrus | 39,40 |
3 | 5.46 | 319 | 6 | –26 | 20 | Thalamus | |
Left Hemisphere | |||||||
3 | 4.50 | 17 | –38 | –33 | 19 | Insula | 13 |
Negative Salience Regions | |||||||
Right Hemisphere | |||||||
2,3,5 | –7.02 | 442 | 6 | 48 | 38 | Medial Frontal Gyrus | 6,8,10 |
2 | –5.28 | 42 | 44 | 5 | –27 | Middle Temporal Gyrus | 21 |
3,4,5 | –5.16 | 155 | 22 | –2 | –28 | Uncus | 28,36, Amygdala |
3 | –4.96 | 47 | 14 | 17 | 6 | Caudate | Caudate Body |
5 | –4.84 | 12 | 43 | –31 | –2 | Superior Temporal Gyrus | 22 |
5 | –4.22 | 34 | 21 | –87 | –11 | Middle Occipital Gyrus | 18 |
Left Hemisphere | |||||||
3 | –5.61 | 50 | –16 | 13 | 16 | Caudate | Caudate Body |
3,5 | –5.44 | 143 | –34 | –14 | –27 | Uncus | 20,28 |
2 | –4.86 | 23 | –20 | 25 | 35 | Middle Frontal Gyrus | 8 |
5 | –4.71 | 18 | –53 | –18 | –24 | Fusiform Gyrus | 20 |
3 | –4.39 | 14 | –9 | –90 | –12 | Lingual Gyrus | 18 |
3 | –4.35 | 64 | –23 | 34 | 22 | Medial Frontal Gyrus | 9 |
3 | –4.33 | 16 | –2 | 15 | 67 | Superior Frontal Gyrus | 6 |
3 | –3.97 | 11 | –41 | 13 | –31 | Superior Temporal Gyrus | 38 |
3 | –3.89 | 13 | 43 | –39 | –2 | ||
2 | –3.78 | 10 | –27 | –46 | –1 | Parahippocampal Gyrus | 19 |
Individuals with +APOE4 genotype exhibited the opposite pattern of brain activity-behavior correlations to –APOE4 individuals at encoding, particularly for encoding of subsequently recognized objects, and did not exhibit significant brain-behavior correlations at retrieval. Specifically, in +APOE4 individuals, activity within right thalamus, right supramarginal gyrus, and left insula during ENC-recog and ENC-source correlated with better subsequent memory for both event types. In contrast, encoding activity in more traditional episodic memory-related areas (negatively-weighted regions) was negatively correlated with subsequent memory in these adults.
Taken together these results indicate that individuals with +APOE4 versus –APOE4 genotype engaged distinct patterns of brain activity at encoding to support subsequent memory. In addition, –APOE4 adults appeared to exhibit an encoding/retrieval flip in the regions supporting memory.
Post-hoc regions of interest (ROI) analyses
We investigated activation profiles for the ROI displaying the highest peaks during encoding in our B-PLS analysis and consistent with our TPLS results (Fig. 6). Multivariate general linear models evaluating group differences in ROI activation, averaged across lags 2–5, indicated no significant group differences during ENC-source or ENC-recog.
Fig. 6
Mean activation across lags 2–5 at encoding for ROI identified via B-PLS. The plots show similar activation patterns for both APOE4 groups during episodic encoding, supporting our T-PLS results. Error bars represent 95% confidence intervals.
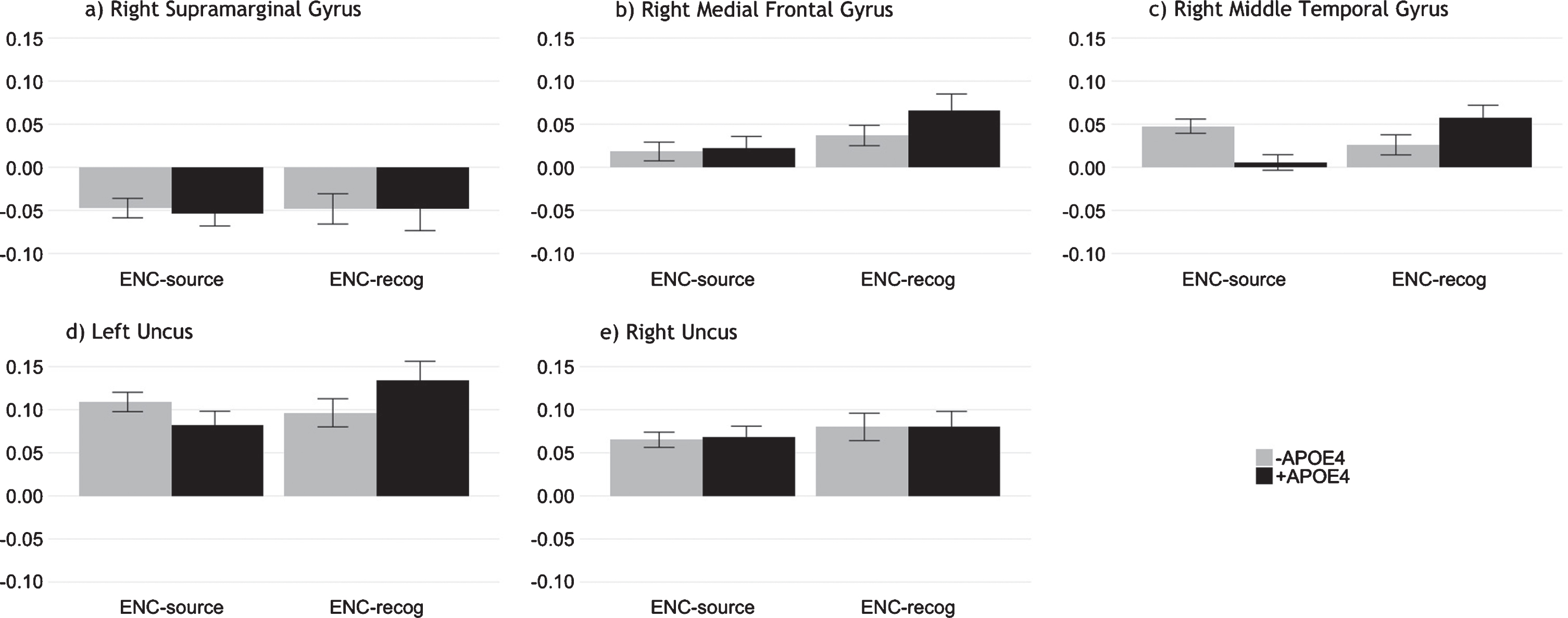
DISCUSSION
The PREVENT-AD study aimed to identify signs of AD pathogenesis in pre-symptomatic older adults with elevated risk of developing late-onset sporadic AD [37]. Because neural changes may occur decades before the onset of clinical AD symptoms [8, 9], PREVENT-AD used sensitive biomarkers such as brain imaging, biochemistry, metabolic, and cognitive measures to explore characteristic changes during disease progression. The PREVENT-AD cohort study directly addressed several shortcomings of previous investigations of prodromal AD [57], including use of sufficiently large sample size, appropriate sample composition (e.g., older adults with family history of AD who may be in an early, pre-symptomatic phase of the disease), longitudinal design, and comprehensive cognitive and biological outcomes. Furthermore, whereas previous studies of AD have often included population samples with mixed family history, here we were able to account for this potential confound by studying a cohort who all had family history of AD.
Here we drew on baseline data from the PREVENT-AD cohort to examine the potential influence of having an APOE4 allele on episodic memory function, over and above the influence of family history. Moreover, few studies have assessed the relationship between APOE4 and brain activity from the whole-brain perspective. Instead studies have often used univariate approaches and focused on specific regions or networks of interest (i.e., the medial temporal lobe system) that are implicated in AD pathology [58–62]. These studies have contributed significantly to our understanding of memory-related dysfunction in preclinical samples with the APOE4 risk factor for AD. The current study aimed to expand on this extant literature by using a data-driven multivariate approach, PLS, to compare brain activity related to episodic memory encoding and retrieval between –APOE4 and +APOE4 individuals with family history of AD. In addition, contrary to previous studies focusing primarily on recognition/novelty, we employed a novel episodic memory task distinguishing object-location source association from recognition via a single response.
Few behavioral group differences
Overall, we found that both –APOE4 and +APOE4 individuals performed well on neuropsychological tests and the fMRI episodic memory task. This result aligns with previous studies that have shown no significant behavioral differences in cognitive performance on the basis of APOE4 status in younger [63] and healthy older adults of similar demographic background [64]. These behavioral findings also echo our previous work, in which we found no effect of APOE4 on episodic memory in middle-aged adults at risk of AD using a similar task ([29] but see [57, 65] for evidence of APOE4 effects on memory in mid-adulthood).
Interestingly, we observed a significant group difference in MoCA scores in the current study, with +APOE4 individuals scoring higher than –APOE4 individuals, on average. Given the high sensitivity of the MoCA for detecting mild cognitive impairment [66], lower MoCA performance among the –APOE4 group may support theories suggesting that carrying an APOE4 allele may paradoxically benefit cognitive function in early and midlife [67]. However, the majority of participants in both groups scored well above the MoCA score cutoff of 26, which is argued by some to be overly conservative even in highly educated older adults [68]. This suggests that most participants in both groups had good cognitive performance, at least as indicated by the MoCA. In addition, evidence suggests a greater effect of APOE4 in women compared to men [69]; this effect may have been masked in the current study, as most of our participants were women. Moreover, both +APOE4 and –APOE4 individuals in this study were relatively young compared to other studies of AD, with median ages of 61 and 62 years (sometimes referred to as “young-old”), respectively. Notably, participants were an estimated 9–10 years away from anticipated symptom onset. This stage may therefore have been too early to detect detrimental changes in cognitive performance on the basis of APOE4, at least at the group level [70].
We expected comparable episodic memory performance in both groups due to the lack of clinical symptoms in our sample. As predicted, we observed no significant effect of +APOE4 on episodic memory performance. Across both groups, participants were more likely to recollect the object-location association (54%) than recognize the object only (i.e., fail to remember the source; 18%). Nevertheless, we observed a non-significant trend whereby –APOE4 individuals had a greater number of FA and fewer correct rejections, as well as low response discriminability (i.e., negative d’, pSource, and pRecog values) and a bias towards selecting “old” (i.e., negative c value); this was not true of the +APOE4 group. Importantly, our task demanded that participants reflect on their memory of a presented object (or lack thereof) and respond in a single four-choice step. Therefore, during each retrieval trial where participants recognized an object as “old”, participants likely attempted to recall the object-location source association first, selecting that the object was simply familiar only if they failed to recall its contextual information. Supporting this interpretation, participants had longer RT during both trials where participants correctly recognized objects but failed to recall the source and where participants misidentified new objects as old (i.e., FA). Conversely, successfully encoded object-location source associations, as well as correct rejections, were identified much more rapidly. These behavioral patterns suggest that recognition trials in the present task reflect objects for which participants could not retrieve source information (i.e., source failures; see also our discussion of task activation and brain-behavior correlations below), with slightly better discriminability in +APOE4 individuals.
Few group differences in task activation patterns
Results from our mean-centered T-PLS analysis support the effectiveness of our task in selectively isolating episodic memory encoding versus retrieval (LV1) and object recognition versus source recollection (LV2). Furthermore, counter to our hypothesis, T-PLS revealed group similarities in task-related activation during episodic encoding and retrieval, as well as for object recognition versus source recollection.
Specifically, T-PLS LV1 demonstrated that individuals in both APOE4 groups activated the ventromedial frontal and temporal cortices more during encoding, compared to retrieval. In contrast, both groups activated the frontal and parietal cortices more during retrieval, compared to encoding. These results align with previous reports of increased activation in left-lateralized medial prefrontal, middle and inferior temporal, as well as primary and secondary sensory cortices (e.g., prefrontal and ventral occipito-temporal areas) for encoding objects and their location; and increased activation in broad bilateral areas including the precuneus, parietal, and medial prefrontal cortices during episodic retrieval in older adults [71, 72]. Prefrontal activity during encoding appears to correlate with successful recall in older adults [71], and may reflect greater strategic elaboration of episodic memories in older age [73].
Similarly, T-PLS LV2 indicated that, in both APOE4 groups, there was greater activity in the bilateral caudate, precuneus, and temporal cortices, as well as left parahippocampal gyrus and right hippocampus during object-location source retrieval, compared object recognition. This result is consistent with prior studies that have also reported increased activity in similar brain regions during successful encoding of contextual information [71], and overlap with areas of the “core recollection network” [74]. Notably, some studies have observed greater task-related fMRI activity in posterior parahippocampal cortex during familiarity-based retrieval, compared to recollection [75]. Yet, others have noted the importance of the posterior parahippocampal region in the encoding of spatial context or source encoding [76]. The current findings suggest that posterior parahippocampal cortex is more active during the retrieval of object-location associations, compared to recognition, and is engaged during the retrieval of spatial contextual details.
We also found greater activity in the bilateral middle and medial prefrontal cortices, angular gyrus, and inferior parietal lobule in both APOE4 groups during object recognition, compared to spatial source retrieval. This is surprising as fronto-parietal activity is often greater during source retrieval, compared to object retrieval, and thought to reflect the greater engagement of cognitive control processes [28, 77]. However, our findings suggest greater cognitive control demands during object recognition, compared to source retrieval, in the present task. The reversed pattern observed here may reflect the nature of our task design. Because the task required participants to select a single response indexing their memory of a presented object, trials in which participants indicated recognition without object-location associations may have reflected their failure to recall contextual information. This may have led to activation of fronto-parietal regions involved in cognitive control processes necessary for memory search, inhibition, and response selection [78], despite failed source recall. Our behavioral results support this interpretation: we observed longer RT during both recognition and FA trials compared to source retrieval. This suggests that participants searched for object-location source association but, in both cases, failed to retrieve the correct information.
Drawing on objective measures of source recall and recognition, our results extend previous findings, which have been largely identified using the traditional “remember versus know” paradigm that often relies on subjective report and may be less sensitive to the effects of age on episodic memory [79]. Our results further indicate partial indices of pathological memory-related brain activity in older adults at higher risk of AD: on the one hand, similar to healthy older adults, participants in the present study demonstrated activation of parahippocampal cortices during encoding of novel stimuli [24]. However, similar to high performing individuals with early dementia [80], our participants demonstrated greater prefrontal and temporal activation during episodic encoding and broad activation in areas affected by AD, including the claustrum, precuneus, inferior parietal, and middle and medial frontal cortices, during retrieval, suggesting possible preclinical indices of pathological aging.
Contrary to our hypothesis, our findings related to performance and task-related activation (i.e., T-PLS) support studies suggesting no measurable distinction in episodic memory performance and underlying brain function based on APOE4 status. Although APOE4 is linked with impaired memory, cognition, and functional activity, more rapid cognitive decline, and hippocampal atrophy in clinical populations (e.g., mild cognitive impairment and AD) and preclinical states such as subjective memory decline [81–83], effects in asymptomatic older adults are less clear. Studies have shown an association between APOE4 and accelerated memory decline later in life [70] as well as altered structure and function in medial temporal lobe circuitry [84, 85]. Moreover, using verbal episodic memory tasks, previous studies have shown greater activation of areas affected in AD (e.g., dorsolateral prefrontal cortex and superior temporal gyrus) during episodic retrieval in young adults with at higher genetic risk of AD [86]. However, although APOE4 is the strongest genetic risk factor for late-onset AD [87], presence of the allele does not appear to be a significant independent predictor of AD [57, 88]. One possible explanation for this discrepancy is family history, which may mediate APOE4 effects on memory and related brain activity. Thus, the independent influence of APOE4 may be moderated when accounting for family history, as we were able to do here. In addition, lifestyle choices (e.g., higher education level, moderate alcohol consumption) may mitigate APOE4 effects and contribute to discrepant reports of its influence on cognitive aging [64]. Although an investigation of lifestyle factors was beyond the scope of this study, high education among both APOE4 groups, coupled with our participants’ heightened awareness of dementia risk due to their family history and eagerness to engage in stimulating activities such as PREVENT-AD, may at least partly explain our lack of observed group differences in behavior and task-related fMRI.
APOE4-based differences in brain-behavior correlations
In contrast to our performance and T-PLS results, and supporting our hypothesis, our B-PLS analysis indicated group differences in correlations between brain activity at encoding and subsequent memory performance. These differences were most pronounced for subsequently recognized objects, where successful recognition performance correlated with encoding-related activity in bilateral medial and middle frontal cortices, temporal areas, uncus, and caudate, as well as left parahippocampus in APOE4 non-carriers, compared to right inferior frontal, superior temporal, and supramarginal cortices, as well as the left precuneus, claustrum, and insula in +APOE4 individuals. We also found differential reliance on brain activity supporting retrieval of object-location source associations in APOE4 carriers versus non-carriers. Whereas performance correlated negatively with activity in regions typically associated with source recollection (e.g., bilateral occipito-temporal cortices, uncus, caudate, and anterior-medial PFC, as well as left parahippocampal gyrus) in non-carriers, this correlation was positive in APOE4 carriers. Notably, we found few group differences in correlations between performance and brain activity during encoding of source memory (ENC-source), or recognition of objects in the absence of source recall (RET-recog).
In summary, we found that, when factoring out family history, +APOE4 and –APOE4 individuals exhibit similar brain activity patterns during episodic encoding and retrieval of both source and object information, but draw on different brain regions to perform the task. In particular, our results support our hypothesis that group differences in brain activity would emerge for object recognition and suggest that source recall compared to object recognition may associate with different neural substrates in –APOE4 versus +APOE4.
These patterns may reflect a difference in approach taken by APOE4 carriers and non-carriers to complete the task. For example, mnemonic strategy training appears to improve performance on face-name associative memory tasks and further correlate with increased frontoparietal activity in older adults with amnesic mild cognitive impairment [89, 90]. We did not assess strategy use in the present study and were therefore unable to test this possibility directly. However, because task instructions did not direct participants to use any particular approach or strategy, and based on the lack of behavioral differences, this seems unlikely to explain the observed findings.
An alternative possibility is that participants here demonstrated a survival effect. Despite comparable levels of education and EYO, and after accounting for age differences, +APOE4 individuals had comparable or slightly better performance on the neuropsychological tests and episodic memory task. The activation patterns and brain-behavior correlations in +APOE4 older adults may therefore support, rather than compensate for, memory performance. In this case, our results may indicate that when considering family history, +APOE4 may lead to altered neural substrates supporting memory performance but, on its own, does not contribute meaningfully to behavioral deficits. Performance benefits in +APOE4 individuals were nevertheless small and did not remain when evaluating performance in each group across age.
Given that participants were nearly 10 years away from estimated symptom onset, on average, the observed group differences in performance-related neural substrates may indicate that +APOE4 individuals were in a transitory period with respect to AD development. Supporting this possibility, we observed a non-significant trend whereby younger APOE4 carriers tended to recognize objects on the episodic memory task better than younger non-carriers, and a reversed pattern in older participants. Interestingly, we found increased activity in right frontal regions in +APOE4 individuals, in the absence of behavioral deficits and marginally better episodic memory performance. This may reflect compensatory neural recruitment of frontal regions in response to decreased performance-related activity in medial temporal regions and grey matter volume loss in critical memory areas, i.e., the hippocampus [91, 92]. Indeed, an earlier study of the PREVENT-AD cohort revealed lower hippocampal subfield volumes, extra-hippocampal white matter integrity, and cognitive performance in individuals with high tau and low amyloid-beta levels in the cerebrospinal fluid, of which nearly all were +APOE4. Moreover, compared to the –APOE4 individuals, the +APOE4 individuals in this study exhibited cerebrospinal fluid biomarker levels consistent with early signs of AD pathology, despite a lack of clinical symptomatology or indices of cognitive decline. Similarly, recent work in a larger sample of older adults who participated in PREVENT-AD suggests morphometric changes in medial prefrontal regions in +APOE4, compared to –APOE4 cohorts [93]. Together these observations are consistent with our interpretation that increased performance-related activity in frontal regions in +APOE4 individuals may reflect functional compensation.
Limitations and future directions
Despite the relatively large number of older adults who participated in the PREVENT-AD study, we excluded many from the present analyses based on genetic composition, performance, and quality of MRI scans. Thus, different patterns may have emerged with a larger sample. However, our results are largely consistent with the previous literature on cognitive performance and task-related brain activity in –APOE4 versus +APOE4 individuals. In addition, here we focused on task-related activity on successful trials because of an insufficient number of unsuccessful trials (i.e., false alarms and misses). Analyzing such trials, when possible, may provide greater insights into the mechanisms underlying changes in episodic memory function with elevated age and AD risk, as has previously been shown for recognition memory in healthy aging [94]. Such analyses should represent a greater focus in future research. Similarly, the present study aimed to examine brain activation patterns associated with a single encoding and retrieval phase, carried out over a relatively short interval. However, using a comparable paradigm, researchers have identified that activity in distinct areas, including posteromedial areas at encoding and frontal areas at retrieval, supports durable memories that last over the course of weeks [95]. Such time course likely reflects a more representative period over which memories form and consolidate over the long term, and may serve as important regions of interest in future investigations, particularly of pathological aging. Finally, the present results reflect only the baseline data from a longitudinal cohort; our future analyses will integrate these findings with performance and memory-related brain activity from follow-up assessments.
Conclusions
Here we examined the relationship between memory performance and brain activity based on APOE4 carrier status in older adults with family history of AD. We found different correlations between brain activity and subsequent memory performance in APOE4 carriers compared to non-carriers, despite similar neuropsychological and episodic memory performance as well as task-related activation during episodic encoding and retrieval for both recognized objects and recalled object-location associations. Consistent with our hypothesis, this difference in brain-behavior relationships was particularly prominent for recognition, compared to source memory. Together, our results suggest that both APOE4 carriers and non-carriers recruit brain regions expected to be active during encoding and retrieval of object recognition and object-location associations in older adults; however, APOE4 status may influence the way in which brain regions that subserve episodic memory encoding support recognition performance. Moreover, our activation-related findings, coupled with performance outcomes, highlight the importance of task considerations in interpreting processes underlying source recall and suggest that recognition may represent a failure of source retrieval.
Our findings are consistent with our previous work on the influence of APOE4 on brain activity in middle-aged adults at risk of AD [29] and are the first to identify recognition-related differences in brain-behavior relationships in asymptomatic older adults at risk of AD. These findings add a nuanced perspective to the study of neural processes associated with recollection and familiarity as well as the role of APOE4 on brain-behavior relationships in asymptomatic older adults with family history of AD, which remains poorly characterized [57]. Future research should further investigate the lifespan effects of APOE4 on neurocognitive processes and the potential to distinguish risk of AD development based on genetic composure, behavior, and associated patterns of brain activity.
AUTHOR CONTRIBUTIONS
Data creation was a collaborative effort from the PREVENT-AD Research Group, with contributions from MELM and support from JB. SR and MNR analyzed and interpreted the data with help from SR and SP. SR wrote the manuscript with contributions from EY and MNR. All authors provided feedback and approved the final version of the manuscript.
ACKNOWLEDGMENTS
We thank members of the Rajah lab, the Cerebral Imaging Centre, and the Stop-AD Centre at the Douglas Hospital Research Centre for their helpful contributions to this work; especially Dr. R. Gordon for her help with E-Prime and Ms. C. Madjar for her help with data collection and quality control. We also acknowledge the Fonds de Recherche Québec –Santé, Natural Sciences and Engineering Research Council, and Canadian Institutes of Health Research for their support of our work.
PREVENT-AD was launched in 2011 as a $13.5 million, 7-year public-private partnership using funds provided by McGill University, the Fonds de Recherche du Québec –Santé, an unrestricted research grant from Pfizer Canada, the Levesque Foundation, the Douglas Hospital Research Centre and Foundation, the Government of Canada, and the Canada Fund for Innovation. Private sector contributions are facilitated by the Development Office of the McGill University Faculty of Medicine and by the Douglas Hospital Research Centre Foundation (http://www.douglas.qc.ca/).
Authors’ disclosures available online (https://www.j-alz.com/manuscript-disclosures/19-1292r1).
REFERENCES
[1] | World Health Organization, Dementia, https://www.who.int/news-room/fact-sheets/detail/dementia |
[2] | Alzheimer’s Association ((2020) ) 2020 Alzheimer’s disease facts and figures. Alzheimers Dement 16: , 391–460. |
[3] | Rabinovici GD ((2019) ) Late-onset Alzheimer disease. Continuum (Minneap Minn) 25: , 14–33. |
[4] | GBD Dementia Collaborators ((2019) ) Global, regional, and national burden of Alzheimer’s disease and other dementias, 1990–2016: A systematic analysis for the Global Burden of Disease Study 2016. Lancet Neurol 18: , 88–106. |
[5] | Frankish H , Horton R ((2017) ) Prevention and management of dementia: A priority for public health. Lancet 390: , 2614–2615. |
[6] | Donix M , Small GW , Bookheimer SY ((2012) ) Family history and APOE-4 genetic risk in Alzheimer’s disease. Neuropsychol Rev 22: , 298–309. |
[7] | van der Lee SJ , Wolters FJ , Ikram MK , Hofman A , Ikram MA , Amin N , van Duijn CM ((2018) ) The effect of APOE and other common genetic variants on the onset of Alzheimer’s disease and dementia: A community-based cohort study. Lancet Neurol 17: , 434–444. |
[8] | Brookmeyer R , Abdalla N , Kawas CH , Corrada MM ((2018) ) Forecasting the prevalence of preclinical and clinical Alzheimer’s disease in the United States. Alzheimers Dement 14: , 121–129. |
[9] | Kern S , Zetterberg H , Kern J , Zettergren A , Waern M , Hoglund K , Andreasson U , Wetterberg H , Borjesson-Hanson A , Blennow K , Skoog I ((2018) ) Prevalence of preclinical Alzheimer disease: Comparison of current classification systems. Neurology 90: , e1682–e1691. |
[10] | Leoutsakos JM , Gross AL , Jones RN , Albert MS , Breitner JCS ((2016) ) ‘Alzheimer’s Progression Score’: Development of a biomarker summary outcome for AD prevention trials. J Prev Alzheimers Dis 3: , 229–235. |
[11] | Molinuevo JL , Gramunt N , Gispert JD , Fauria K , Esteller M , Minguillon C , Sanchez-Benavides G , Huesa G , Moran S , Dal-Re R , Cami J ((2016) ) The ALFA project: A research platform to identify early pathophysiological features of Alzheimer’s disease. Alzheimers Dement (N Y) 2: , 82–92. |
[12] | Weiner MW , Veitch DP ((2015) ) Introduction to special issue: Overview of Alzheimer’s Disease Neuroimaging Initiative. Alzheimers Dement 11: , 730–733. |
[13] | Crous-Bou M , Minguillon C , Gramunt N , Molinuevo JL ((2017) ) Alzheimer’s disease prevention: From risk factors to early intervention. Alzheimers Res Ther 9: , 71. |
[14] | National Academies of Sciences, Engineering, and Medicine (2017) Preventing Cognitive Decline and Dementia: A Way Forward, Washington (DC). |
[15] | Weimer DL , Sager MA ((2009) ) Early identification and treatment of Alzheimer’s disease: Social and fiscal outcomes. Alzheimers Dement 5: , 215–226. |
[16] | Gardiner JM ((2001) ) Episodic memory and autonoetic consciousness: A first-person approach. Philos Trans R Soc Lond B Biol Sci 356: , 1351–1361. |
[17] | Bateman RJ , Xiong C , Benzinger TL , Fagan AM , Goate A , Fox NC , Marcus DS , Cairns NJ , Xie X , Blazey TM , Holtzman DM , Santacruz A , Buckles V , Oliver A , Moulder K , Aisen PS , Ghetti B , Klunk WE , McDade E , Martins RN , Masters CL , Mayeux R , Ringman JM , Rossor MN , Schofield PR , Sperling RA , Salloway S , Morris JC , Dominantly Inherited Alzheimer Network ((2012) ) Clinical and biomarker changes in dominantly inherited Alzheimer’s disease. N Engl J Med 367: , 795–804. |
[18] | Backman L , Small BJ , Fratiglioni L ((2001) ) Stability of the preclinical episodic memory deficit in Alzheimer’s disease. Brain 124: , 96–102. |
[19] | Sperling RA , Dickerson BC , Pihlajamaki M , Vannini P , LaViolette PS , Vitolo OV , Hedden T , Becker JA , Rentz DM , Selkoe DJ , Johnson KA ((2010) ) Functional alterations in memory networks in early Alzheimer’s disease. Neuromolecular Med 12: , 27–43. |
[20] | Morrison C , Rabipour S , Knoefel F , Sheppard C , Taler V ((2018) ) Auditory event-related potentials in mild cognitive impairment and Alzheimer’s disease. Curr Alzheimer Res 15: , 702–715. |
[21] | Morrison C , Rabipour S , Taler V , Sheppard C , Knoefel F ((2019) ) Visual event-related potentials in mild cognitive impairment and Alzheimer’s disease: A literature review. Curr Alzheimer Res 16: , 67–89. |
[22] | Genon S , Collette F , Moulin CJ , Lekeu F , Bahri MA , Salmon E , Bastin C ((2013) ) Verbal learning in Alzheimer’s disease and mild cognitive impairment: Fine-grained acquisition and short-delay consolidation performance and neural correlates. Neurobiol Aging 34: , 361–373. |
[23] | Westerberg C , Mayes A , Florczak SM , Chen Y , Creery J , Parrish T , Weintraub S , Mesulam MM , Reber PJ , Paller KA ((2013) ) Distinct medial temporal contributions to different forms of recognition in amnestic mild cognitive impairment and Alzheimer’s disease. Neuropsychologia 51: , 2450–2461. |
[24] | Zamboni G , Wilcock GK , Douaud G , Drazich E , McCulloch E , Filippini N , Tracey I , Brooks JC , Smith SM , Jenkinson M , Mackay CE ((2013) ) Resting functional connectivity reveals residual functional activity in Alzheimer’s disease. Biol Psychiatry 74: , 375–383. |
[25] | Cansino S , Estrada-Manilla C , Hernandez-Ramos E , Martinez-Galindo JG , Torres-Trejo F , Gomez-Fernandez T , Ayala-Hernandez M , Osorio D , Cedillo-Tinoco M , Garces-Flores L , Gomez-Melgarejo S , Beltran-Palacios K , Guadalupe Garcia-Lazaro H , Garcia-Gutierrez F , Cadena-Arenas Y , Fernandez-Apan L , Bartschi A , Resendiz-Vera J , Rodriguez-Ortiz MD ((2013) ) The rate of source memory decline across the adult life span. Dev Psychol 49: , 973–985. |
[26] | Spaniol J , Grady C ((2012) ) Aging and the neural correlates of source memory: Over-recruitment and functional reorganization. Neurobiol Aging 33: , 425 e423–418. |
[27] | Wolk DA , Manning K , Kliot D , Arnold SE ((2013) ) Recognition memory in amnestic-mild cognitive impairment: Insights from event-related potentials. Front Aging Neurosci 5: , 89. |
[28] | Ankudowich E , Pasvanis S , Rajah MN ((2016) ) Changes in the modulation of brain activity during context encoding vs. context retrieval across the adult lifespan. Neuroimage 139: , 103–113. |
[29] | Rajah MN , Wallaceb LMK , Ankudowich , Yu EH , Swierkot A , Patel R , Chakravarty MM , Naumova D , Pruessner J , Joober R , Gauthier S , Pasvanis S ((2017) ) Family history and APOE4 risk for Alzheimer’s disease impact the neural correlates of episodic memory by early midlife. Neuroimage Clin 14: , 760–774. |
[30] | Meyer PF , Savard M , Poirier J , Labonte A , Rosa-Neto P , Weitz TM , Town T , Breitner J , Alzheimer’s Disease Neuroimaging Initiative; PREVENT-AD Research Group ((2018) ) Bi-directional association of cerebrospinal fluid immune markers with stage of Alzheimer’s disease pathogenesis. J Alzheimers Dis 63: , 577–590. |
[31] | Tardif CL , Devenyi GA , Amaral RSC , Pelleieux S , Poirier J , Rosa-Neto P , Breitner J , Chakravarty MM , Group P-AR ((2018) ) Regionally specific changes in the hippocampal circuitry accompany progression of cerebrospinal fluid biomarkers in preclinical Alzheimer’s disease. Hum Brain Mapp 39: , 971–984. |
[32] | Villeneuve S , Vogel JW , Gonneaud J , Pichet Binette A , Rosa-Neto P , Gauthier S , Bateman RJ , Fagan AM , Morris JC , Benzinger TLS , Johnson SC , Breitner JCS , Poirier J , Presymptomatic Evaluation of Novel or Experimental Treatments for Alzheimer Disease Research Group ((2018) ) Proximity to parental symptom onset and amyloid-beta burden in sporadic Alzheimer disease. JAMA Neurol 75: , 608–619. |
[33] | Vogel JW , Vachon-Presseau E , Pichet Binette A , Tam A , Orban P , La Joie R , Savard M , Picard C , Poirier J , Bellec P , Breitner JCS , Villeneuve S , Alzheimer’s Disease Neuroimaging Initiative, the PREVENT-AD Research Group ((2018) ) Brain properties predict proximity to symptom onset in sporadic Alzheimer’s disease. Brain 141: , 1871–1883. |
[34] | Eid A , Mhatre I , Richardson JR ((2019) ) Gene-environment interactions in Alzheimer’s disease: A potential path to precision medicine. Pharmacol Ther 199: , 173–187. |
[35] | Andersen AH , Rayens WS , Liu Y , Smith CD ((2012) ) Partial least squares for discrimination in fMRI data. Magn Reson Imaging 30: , 446–452. |
[36] | McIntosh AR , Bookstein FL , Haxby JV , Grady CL ((1996) ) Spatial pattern analysis of functional brain images using partial least squares. Neuroimage 3: , 143–157. |
[37] | Breitner JCS , Poirier J , Etienne PE , Leoutsakos JM ((2016) ) Rationale and structure for a new center for Studies on Prevention of Alzheimer’s Disease (StoP-AD). J Prev Alzheimers Dis 3: , 236–242. |
[38] | Tschanz JT , Norton MC , Zandi PP , Lyketsos CG ((2013) ) The Cache County Study on Memory in Aging: Factors affecting risk of Alzheimer’s disease and its progression after onset. Int Rev Psychiatry 25: , 673–685. |
[39] | Gosselin N , De Beaumont L , Gagnon K , Baril AA , Mongrain V , Blais H , Montplaisir J , Gagnon JF , Pelleieux S , Poirier J , Carrier J ((2016) ) BDNF Val66Met polymorphism interacts with sleep consolidation to predict ability to create new declarative memories. J Neurosci 36: , 8390–8398. |
[40] | Tremblay-Mercier J , Madjar C , Das S , Dyke SOM , Étienne P , Lafaille-Magnan M-E , Bellec P , Collins DL , Rajah MN , Bohbot VD , Leoutsakos J-M , Iturria-Medina Y , Kat J , Hoge RD , Gauthier S , Chakravarty MM , Poline J-B , Rosa-Neto P , Villeneuve S , Evans AC , Poirier J , Breitner JCS , PREVENT-AD Research Group ((2020) ) Creation of an open science dataset from PREVENT-AD, a longitudinal cohort study of pre-symptomatic Alzheimer’s disease. BiorXiv, doi: 10.1101/2020.03.04.976670 |
[41] | Randolph C , Tierney M , Mohr E , Chase T ((1998) ) The Repeatable Battery for the Assessment of Neuropsychological Status (RBANS): Preliminary clinical validity. J Clin Exp Neuropsychol 20: , 310–319. |
[42] | Galvin JE , Roe CM , Powlishta KK , Coats MA , Muich SJ , Grant E , Miller JP , Storandt M , Morris JC ((2005) ) The AD8: A brief informant interview to detect dementia. Neurology 65: , 559–564. |
[43] | Berg L ((1984) ) Clinical Dementia Rating. Br J Psychiatry 145: , 339. |
[44] | Nasreddine ZS , Chertkow H , Phillips N , Whitehead V , Collin I , Cummings JL ((2004) ) The Montreal Cognitive Assessment (MoCA): A brief cognitive screening tool for detection of mild cognitive impairment. Neurology 62: , A132–A132. |
[45] | Brodeur MB , Guerard K , Bouras M ((2014) ) Bank of Standardized Stimuli (BOSS) phase II: 930 new normative photos. Plos One 9: , e106953. |
[46] | Dale AM , Buckner RL ((1997) ) Selective averaging of rapidly presented individual trials using fMRI. Hum Brain Mapp 5: , 329–340. |
[47] | Gur RE , Gur RC ((2002) ) Gender differences in aging: Cognition, emotions, and neuroimaging studies. Dialogues Clin Neurosci 4: , 197–210. |
[48] | McCarrey AC , An Y , Kitner-Triolo MH , Ferrucci L , Resnick SM ((2016) ) Sex differences in cognitive trajectories in clinically normal older adults. Psychol Aging 31: , 166–175. |
[49] | Subramaniapillai S , Rajagopal S , Elshiekh A , Pasvanis S , Ankudowich E , Rajah MN ((2019) ) Sex differences in the neural correlates of spatial context memory decline in healthy aging. J Cogn Neurosci 31: , 1895–1916. |
[50] | Stanislaw H , Todorov N ((1999) ) Calculation of signal detection theory measures. Behav Res Methods Instrum Comput 31: , 137–149. |
[51] | McIntosh AR , Chau WK , Protzner AB ((2004) ) Spatiotemporal analysis of event-related fMRI data using partial least squares. Neuroimage 23: , 764–775. |
[52] | Krishnan A , Williams LJ , McIntosh AR , Abdi H ((2011) ) Partial Least Squares (PLS) methods for neuroimaging: A tutorial and review. Neuroimage 56: , 455–475. |
[53] | McIntosh AR , Lobaugh NJ ((2004) ) Partial least squares analysis of neuroimaging data: Applications and advances. Neuroimage 23 Suppl 1: , S250–263. |
[54] | Lancaster JL , Tordesillas-Gutiérrez D , Martinez M , Salinas F , Evans A , Zilles K , Mazziotta JC , Fox PT ((2007) ) Bias between MNI and Talairach coordinates analyzed using the ICBM-152 Brain Template. Hum Brain Mapp 28: , 1194–1205. |
[55] | Eickhoff SB , Laird AR , Grefkes C , Wang LE , Zilles K , Fox PT ((2009) ) Coordinate-based activation likelihood estimation meta-analysis of neuroimaging data: A random-effects approach based on empirical estimates of spatial uncertainty. Hum Brain Mapp 30: , 2907–2926. |
[56] | Talairach J , Tournoux P ((1958) ) [Stereotaxic localization of central gray nuclei]. Neurochirurgia (Stuttg) 1: , 88–93. |
[57] | O’Donoghue MC , Murphy SE , Zamboni G , Nobre AC , Mackay CE ((2018) ) APOE genotype and cognition in healthy individuals at risk of Alzheimer’s disease: A review. Cortex 104: , 103–123. |
[58] | Bassett SS , Yousem DM , Cristinzio C , Kusevic I , Yassa MA , Caffo BS , Zeger SL ((2006) ) Familial risk for Alzheimer’s disease alters fMRI activation patterns. Brain 129: , 1229–1239. |
[59] | Dennis NA , Browndyke JN , Stokes J , Need A , Burke JR , Welsh-Bohmer KA , Cabeza R ((2010) ) Temporal lobe functional activity and connectivity in young adult APOE varepsilon4 carriers. Alzheimers Dement 6: , 303–311. |
[60] | Filippini N , MacIntosh BJ , Hough MG , Goodwin GM , Frisoni GB , Smith SM , Matthews PM , Beckmann CF , Mackay CE ((2009) ) Distinct patterns of brain activity in young carriers of the APOE-epsilon4 allele. Proc Natl Acad Sci U S A 106: , 7209–7214. |
[61] | Johnson SC , Schmitz TW , Trivedi MA , Ries ML , Torgerson BM , Carlsson CM , Asthana S , Hermann BP , Sager MA ((2006) ) The influence of Alzheimer disease family history and apolipoprotein E epsilon4 on mesial temporal lobe activation. J Neurosci 26: , 6069–6076. |
[62] | Xu G , McLaren DG , Ries ML , Fitzgerald ME , Bendlin BB , Rowley HA , Sager MA , Atwood C , Asthana S , Johnson SC ((2009) ) The influence of parental history of Alzheimer’s disease and apolipoprotein E epsilon4 on the BOLD signal during recognition memory. Brain 132: , 383–391. |
[63] | Taylor WD , Boyd B , Turner R , McQuoid DR , Ashley-Koch A , MacFall JR , Saleh A , Potter GG ((2017) ) APOE epsilon4 associated with preserved executive function performance and maintenance of temporal and cingulate brain volumes in younger adults. Brain Imaging Behav 11: , 194–204. |
[64] | Reas ET , Laughlin GA , Bergstrom J , Kritz-Silverstein D , Barrett-Connor E , McEvoy LK ((2019) ) Effects of APOE on cognitive aging in community-dwelling older adults. Neuropsychology 33: , 406–416. |
[65] | Eich TS , Tsapanou A , Stern Y ((2019) ) When time’s arrow doesn’t bend: APOE-epsilon4 influences episodic memory before old age. Neuropsychologia 133: , 107180. |
[66] | Pinto TCC , Machado L , Bulgacov TM , Rodrigues-Junior AL , Costa MLG , Ximenes RCC , Sougey EB ((2019) ) Is the Montreal Cognitive Assessment (MoCA) screening superior to the Mini-Mental State Examination (MMSE) in the detection of mild cognitive impairment (MCI) and Alzheimer’s disease (AD) in the elderly? Int Psychogeriatr 31: , 491–504. |
[67] | Evans S , Dowell NG , Tabet N , Tofts PS , King SL , Rusted JM ((2014) ) Cognitive and neural signatures of the APOE E4 allele in mid-aged adults. Neurobiol Aging 35: , 1615–1623. |
[68] | Elkana O , Tal N , Oren N , Soffer S , Ash EL ((2020) ) Is the cutoff of the MoCA too high? Longitudinal data from highly educated older adults. J Geriatr Psychiatry Neurol 33: , 155–160. |
[69] | Qiu C , Kivipelto M , Aguero-Torres H , Winblad B , Fratiglioni L ((2004) ) Risk and protective effects of the APOE gene towards Alzheimer’s disease in the Kungsholmen project: Variation by age and sex. J Neurol Neurosurg Psychiatry 75: , 828–833. |
[70] | Rawle MJ , Davis D , Bendayan R , Wong A , Kuh D , Richards M ((2018) ) Apolipoprotein-E (Apoe) epsilon4 and cognitive decline over the adult life course. Transl Psychiatry 8: , 18. |
[71] | Maillet D , Rajah MN ((2014) ) Age-related differences in brain activity in the subsequent memory paradigm: A meta-analysis. Neurosci Biobehav Rev 45: , 246–257. |
[72] | Salami A , Eriksson J , Nyberg L ((2012) ) Opposing effects of aging on large-scale brain systems for memory encoding and cognitive control. J Neurosci 32: , 10749–10757. |
[73] | Shing YL , Brehmer Y , Heekeren HR , Backman L , Lindenberger U ((2016) ) Neural activation patterns of successful episodic encoding: Reorganization during childhood, maintenance in old age. Dev Cogn Neurosci 20: , 59–69. |
[74] | Rugg MD , Vilberg KL ((2013) ) Brain networks underlying episodic memory retrieval. Curr Opin Neurobiol 23: , 255–260. |
[75] | Daselaar SM , Fleck MS , Cabeza R ((2006) ) Triple dissociation in the medial temporal lobes: Recollection, familiarity, and novelty. J Neurophysiol 96: , 1902–1911. |
[76] | Davachi L ((2006) ) Item, context and relational episodic encoding in humans. Curr Opin Neurobiol 16: , 693–700. |
[77] | Rajah MN , Languay R , Valiquette L ((2010) ) Age-related changes in prefrontal cortex activity are associated with behavioural deficits in both temporal and spatial context memory retrieval in older adults. Cortex 46: , 535–549. |
[78] | Rajah MN , Languay R , Grady CL ((2011) ) Age-related changes in right middle frontal gyrus volume correlate with altered episodic retrieval activity. J Neurosci 31: , 17941–17954. |
[79] | Koen JD , Yonelinas AP ((2014) ) The effects of healthy aging, amnestic mild cognitive impairment, and Alzheimer’s disease on recollection and familiarity: A meta-analytic review. Neuropsychol Rev 24: , 332–354. |
[80] | Grady CL , McIntosh AR , Beig S , Keightley ML , Burian H , Black SE ((2003) ) Evidence from functional neuroimaging of a compensatory prefrontal network in Alzheimer’s disease. J Neurosci 23: , 986–993. |
[81] | Cosentino S , Scarmeas N , Helzner E , Glymour MM , Brandt J , Albert M , Blacker D , Stern Y ((2008) ) APOE epsilon 4 allele predicts faster cognitive decline in mild Alzheimer disease. Neurology 70: , 1842–1849. |
[82] | Farlow MR , He Y , Tekin S , Xu J , Lane R , Charles HC ((2004) ) Impact of APOE in mild cognitive impairment. Neurology 63: , 1898–1901. |
[83] | Striepens N , Scheef L , Wind A , Meiberth D , Popp J , Spottke A , Kolsch H , Wagner M , Jessen F ((2011) ) Interaction effects of subjective memory impairment and ApoE4 genotype on episodic memory and hippocampal volume. Psychol Med 41: , 1997–2006. |
[84] | Gallagher M , Koh MT ((2011) ) Episodic memory on the path to Alzheimer’s disease. Curr Opin Neurobiol 21: , 929–934. |
[85] | Matura S , Prvulovic D , Jurcoane A , Hartmann D , Miller J , Scheibe M , O’Dwyer L , Oertel-Knochel V , Knochel C , Reinke B , Karakaya T , Fusser F , Pantel J ((2014) ) Differential effects of the ApoE4 genotype on brain structure and function. Neuroimage 89: , 81–91. |
[86] | Braskie MN , Medina LD , Rodriguez-Agudelo Y , Geschwind DH , Macias-Islas MA , Thompson PM , Cummings JL , Bookheimer SY , Ringman JM ((2013) ) Memory performance and fMRI signal in presymptomatic familial Alzheimer’s disease. Hum Brain Mapp 34: , 3308–3319. |
[87] | Hersi M , Irvine B , Gupta P , Gomes J , Birkett N , Krewski D ((2017) ) Risk factors associated with the onset and progression of Alzheimer’s disease: A systematic review of the evidence. Neurotoxicology 61: , 143–187. |
[88] | Jessen F , Wiese B , Bickel H , Eifflander-Gorfer S , Fuchs A , Kaduszkiewicz H , Kohler M , Luck T , Mosch E , Pentzek M , Riedel-Heller SG , Wagner M , Weyerer S , Maier W , van den Bussche H , AgeCoDe Study G ((2011) ) Prediction of dementia in primary care patients. PLoS One 6: , e16852. |
[89] | Simon SS , Hampstead BM , Nucci MP , Duran FLS , Fonseca LM , Martin M , Avila R , Porto FHG , Brucki SMD , Martins CB , Tascone LS , Amaro E Jr. , Busatto GF , Bottino CMC ((2018) ) Cognitive and brain activity changes after mnemonic strategy training in amnestic mild cognitive impairment: Evidence from a randomized controlled trial. Front Aging Neurosci 10: , 342. |
[90] | Simon SS , Hampstead BM , Nucci MP , Duran FLS , Fonseca LM , Martin M , Avila R , Porto FHG , Brucki SMD , Martins CB , Tascone LS , Amaro E Jr. , Busatto GF , Bottino CMC ((2019) ) Training gains and transfer effects after mnemonic strategy training in mild cognitive impairment: A fMRI study. Int J Psychophysiol, doi: 10.1016/j.ijpsycho.2019.03.014. |
[91] | Han SD , Bondi MW ((2008) ) Revision of the apolipoprotein E compensatory mechanism recruitment hypothesis. Alzheimers Dement 4: , 251–254. |
[92] | Tuminello ER , Han SD ((2011) ) The apolipoprotein e antagonistic pleiotropy hypothesis: Review and recommendations. Int J Alzheimers Dis 2011: , 726197. |
[93] | Pichet Binette A , Gonneaud J , Vogel JW , La Joie R , Rosa-Neto P , Collins DL , Poirier J , Breitner JCS , Villeneuve S , Vachon-Presseau E , Alzheimer’s Disease Neuroimaging Initiative, PREVENT-AD Research Group ((2020) ) Morphometric network differences in ageing versus Alzheimer’s disease dementia. Brain 143: , 635–649. |
[94] | Stevens WD , Hasher L , Chiew KS , Grady CL ((2008) ) A neural mechanism underlying memory failure in older adults. J Neurosci 28: , 12820–12824. |
[95] | Vidal-Pineiro D , Sneve MH , Storsve AB , Roe JM , Walhovd KB , Fjell AM ((2017) ) Neural correlates of durable memories across the adult lifespan: Brain activity at encoding and retrieval. Neurobiol Aging 60: , 20–33. |