Volume Analysis of Brain Cognitive Areas in Alzheimer’s Disease: Interim 3-Year Results from the ASCOMALVA Trial
Abstract
Background:
Cerebral atrophy is a common feature of several neurodegenerative disorders, including Alzheimer’s disease (AD). In AD, brain atrophy is associated with loss of gyri and sulci in the temporal and parietal lobes, and in parts of the frontal cortex and cingulate gyrus.
Objective:
The ASCOMALVA trial has assessed, in addition to neuropsychological analysis, whether the addition of the cholinergic precursor choline alphoscerate to treatment with donepezil has an effect on brain volume loss in patients affected by AD associated with cerebrovascular injury.
Methods:
56 participants to the randomized, placebo-controlled, double-blind ASCOMALVA trial were assigned to donepezil + placebo (D + P) or donepezil + choline alphoscerate (D + CA) treatments and underwent brain magnetic resonance imaging and neuropsychological tests every year for 4 years. An interim analysis of 3-year MRI data was performed by voxel morphometry techniques.
Results:
The D + P group (n = 27) developed atrophy of the gray and white matter with concomitant increase in ventricular space volume. In the D + CA group (n = 29) the gray matter atrophy was less pronounced compared to the D + P group in frontal and temporal lobes, hippocampus, and amygdala. These morphological data are consistent with the results of the neuropsychological tests.
Conclusion:
Our findings indicate that the addition of choline alphoscerate to standard treatment with the cholinesterase inhibitor donepezil counters to some extent the loss in volume occurring in some brain areas of AD patients. The observation of parallel less pronounced decrease in cognitive and functional tests in patients with the same treatment suggests that the morphological changes observed may have functional relevance.
INTRODUCTION
More than one hundred years have passed since the first pathophysiological characteristics of Alzheimer’s disease (AD) [1]. In AD, brain atrophy is generally widespread: involvement is more severe in the temporal lobes of the hippocampus. Some studies highlight a significant correlation between the severity of dementia and the degree of atrophy of the temporal lobe measured by magnetic resonance imaging (MRI) [2]. Microscopic analyses of brains of patients affected by AD have revealed neuronal loss (considered the main cause of the cognitive deficits) and regressive phenomena affecting residual neurons, characterized by unique traits: filamentous structures (AD neurofibrillar degeneration), ovoid inclusions (granulo-vacuolar degeneration) and increase in the density of senile plaques [3, 4].
The cholinergic hypothesis was the first attempt to explain the pathophysiology of AD from a molecular point of view. It was formulated more than 30 years ago as a primary degenerative process affecting primarily groups of cholinergic neurons in the hippocampus, the frontal cortex, the amygdala, the basal nucleus, and the medial septum, regions and structures with a relevant role in attention, learning, and memory processes [5]. This selective alteration determines a reduction in the expression of cholinergic markers, such as choline acetyltransferase and acetylcholinesterase, which is associated with the onset of cognitive impairment [6]. The decrease in cholinergic markers is proportional to the density of neurofibrillary changes and the severity of the disease. Non-selective muscarinic antagonists, such as scopolamine, which decreases cognitive functions, promote the production of amyloid-β peptide and decrease the activity of α-secretase [7]. Some triterpene saponins reduce amnesia induced by scopolamine [8, 9] and non-selective and selective muscarinic agonists have been shown to improve learning and memory [10]. The exact molecular mechanisms that mediate the effect of cholinergic drugs in learning and memory and their viability in clinical treatment are still under study [11, 12]. A decreased number and density of nicotinic receptors in patients with AD (mainly α4β2 subtype), reduced expression of α3, α4, and α7 subunits in the cortex and in the hippocampus, and in binding capacity of α7 and α4 hippocampal cortical receptors were documented as well [13–15].
In AD, damaged cholinergic signaling has been reported [16]. Acetylcholine and its receptors, in particular (α7), are considered neuroprotective as they modulate neuronal excitability mediated by glutamate [17, 18]. Abnormalities in glutamatergic neurotransmission in AD are initially observed in the entorhinal cortex, followed by an involvement of hippocampus, amygdala, frontal cortex and parietal cortex [19].
The cholinergic hypothesis was the basis for the first treatment strategies and approaches to drug development (acetylcholinesterase inhibitors, cholinergic precursors, cholinergic receptor agonists, cholinergic allosteric enhancers) for AD. It was hypothesized that the observed relationship between cognitive dysfunction and reduced cholinergic transmission in the brain plays an important role in AD, but does not in itself determine the ultimate cause of the disease [20, 21].
Diagnostic techniques, such as positron emission tomography (PET) and MRI, are increasingly used [22] in clinical practice to have more objective and uniform parameters to measure. With MRI, it is possible to detect the disease even in the initial stages and to distinguish mild forms from more severe forms of the disease by assessing the level of cerebral atrophy. AD diagnosis is primarily based on clinical criteria [23, 24], although biomarkers enhance the accuracy of diagnosis and their use is becoming a standard in the evaluation of dementia [25–28].
Starting from the so-called cholinergic hypothesis of geriatric memory dysfunction, various pharmacological approaches were proposed in attempts to correct the cholinergic deficit observed in the central nervous system of patients affected by vascular dementia and AD [29–31]. Cholinesterase inhibitors (ChE-I) (in particular donepezil, galantamine, and rivastigmine), initially proposed mainly for treating the cognitive symptoms of AD, are nowadays actively studied for other adult-onset cognitive dysfunction disorders, including vascular dementia [29, 31, 32]. The three above drugs exert modest, but positive effects on the cognitive dysfunctions and behavioral disorders typical of adult-onset dementia disorders. The precursor loading strategy proposed in parallel with the development of the geriatric cholinergic dysfunction hypothesis provided inconsistent results in clinical trials and was therefore left early. A subsequent analysis of choline-containing phospholipids acting as acetylcholine precursors has shown that the ineffectiveness reported primarily for the cholinergic precursor phosphatidylcholine (lecithin) is not shared by all the compounds of this class. CDP-choline and choline alphoscerate (L-alpha-glycerylphosphorylcholine, GFC) have proved to exert some effects on cognitive functions in adult-onset dementia disorders [33, 34]. On the other hand, choline alphoscerate has shown a higher activity compared to other cholinergic precursors of the same class [34].
Based on the above considerations, a clinical study (Association between the cholinesterase inhibitor donepezil and the cholinergic precursor choline alphoscerate in Alzheimer’s disease, ASCOMALVA) was designed to assess whether the combination of a cholinergic precursor with ChE-I, which has proved to be effective in preclinical studies [35, 36], may be a therapeutic option for enhancing the effect of cholinergic therapies in AD patients with concomitant ischemic cerebrovascular disease. The Interim results of the ASCOMALVA trial after the first and second year of treatment were published [37, 38]. The present study summarizes the results of the ASCOMALVA trial after 3 years of observation, including brain morphometric data. Quantitative brain volume analysis is an extension versus the previous interim results of ASCOMALVA which were limited to neuropsychological analyses.
METHODS
The trial
ASCOMALVA is a multicenter, randomized, placebo-controlled, double-blind clinical trial that was designed spontaneously by the investigators. Characteristics of the trial, centers involved and their role are reported elsewhere [37, 38].
Initially, the protocol plan was to treat patients either with donepezil + choline alphoscerate (treatment group D + CA) or donepezil + placebo (control group D + P) for 24 months. Based on the encouraging results obtained from an interim analysis, an amendment to the protocol was proposed and authorization to prolong the study for another two years was obtained. Therefore, ASCOMALVA has observed enrolled patients for 4 years. By maintaining the double-blind, the protocol enabled the coordinating center to evaluate the course of the parameters, which were analyzed via the web after 3, 6, 9, 12, 18, 24, 30, 36, 42, and 48 months of treatment. Intermediate evaluation, without breaking the blind, was possible because all study data, excluding patient identity, were available on a web platform managed by the coordinating center. Only the coordinator knew the type of treatment (active or control) assigned to individual patients.
The trial recruited AD patients with concurrent cerebrovascular damage. These patients represent a population with major cholinergic hypofunction [39, 40], who can benefit from “reinforced” (biosynthesis precursor, e.g., choline alphoscerate + degradation inhibitor, e.g. donepezil) cholinergic therapy. Diagnosis of AD disease was established according to NINCDS-ADRDA criteria [41]. Vascular damage was evaluated using the new scale of assessment for White-Age-Related Matter Changes (ARWMC) based on the identification of cerebral ischemic damage with computed tomography and/or magnetic resonance of the brain. Patients showed comparable scores in the scale with no significant differences between groups at baseline nor at controls made every year. Inclusion and exclusion criteria are reported elsewhere [37, 38].
Three years of treatment were achieved in 113 patients (67 females and 46 males). These individuals underwent follow-up visits at 3, 6, 9, 12, 18, 24, 30, and 36 months. During each follow-up visit, patients were examined and the tests listed below were performed [37, 38]: Mini-Mental State Examination (MMSE) and AD Cognitive Scale subscale (ADAS-cog); the Basic Activities of Daily Life (BADL) and instrumental Activities of Daily Living (IADL); and the Neuropsychiatric Inventory frequency x severity (NPI-F) and caregiver stress (NPI-D).
Statistical analysis of the differences between the scores related to the various parameters investigated in the two groups of patients (D + CA versus D + P) was performed by the analysis of variance (ANOVA). Significance of the differences between the two groups was assessed by the two-tailed Student’s “t” test, with Bonferroni correction for multiple analysis.
MRI analysis
The patients participating in the study were required to carry out MRI analyzes at the hospital clinics or at centers affiliated to the national health service. This was for budgetary reasons due to the spontaneous generation type of the study. As a consequence of it, patients may have MRIs made with different machines or not at the same resolution. Patients who had no comparable MRI tests were discarded. After this analysis, 56 patients of which 27 treated with D + P and 29 treated with D + CA remained. Further details are shown in the flowchart of the trial (Fig. 1).
Fig. 1
Flowchart of the study.
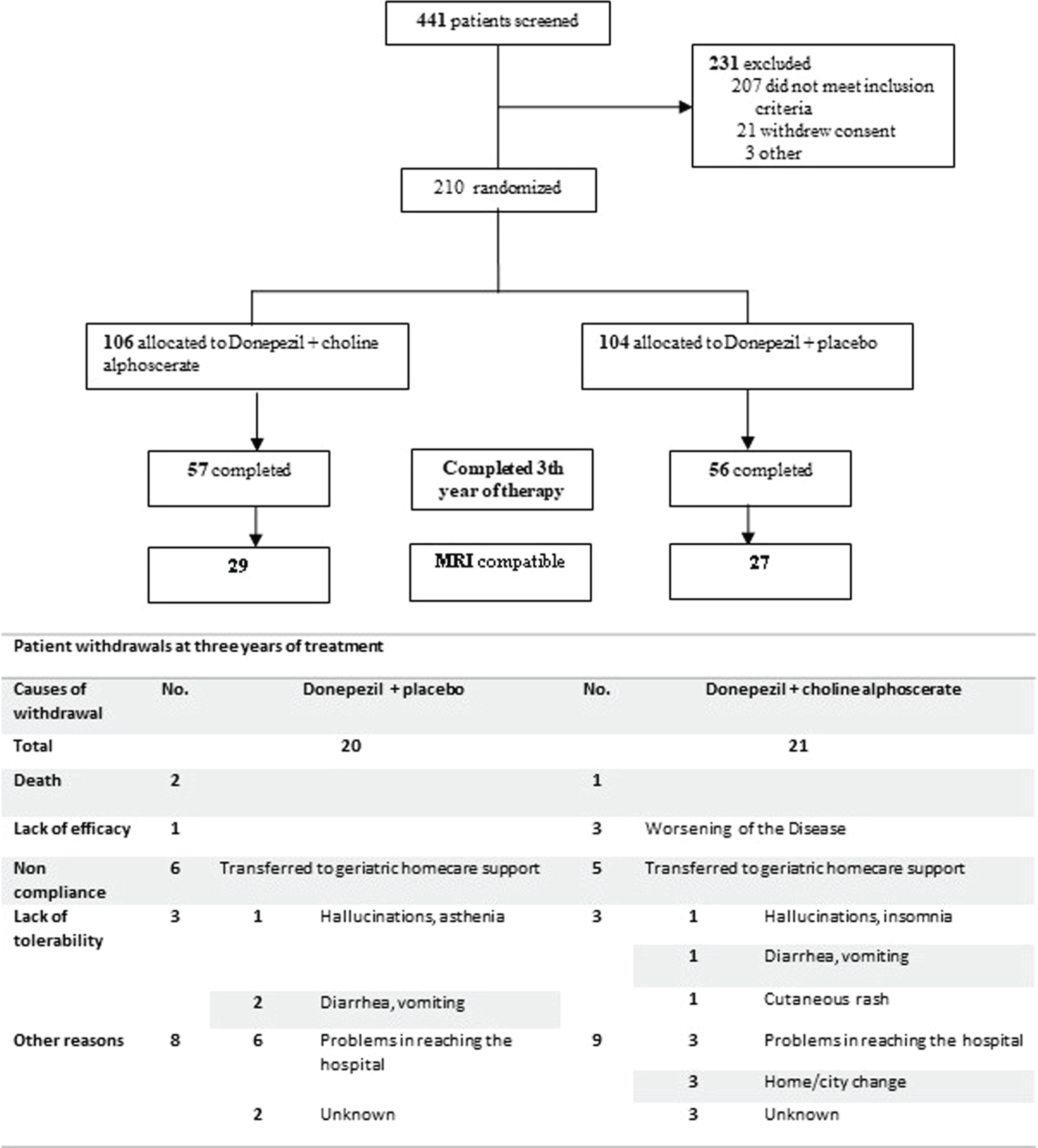
Target brain areas were selected prior of the evaluation, according to the literature [42–48], among the brain areas most affected by atrophy in AD.
MRI processing
The routine brain MRI protocol included axial (T1WI and T2WI), coronal PDWI, and sagittal T1WI. 3D T1 sequence was performed with slice thickness 0.5 mm. The brain images were transferred to a personal computer workstation using Slicer 4.4.0. software, which is a multiplatform, free open source software package for visualization and medical image computing developed by Harvard University and approved for medical research (http://www.slicer.org/). It provides reliable morphometry of structures of interest by manual and semiautomated tracing.
3D T1-weighted images, axial and sagittal, were used for volumetric analysis. The volume of each region of interest was automatically calculated by summing the trace area of each slice multiplied by the slice thickness. The volumes were measured after tracing both the right and left sides. Brain regions of interest were delineated by drawing them using a semi-automated tool in the slices where structures were visible. The traced volumes of each region of interest were recorded in mm3, but were expressed as a percentage variation of the traced volume out of the same traced volume at baseline to minimize interpersonal and gender-related variability.
Data analysis
Data obtained via the volume analysis were transferred into a Microsoft Excel database and then divided according to the patients’ treatment scheme and the parameter changes were evaluated over time. Data were then analyzed statistically using analysis of variance (ANOVA) for comparisons between treatments. Student’s t-test for paired data was used to assess statistical significance of differences between groups. Comparison of data obtained at recruitment and at the various visits was made using the correlation coefficient of Pearson and the specific data analysis program Origin 9.1 (OriginLab Corporation). Average brain volumes of subjects of the two groups investigated (D + P versus D + CA) were compared by one-way analysis of variance (ANOVA), setting p < 0.05 as the cutoff level of significance, with Bonferroni correction for multiple analysis.
RESULTS
Twenty patients assigned to D + P (17.7%) and twenty-one patients (18.6%) assigned to D + CA withdrew from the study. Tolerability of treatment was similar in the two groups of patients. The reasons for withdrawal are summarized in Fig. 1.
Neuropsychological analysis
Cognitive assessment, showed a significant difference between the two experimental groups (D + CA and D + P). Specifically, the subjects of the D + P group showed a significant worsening of the global cognitive functions measured through the MMSE (Fig. 2A) and the ADAS-cog (Fig. 2B) compared to the group D + CA, from the 24th month of observation up to three years of treatment.
Fig. 2
Evaluation of cognitive (MMSE, A; ADAS-cog, B), functional (BADL, C; IADL, D) and behavioral (NPI-F, E; NPI-D, F) tests during the ASCOMALVA study. Data are means±S.E.M. *p < 0.05 versus baseline; #p < 0.05 Donepezil + Placebo versus Donepezil + choline alphoscerate.
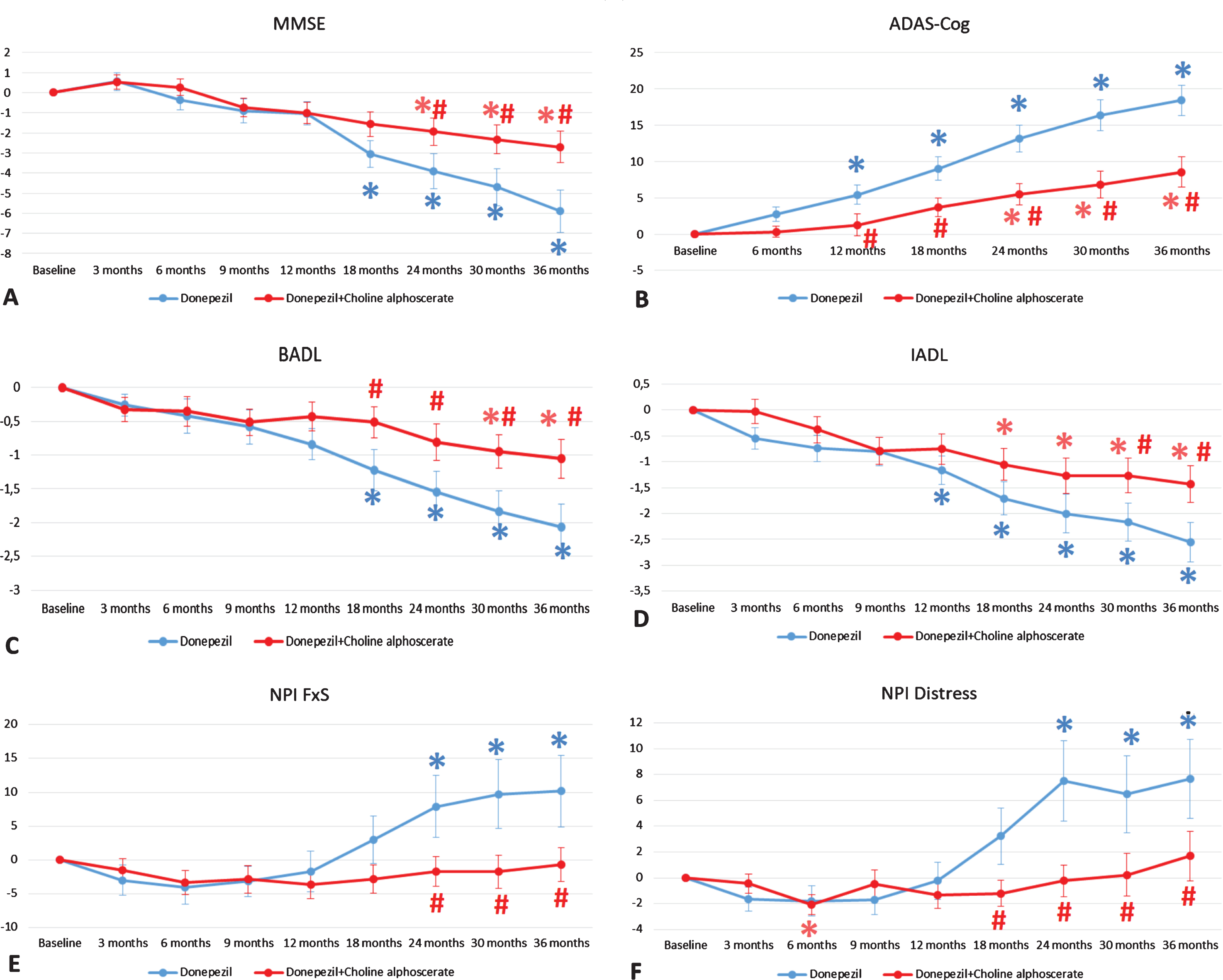
Functional assessment resulted in a significant difference between the two groups. Specifically, the D + P group showed a significant worsening of the BADL (Fig. 2C) and IADL (Fig. 2D) scores, respectively, from 18th month and 30th month of observation up to three years of treatment, compared to the D + CA group.
The results of the behavioral assessment on the NPI scale showed a significant decrease in the severity (NPI-F, Fig. 2E) and the caregiver distress (NPI-D, Fig. 2F), in patients treated with D + CA compared to D + P, from the 24th month of observation up to three years of treatment.
MRI analysis
Baseline demographic data of the patients and MRI 5 volumes at baseline are reported in Table 1.
Table 1
Demographics of patients recruited for the trial ASCOMALVA analyzed for MRI volumes
Donepezil | Donepezil + Choline alphoscerate | |
Sex (M/F) | ♂15 (54%)/♀12 (46%) | ♂11 (38%)/♀18 (62%) |
Age (y) | 74±6 | 74±6 |
Education (y) | 7±5 | 7±2 |
MMSE at baseline | 20.8±4.0 | 19.8±2.8 |
ADAS-Cog at baseline | 24.5±7.3 | 28.0±6.7 |
BADL at baseline | 4.7±1.6 | 5.5±0.8 |
IADL at baseline | 3.2±1.7 | 3.7±1.8 |
NPI FxS at baseline | 19.5±20.9 | 21.3±18.6 |
NPI Distress at baseline | 7.6±7.6 | 10.0±8.9 |
Gray matter volume at baseline (mm3) | 673±142 | 602±139 |
White matter volume at baseline (mm3) | 374±130 | 387±115 |
CSF matter volume at baseline (mm3) | 199±53 | 230±50 |
Figure 3 shows segmentation of the cerebral areas obtained by the MRI analysis. This analysis enabled extrapolation of the volumes of the gray matter, white matter, and cerebrospinal fluid (CSF).
Fig. 3
Changes in the percentage of gray (A) and white matter (B), and CSF volumes (C) in the two groups of patients over the three years of observation. The data are means of the percentage variation ± S.E.M. *p < 0.05 versus baseline; #p < 0.05 versus donepezil and placebo.
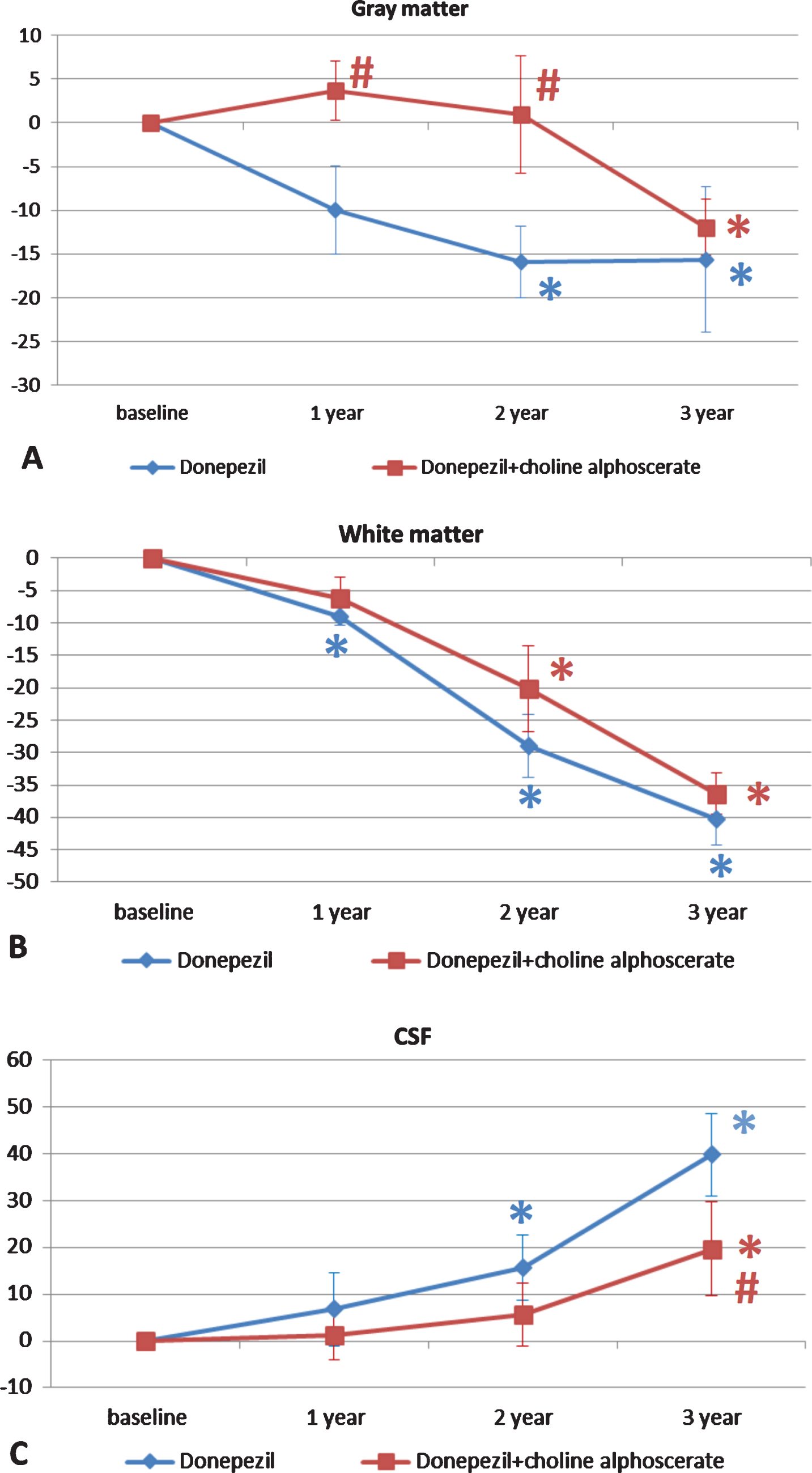
Patients in the D + P group showed a statistically significant reduction in gray matter from baseline in the second and third years of treatment. D + CA treatment resulted in a significant difference from baseline only in the third year of treatment. Differences between the two groups were statistically significant during the first two years of treatment (Fig. 3A).
The decrease in the volume of the white matter was compared versus baseline over the three years of treatment in the D + P group and in the last two years in the group treated with D + CA. No statistically significant differences were noticeable between the two study groups (Fig. 3B). The reduction of the volumes of grey and white matter were compensated by a significant increase in CSF volume from baseline in the second and third year of treatment in patients receiving D + P and in the third year of treatment in patients receiving D + CA. The difference between groups became significant after the third year of treatment (Fig. 3C).
Figure 4 shows the results of volume analysis of hippocampus and amygdala grey matter. A progressive reduction in the volume of the hippocampus was noticeable along the course of the study (Fig. 4A, B). This reduction was more pronounced in the D + P group than in the D + CA group. The difference between the two groups was significant for the right hippocampus throughout the three years of treatment (Fig. 4B), whereas this significance was recorded after the second and third year of treatment for the left hippocampus (Fig. 4A). Similar right-left differences not statistically significant were found between the two groups for the amygdala (Fig. 4C, D).
Fig. 4
Changes in the percentage of the volume of left (L) and right (R) hippocampus (A and B respectively) and of amygdala (C and D respectively) in the two groups of patients over the three years of observation. The data are means of the percentage variation ± S.E.M. *p < 0.05 versus baseline; #p < 0.05 versus donepezil plus placebo.
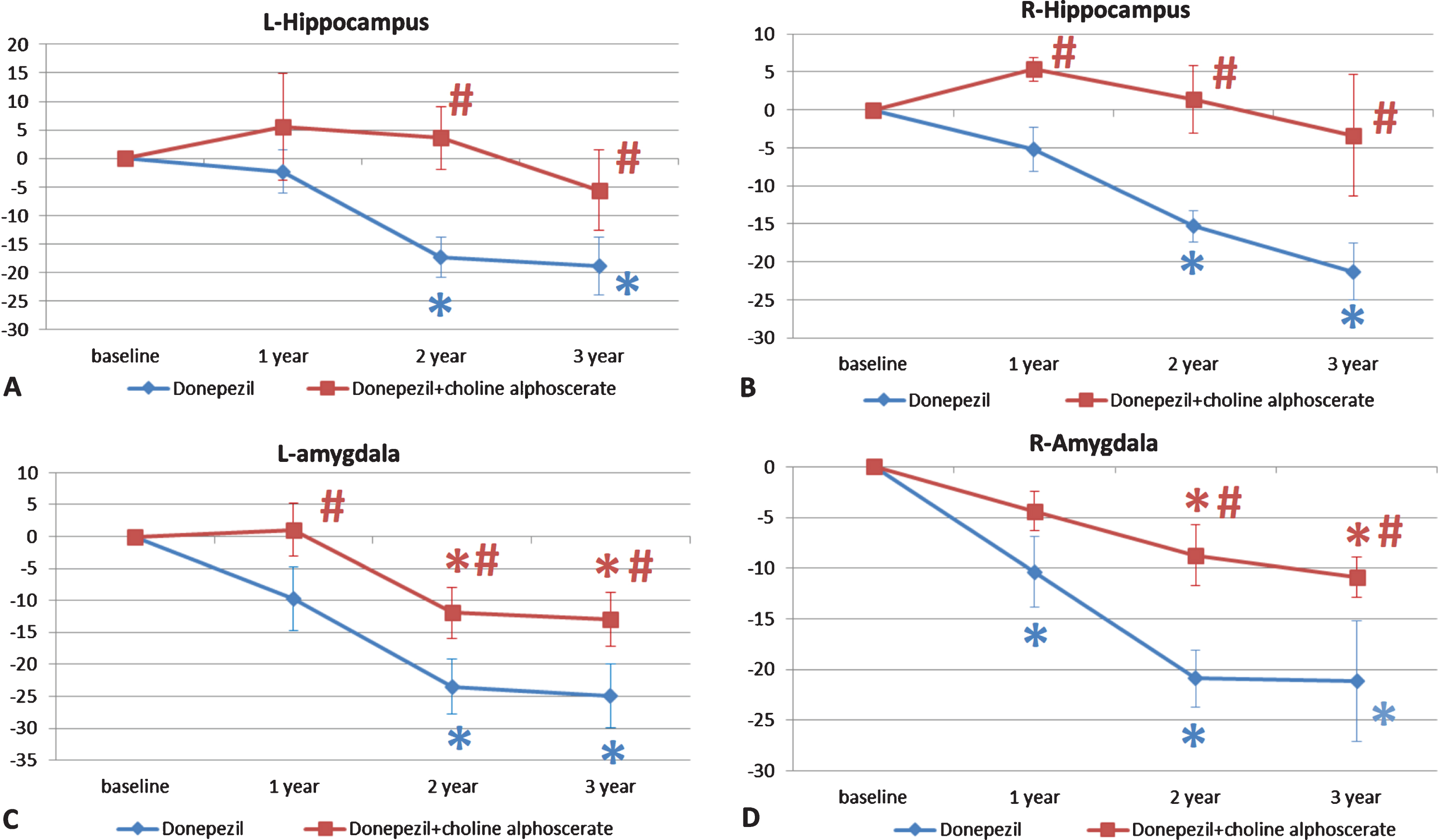
Morphometric analysis was also extended to parahippocampal gyrus, basal ganglia (caudate nucleus, putamen, globus pallidus), and frontal cortex (superior and middle frontal gyri). The trend of cerebral atrophy was similar as described above for the limbic areas considered. In the parahippocampal area, atrophy was less pronounced in the first and second year of treatment (Fig. 5A). In the superior frontal gyrus, differences were significant between the two treatment groups over the three years of treatment (Fig. 5B), whereas in the middle frontal convolution, differences were statistically significant during the first two years of treatment (Fig. 5C).
Fig. 5
Changes in the percentage of the volume of left (L) and right (R) caudate nucleus (A and B respectively) and putamen (C and D respectively) in the two groups of patients along the three years of observation. The data are the means of the percentage variation±S.E.M. *p < 0.05 versus baseline; #p < 0.05 versus donepezil plus placebo.
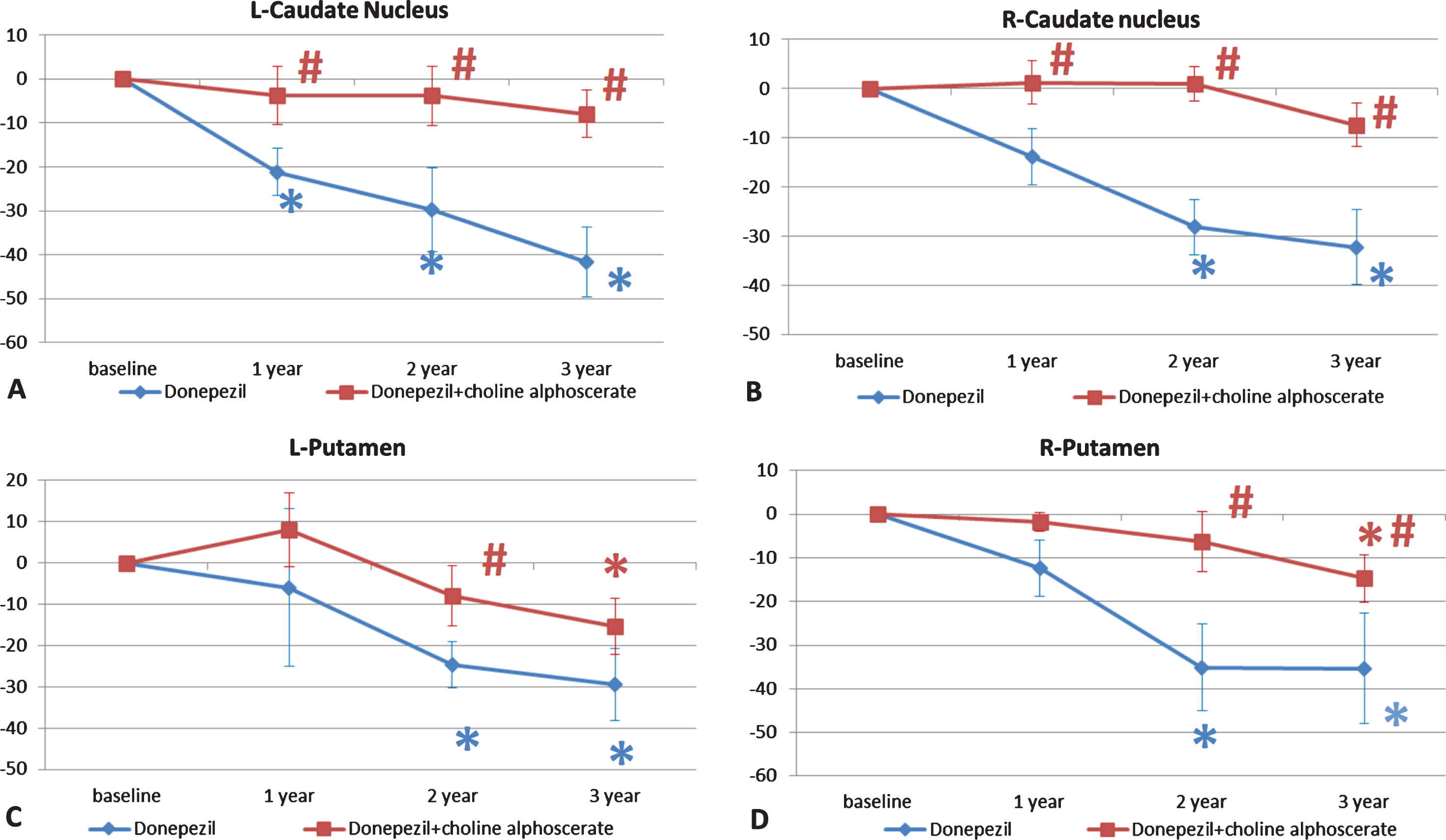
A gradual reduction of the volume of the caudate nucleus, more pronounced in the D + P group was also observed (Fig. 6A, B). Analysis of the volume of the putamen revealed progressive atrophy, with a slower rate in the D + CA group of patients. This reduction was statistically significant in the left putamen after two years of treatment (Fig. 6C) and in the right putamen starting from 2 years of treatment (Fig. 6D).
Fig. 6
Changes in the parahippocampal gyrus (A), superior (B) and middle (C) frontal gyri, and globus pallidus (D) in the two groups of patients along the three years of observation. The data are the means of the percentage variation±S.E.M. *p < 0.05 versus baseline; #p < 0.05 versus monotherapy with donepezil alone.
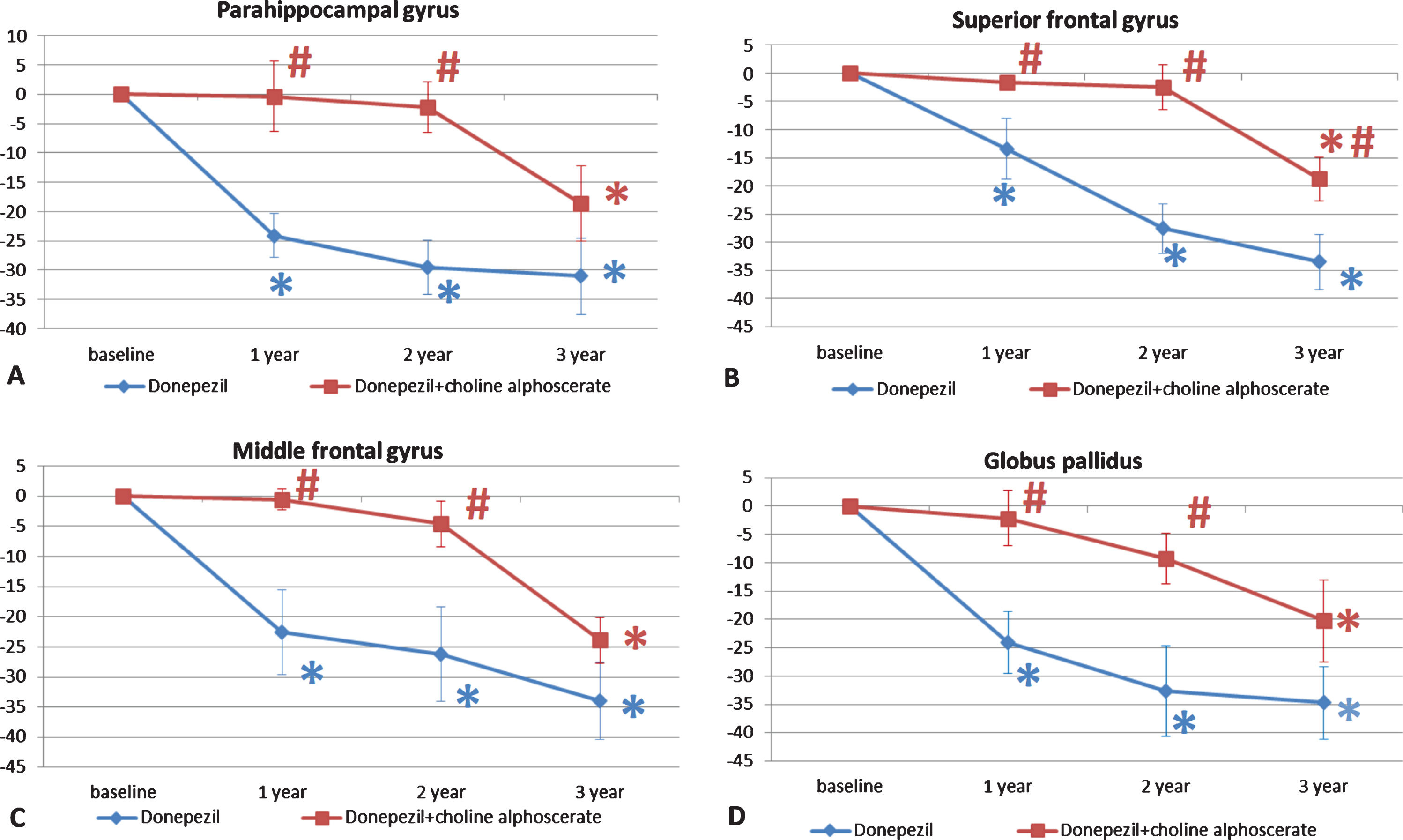
Volume analysis of the globus pallidus revealed a similar trend, with progressive atrophy during the three years of treatment, which was less pronounced in patients treated with D + CA (Fig. 5D). Analysis of the superior and middle frontal gyri revealed in the first of the two frontal cortex areas statistically significant differences between the 2 patient groups over the three years of treatment (Fig. 5B). In the middle frontal gyrus, the difference was statistically significant in the first two years of treatment (Fig. 5C).
Correlation between brain area volumes and neuropsychological tests were evaluated and detailed in Table 2.
Table 2
Evaluation of the correlation between neuropsychological tests and brain volume changes
Gray matter | White matter | CSF | ||
MMSE | Pearson’s Correlation | 0.70161 | 0.68361 | -0.25271 |
p value | 3,75255E-14* | 6.95737E-11* | 0.0182* | |
ADAS-Cog | Pearson’s Correlation | –0.5669 | –0.56005 | 0.26828 |
p value | 1.03909E-8* | 4.62971E-7* | 0.01199* | |
BADL | Pearson’s Correlation | 0.43894 | 0.56049 | –0.19965 |
p value | 2.11079E-5* | 4.51634E-7* | 0.06374 | |
IADL | Pearson’s Correlation | 0.3533 | 0.36629 | –0.27626 |
p value | 7.89015E-4* | 0.00182* | 0.0096* | |
NPI FxS | Pearson’s Correlation | 0.00397 | –0.15627 | –0.15072 |
p value | 0.9709 | 0.1964 | 0.16347 | |
NPI Distress | Pearson’s Correlation | –0.07052 | –0.10924 | 0.0048 |
p value | 0.51631 | 0.36801 | 0.96484 |
*p < 0.05 correlation at Pearson’s test.
DISCUSSION
The study of changes in the human brain through neuroimaging techniques has undergone great development. In particular the study of mesial temporal atrophy, focusing on the hippocampus, a key structure in the development of AD, has proved to be a sensitive and suitable approach to confirm the presence of AD. It has been demonstrated that the hippocampus exhibits degeneration even before the onset of symptoms and is the first region to degenerate followed by other brain areas at later stages. Construction of morphological models of the hippocampus is a starting point of fundamental importance in the identification of the tools that enable early diagnosis of the disease [49].
ChE-I are the drugs most widely used to improve cholinergic neurotransmission in patients with AD. Some studies have suggested that ChE-I exerts neuroprotective effects, which could slow down the progression of the disease [50], although the benefits and costs/benefits of acetylcholinesterase/ChE-I are modest and, according to some studies, of doubtful clinical relevance [51]. One of the main problems of ChE-I therapy is the decrease in treatment efficacy over time [52]. Another problem is the treatment of particular categories of patients (very old subjects, i.e., over 85 years old, or patients with bradycardia, bronchial asthma, or chronic obstructive pulmonary disease) in which ChE-Is are not indicated [53]. The use of ChE-I at higher doses is also potentially associated with major side effects. Consequently, patients have to be selected carefully and then monitored.
Cholinergic precursors were among the first compounds used for treating AD, but their activity was modest if any in clinical trials [30]. Choline alphoscerate is, among the clinically tested cholinergic precursors, the compound that has probably shown the greatest efficacy, as well as good tolerability in patients with mild to moderate AD and vascular dementia [53]. Since it crosses the blood-brain barrier easily, it probably acts as a metabolically active choline donor in the brain and has proved to exert neuroprotective effects in experimental animals with vascular cerebral lesions.
The cholinergic basal structures of the forebrain involved in cognitive activities are particularly sensitive to ischemia. This observation could explain the marked cholinergic deficits reported in the vascular neurodegenerative forms of dementia [54]. Based on these considerations, ASCOMALVA recruited patients with AD associated with vascular injury, i.e., a patient population characterized by marked cholinergic hypofunction [39, 40], who could benefit from a strong cholinergic support given by the combination of high doses of choline alphoscerate with 10 mg of donepezil a day (D + CA). Neuropsychological testing revealed a statistically significant advantage in patients treated with D + CA versus D + P. The efficacy of D + CA was greater mainly on cognitive and behavioral symptoms, with a significant reduction in caregiver stress starting from the first year. With regard to daily functional activities, the difference became significant after two years of treatment. Advantages with D + CA were noticeable primarily after long-term (two years) treatment, suggesting that some time is needed to detect the activity of choline alphoscerate.
AD is characterized by a decrease in the weight and volume of the brain, due to cortical atrophy, with enlargement of the grooves and corresponding flattening of the convolutions. Cerebral atrophy involving primarily the hippocampus is related to the progression of cognitive impairment and to conversion from mild cognitive impairment to overt AD [42, 43]. Hippocampal volume is one of the main biomarkers of AD and the morphometric analysis of this parameter based on MRI is one of the most used systems to assess the progression of AD [44]. Amygdala atrophy has previously been identified in other neurodegenerative disorders, such as frontotemporal dementia, Lewy body dementia, and vascular dementia [45, 46], as it plays a fundamental role in the enhancement of explicit memory for both pleasant and unpleasant emotional stimuli, modulating codification and consolidation processes [47, 48].
In MRI, water, the main component of the CSF, is darker than cellular matter and thus easily recognizable. Measures of the various shades of gray of the hippocampus and surrounding areas enable estimation of the degree of atrophy. In several studies, hippocampal atrophy was assessed by manual analysis of MRI images. Manual analysis of hippocampal atrophy is time consuming and has the potential bias deriving from subjective analysis [49]. It is therefore preferable to use automated systems performing analysis of MRI images regardless of the presence or absence of the disease. MRI studies have shown significant hippocampal atrophy in patients with AD, with a 30–40% reduction compared to controls. The MRI examination enables detailed visualization of the hippocampus and after the acquisition of a high-resolution 3D MRI sequence of the human brain, images can be processed using specific algorithms and then processed according to validated protocols [44, 55–57].
The analysis of the data referring to the patients of the ASCOMALVA study revealed predictable cerebral atrophy involving primarily the hippocampus and amygdala, in all patients with compensatory increase in CSF volume. The rate of progression of the atrophy was significantly higher in the group receiving only donepezil. The reduction of atrophy in the hippocampal and amygdaloid areas is consistent with the clinical efficacy shown in terms of reduction in cognitive loss (MMSE and ADAS-Cog scores) and in the improvement in behavioral parameters, as well as with improvement in caregiver perceptions (NPI). Correlation study shows that cognitive and functional tests are strongly related to gray and white matter atrophy, with a weaker (but significant) relation to CSF volume increase. No significant relationship was found between behavioral tests and brain volume changes.
The less pronounced hippocampal and amygdaloid atrophy occurring in patients receiving choline alphoscerate in addition to standard donepezil treatment suggests that the marked cholinergic challenge induced by the ChE-I + cholinergic precursor treatment may slow-down the progression of brain atrophy typical of AD. Our findings confirmed the slower atrophic progression involving frontal convolutions and the slower worsening of behavioral performances, especially in terms of apathy, which had been previously observed in the patients of the study [58, 59].
A limitation of this study is the global cognitive and behavior assessment. This limitation should be addressed in a future study with the evaluation of ADAS-cog subtests scores and NPI subtest scores, in a larger sample group. The sample size of the present study is rather small and therefore the results obtained should be interpreted with caution and hopefully replicated and confirmed in future independent studies.
The results of our study are related to a population of patients with AD associated with vascular damage, which represents, in absolute terms, the largest proportion of subjects with dementia, as demonstrated by pathological [60], epidemiological [61], and retrospective studies [62]. Patients with vascular lesions should be carefully monitored, because they are the most likely to undergo rapid disease progression [63].
Based on our finding we can suggest that cognitive impairment associated with cerebrovascular involvement may be more effectively countered, similarly as shown in preclinical studies, by combining a cholinergic precursor as choline alphoscerate to standard treatment with ChE-I. These two treatments in combination seem to exert synergistic effects and, consequently, could represent a therapeutic option to be considered in AD associated with cerebrovascular damage.
ACKNOWLEDGMENTS
The authors thank MDM SpA, Monza (Italy) for having provided choline alphoscerate and placebo used in the ASCOMALVA trial and for supporting with a bursary neuropsychological work of Dr. Anna Carotenuto. She was also supported by a postdoc fellowship (assegno di ricerca) from the University of Camerino.
Authors’ disclosures available online (https://www.j-alz.com/manuscript-disclosures/19-0623r3).
REFERENCES
[1] | Alzheimer A ((1906) ) Über einen eigenartigen schweren erkrankungsprozeβ der hirnrincle. Neurol Cent 25: , 1134. |
[2] | Perl DP ((2010) ) Neuropathology of Alzheimer’s disease. Mt Sinai J Med 77: , 32–42. |
[3] | Serrano-Pozo A , Frosch MP , Masliah E , Hyman BT ((2011) ) Neuropathological alterations in Alzheimer disease. Cold Spring Harb Perspect Med 1: , a006189. |
[4] | Cavedo E , Pievani M , Boccardi M , Galluzzi S , Bocchetta M , Bonetti M , Thompson PM , Frisoni GB ((2014) ) Medial temporal atrophy in early and late-onset Alzheimer’s disease. Neurobiol Aging 35: , 2004–2012. |
[5] | Terry AV Jr , Buccafusco JJ ((2003) ) The cholinergic hypothesis of age and Alzheimer’s disease-related cognitive deficits: Recent challenges and their implications for novel drug development. J Pharmacol Exp Ther 306: , 821–827. |
[6] | Schaeffer EL , Gattaz WF ((2008) ) Cholinergic and glutamatergic alterations beginning at the early stages of Alzheimer disease: Participation of the phospholipase A2 enzyme. Psychopharmacology (Berl) 198: , 1–27. |
[7] | Liskowsky W , Schliebs R ((2006) ) Muscarinic acetylcholine receptor inhibition in transgenic Alzheimer-like Tg2576 mice by scopolamine favours the amyloidogenic route of processing of amyloid precursor protein. Int J Dev Neurosci 24: , 149–156. |
[8] | Saraf MK , Anand A , Prabhakar S ((2010) ) Scopolamine induced amnesia is reversed by Bacopa monniera through participation of kinase-CREB pathway. Neurochem Res 35: , 279–287. |
[9] | Saraf MK , Prabhakar S , Khanduja KL , Anand A ((2011) ) Bacopa monniera attenuates scopolamine induced impairment of spatial memory in mice. Evid Based Complement Alternat Med 2011: , 236186. |
[10] | Li Y , Yuan X , Shen Y , Zhao J , Yue R , Liu F , He W , Wang R , Shan L , Zhang W ((2016) ) Bacopaside I ameliorates cognitive impairment in APP/PS1 mice via immune-mediated clearance of β-amyloid. Aging (Albany NY) 8: , 521–533. |
[11] | Caccamo A , Oddo S , Billings LM , Green KN , Martinez-Coria H , Fisher A , LaFerla FM ((2006) ) M1 receptors play a central role in modulating AD-like pathology in transgenic mice. Neuron 49: , 671–682. |
[12] | Fisher A , Medeiros R , Barner N , Natan N , Brandeis R , Elkon H , Nahum V , Grigoryan G , Segal M , LaFerla F ((2014) ) M1 muscarinic agonists and a multipotent activator of sigma1/M1 muscarinic receptors: Future therapeutics of Alzheimer’s disease. Alzheimers Demen 10: , P123. |
[13] | Ellis JR , Ellis KA , Bartholomeusz CF , Harrison BJ , Wesnes KA , Erskine FF , Vitetta L , Nathan PJ ((2006) ) Muscarinic and nicotinic receptors synergistically modulate working memory and attention in humans. Int J Neuropsychopharmacol 9: , 175–189. |
[14] | Ringman JM , Cummings JL ((2006) ) Current and emerging pharmacological treatment options for dementia. Behav Neurol 17: , 5–16. |
[15] | Wu J , Ishikawa M , Zhang J , Hashimoto K ((2010) ) Brain imaging of nicotinic receptors in Alzheimer’s disease. Int J Alzheimers Dis 2010: , 548913. |
[16] | Dong XX , Wang Y , Qin Z ((2009) ) Molecular mechanisms of excitotoxicity and their relevance to pathogenesis of neurodegenerative diseases. Acta Pharmacol Sin 30: , 379–387. |
[17] | Geerts H , Grossberg GT ((2006) ) Pharmacology of acetylcholinesterase inhibitors and N-methyl-D-aspartate receptors for combination therapy in the treatment of Alzheimer’s disease.8S–16S. J Clin Pharmacol 46: . |
[18] | Lin H , Vicini S , Hsu FC , Doshi S , Takano H , Coulter DA , Lynch DR ((2010) ) Axonal α7 nicotinic ACh receptors modulate presynaptic NMDA receptor expression and structural plasticity of glutamatergic presynaptic boutons. Proc Natl Acad Sci U S A 107: , 16661–16666. |
[19] | Mohandas E , Rajmohan V , Raghunath B ((2009) ) Neurobiology of Alzheimer’s disease. Indian J Psychiatry 51: , 55. |
[20] | Doggrell SA , Evans S ((2003) ) Treatment of dementia with neurotransmission modulation. Expert Opin Investig Drugs 12: , 1633–1654. |
[21] | Peña F , Gutiérrez-Lerma A , Quiroz-Baez R , Arias C ((2006) ) The role of beta-amyloid protein in synaptic function: Implications for Alzheimer’s disease therapy. Curr Neuropharmacol 4: , 149–163. |
[22] | Jack CR Jr , Holtzman DM ((2013) ) Biomarker modeling of Alzheimer’s disease. Neuron 80: , 1347–1358. |
[23] | Albert MS , DeKosky ST , Dickson D , Dubois B , Feldman HH , Fox NC , Gamst A , Holtzman DM , Jagust WJ , Petersen RC , Snyder PJ , Carrillo MC , Thies B , Phelps CH ((2011) ) The diagnosis of mild cognitive impairment due to Alzheimer’s disease: Recommendations from the National Institute on Aging-Alzheimer’s Association workgroups on diagnostic guidelines for Alzheimer’s disease. Alzheimers Dement 7: , 270–279. |
[24] | McKhann GM , Knopman DS , Chertkow H , Hyman BT , Jack CR Jr , Kawas CH , Klunk WE , Koroshetz WJ , Manly JJ , Mayeux R , Mohs RC , Morris JC , Rossor MN , Scheltens P , Carrillo MC , Thies B , Weintraub S , Phelps CH ((2011) ) The diagnosis of dementia due to Alzheimer’s disease: Recommendations from the National Institute on Aging-Alzheimer’s Association workgroups on diagnostic guidelines for Alzheimer’s disease. Alzheimers Dement 7: , 263–269. |
[25] | Jack CR Jr , Albert MS , Knopman DS , McKhann GM , Sperling RA , Carrillo MC , Thies B , Phelps CH ((2011) ) Introduction to the recommendations from the National Institute on Aging-Alzheimer’s Association workgroups on diagnostic guidelines for Alzheimer’s disease. Alzheimers Dement 7: , 257–262. |
[26] | Khan TK ((2018) ) An algorithm for preclinical diagnosis of Alzheimer’s disease. Front Neurosci 12: , 275. |
[27] | Nestor PJ , Altomare D , Festari C , Drzezga A , Rivolta J , Walker Z , Bouwman F , Orini S , Law I , Agosta F , Arbizu J , Boccardi M , Nobili F , Frisoni GB , EANM-EAN Task Force for the Prescription of FDG-PET for Dementing Neurodegenerative Disorders ((2018) ) Clinical utility of FDG-PET for the differential diagnosis among the main forms of dementia. Eur J Nucl Med Mol Imaging 45: , 1509–1525. |
[28] | Shimizu S , Hirose D , Hatanaka H , Takenoshita N , Kaneko Y , Ogawa Y , Sakurai H , Hanyu H ((2018) ) Role of neuroimaging as a biomarker for neurodegenerative diseases. Front Neurol 9: , 265. |
[29] | Giacobini E ((1998) ) Cholinergic foundation of Alzheimer’s disease therapy. J Physiol Paris 92: , 283–287. |
[30] | Amenta F , Parnetti L , Gallai V , Wallin A ((2001) ) Treatment of cholinergic dysfunction associated with Alzheimer’s disease with cholinergic precursors. Ineffective treatments or inappropriate approaches? Mech Ageing Dev 122: , 2025–2040. |
[31] | Gauthier S , Feldman H , Hecker J , Vellas B , Emir B , Subbiah P ((2002) ) Donepezil MSAD Study Investigators’ Group. Functional, cognitive and behavioral effects of donepezil in patients with moderate Alzheimer’s disease. Curr Med Res Opin 18: , 347–354. |
[32] | Grantham C , Geerts H ((2002) ) The rationale behind cholinergic drug treatment for dementia related to cerebrovascular disease. J Neurol Sci 203-204: , 131–136. |
[33] | Traini E , Bramanti V , Amenta F ((2013) ) Choline alphoscerate (alpha-glyceryl-phosphoryl-choline) an old choline-containing phospholipid with a still interesting profile as cognition enhancing agent. Curr Alzheimer Res 10: , 1070–1079. |
[34] | Parnetti L , Amenta F , Gallai V ((2001) ) Choline alphoscerate in cognitive decline and in acute cerebrovascular disease: An analysis of published clinical data. Mech Ageing Dev 122: , 2041–2155. |
[35] | Amenta F , Tayebati SK , Vitali D , Di Tullio MA ((2006) ) Association with the cholinergic precursor coline alphoscerate and the cholinesterase inhibitor rivastigmine: An approach for enhancing cholinergic neurotransmission. Mech Ageing Dev 127: , 173–179. |
[36] | Tayebati SK , Di Tullio MA , Tomassoni D , Amenta F ((2009) ) Neuroprotective effect of treatment with galantamine and choline alphoscerate on brain microanatomy in spontaneously hypertensive rats. J Neurol Sci 283: , 187–194. |
[37] | Amenta F , Carotenuto A , Fasanaro AM , Rea R , Traini E ((2012) ) The ASCOMALVA trial: Association between the cholinesterase inhibitor donepezil and the cholinergic precursor choline alphoscerate in Alzheimer’s disease with cerebrovascular injury: Interim results. J Neurol Sci 322: , 96–101. |
[38] | Amenta F , Carotenuto A , Fasanaro AM , Rea R , Traini E ((2014) ) The ASCOMALVA (Association between the Cholinesterase Inhibitor Donepezil and the Cholinergic Precursor Choline Alphoscerate in Alzheimer’s Disease) Trial: Interim results after two years of treatment.S. J Alzheimers Dis 42: , 281–288. |
[39] | Auld DS , Kornecook TJ , Bastianetto S , Quinron R ((2002) ) Alzheimer’s disease and the basal forebrain cholinergic system: Relations to beta-amyloid peptides, cognition, and treatment strategies. Prog Neurobiol 68: , 209–245. |
[40] | Schliebs R , Arendt T ((2006) ) The significance of the cholinergic system in the brain during aging and in Alzheimer’s disease. J Neural Transm 113: , 1625–2644. |
[41] | McKhann G , Drachman D , Folstein M , Katzman R , Price D , Stadlan EM ((1984) ) Clinical diagnosis of Alzheimer’s disease: Report of the NINCDS-ADRDA Work Group under the auspices of Department of Health and Human Services Task Force on Alzheimer’s Disease. Neurology 34: , 939–44. |
[42] | Jack CR Jr , Shiung MM , Gunter JL , O’Brien PC , Weigand SD , Knopman DS , Boeve BF , Ivnik RJ , Smith GE , Cha RH , Tangalos EG , Petersen RC ((2004) ) Comparison of different MRI brain atrophy rate measures with clinical disease progression in AD. Neurology 62: , 591–600. |
[43] | Carmichael OT , Lopez O , Becker JT , Kuller L ((2009) ) Trajectories of brain loss in aging and the development of cognitive impairment. Neurology 72: , 771–772. |
[44] | Laakso MP , Hallikainen M , Hanninen T , Partanen K , Soininen H ((2000) ) Diagnosis of Alzheimer’s disease: MRI of the hippocampus vs delayed recall. Neuropsychologia 38: , 579–584. |
[45] | Thompson PM , Hayashi KM , de Zubicaray GI , Janke AL , Rose SE , Semple J , Hong MS , Herman DH , Gravano D , Doddrell DM , Toga AW ((2004) ) Mapping hippocampal and ventricular change in Alzheimer disease. Neuroimage 22: , 1754–1766. |
[46] | Cavedo E , Boccardi M , Ganzola R , Canu E , Beltramello A , Caltagirone C , Thompson PM , Frisoni GB ((2011) ) Local amygdala structural differences with 3T MRI in patients with Alzheimer disease. Neurology 76: , 727–733. |
[47] | Hamann S ((2001) ) Cognitive and neural mechanisms of emotional memory. Trends Cogn Sci 5: , 394–400. |
[48] | Prestia A , Boccardi M , Galluzzi S , Cavedo E , Adorni A , Soricelli A , Bonetti M , Geroldi C , Giannakopoulos P , Thompson P , Frisoni G ((2011) ) Hippocampal and amygdalar volume changes in elderly patients with Alzheimer’s disease and schizophrenia. Psychiatry Res 192: , 77–83. |
[49] | Chetelat G , Chupin M , Desgranges B , Magnin B , Benali H , Dubois B , Garnero L , Eustache F , Lehéricy S , Colliot O ((2008) ) Discrimination between Alzheimer disease, mild cognitive impairment, and normal aging by using automated segmentation of the hippocampus. Radiology 248: , 194–201. |
[50] | Francis PT , Ramírez MJ , Lai MK ((2010) ) Neurochemical basis for symptomatic treatment of Alzheimer’s disease. Neuropharmacology 59: , 221–229. |
[51] | Kavirajan H , Schneider LS ((2007) ) Efficacy and adverse effects of cholinesterase inhibitors and memantine in vascular dementia: A meta-analysis of randomised controlled trials. Lancet Neurol 6: , 782–792. |
[52] | Hogan DB ((2014) ) Long-term efficacy and toxicity of cholinesterase inhibitors in the treatment of Alzheimer disease. Can J Psychiatry 59: , 618–623. |
[53] | Parnetti L , Mignini F , Tomassoni D , Traini E , Amenta F ((2007) ) Cholinergic precursors in the treatment of cognitive impairment of vascular origin: Ineffective approaches or need for reevaluation? J Neurol Sci 257: , 264–269. |
[54] | Román GC ((2003) ) Vascular dementia: Distinguishing characteristics, treatment, and prevention. J Am Geriatr Soc 51: , S296–304. |
[55] | Frisoni GB , Testa C , Zorzan A , Sabattoli F , Beltramello A , Soininen H , Laakso MP ((2002) ) Detection of grey matter loss in mild Alzheimer’s disease with voxel based morphometry. J Neurol Neurosurg Psychiatry 73: , 657–664. |
[56] | Laakso MP , Frisoni GB , Kononen M , Mikkonen M , Beltramello A , Geroldi C , Bianchetti A , Trabucchi M , Soininen H , Aronen HJ ((2000) ) Hippocampus and entorhinal cortex in frontotemporal dementia and Alzheimer’s disease: A morphometric MRI study. Biol Psychiatry 47: , 1056–1063. |
[57] | Frisoni GB , Scheltens P , Galluzzi S , Nobili FM , Fox NC , Robert PH , Soininen H , Wahlund LO , Waldemar G , Salmon E ((2003) ) Neuroimaging tools to rate regional atrophy, subcortical cerebrovascular disease, and regional cerebral blood flow and metabolism: Consensus paper of the EADC. J Neurol Neurosurg Psychiatry 74: , 1371–1381. |
[58] | Rea R , Carotenuto A , Traini E , Fasanaro AM , Manzo V , Amenta F ((2015) ) Apathy treatment in Alzheimer’s disease: Interim results of the ASCOMALVA trial. J Alzheimers Dis 48: , 377–83. |
[59] | Carotenuto A , Rea R , Traini E , Fasanaro AM , Ricci G , Manzo V , Amenta F ((2017) ) The effect of the association between donepezil and choline alphoscerate on behavioral disturbances in Alzheimer’s disease: Interim results of the ASCOMALVA Trial. J Alzheimers Dis 56: , 805–815. |
[60] | Snowdon DA , Greiner LH , Mortimer JA , Riley KP , Greiner PA , Markesbery WR ((1997) ) Brain infarction and the clinical expression of Alzheimer disease. The Nun Study. JAMA 277: , 813–817. |
[61] | Aguero-Torres H , Kivipelto M , von Strauss E ((2006) ) Rethinking the dementia diagnoses in a population-based study: What is Alzheimer’s disease and what is vascular dementia? A study from the kungsholmen project. Dement Geriatr Cogn Disord 22: , 244–249. |
[62] | Carotenuto A , Rea R , Colucci L , Ziello AR , Molino I , Carpi S , Traini E , Amenta F , Fasanaro AM ((2012) ) Late and early onset dementia: What is the role of vascular factors? A retrospective study. J Neurol Sci 322: , 170–175. |
[63] | Viswanathan A , Rocca WA , Tzourio C ((2009) ) Vascular risk factors and dementia: How to move forward? Neurology 72: , 368–374. |