Effects of Regular and Long-Acting Insulin on Cognition and Alzheimer’s Disease Biomarkers: A Pilot Clinical Trial
Abstract
Background: Long acting insulin detemir administered intranasally for three weeks enhanced memory for adults with Alzheimer’s disease dementia (AD) or amnestic mild cognitive impairment (MCI). The investigation of longer-term administration is necessary to determine whether benefits persist, whether they are similar to benefits provided by regular insulin, and whether either form of insulin therapy affects AD biomarkers.
Objective: The present study aimed to determine whether four months of treatment with intranasal insulin detemir or regular insulin improves cognition, daily functioning, and AD biomarkers for adults with MCI or AD.
Methods: This randomized, double-blind, placebo-controlled trial included an intent-to-treat sample consisting of 36 adults diagnosed with MCI or mild to moderate AD. Participants received placebo (n = 12), 40 IU of insulin detemir (n = 12), or 40 IU of regular insulin (n = 12) daily for four months, administered with a nasal delivery device. A cognitive battery was administered at baseline and after two and four months of treatment. MRI was administered for all participants and lumbar puncture for a subset (n = 20) at baseline and four months. The primary outcome was change from baseline to four months on a memory composite (sum of Z scores for delayed list and story recall). Secondary outcomes included: global cognition (Alzheimer’s Disease Assessment Scale-Cognition), daily functioning (Dementia Severity Rating Scale), MRI volume changes in AD-related regions of interest, and cerebrospinal fluid AD markers.
Results: The regular insulin treated group had better memory after two and four months compared with placebo (p < 0.03). No significant effects were observed for the detemir-assigned group compared with the placebo group, or for daily functioning for either group. Regular insulin treatment was associated with preserved volume on MRI. Regular insulin treatment was also associated with reduction in the tau-P181/Aβ42 ratio.
Conclusion: Future research is warranted to examine the mechanistic basis of treatment differences, and to further assess the efficacy and safety of intranasal insulin.
INTRODUCTION
Efficient insulin metabolism is a key determinant of healthy aging, and conditions associated with insulin dysregulation increase the risk of developing Alzheimer’s disease (AD) and related dementias, as well as impacting AD pathological processes [1]. Insulin protects against synaptotoxic effects of amyloid-β (Aβ) [2, 3], and reduces tau hyperphosphorylation in preclinical models [4]. In human AD brain, abnormal serine phosphorylation of insulin signaling molecules that impede its actions are co-localized with neurofibrillary tangles [5]. Reduced levels of insulin in cerebrospinal fluid (CSF) have also been reported in AD, possibly due to reduced transport of insulin across the blood-brain barrier (BBB) [6]. Perturbed insulin metabolism in AD may have important pathophysiologic consequences, as insulin has been implicated in clearance of Aβ across the BBB, in tau phosphorylation, and in memory via its effects on synaptic function and long-term potentiation [1]. Enhancing brain insulin function may thus be a viable approach to ameliorating AD symptoms and attenuating AD-related pathophysiologicprocesses.
One approach to addressing brain insulin dysregulation is to provide insulin directly to the brain via intranasal delivery. Intranasally administered peptides travel via convective bulk flow along perivascular pathways following the olfactory and trigeminal nerves and bypassing the BBB; through these pathways, peptides can rapidly access hippocampus and cortex within 15 to 30 minutes [7]. Little or no peptide reaches the peripheral circulation [8]. Thus intranasal insulin delivery is not affected by impaired BBB transport, and does not induce peripheral hypoglycemia. Several prior studies in adults with amnestic mild cognitive impairment (MCI) or AD have demonstrated beneficial effects on cognition, activities of daily living, or cerebral glucose metabolism [9, 10]. Prior studies have also demonstrated that the therapeutic response to intranasal insulin may differ for carriers of the apolipoprotein E ɛ4 (APOE ɛ4) allele, the most potent genetic risk factor for sporadic AD [11].
Several forms of insulin are commercially available that differ in terms of their pharmacokinetic profile. Most previous clinical trials used regular insulin, which has a relatively short half-life and mimics post-prandial release. Long-acting forms of insulin have been developed that provide a more protracted profile that results in longer exposure and less risk of acute hypoglycemia. The long-acting insulin analog insulin detemir was developed via acylation of a 14-carbon fatty acid to lysine at locus B29, and as a result has an increased tendency toward self-association and reversible albumin binding which delays its absorption [12, 13]. It has been proposed that its increased lipophilicity may enable it to better penetrate brain parenchyma and induce greater cognitive benefit [14]. In an initial three-week pilot study, intranasal insulin detemir improved memory, with no noted safety issues [9]. In the current study, we examined the effects of longer-term (four month) insulin detemir therapy compared with placebo on memory and on AD imaging and CSF biomarkers. The effects of regular insulin compared with placebo were also assessed. Given previous findings of APOE-related differences in treatment response, ApoE ɛ4 carrier status was examined as a possible mediating factor in pre-specified secondary analyses.
MATERIALS AND METHODS
Participants
The trial was registered at clinicaltrials.gov (NCT01595646) and conducted over a 2-year period at the Veterans Affairs Puget Sound Health Care System and the Wake Forest School of Medicine. The study was approved by the Institutional Review Boards of the University of Washington, the Veterans Affairs Puget Sound Health Care System and Wake Forest, and was conducted in the Veterans Affairs and Wake Forest Clinical Research Units. Written informed consent was obtained from all participants and/or legally designated representatives. A total of 36 older adults enrolled in the study (22 participants with amnestic MCI, and 14 participants with probable AD with Mini-Mental State Examination (MMSE) scores >15). Diagnoses and eligibility were determined by consensus of expert physicians and neuropsychologists following cognitive testing, evaluation of medical history, physical examination, and clinical laboratory screening using modified Petersen criteria for the diagnosis of amnestic MCI [15], and National Institute for Neurological and Communicative Disorders and Stroke–Alzheimer’s Disease and Related Disorders Association criteria for AD [16]. When evaluating cognitive performance, test scores were compared with an age- and education-adjusted estimate of the participant’s premorbid intellectual ability using the Shipley-2 Vocabulary test. Scores 1.5 SD or more below this estimate were considered to be in the impaired range, and diagnosis was then determined by expert consensus using all available clinical and cognitive data, following published criteria [15].
Participants were free from alcoholism, severe head trauma, hypoxia, neurologic disorders other than amnestic MCI or AD, renal or hepatic disease, diabetes mellitus, chronic obstructive pulmonary disease, unstable cardiac disease and psychiatric disease other than mild controlled depression. Participants and all study personnel involved in data collection were blinded to treatment assignment. Treatment groups did not differ significantly in terms of age, education, body mass index, general cognitive status as assessed by the modified MMSE, sex, diagnosis, whether they received anticholinesterase inhibitors or memantine, or percentage of APOE ɛ4 carriers. Participants receiving anti-diabetic medications or agents with prominent CNS effects (other than anti-depressants and AD medications) were excluded. Enrollment data are presented in Fig. 1, and demographic information in Table 1.
Fig.1
Enrollment data for study participants.
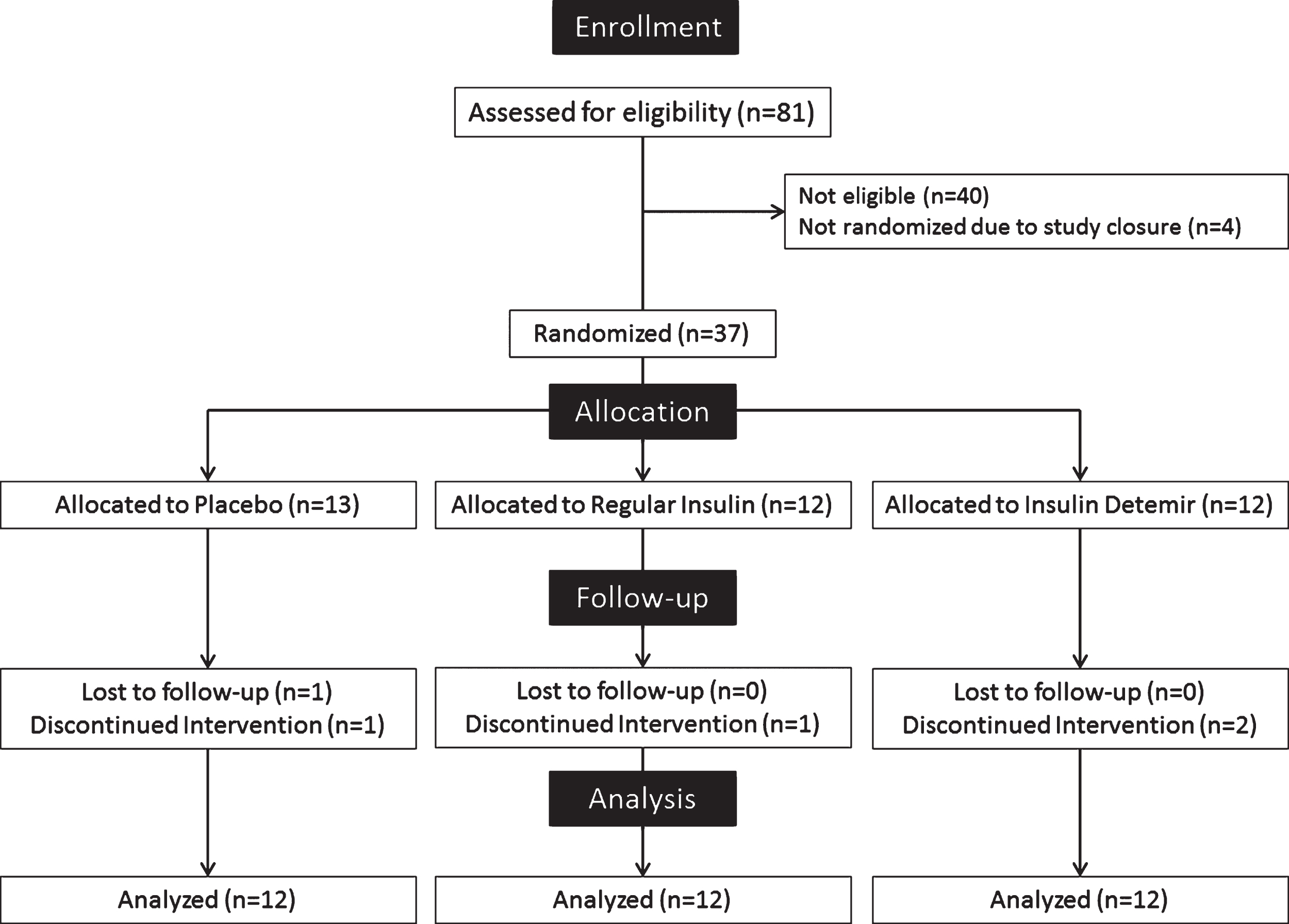
Table 1
Demographic characteristics of study participants
Placebo n = 12 | Regular Insulin n = 12 | Detemir n = 12 | |
Age (y) | 68.4 (8.9) | 70.5 (9.1) | 67.3 (7.8) |
Education (y) | 16.5 (2.0) | 15.6 (2.8) | 14.8 (2.4) |
MMSE | 24.8 (4.2) | 26.0 (3.7) | 25.2 (4.1) |
Sex (F/M) | 6/6 | 7/5 | 6/6 |
Diagnosis (MCI/ AD) | 8/4 | 7/5 | 7/5 |
ApoE4 +/– | 8/4 | 8/4 | 6/6 |
Body mass index | 26.7 (3.3) | 28.8 (6.1) | 29.4 (6.6) |
Procedures
Participants were randomly assigned to receive a daily dosage of 40 IU of insulin detemir (20 IU detemir b.i.d.), 40 IU of regular insulin (20 IU regular insulin b.i.d.), or placebo (saline b.i.d.) for 4 months. Saline, regular insulin (Humulin R U-100, Eli Lilly and Co.), or insulin detemir (Levemir®; Novo Nordisk, Princeton, New Jersey) was administered 30 minutes after breakfast and dinner with a ViaNase nasal delivery device (Kurve Technology, Bothell, WA) designed to deliver drugs to the paranasal sinuses containing the olfactory cleft region, a dispersion pattern postulated to maximize transport to the central nervous system compared with more diffuse dispersion, potentially due to vascular characteristics of this region [17, 18]. The Kurve device uses a controlled particle dispersion platform that produces a vertical distribution of particles between 15–20μm, a size shown to minimize peripheral deposition (http://www.kurvetech.com/pdf/ONdrugDelivery-Kurve.pdf). This device had been used successfully in a previous 4-month study of intranasal insulin [10]. Participants inserted a needle-less syringe containing the measured amount of insulin into the device chamber. The device then released a metered dose of saline or insulin into a chamber covering the participant’s nose over a 2-minute period, which was inhaled by breathingregularly.
Cognitive/functional measures
Parallel versions of the cognitive and functional protocol were administered at baseline and after 2 and 4 months of treatment. Testing occurred in the morning after a standard meal. Participants were instructed to skip their morning dose on the day of testing and thus had received their last dose more than 12 h prior to cognitive testing. The primary outcome measure was a delayed verbal memory composite score, calculated from the sum of z-scores from delayed story recall and delayed Selective Reminding Test recall. As previously described, delayed story recall was determined after a story containing 44 informational bits was read a single time to participants who were then asked to recall the story immediately and again after a 20-minute delay [19]. Delayed word list recall scores were derived from a 12-word Selective Reminding Word List task [20]. A higher score on the verbal memory composite indicates a better performance on these verbal memory measures. A general measure of cognition, the Alzheimer’s Disease Assessment Scale for Cognition [21] was also administered, using a version that included delayed list recall (ADAS-Cog12). The ADAS-Cog12 includes measures of memory, praxis, orientation, and language. Higher scores indicate greater impairment. Finally, functional ability was measured by the Dementia Severity Rating Scale (DSRS) [22], in which the study partner rated the change in the participant’s cognitive, social, and functional status over a specified period of time, with higher scores indicating greater impairment.
MRI
Images were acquired on a 3.0 T Siemens Skyra (Siemens Healthcare GmbH, Erlangen, Germany) or 3.0 T Phllips Achieva MRI scanner (Koninklijke Philips N.V., Eindhoven, Netherlands). T1 anatomical images were obtained using a 3D volumetric MPRAGE sequence with isotropic resolution of 1.0 mm (For Siemens Skyra: TR = 2300 ms; TE = 2.9 8 ms; TI = 900 ms; FA = 9; 192 slices, and for Philips Achieva: TR = 5.42 ms; TE = 2.64; TI = 1000; FA = 8; 176 slices). Images were processed using Wake Forest’s automated processing pipeline that features distributed grid processing, automated error recovery, and data provenance [23]. T1 images were segmented into grey matter, white matter and CSF, normalized to Montreal Neurologic Imaging (MNI) space, and modulated with the Jacobian determinants of the warping procedure to generate volumetric tissue maps using the Dartel high-dimensional warping and the SPM8 [24] new segment procedure, as implemented in the VBM8 toolbox (http://dbm.neuro.uni-jena.de/vbm.html). Region of interest (ROI)–based measures were generated using the automated anatomical labeling (AAL) atlas, as implemented in the wfu_pickatlas.
Lumbar puncture
CSF (25 ml) was collected in the morning after overnight fast using the standardized best practice guidelines recommended by the National Alzheimer’s Coordinating Center which include the use of 20 gauge Sprotte spinal needles and polypropylene tubes (https://www.alz.washington.edu/BiospecimenTaskForce.html). Concentrations of CSF Aβ42, tau, and phospho-tau (tau-P181) were measured with the AlzBio3 multiplex assay from FujiRebio Europe (formerly Innogenetics, Belgium).
Safety and compliance
Study partners supervised participants in the administration of intranasal treatment. Blood glucose levels were measured daily for the first week and then weekly thereafter; no episodes of hypoglycemia were observed. Compliance was monitored by quantifying unused medication and via self-report. Safety data were reviewed semiannually by a data safety monitoring board. Adverse event reporting followed standard guidelines.
Data analysis
Data were analyzed with SAS v9.3. A delayed memory composite (primary outcome) was constructed from the sum of Z scores for delayed story recall and delayed Selective Reminding Test. For the intent-to-treat group, the memory composite and ADAS-Cog12 total scores were subjected to mixed model repeated measures analysis of covariance (ANCOVA), with time (baseline, month 2, month 4) as the repeated measure, and treatment assignment as the between subjects measure. APOE ɛ4 allele carriage, gender, age, years of education, and baseline MMSE were included as covariates, and dropped if they were not substantively related to the model (p > 0.15). As pre-specified, one analysis was conducted comparing placebo and regular insulin, and a second analysis was conducted comparing placebo and insulin detemir. In the event of a significant time by treatment interaction, pre-specified contrasts between baseline and month 2, and baseline and month 4 were performed with means adjusted for significant covariates (LSMEANS). Missing cognitive data comprised <3% of total data (one participant in the regular insulin group dropped after baseline, and one participant in the insulin detemir group dropped after month 2), and were replaced by imputation. Additional sensitivity analyses were conducted using last observation carried forward (LOCF) and per protocol completer analyses and yielded comparable results. MRI analyses were restricted to brain volumes in 12 preselected ROIs most frequently affected in AD based on a meta-analysis by Wang et al. [25]: superior frontal, inferior frontal, superior temporal, and superior parietal cortices; parahippocampal gyrus, hippocampus, insula, gyrus rectus, cuneus/precuneus, and anterior, middle and posterior cingulate. Twenty participants agreed to LP for CSF biomarker measurement: Aβ42, total tau, tau-P181, and the calculated tau-P181/Aβ42 ratio; four participants did not have post-treatment values (two participants dropped as described above, and for two participants, post-treatment CSF could not be obtained). CSF and MRI data were subjected to a similar analytic strategy as cognitive variables with the same covariates, with the exception that only data from participants with both pre and post-values were analyzed.
RESULTS
Demographics
Sample characteristics are presented in Table 1. No differences were observed between treatment groups for any demographic variable.
Cognitive/functional outcomes
A significant time by treatment interaction was observed for the primary outcome (delayed memory composite) for placebo and regular insulin groups (p < 0.03; Fig. 2), with age, gender, and APOE ɛ4 carrier status retained as covariates in the model. Planned contrasts showed that participants assigned to regular insulin treatment had improved memory after 2 months and after 4 months compared with the placebo group (ps < 0.03 and 0.05; Fig. 2). No significant effects were observed for the detemir group compared with the placebo group.
Fig.2
Change from baseline for delayed memory composite Z score for placebo-assigned, regular insulin-treated, and detemir-treated groups. The regular insulin group had improved memory compared with the placebo group after two (p < 0.03) and four (p < 0.05) months of treatment. No differences were observed between placebo and detemir groups.
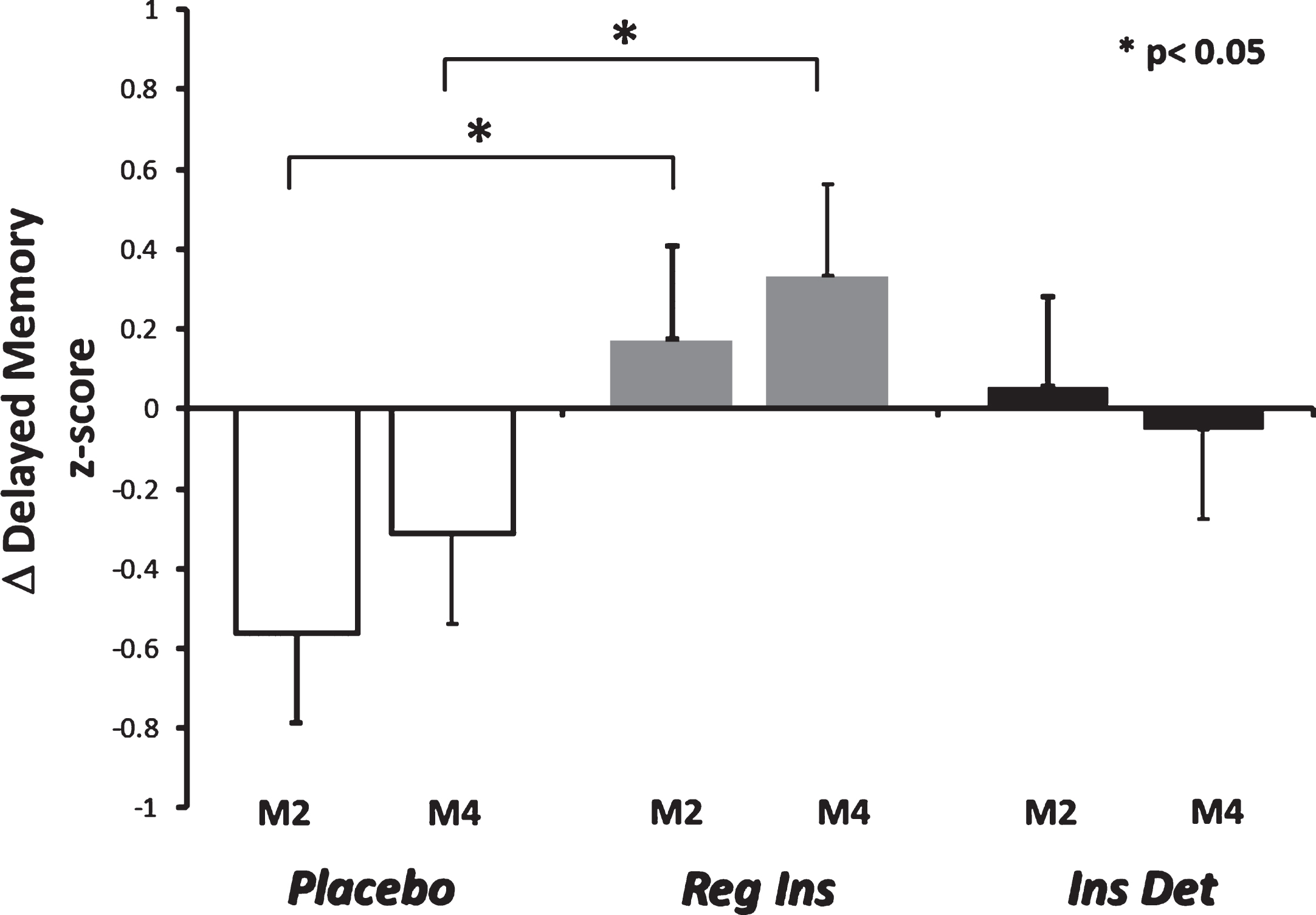
In previous studies, response to regular insulin and detemir was moderated by APOE genotype, and as noted APOE was retained as a significant contributor in the model for the delayed memory composite analysis that compared regular insulin and placebo groups. Although the present study is insufficiently powered to address the question of APOE-related treatment differences, we conducted exploratory analyses that could support investigation in future larger trials. At month 2, ɛ4 carriers treated with detemir had greater preservation of memory compared with placebo-assigned ɛ4 carriers (mean change from baseline in delayed memory composite for ɛ4 + placebo = –1.02, SEM = 0.28; for ɛ4 + detemir = –0.09, SEM = 0.32, p = 0.04). This effect is consistent with our previous report of detemir’s effects after short term administration [9]. However, by month 4, this effect had weakened (mean change from baseline in delayed memory composite for placebo = –0.55, SEM = 0.27; for detemir = 0.01, SEM = 0.31, p = 0.18).
Regarding APOE moderation of regular insulin effects, a pattern similar to previous reports was observed. For the regular insulin-treated group, ɛ4- participants showed greater improvement compared with ɛ4 + participants at month 2 (mean change from baseline in delayed memory composite for ɛ4– = 0.70, SEM = 0.41; for ɛ4 + = –0.33, SEM = 0.28, p < 0.05). At month 4, the ɛ4- regular insulin treated participants showed greater improvement than all other groups, an effect that reached significance in comparison with the ɛ4 + placebo group, and approached significance for the remaining groups (mean change for ɛ4- regular insulin group = 0.86, SEM = 0.40; for ɛ4 + placebo = –0.56, SEM = 0.28, p < 0.01; for ɛ4- placebo mean = –0.08, SEM = 0.39, p = 0.10; for ɛ4 + regular insulin mean = –0.09, SEM = 0.27, p = 0.06; for ɛ4- detemir mean = –0.12, SEM = 0.32, p = 0.07; for ɛ4 + detemir mean = 0.01, SEM = 0.32, p = 0.11). It should be noted that the ɛ4 + participants in the placebo group had the greatest decline in memory at both months 2 and 4, and that ɛ4 + participants in both the regular insulin and detemir treated groups showed less decline; although these effects were not significant at month 4, it is possible with greater power or length of administration suchdifferences would reach significance, indicating benefit for ɛ4 + participants. No significant effects were observed in analyses with placebo and either regular insulin or detemir treated groups for the ADAS-Cog12, or for function as assessed by the DSRS (Supplementary Table 1).
MRI volumetrics
Increased or preserved volumes were noted in four ROIs for the regular insulin treated group compared with the placebo group: left superior parietal cortex, right middle cingulum, left cuneus, right parahippocampal gyrus (all ps < 0.05; Fig. 3A-D). Similar trends were noted in right cuneus and right superior frontal cortex (ps < 0.08). The results of comparisons between the detemir and placebo groups were more variable: decreased volume was observed for the detemir group relative to placebo for two ROIs (right cuneus and hippocampus, ps < 0.01 and 0.05, Fig. 3E, F), whereas preserved volume was observed for two ROIs (left anterior and middle cingulum, ps < 0.05, Fig. 3G, H).
Fig.3
Change from baseline volume (cm3) for key AD-related ROIs. Increased or preserved volumes were noted in four ROIs for the regular insulin-treated group compared with the placebo group: (A) left superior parietal cortex; (B) right middle cingulum; (C) left cuneus; (D) right parahippocampal gyrus (all ps < 0.05). Decreased volume was observed for the detemir group relative to placebo for (E) right cuneus and (F) right hippocampus (ps < 0.01 and 0.05), whereas preserved volume was observed for (G) left anterior cingulum and (H) left middle cingulum (ps < 0.05).
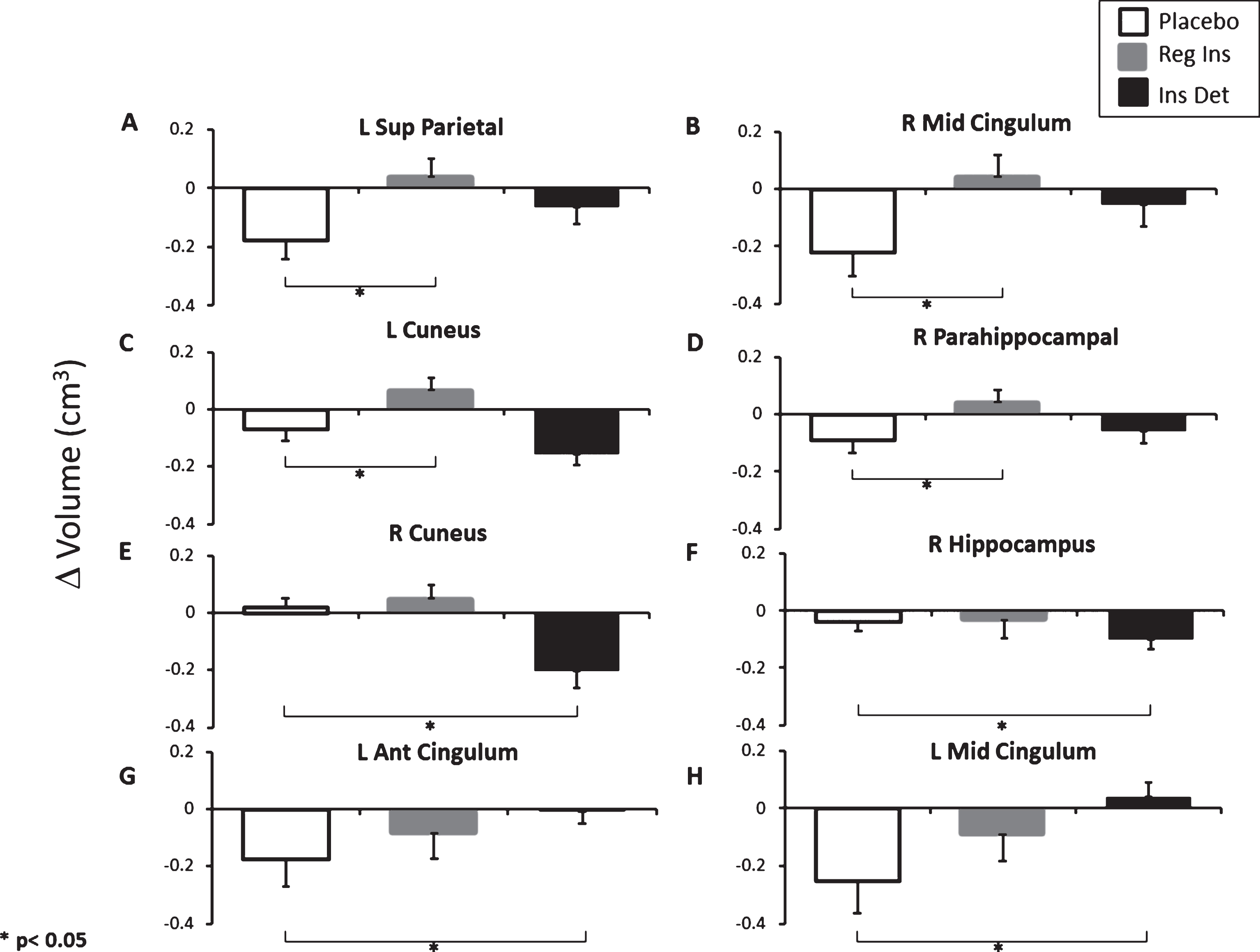
Relationships among MRI and cognitive outcomes
In regular insulin-treated participants, improved memory composite scores were associated with increased volume for several ROIs (left and right middle cingulum (rs = 0.60 and 0.62, ps < 0.05), left cuneus (r = 0.82, p < 0.01), hippocampus (r = 0.64, p < 0.04), right superior frontal (r = 0.67, p < 0.03), and parietal regions (r = 0.65, p < 0.04). In the placebo group, participants who had less volume loss in right superior frontal cortex and parahippocampal gyrus, and left inferior parietal cortex showed less memory decline (rs = 0.64, 0.72 and 0.65, ps < 0.05). No relationships between ROI volumes and memory were observed for the detemir treated group. Representative scatterplots are shown in Fig. 4.
Fig.4
Representative relationships between changes in MRI volume and cognitive outcomes. For regular insulin-treated participants, improved memory composite Z scores were associated with increased volume for (A) right middle cingulum (r = 0.62, p < 0.05), (B) left cuneus (r = 0.82, p < 0.01), (C) left inferior parietal (r = 0.61, p < 0.04) and (D) right superior frontal (r = 0.67, p < 0.03). For the placebo group, participants who had less volume loss in (C) left inferior parietal or (D) right superior frontal cortex showed less memory decline (rs = 0.65 and 0.64, ps < 0.05). No relationships between ROI volumes and memory were observed for the detemir-treated group.
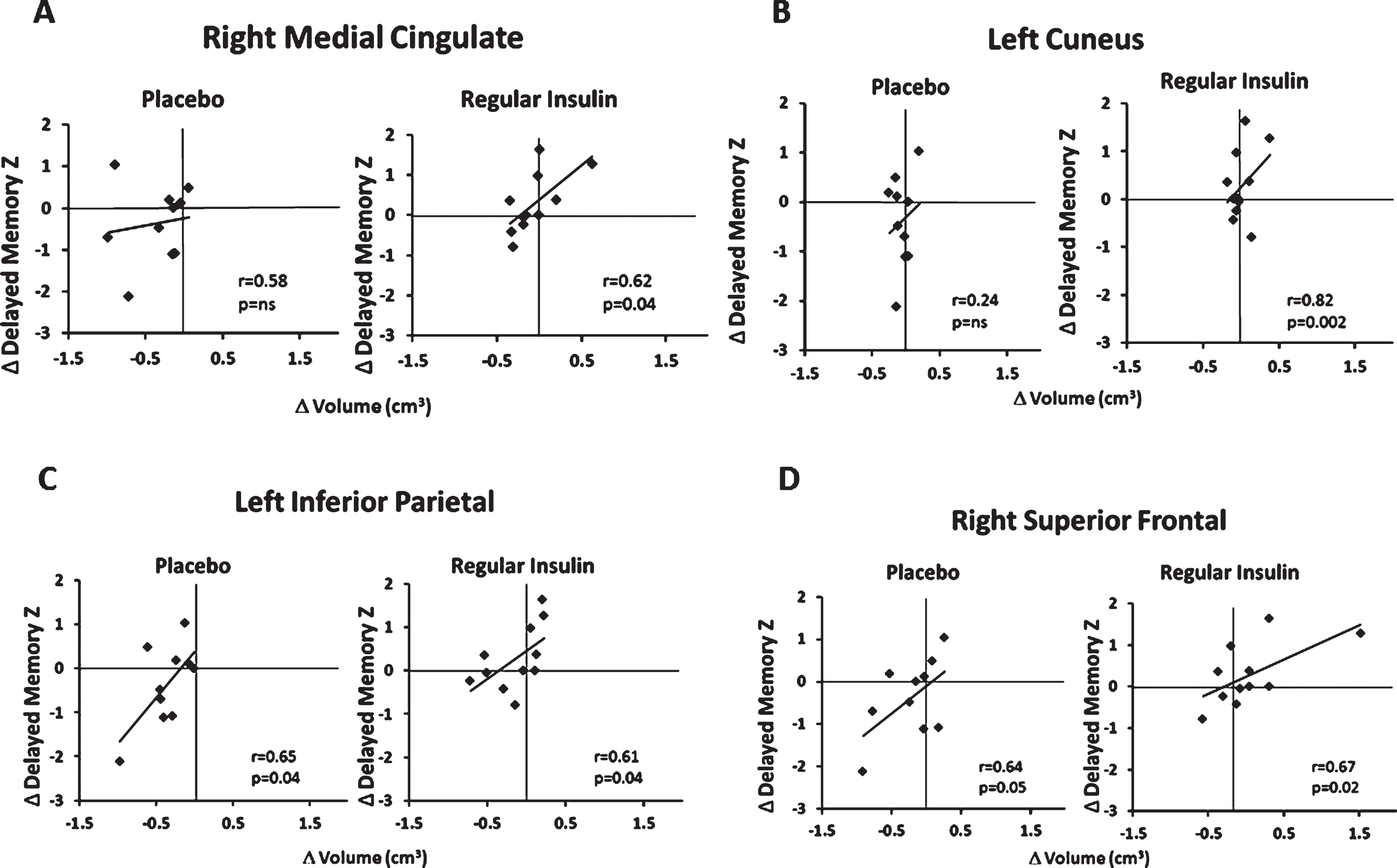
CSF biomarkers
The concentration of CSF Aβ42, tau, and tau-P181 did not change significantly with treatment with either regular insulin or detemir compared with placebo (Supplementary Table 1). The ratio of tau-P181 to CSF Aβ42, which has recently been identified as a sensitive marker of AD pathology [26], was lowered following regular insulin treatment relative to placebo (mean change from baseline with (SEM) = 0.02 (0.02) for placebo; –0.04 (0.02) for regular insulin, p < 0.01). No differences between placebo and detemir were observed. Although provocative, this finding must be considered exploratory given the small n.
Safety/compliance
In general, the safety profile was benign for both regular insulin and detemir, with rhinitis and upper respiratory symptoms the most common complaints (Supplementary Table 2). No Serious Adverse Events were deemed related to investigational product.
DISCUSSION
In previous work, we reported that treatment with regular insulin and with long-acting insulin detemir improved memory in adults with MCI and AD [9, 10]. The current study extended these findings by examining effects of regular insulin and detemir on structural MRI and CSF AD biomarkers, and by determining longer-term effects of detemir. Our results suggest that the efficacy of insulin detemir decreased over longer-term administration, whereas regular insulin continued to provide beneficial effects on memory. Further, regular insulin treatment was associated with preserved or increased volumes in several ROIs vulnerable to AD pathology, and with an improved CSF biomarker profile, raising the possibility that insulin modifies AD pathological processes as well as symptoms.
There are several potential mechanisms underlying the diminishing effects of detemir, although none have been definitively tested. It has been proposed that long-acting insulins may desensitize the insulin receptor or induce chronic hyperinsulinemia and thereby increase insulin resistance. Differential effects of detemir may also be due to its unique interactions with the insulin receptor, that activate different signaling pathways than regular insulin and may not invoke persistent cognitive benefit [27]. Insulin detemir is also more likely to self-associate due to its albumin-binding properties and may become less effective over time [28]. Further, the binding of detemir to albumin may interfere with reported interactions between Aβ and albumin [29], in a manner that counteracts longer-term benefits derived from its insulinogenic properties. Given that molecular weight may affect brain access following intranasal delivery [7], detemir’s self-association properties and/or its binding to albumin may also result in a larger particle that is not as readily transferred across epithelia or conveyed along perivascular channels. It is also possible that the dose of insulin detemir was not optimal; in a study of aged F344 rats, lower doses of detemir enhanced Morris water maze performance and corrected age-related hippocampal calcium dyshomeostasis as evidenced by reductions in the amplitude of CA2+ hyperpolarizing potentials in the CA1 field [30]. If long-acting insulin is less effective due to one or more of these mechanisms, then rapid acting forms of insulin that produce higher acute elevations, do not bind to albumin, and are more likely to remain monomeric and to be rapidly cleared, may provide greater therapeutic benefit. Consistent with this possibility, a study with normal adults reported greater memory benefit from rapid acting insulin compared with regular insulin [31].
The present study replicated previous studies showing that intranasally-administered regular insulin can improve memory for adults with MCI and AD [10]. Our results also showed for the first time that insulin-mediated memory benefits were accompanied by preserved or increased volume in brain regions affected by AD pathology, and that these changes were associated with memory improvement. In particular, we observed volume preservation in the cuneus, a region affected early by AD pathology, and for which preserved cerebral glucose metabolism had been observed in a previous study of four months treatment with intranasally-administered regular insulin [10]. Furthermore, regular insulin lowered the CSF tau-P181/Aβ42 ratio, which has been shown to be a sensitive indicator of AD risk and progression [26]. Taken together, these findings raise the possibility that insulin is modifying AD-related pathophysiologic processes, not merely improving symptoms of memory impairment.
Our results are consistent with previous studies demonstrating that insulin’s effects are modulated by APOE genotype [19]. In those studies, APOE ɛ4 carriers showed less memory improvement than did non-carriers when regular insulin was administered for 3-weeks or 4-months. A different profile was observed in a study of short-term detemir administration, in which detemir enhanced memory for APOE ɛ4 carriers who received treatment for three weeks [9]. Consistent with previous work, we observed that ɛ4- participants showed memory enhancement with regular insulin whereas regular insulin-treated ɛ4 + participants did not. It should be noted, however, that placebo-assigned ɛ4 + participants had the greatest decline in memory at both months 2 and 4, and ɛ4 + participants in both regular insulin and detemir groups showed less decline, although the effects were not significant. Lack of power clearly impairs our ability to address this issue definitively. An ongoing larger study testing 12 months of insulin treatment (clinicaltrials.gov NCT01767909) will provide greater power to examine the important question of APOE-related treatment response.
As noted, the most obvious limitation of the study is the small sample size. This pilot study was designed to provide evidence supporting a larger trial with detemir; given that the results showed significant effects for regular insulin and not detemir, and lack of persistent benefits from detemir for ɛ4 + participants, we were not able to provide support for further study with detemir. It remains possible, however, that different doses of detemir than were tested in the current study may provide therapeutic benefit. Another limitation includes the relatively brief length of administration. Clearly longer, larger trials with regular insulin are needed to address issues about disease modification, persistence of benefits, long-term safety, and responder characteristics such as APOE genotype.
In summary, the present study provides support for the continued investigation of intranasally-administered regular insulin, or new investigation of rapid acting insulins, as a therapy for MCI and AD. The findings that regular insulin preserved or enhanced brain volumes and lowered the tau-P181/Aβ42 ratio suggest that intranasal insulin may have disease-modifying effects on AD pathological processes, a possibility that is being pursued in an ongoing 12-month trial, and may be further elucidated in mechanistic studies.
ACKNOWLEDGMENTS
This work was supported by the Alzheimer’s Association Zenith Grant (ZEN-10-173646), by the Department of Veteran’s Affairs, and by the Roena B. Kulynych Center for Memory & Cognition Research. Intranasal insulin delivery devices were donated by Kurve Technology (Bothell, Washington).
Authors’ disclosures available online (http://j-alz.com/manuscript-disclosures/16-1256r2).
Appendices
The supplementary material is available in the electronic version of this article: http://dx.doi.org/10.3233/JAD-161256.
REFERENCES
[1] | Ribe EM , Lovestone S ((2016) ) Insulin signalling in Alzheimer’s disease and diabetes: From epidemiology to molecular links. J Intern Med 280: , 430–442. |
[2] | Gasparini L , Gouras GK , Wang R , Gross RS , Beal MF , Greengard P , Xu H ((2001) ) Stimulation of beta-amyloid precursor protein trafficking by insulin reduces intraneuronal beta-amyloid and requires mitogen-activated protein kinase signaling. J Neurosci 21: , 2561–2570. |
[3] | Lee CC , Kuo YM , Huang CC , Hsu KS ((2009) ) Insulin rescues amyloid beta-induced impairment of hippocampal long-term potentiation. Neurobiol Aging 30: , 377–387. |
[4] | De Felice FG , Vieira MN , Bomfim TR , Decker H , Velasco PT , Lambert MP , Viola KL , Zhao WQ , Ferreira ST , Klein WL ((2009) ) Protection of synapses against Alzheimer’s-linked toxins: Insulin signaling prevents the pathogenic binding of Abeta oligomers. Proc Natl Acad Sci U S A 106: , 1971–1976. |
[5] | Yarchoan M , Toledo JB , Lee EB , Arvanitakis Z , Kazi H , Han LY , Louneva N , Lee VM , Kim SF , Trojanowski JQ , Arnold SE ((2014) ) Abnormal serine phosphorylation of insulin receptor substrate 1 is associated with tau pathology in Alzheimer’s disease and tauopathies. Acta Neuropathol 128: , 679–689. |
[6] | Craft S , Peskind E , Schwartz MW , Schellenberg GD , Raskind M , Porte D Jr ((1998) ) Cerebrospinal fluid and plasma insulin levels in Alzheimer’s disease: Relationship to severity of dementia and apolipoprotein E genotype. Neurology 50: , 164–168. |
[7] | Lochhead JJ , Wolak DJ , Pizzo ME , Thorne RG ((2015) ) Rapid transport within cerebral perivascular spaces underlies widespread tracer distribution in the brain after intranasal administration. J Cereb Blood Flow Metab 35: , 371–381. |
[8] | Born J , Lange T , Kern W , McGregor GP , Bickel U , Fehm HL ((2002) ) Sniffing neuropeptides: A transnasal approach to the human brain. Nat Neurosci 5: , 514–516. |
[9] | Claxton A , Baker LD , Hanson A , Trittschuh EH , Cholerton B , Morgan A , Callaghan M , Arbuckle M , Behl C , Craft S ((2015) ) Long acting intranasal insulin detemir improves cognition for adults with mild cognitive impairment or early-stage Alzheimer’s disease dementia. J Alzheimers Dis 45: , 1269–1270. |
[10] | Craft S , Baker LD , Montine TJ , Minoshima S , Watson GS , Claxton A , Arbuckle M , Callaghan M , Tsai E , Plymate SR , Green PS , Leverenz J , Cross D , Gerton B ((2012) ) Intranasal insulin therapy for Alzheimer disease and amnestic mild cognitive impairment: A pilot clinical trial. Arch Neurol 69: , 29–38. |
[11] | Claxton A , Baker LD , Wilkinson CW , Trittschuh EH , Chapman D , Watson GS , Cholerton B , Plymate SR , Arbuckle M , Craft S ((2013) ) Sex and ApoE genotype differences in treatment response to two doses of intranasal insulin in adults with mild cognitive impairment or Alzheimer’s disease. J Alzheimers Dis 35: , 789–797. |
[12] | Kurtzhals P ((2004) ) Engineering predictability and protraction in a basal insulin analogue: The pharmacology of insulin detemir. Int J Obes Relat Metab Disord 28: (Suppl 2), S23–S28. |
[13] | De Leeuw I , Vague P , Selam JL , Skeie S , Lang H , Draeger E , Elte JW ((2005) ) Insulin detemir used in basal-bolus therapy in people with type 1 diabetes is associated with a lower risk of nocturnal hypoglycaemia and less weight gain over 12 months in comparison to NPH insulin. Diabetes Obes Metab 7: , 73–82. |
[14] | Tschritter O , Hennige AM , Preissl H , Porubska K , Schafer SA , Lutzenberger W , Machicao F , Birbaumer N , Fritsche A , Haring HU ((2007) ) Cerebrocortical beta activity in overweight humans responds to insulin detemir. PLoS One 2: , e1196. |
[15] | Roberts RO , Geda YE , Knopman DS , Cha RH , Pankratz VS , Boeve BF , Ivnik RJ , Tangalos EG , Petersen RC , Rocca WA ((2008) ) The Mayo Clinic Study of Aging: Design and sampling, participation, baseline measures and sample characteristics. Neuroepidemiology 30: , 58–69. |
[16] | McKhann G , Drachman D , Folstein M , Katzman R , Price D , Stadlan EM ((1984) ) Clinical diagnosis of Alzheimer’s disease: Report of the NINCDS-ADRDA Work Group under the auspices of Department of Health and Human Services Task Force on Alzheimer’s Disease. Neurology 34: , 939–944. |
[17] | Jiang Y , Li Y , Liu X ((2015) ) Intranasal delivery: Circumventing the iron curtain to treat neurological disorders. Expert Opin Drug Deliv 12: , 1717–1725. |
[18] | Kumar NN , Gautam M , Lochhead JJ , Wolak DJ , Ithapu V , Singh V , Thorne RG ((2016) ) Relative vascular permeability and vascularity across different regions of the rat nasal mucosa: Implications for nasal physiology and drug delivery. Sci Rep 6: , 31732. |
[19] | Reger MA , Watson GS , Green PS , Wilkinson CW , Baker LD , Cholerton B , Fishel MA , Plymate SR , Breitner JC , DeGroodt W , Mehta P , Craft S ((2008) ) Intranasal insulin improves cognition and modulates beta-amyloid in early AD. Neurology 70: , 440–448. |
[20] | Coen R , Kinsella A , Lambe R , Kenny M , Darragh A ((1990) ) Creating equivalent word lists for the Buschke Selective Reminding Test. Hum Psychopharmacol 5: , 47–51. |
[21] | Rosen WG , Mohs RC , Davis KL ((1984) ) A new rating scale for Alzheimer’s disease. Am J Psychiatry 141: , 1356–1364. |
[22] | Clark CM , Ewbank DC ((1996) ) Performance of the dementia severity rating scale: A caregiver questionnaire for rating severity in Alzheimer disease. Alzheimer Dis Assoc Disord 10: , 31–39. |
[23] | Maldjian JA , Baer AH , Kraft RA , Laurienti PJ , Burdette JH ((2009) ) Fully automated processing of fMRI data in SPM: From MRI scanner to PACS. Neuroinformatics 7: , 57–72. |
[24] | Ashburner J , Friston KJ ((2000) ) Voxel-based morphometry–the methods. Neuroimage 11: , 805–821. |
[25] | Wang WY , Yu JT , Liu Y , Yin RH , Wang HF , Wang J , Tan L , Radua J , Tan L ((2015) ) Voxel-based meta-analysis of grey matter changes in Alzheimer’s disease. Transl Neurodegener 4: , 6. |
[26] | Harari O , Cruchaga C , Kauwe JS , Ainscough BJ , Bales K , Pickering EH , Bertelsen S , Fagan AM , Holtzman DM , Morris JC , Goate AM , Alzheimer’s Disease Neuroimaging Initiative ((2014) ) Phosphorylated tau-Abeta42 ratio as a continuous trait for biomarker discovery for early-stage Alzheimer’s disease in multiplex immunoassay panels of cerebrospinal fluid. Biol Psychiatry 75: , 723–731. |
[27] | Sciacca L , Cassarino MF , Genua M , Pandini G , Le Moli R , Squatrito S , Vigneri R ((2010) ) Insulin analogues differently activate insulin receptor isoforms and post-receptor signalling. Diabetologia 53: , 1743–1753. |
[28] | Kurtzhals P ((2007) ) Pharmacology of insulin detemir. Endocrinol Metab Clin North Am 36: (Suppl 1), 14–20. |
[29] | Stanyon HF , Viles JH ((2012) ) Human serum albumin can regulate amyloid-beta peptide fiber growth in the brain interstitium: Implications for Alzheimer disease. J Biol Chem 287: , 28163–28168. |
[30] | Maimaiti S , Anderson KL , DeMoll C , Brewer LD , Rauh BA , Gant JC , Blalock EM , Porter NM , Thibault O ((2016) ) Intranasal insulin improves age-related cognitive deficits and reverses electrophysiological correlates of brain aging. J Gerontol A Biol Sci Med Sci 71: , 30–39. |
[31] | Benedict C , Hallschmid M , Schmitz K , Schultes B , Ratter F , Fehm HL , Born J , Kern W ((2007) ) Intranasal insulin improves memory in humans: Superiority of insulin aspart. Neuropsychopharmacology 32: , 239–243. |