Lipid Peroxidation Markers in Coronary Artery Disease Patients with Possible Vascular Mild Cognitive Impairment
Abstract
This study examined associations between lipid peroxidation markers and cognition, and associations between these markers and cognitive response to an exercise intervention program, in adults with coronary artery disease at risk of dementia. Lipid peroxidation products were measured in serum in 118 patients (29 possible vascular mild cognitive impairment and 89 controls). Ratios of early- (lipid hydroperoxides, LPH) to late-stage (8-isoprostane, 8-ISO; 4-hydroxy-2-nonenal, 4-HNE) lipid peroxidation products were calculated. Cognitive performance was assessed before and at completion of a 24-week exercise intervention program. A global effect of group on lipid peroxidation markers was observed, adjusting for sex, years of education, and cardiopulmonary fitness (main effect of group F (3,102) = 2.957, p = 0.036). Lower lipid peroxidation at baseline, as determined by lower 8-ISO concentration, was associated with greater improvement in verbal memory (F (1, 64) = 4.738, p = 0.03) and executive function (F (1, 64) = 5.219, p = 0.026) performance. Similarly, higher ratios of 8-ISO/LPH (F (1, 65) = 6.592, p = 0.013) and (8-ISO+4-HNE) to LPH (F (1, 65) = 3.857, p = 0.054), were associated with less improvement in executive function performance over a 24-week exercise intervention. Lipid peroxidation may be a biomarker of early vascular cognitive impairment, and elevated lipid peroxidation might limit the cognitive benefits of exercise in this high-risk population.
INTRODUCTION
Cerebrovascular disease is the second most common cause of cognitive impairment in older adults [1, 2]. Vascular mild cognitive impairment (VaMCI) is highly prevalent among patients with coronary artery disease (CAD), and those with VaMCI are at higher risk of cognitive and functional decline leading to dementia [3–6]. VaMCI describes mild cognitive deficits due to cerebrovascular disease that are not severe enough to be classified as dementia [7]. Predominant executive dysfunction has long been considered a salient feature of the disease, although deficits in other cognitive domains such as verbal and visuospatial memory as well as processing speed are also commonly present [8–10].
Oxidative stress (OS) plays a central role in age-related neurodegeneration [11]. Reactive oxygen species (ROS) cause cellular damage and mitochondrial dysfunction and alter the DNA repair system, all of which are key factors involved in the propagation of neuronal injury leading to apoptosis [12]. An imbalance in the production and elimination of ROS is associated with neurodegenerative diseases. Cumulative ROS can react with neuronal membrane lipids to generate lipid hydroperoxides (LPH), which under normal physiological conditions, are detoxified by the reparative actions of exogenous and endogenous antioxidants [13]. As the lipid peroxidation cascade continues, LPH, the initial product, reacts with another fatty acid resulting in the formation of more stable late-stage products including 4-hydroxy-2-nonenal (4-HNE) and 8-isoprostane (8-ISO) [13]. To prevent the accumulation of these late-stage products, a natural cellular response is to increase antioxidant activity or accelerate the elimination rate of lipid peroxidation products [14, 15].
Emerging evidence suggests that an altered antioxidant defense system and increased lipid peroxidation products might represent an early event in neurodegenerative processes. A recent meta-analysis found that several lipid peroxidation markers were significantly elevated while a range of antioxidant molecules were reduced in MCI patients [16, 17]. OS damage may contribute to cognitive deficits in CAD patients, as cerebral hypoperfusion can cause ischemia- and inflammation-related increases in OS [18].
Regular exercise has beneficial systemic effects including the upregulation of the enzymatic antioxidant system and modulation of oxidative damage [19–21]. As such, regular exercise could play an important therapeutic role in the prevention of cognitive decline in the prodromal stages of neurodegenerative diseases [22]. However, the cognitive benefits of exercise are variable and particularly modest among patients with mild cognitive symptoms [23–25]. Since OS damage occurs early in neurodegenerative disease processes and might precede other neuropathological alterations, there is considerable benefit to identifying an easily obtainable biological marker that could predict treatment response as well as to identify patients at the prodromal stage who are more likely to develop clinical and functional decline.
The present study aims to evaluate whether elevated levels of lipid peroxidation products might be an early stage marker of VaMCI. We compared concentrations of lipid peroxidation products in CAD patients without cognitive impairment and those with possible VaMCI [7]. We also sought to investigate whether higher lipid peroxidation products at baseline might predict cognitive response to exercise intervention. Our primary hypothesis was that lipid peroxidation markers would be elevated in patients with possible VaMCI relative to CAD controls. We also hypothesized that higher level of lipid peroxidation at baseline would be associated with less improvement in cognitive performance, particularly in executive function, following a 24-week exercise intervention.
MATERIALS AND METHODS
Design
The Research Ethics Boards at Sunnybrook Health Sciences Centre and the University Health Network (UHN) approved this study, and informed consent was obtained from all participants prior to study enrolment. CAD patients were recruited from a cardiac rehabilitation (CR) program at the UHN Toronto Rehabilitation Institute (TRI) as described previously [26–28]. Briefly, the CR program is a 24-week program comprised of both aerobic and resistance exercise under the supervision of exercise and medical specialists. As part of the CR program, participants attended weekly exercise classes that included an aerobic walk or walk/jog, and exercised 3–6 days per week at home depending on their fitness level. At baseline (pre-exercise), lipid peroxidation assays were performed and cardiopulmonary fitness was assessed by measuring the peak volume of oxygen uptake (VO2 peak) during an exercise stress test. Cognitive assessment was performed at baseline and at 24 weeks (follow-up).
Participants
Eligible patients were 50–75 years old, could speak and understand English, and had evidence of stable CAD (previous hospitalization for acute myocardial infarction, coronary angiographic evidence of ≥50% blockage in one or more major coronary artery, percutaneous coronary intervention, or coronary artery bypass grafting). All subjects had dyslipidemia and were being treated with statins. Subjects were excluded based on previously diagnosed neurodegenerative illness including all-cause dementia, active cancer, surgery planned within 12 months, schizophrenia, bipolar affective disorder, and substance abuse. The Standardized Mini Mental Status Examination (sMMSE) [29] was used to screen for dementia and subjects with sMMSE <24 were excluded.
Demographic and clinical characteristics, as well as detailed medical history including comorbidities independent of CAD, were collected from patient interviews. Cardiac medical history, concomitant medications, and cardiac health indicators (body mass, height, hyperlipidemia, hypertension, diabetes, and waist circumference) were obtained from patient charts at TRI. Body mass index (BMI) was calculated per standard definition [mass (kg) / height (m2)].
VaMCI was defined as patients whose baseline composite Z-scores on either verbal memory or executive function tests were 1 standard deviation (SD) below population norm. CAD controls demonstrated normal cognitive function (i.e., composite Z-scores >– 1 on both memory and executive function tests) [7].
Cognitive assessments
A battery of cognitive tests was used to measure executive function, processing speed, and memory [30]. Executive function was assessed using the Trail Making Test B (Trails B), Controlled Oral Word Association (verbal fluency FAS test), and category fluency Animal Naming. Verbal memory was assessed using the California Verbal Learning Test 2nd Ed. (CVLT-II). Visuospatial function was assessed using the Revised Brief Visuospatial Memory Test (BVMT-R). Processing speed was assessed using the Digit Symbol Coding task and the Trail Making Test A (Trails A). For each cognitive task, a Z-score was computed based on published age- and sex-matched norms. The Z-score follows a normal distribution with better performance being more positive and poorer performance being more negative distribution. For executive function, the Z-scores of the Trails B, FAS test, and Animal Naming test were summed. For verbal memory, three Z-scores from the CVLT-II, verbal learning, short delay, and long delay free recall were summed. For visuospatial function, two Z-scores from the BVMT-R, visuospatial learning and recall were summed. For processing speed, the Trails A and Digit Symbol Coding task were summed.
Lipid peroxidation markers
Serum concentrations of lipid peroxidation markers (LPH, 8-ISO, 4-HNE) were measured as previously described [31, 32]. Briefly, LPH was assessed using the colorimetric LPH assay kit (Cayman Chemical; item 705002) according to manufacturer’s instructions. The sensitivity of this kit is in between 0.25 and 5 nmol hydroperoxides. The intra-assay coefficients of variation (CV) were between 2% and 15% and the inter-assay CV was 9%. Data are presented as the LPH (μM) and log-transformed for analysis. 4-HNE was measured via Michael addition to lysine, histidine or cysteine using an enzyme-linked immunosorbent assay (ELISA) (Cell Biolabs, Inc.; item number STA-338) according to manufacturer’s instructions. The sensitivity of this assay is in between 0.5 and 10μg/mL of 4-HNE. The intra-assay CVs were between 1% and 16% and the inter-assay CV was 7.5%. Results were expressed as fmol/μg of protein and log-transformed for analysis. 8-ISO was measured using a standard competitive sandwich ELISA (Cayman Chemical; item 516351) according to manufacturer’s instructions. The sensitivity of this assay is in between 0.8 and 500 pg/mL of 8-ISO. The intra-assay CVs were between 1% and 19% and the inter-assay CV was 9.1%. Results were expressed as pg/mL and log-transformed for analysis.
Statistical analyses
Sample mean and SD for continuous demographic and baseline clinical characteristics were calculated. Categorical variables were described with frequencies and percentages. Differences in demographic and clinical variables between the groups were assessed using independent t-tests.
For the primary analysis, a Multivariate Analysis of Variance (MANOVA) model adjusting for sex, years of education, BMI, and VO2 peak were used to examine the overall difference in lipid peroxidation marker levels between the groups. The early- (LPH) and late-stage (8-ISO and 4-HNE) lipid peroxidation markers were inputted as the dependent variables. The main effect of group was considered significant at the conventional p≤0.05. Post-hoc univariate ANOVA were conducted to evaluate differences between the groups for each individual marker.
Ratios of late-stage to early-stage lipid peroxidation were used previously to measure the balance of the system and explore if there is an accumulation of late-stage products [32]. In the secondary analyses, the ratios of late- to early-stage markers were calculated by dividing concentrations of 8-ISO and 4-HNE by the concentration of LPH, yielding 8-ISO/LPH, 4-HNE/LPH and (8-ISO+4-HNE)/LPH [31, 32]. The individual concentrations and ratios were log-transformed to ensure consistent normality between them, and the resulting values were used for analyses. Univariate ANCOVA were performed to investigate the effect of group on lipid peroxidation using ratios between late stage markers 4-HNE and 8-ISO and the early-stage marker LPH (i.e., 8-ISO/LPH, 4-HNE/LPH, (8-ISO+4-HNE)/LPH) as dependent variables and group as fixed factor. Consistent with the primary analysis, sex, years of education, and VO2 peak were also included as covariates.
The baseline individual concentrations of 8-ISO, 4-HNE, LPH, and ratios of 8-ISO/LPH, 4-HNE/LPH, and (8-ISO+4-HNE)/LPH were assessed as predictors of cognitive performance change following 24 weeks of exercise intervention. The repeated measures general linear models were performed using the cognitive Z-scores at baseline and at 24-weeks as repeated variables, with diagnosis group added as a fixed factor. Analyses were performed separately for each lipid peroxidation marker and ratio. Possible confounding variables such as sex, years of education, BMI, and VO2 peak were added as covariates. Linear regression analyses to examine associations between lipid peroxidation markers and changes in cognition over 24 weeks were also investigated.
For post-hoc analyses, we divided the CAD sample into high and low OS group based on the overall ratio of (8-ISO+4-HNE)/LPH concentrations. Differences in verbal memory and executive function Z-scores between the low and high OS groups were evaluated using univariate ANCOVA, adjusting for possible confounding variables, baseline cognitive Z-scores, and years of education as covariates. All analyses were performed using SPSS statistical software and considered to be significant at a two-tailed p≤0.05.
RESULTS
Clinical characteristics
Participant demographics and clinical characteristics are presented in Table 1. There were no significant differences between subjects with VaMCI and CAD controls with respect to sex, BMI, plasma cholesterol level, triglycerides level, diabetes, or smoking habit. However, the VaMCI group had significantly lower cardiopulmonary fitness (F (1, 114) = 4.845, p = 0.035) and total years of education (F (1, 114) = 17.094, p < 0.001). As expected, patients with VaMCI had significantly poorer cognitive performance across all cognitive domains, including verbal and visuospatial memory, executive function, and processing speed (Table 1).
Table 1
Baseline patient characteristics
CAD controls | VaMCI | Sig | |||||
(n = 89) | (n = 29) | ||||||
Demographics | Mean | SD | Mean | SD | F / x2 | df | p |
Male (%) | 83.1 | 89.7 | 0.717 | 1 | 0.397 | ||
Age | 63.7 | 6.2 | 63.2 | 7.0 | 0.165 | 1 | 0.685 |
BMI | 29.1 | 5.3 | 29.3 | 4.7 | 0.005 | 1 | 0.886 |
Total years of education | 17.0 | 3.1 | 14.1 | 3.7 | 17.094 | 1 | <0.001 |
Cholesterol | 3.5 | 0.8 | 3.5 | 0.9 | 0.003 | 1 | 0.991 |
Triglycerides | 1.3 | 0.8 | 1.3 | 0.7 | 0.006 | 1 | 0.889 |
VO2 peak | 21.6 | 5.6 | 19.0 | 5.3 | 4.854 | 1 | 0.035 |
Vascular Risk Factors (%) | |||||||
Smoking history | 59.6 | 62.1 | 0.058 | 1 | 0.81 | ||
Hypertension | 59.1 | 69.0 | 0.899 | 1 | 0.343 | ||
Hyperlipidemia | 100 | 100 | |||||
Diabetes | 16.9 | 17.2 | 0.002 | 1 | 0.961 | ||
BMI >30 kg/m2 | 33.7 | 41.4 | 0.562 | 1 | 0.454 | ||
Waist circumference* | 40.4 | 41.4 | 0.008 | 1 | 0.929 | ||
Total # of vascular risk factors | 3.1 | 1.4 | 3.3 | 1.3 | 0.444 | 1 | 0.501 |
*Waist circumference >102 cm for male and >88 cm for female, denoting obesity. | |||||||
Cognitive Performance | |||||||
Verbal Memory | 3.07 | 2.94 | 0.54 | 2.84 | 15.635 | 3 | <0.001 |
Visuospatial Function | 0.89 | 1.86 | –1.22 | 2.4 | 25.197 | 3 | <0.001 |
Processing Speed | 0.49 | 1.27 | –1.23 | 1.17 | 40.213 | 3 | <0.001 |
Executive Function | 0.66 | 1.61 | –2.66 | 1.38 | 96.283 | 3 | <0.001 |
Lipid peroxidation markers in CAD patients with VaMCI and CAD controls
In our primary analyses, a global effect of group on lipid peroxidation markers was observed when adjusting for age and sex (main effect of group F (3,102) = 2.957, p = 0.036, Table 2). The post-hoc Univariate ANCOVA revealed that serum LPH concentrations were significantly lower in patients with VaMCI compared to CAD controls (1.21±0.61 versus 1.51±0.41 (F (1, 104) = 8.913, p = 0.004) (Fig. 1). There were no significant differences between the groups in 8-ISO (F (1,111) = 0.698, p = 0.405) and 4-HNE concentrations (F (1,101) = 0.001, p = 0.975).
Fig.1
Box plot displaying the distribution of the data based on median and interquartile range showing differences in lipid peroxidation markers (A) LPH; (B) 8-ISO/LPH; (C) 4-HNE/LPH); (D) (8-ISO+4-HNE)/LPH between CAD patients without cognitive impairment (CAD controls; n = 89) and those with cognitive impairment (VaMCI group; n = 29). The LPH concentration was significantly lower in VaMCI group as compared of CAD controls, but the ratio between late to early stage lipid peroxidation markers were significantly greater in the VaMCI group as compared to CAD controls. Values are adjusted group mean±SD.
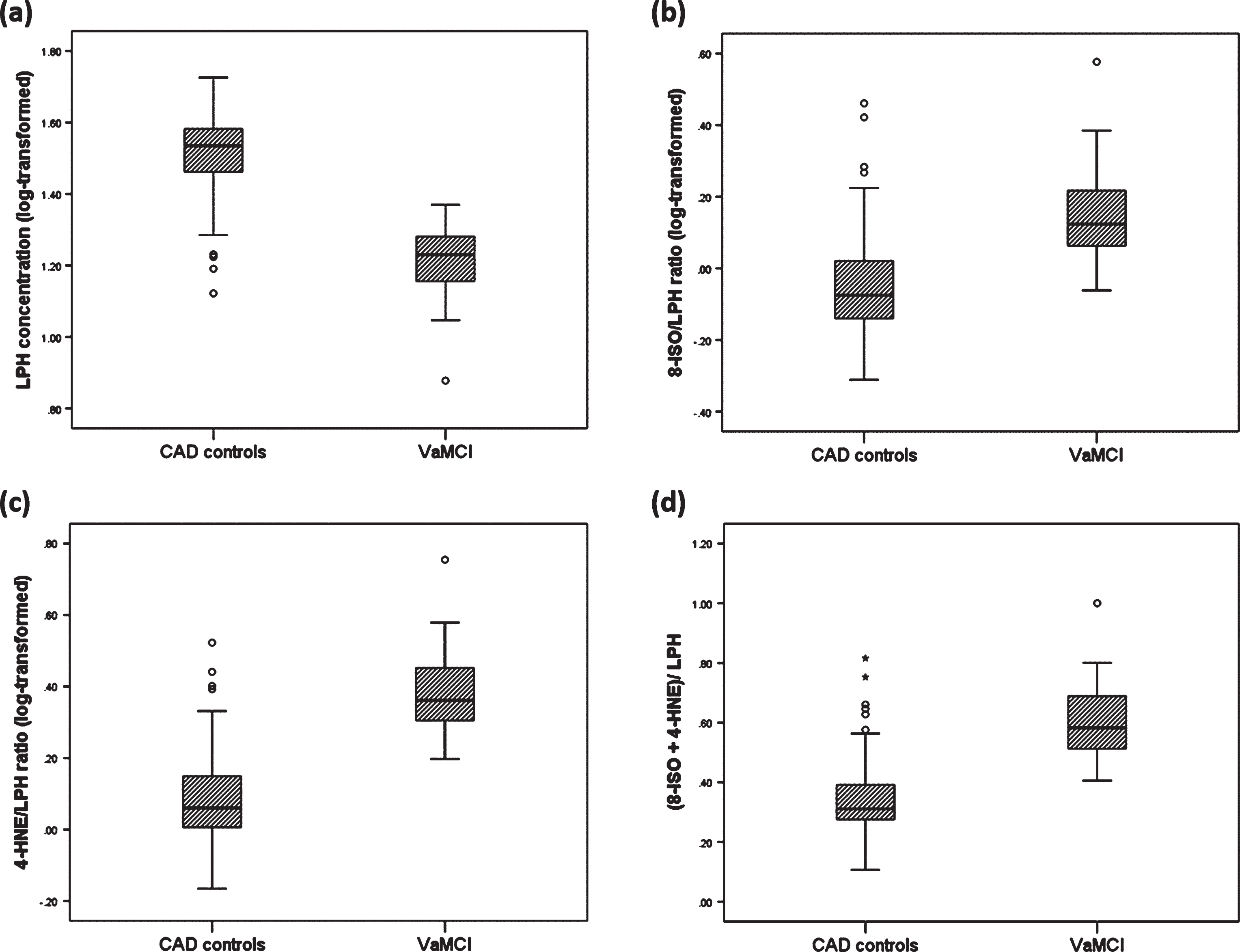
Table 2
Lipid peroxidation markers in CAD patients without cognitive impairment (CAD controls) and those with cognitive impairment (VaMCI group). Sex, VO2 peak, BMI, and years of education added as covariates
CAD controls | VaMCI | Sig | |||||
(n = 89) | (n = 29) | ||||||
Raw data | Mean | SD | Mean | SD | F/ x2 | df | p |
LPH (μM) | 44.9 | 31.4 | 31.1 | 28.1 | 3.983 | 5 | 0.049 |
8-ISO (pg/mL) | 35 | 23.4 | 27.1 | 14.1 | 1.716 | 5 | 0.193 |
4-HNE (fmol/μg) | 40.7 | 9.3 | 40.0 | 7.3 | 0.054 | 5 | 0.817 |
8-ISO/LPH | 1.9 | 5.5 | 5.1 | 10.0 | 5.965 | 5 | 0.016 |
4-HNE/LPH | 2.4 | 5.5 | 7.3 | 14.8 | 8.278 | 5 | 0.005 |
(8-ISO+4-HNE)/LPH | 0.5 | 0.1 | 0.4 | 0.1 | 6.629 | 5 | 0.011 |
Log-transformed data | |||||||
LPH (μM) | 1.5 | 0.4 | 1.2 | 0.6 | 8.913 | 5 | 0.004 |
8-ISO (pg/mL) | 1.4 | 0.3 | 1.4 | 0.3 | 0.698 | 5 | 0.405 |
4-HNE (fmol/μg) | 1.6 | 0.1 | 1.6 | 0.1 | 0.001 | 5 | 0.975 |
8-ISO/LPH | –0.1 | 0.5 | 0.2 | 0.7 | 4.145 | 5 | 0.004 |
4-HNE/LPH | 0.1 | 0.4 | 0.4 | 0.6 | 8.599 | 5 | 0.004 |
(8-ISO+4-HNE)/LPH | 0.3 | 0.4 | 0.6 | 0.6 | 7.022 | 5 | 0.009 |
In our secondary analyses, we found that there were significant differences between the groups in the ratios between late- and early- stage lipid peroxidation products. Patients with VaMCI showed a trend toward higher ratio of 8-ISO to LPH compared to that of CAD controls (0.15±0.66 versus – 0.05±0.45 (F (1, 104) = 4.145, p = 0.044) (Fig. 1). Similarly, the ratios of 4-HNE to LPH and (8-ISO+4-HNE) to LPH were significantly higher in the VaMCI group as compared to CAD controls (4-HNE to LPH: 0.39±0.61 versus 0.08±0.43 (F (1, 104) = 8.599, p = 0.004; (8-ISO+4-HNE) to LPH: 0.60±0.62 versus 0.34±0.42 (F (1, 104) = 7.022, p = 0.009) (Fig. 1).
Higher baseline lipid peroxidation levels and exercise effect on cognition
In all CAD patients, there were significant improvements over the 24-week exercise intervention in verbal memory (t (1, 69) = –7.765, p < 0.001), processing speed (t (1, 70) = –4.452, p < 0.001), and executive function performance (t (1, 70) = –4.525, p < 0.001), but no difference between baseline and follow-up in visuospatial function performance (t (1, 70) = 1.231, p = 0.223). The differences were no longer significant when we adjusted for possible covariates. Repeated measures ANOVA of cognitive performance at baseline and 6 months adjusting for age, gender, education years, and VO2 peak revealed no significant time and time-by-group interactions in any of the cognitive domains (all p values >0.05).
Linear regression analyses revealed a significant association between baseline serum concentration of 8-ISO and change in verbal memory (R2 = 0.124, p = 0.033; Fig. 2A) and executive function z-scores (R2 = 0.137, p = 0.026; Fig. 2B) in a model that included sex, years of education, VO2 peak, and BMI as possible covariates. Similarly, in separate analyses, there were significant associations between smaller improvement in executive function performance over 24 weeks and the 8-ISO/LPH (R2 = 0.154, p = 0.013; Fig. 2C), and only a trend toward significant with (8-ISO+4-HNE)/LPH ratios (p = 0.054). The results remained significant when patient group (VaMCI or CAD control) was added as a covariate. None of the other lipid peroxidation markers or ratios were found to be significant predictors of change in processing speed or visuospatial function.
Fig.2
Scatterplot illustrating statistically significant associations between (A) 8-ISO concentration and change in verbal memory performance (R2 = 0.124, p = 0.033) following 24 weeks of exercise intervention and; (B) significant associations between executive function and 8-ISO (R2 = 0.137, p = 0.026), (C) 8-ISO/LPH (R2 = 0.154, p = 0.013) when adjusting for sex, BMI, years of education, and VO2 peak. Y-axis represents adjusted predicted value based on applied linear regression model.
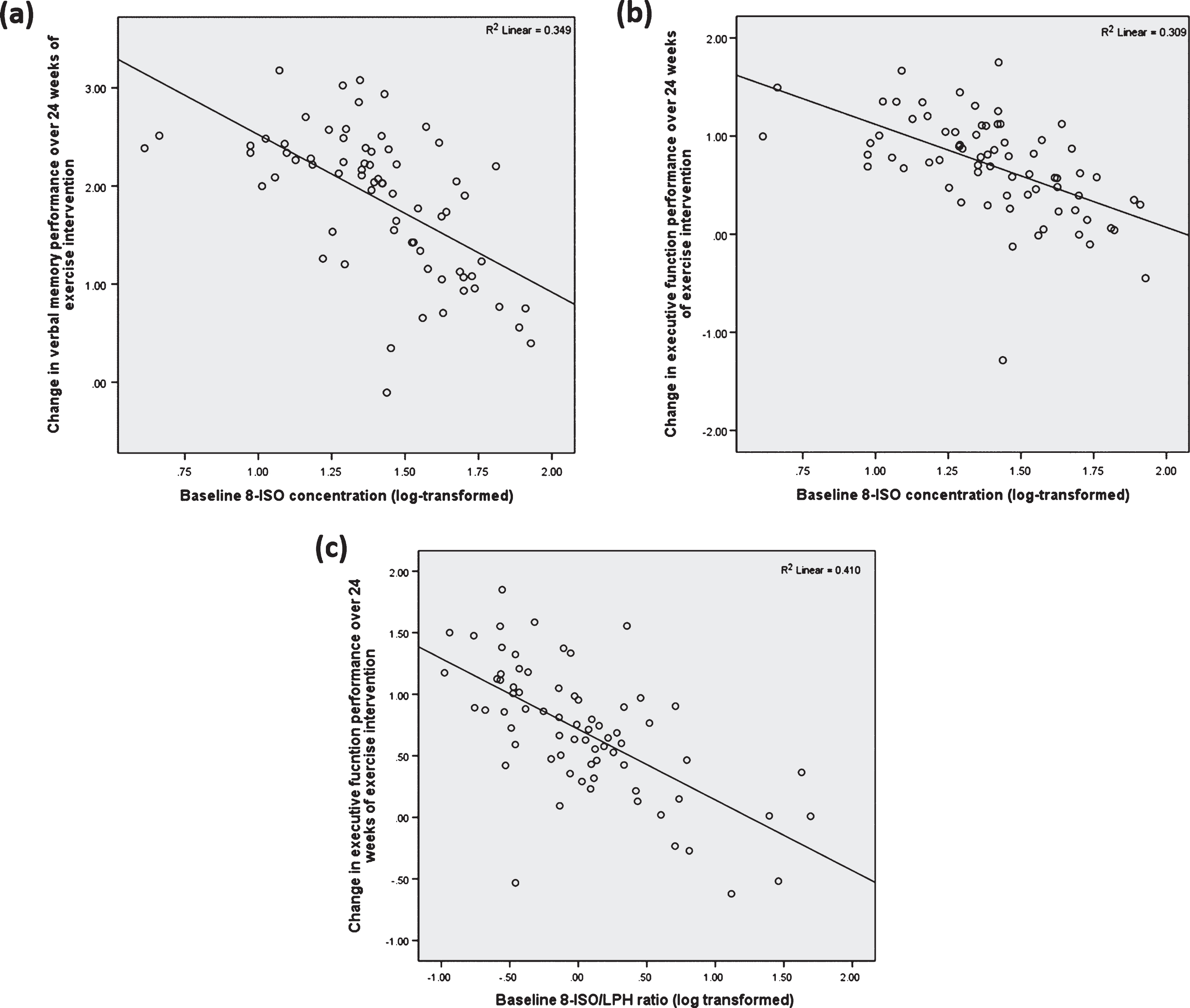
To confirm our results, we also did post-hoc analyses using repeated measure ANCOVA, where we found significant time by 8-ISO effect on verbal memory (F (1, 64) = 4.738, p = 0.030) and executive function (F (1, 64) = 5.219, p = 0.026) at baseline and follow up. Similarly, there was a significant time by 8-ISO/LPH (F (1, 65) = 6.592, p = 0.013) interaction effect and a trending (8-ISO+4HNE)/LPH (F (1, 65) = 3.857, p = 0.054) interaction effect on executive function performance at baseline and follow-up. A univariate analysis to compare the effect of exercise on cognition between CAD patients who had high and low lipid peroxidation levels at baseline indicated that those in the low lipid peroxidation group had significantly greater improvement in executive function, after controlling for baseline cognitive score, sex, BMI, VO2 peak, and years of education (high versus low for (8-ISO+4-HNE)/LPH: (F (1,64) = 6.690, p = 0.012); high versus low 8-ISO to LPH ratio: (F (1, 64) = 5.965, p = 0.017); Fig. 3).
Fig.3
Lipid peroxidation ratios might predict the effect of exercise on cognition. Box plot displaying the distribution of the data based on median and interquartile range showing that CAD patients who had lower OS levels at baseline, as determined by overall ratio of (A) (8-ISO+4-HNE) to LPH (F (1,64) = 6.690, p = 0.012) and (B) 8-ISO to LPH (F (1, 64) = 5.965, p = 0.017), demonstrated greater improvement in executive function performance following 24 weeks of exercise intervention in a model that included baseline cognitive score, VO2 peak, BMI, sex and years of education. Values are presented as adjusted group mean±SD.
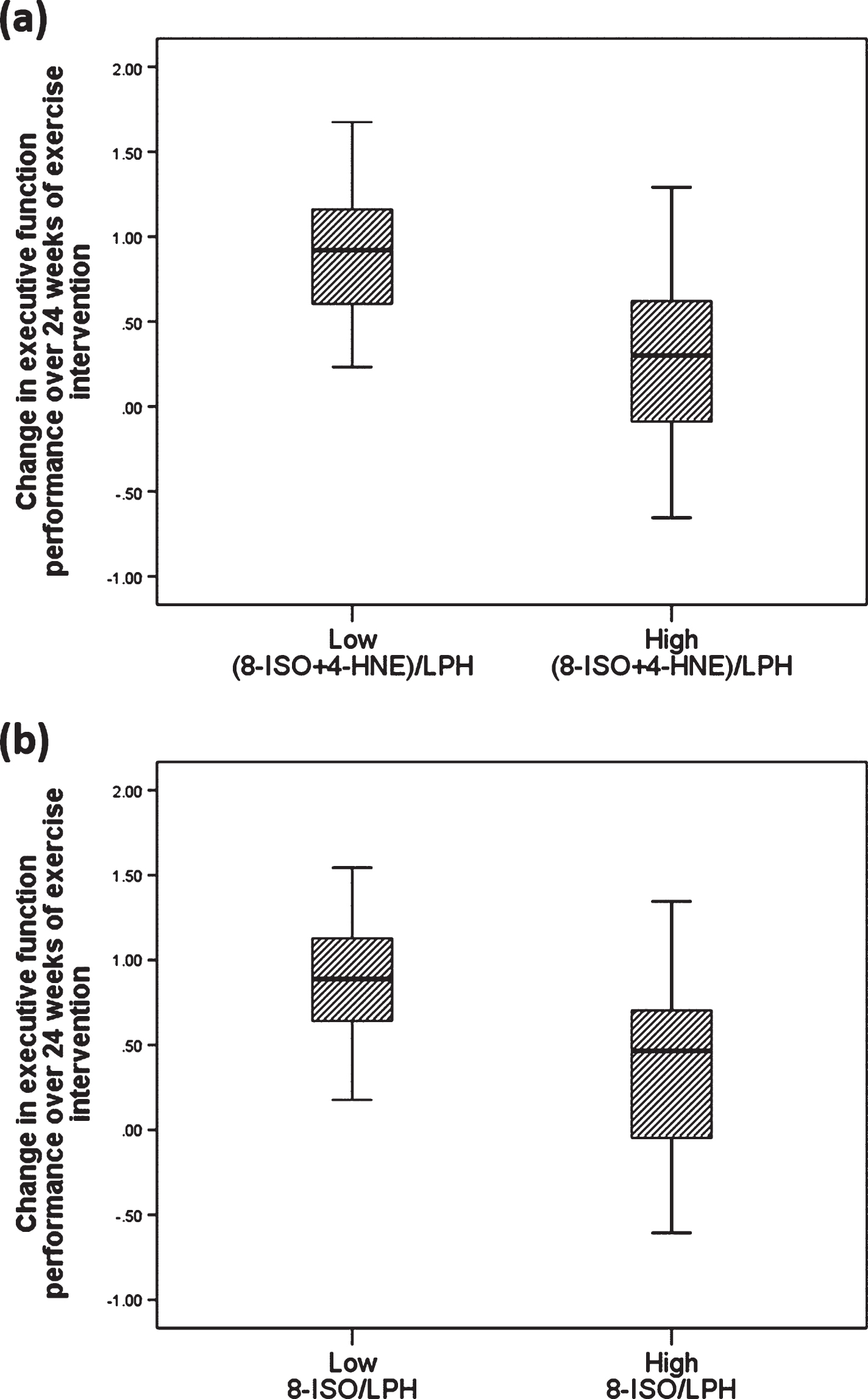
DISCUSSION
The present study highlights the possible contribution of lipid peroxidation in the etiology of early cognitive deficits due to vascular pathology. We found a significant group effect (VaMCI versus CAD control) in a model that includes all three lipid peroxidation products as the dependent variables, as well as sex, cardiopulmonary fitness, and years of education as potential confounding variables. The ratios between late- and early-stage lipid peroxidation markers (8-ISO/LPH, 4-HNE/LPH, and (8-ISO+4-HNE)/LPH) were significantly elevated in the VaMCI group as compared to CAD controls. The present study also sought to explore the influence of lipid peroxidation levels at baseline in mediating the benefit of exercise intervention on cognition. We found that higher lipid peroxidation levels at baseline, as indicated by greater 8-ISO and ratios between late- and early-stage markers, were associated with less improvement in executive function and verbal memory performance following a 24-week exercise intervention. OS has been proposed to represent an early event in the neurodegenerative process. Our results are consistent with a growing body of evidence, which has found that lipid peroxidation markers are significantly elevated in the blood, cerebrospinal fluid, and brain of patients with MCI [33, 34]. Ratios of lipid peroxidation markers, including those comparing late-stage markers 8-ISO, 4-HNE, and (8-ISO+4-HNE) to the early-stage marker LPH might be used to measure the balance of the redox system [31, 32]. The VaMCI cohort in the present study represents a subgroup of individuals who have not yet developed dementia and are independent in activities of daily living. Together with their subtle cognitive impairment, they have important vascular risk factors, as shown in Table 1. Despite the important contribution of vascular risk factors in neurodegenerative diseases, there is currently no reliable biomarker that defines MCI due to vascular disease. Our results suggest that accelerated lipid peroxidation progression might contribute to early cognitive changes due to vascular disease and may represent a risk biomarker for those with VaMCI. The Canadian Study of Health and Aging has reported that the failure to consider VaMCI underestimates the prevalence of impairment and the risk for adverse outcomes associated with vascular cognitive impairment, as the rates of institutionalization and mortality of those with cognitive impairment were significantly higher than those without cognitive impairment [35]. Our findings may provide an important lead into the search for a therapeutic target that can be used to treat this high-risk subpopulation at the earliest stage of the disease process. The next step would be to perform a longer longitudinal study to evaluate lipid peroxidation markers in a cohort of VaMCI patients who have evidence of both cognitive impairment and subcortical ischemic vascular disease on MRI [7, 36]. While the cardiovascular benefits of physical exercise in CAD patients are well-established [37, 38], less attention has been paid to maximizing cognitive benefits. The present study investigated lipid peroxidation markers as predictors of cognitive response to exercise in CAD patients.
In addition to lipid peroxidation, an increase in protein oxidation has also been demonstrated in the brains of patients with Alzheimer’s disease and MCI [39, 40]. In addition, oxidative damage to nuclear and mitochondrial DNA was found in temporal, frontal, and parietal lobe of MCI patients [40]. The accumulation of these oxidized proteins and DNA is known to disrupt neuronal function and lead to an increase in cytoplasmic inclusions and loss of synaptic transmission, all of which might strongly contribute to exacerbating cognitive dysfunction and neurodegenerative processes involved in dementia [41].
Cardiopulmonary fitness is associated with better executive function performance in CAD patients [28], suggesting that exercise intervention might confer neuroprotective benefits. Meta-analyses of randomized controlled trials in patients with MCI have demonstrated that exercise significantly improves cognitive performance on multiple tests examining global cognition and executive function domains [42, 43]. However, the cognitive benefits of exercise are of variable efficacy, especially in those without dementia but at high risk of cognitive decline [24, 44]. Increased lipid peroxidation may, in part, result from a relative decrease in antioxidant enzyme activities [45]. One of the mechanisms by which exercise improves cognition is by increasing the brain’s natural antioxidant defense mechanism, which facilitates adaptation of the antioxidant repair system and increases its resistance to the harmful effects of OS [46]. However, elevated lipid peroxidation ratios at baseline might decrease the capacity of exercise to restore the antioxidant defense system, limiting its beneficial effect on cognition. This notion is supported by our current findings, which showed that greater lipid peroxidation ratios measured at baseline was associated with less improvement in verbal memory and executive function performance over 24 weeks of a CR program. In addition to measuring lipid peroxidation markers, future studies should evaluate differences in antioxidant levels and the effect of antioxidant systems (glutathione, glutathione peroxidase, glutathione reductase, and glutathione S-transferases) on cognitive response to exercise. Further, increasing the antioxidant defense system through multimodal interventions involving both exercise and antioxidant therapy might create an additive or synergistic positive effect on cognition. Our previous work in CAD patients showed that combining exercise intervention and treatment with omega-3 polyunsaturated fatty acid did not improve overall cognitive performance, although some benefit was demonstrated for verbal memory [47]. It has yet to be determined whether adding antioxidant therapy to exercise would be more beneficial.
Many assays are available to measure lipid peroxidation; however, no single assay is an accurate measure of the whole process [48]. An increase in concentrations of late-stage lipid peroxidation products, in particular the 8-isoprostane and malondialdehyde, is the evidence most frequently reported as the extent of lipid damage due to ROS. In the present study, we evaluated the possible involvement of lipid peroxidation in patients with VaMCI by assessing differences in the individual concentration of lipid peroxidation products, as well as the ratios of late- to early- stage products. Since lipid peroxidation can be divided into multiple steps with each step giving rise to different end products, assessing the relative abundance of early- and late-stage products provide a preliminary measure of lipid peroxidation progression. Although elevated concentrations of the individual products have traditionally been considered as evidence of lipid peroxidation, the ratios between late- to early- stage products were explored to possibly provide an alternative approach, reflecting a more dynamic measure associated with the multi-step process of lipid peroxidation. The use of ratios was chosen to understand the balance of the system and to eliminate potential influence of external factors, such as nutritional status [49]. Quantifying ratios in longitudinal studies may help clarify the role of altered lipid peroxidation and cognitive decline in patients with VaMCI.
The use of monoclonal-antibody techniques directed against LDL that has undergone peroxidation would be valuable to increase the specificity of lipid peroxidation assessment in plasma samples. In addition, malondialdehyde (MDA) is the most abundant aldehyde product resulting from lipid peroxidation and widely used as a biomarker of lipid peroxidation [50]. Increased levels of MDA have been found in plasma of patients with MCI and Alzheimer’s disease [51]. Future studies should assess MDA and oxidized LDL levels to evaluate whether findings can be replicated using these OS parameters.
The present study has some limitations. First, the relatively small number of individuals in the VaMCI group may limit our ability to detect significant differences in individual lipid peroxidation markers, though significant differences between the groups in the ratios of late- to early-stage markers were detected. However, as expected, the proportion of VaMCI patients in our CAD population is higher than the prevalence reported for general elderly population [7]. Future studies with larger sample sizes are warranted. Although we included VO2 peak as a covariate in all of our analyses examining associations between baseline lipid peroxidation markers and changes in cognition, the VaMCI group had significantly lower cardiopulmonary fitness, which has been shown previously to predict less cognitive improvement following CR [28]. However, the results were confirmed by our post-hoc analysis, which showed that CAD patients in the low lipid peroxidation group demonstrated greater improvement in executive function compared to those in the high lipid peroxidation group, suggesting that baseline lipid peroxidation levels may be an important mediator of cognitive response to exercise. Similarly, the total years of education was greater in the VaMCI group compared to CAD controls. While education was added as a covariate in all analyses, this issue can only be partially mitigated by statistical correction and may still have influenced the findings. Third, our VaMCI criteria did not include neuroimaging evidence of small vessel disease. While neuroimaging provides important evidence for cerebrovascular disease, clinical history and neurological examination can also be reliable sources of objective evidence in the absence of neuroimaging. As shown in Table 1 and by our previous work, the CAD patients in this study have demonstrated cerebrovascular risk factors [52], neuroimaging changes [53, 54], and cognitive impairment [27, 28] that would meet the criteria of possible VaMCI. Nevertheless, future studies should confirm the current findings in VaMCI patients who also have neuroimaging evidence of small vessel disease. Finally, this study investigated only two markers of late-stage lipid peroxidation (4-HNE and 8-ISO), and examining other markers of lipid peroxidation may be warranted.
In conclusion, cognitive impairment is highly prevalent in those with CAD. Our results suggest that altered lipid peroxidation might be implicated in VaMCI. Greater lipid peroxidation ratios also predicted smaller cognitive improvements in response to a 24-week exercise intervention. A redox imbalance might lead to neuronal mitochondrial damage, which may play an important role in accelerating the cognitive consequences of vascular disease. Future efforts should aim to confirm these findings in a larger and longer longitudinal study with a well-defined cohort of patients with VaMCI to validate lipid peroxidation markers as early risk markers of prodromal vascular neurocognitive disorder. Antioxidant therapy might be explored as a promising therapeutic strategy to enhance the benefit of exercise to improve cognition in VaMCI.
ACKNOWLEDGMENTS
Funding support was provided by the Canadian Institutes of Health Research (Lanctot MOP-114913) and the Alzheimer Society of Canada Research Program Doctoral Award.
Authors’ disclosures available online (http://j-alz.com/manuscript-disclosures/16-1248r2).
REFERENCES
[1] | de Bruijn RF , Ikram MA ((2014) ) Cardiovascular risk factors and future risk of Alzheimer’s disease. BMC Med 12: , 130. |
[2] | Newman AB , Fitzpatrick AL , Lopez O , Jackson S , Lyketsos C , Jagust W , Ives D , Dekosky ST , Kuller LH ((2005) ) Dementia and Alzheimer’s disease incidence in relationship to cardiovascular disease in the Cardiovascular Health Study cohort. J Am Geriatr Soc 53: , 1101–1107. |
[3] | Evered LA , Silbert BS , Scott DA , Maruff P , Ames D ((2016) ) Prevalence of dementia 7.5 years after coronary artery bypass graft surgery. Anesthesiology 125: , 62–71. |
[4] | Ganguli M , Lee CW , Snitz BE , Hughes TF , McDade E , Chang CC ((2015) ) Rates and risk factors for progression to incident dementia vary by age in a population cohort. Neurology 84: , 72–80. |
[5] | Kumral E , Gulluoglu H , Alakbarova N , Deveci EE , Colak AY , Caginda AD , Evyapan D , Orman M ((2015) ) Cognitive decline in patients with leukoaraiosis within 5 years after initial stroke. J Stroke Cerebrovasc Dis 24: , 2338–2347. |
[6] | Lorius N , Locascio JJ , Rentz DM , Johnson KA , Sperling RA , Viswanathan A , Marshall GA , Alzheimer’s Disease Neuroimaging Initiative ((2015) ) Vascular disease and risk factors are associated with cognitive decline in the Alzheimer disease spectrum. Alzheimer Dis Assoc Disord 29: , 18–25. |
[7] | Gorelick PB , Scuteri A , Black SE , Decarli C , Greenberg SM , Iadecola C , Launer LJ , Laurent S , Lopez OL , Nyenhuis D , Petersen RC , Schneider JA , Tzourio C , Arnett DK , Bennett DA , Chui HC , Higashida RT , Lindquist R , Nilsson PM , Roman GC , Sellke FW , Seshadri S ; American Heart Association Stroke Council, Council on Epidemiology Prevention, Council on Cardiovascular Nursing, Council on Cardiovascular Radiology and Intervention, and Council on Cardiovascular Surgery and Anesthesia ((2011) ) Vascular contributions to cognitive impairment and dementia: A statement for healthcare professionals from the American Heart Association/American Stroke Association. Stroke 42: , 2672–2713. |
[8] | O’Brien JT , Erkinjuntti T , Reisberg B , Roman G , Sawada T , Pantoni L , Bowler JV , Ballard C , DeCarli C , Gorelick PB , Rockwood K , Burns A , Gauthier S , DeKosky ST ((2003) ) Vascular cognitive impairment. Lancet Neurol 2: , 89–98. |
[9] | Gainotti G , Ferraccioli M , Vita MG , Marra C ((2008) ) Patterns of neuropsychological impairment in MCI patients with small subcortical infarcts or hippocampal atrophy. J Int Neuropsychol Soc 14: , 611–619. |
[10] | Reed BR , Mungas DM , Kramer JH , Ellis W , Vinters HV , Zarow C , Jagust WJ , Chui HC ((2007) ) Profiles of neuropsychological impairment in autopsy-defined Alzheimer’s disease and cerebrovascular disease. Brain 130: , 731–739. |
[11] | Barnham KJ , Masters CL , Bush AI ((2004) ) Neurodegenerative diseases and oxidative stress. Nat Rev Drug Discov 3: , 205–214. |
[12] | Ho E , Karimi Galougahi K , Liu CC , Bhindi R , Figtree GA ((2013) ) Biological markers of oxidative stress: Applications to cardiovascular research and practice. Redox Biol 1: , 483–491. |
[13] | Halliwell B ((2007) ) Biochemistry of oxidative stress. Biochem Soc Trans 35: , 1147–1150. |
[14] | Yao JK , Keshavan MS ((2011) ) Antioxidants, redox signaling, and pathophysiology in schizophrenia: An integrative view. Antioxid Redox Signal 15: , 2011–2035. |
[15] | Gutteridge JM , Halliwell B ((2000) ) Free radicals and antioxidants in the year 2000. A historical look to the future. Ann N Y Acad Sci 899: , 136–147. |
[16] | Lopes da Silva S , Vellas B , Elemans S , Luchsinger J , Kamphuis P , Yaffe K , Sijben J , Groenendijk M , Stijnen T ((2014) ) Plasma nutrient status of patients with Alzheimer’s disease: Systematic review and meta-analysis. Alzheimers Dement 10: , 485–502. |
[17] | Pratico D , Clark CM , Liun F , Rokach J , Lee VY , Trojanowski JQ ((2002) ) Increase of brain oxidative stress in mild cognitive impairment: A possible predictor of Alzheimer disease. Arch Neurol 59: , 972–976. |
[18] | Liu H , Zhang J ((2012) ) Cerebral hypoperfusion and cognitive impairment: The pathogenic role of vascular oxidative stress. Int J Neurosci 122: , 494–499. |
[19] | de Sousa CV , Sales MM , Rosa TS , Lewis JE , de Andrade RV , Simoes HG ((2017) ) The antioxidant effect of exercise: A systematic review and meta-analysis. Sports Med 47: , 277–293. |
[20] | Radak Z , Suzuki K , Higuchi M , Balogh L , Boldogh I , Koltai E ((2016) ) Physical exercise, reactive oxygen species and neuroprotection. Free Radic Biol Med 98: , 187–196. |
[21] | Radak Z , Chung HY , Goto S ((2008) ) Systemic adaptation to oxidative challenge induced by regular exercise. Free Radic Biol Med 44: , 153–159. |
[22] | Ahlskog JE , Geda YE , Graff-Radford NR , Petersen RC ((2011) ) Physical exercise as a preventive or disease-modifying treatment of dementia and brain aging. Mayo Clin Proc 86: , 876–884. |
[23] | Gates N , Fiatarone Singh MA , Sachdev PS , Valenzuela M ((2013) ) The effect of exercise training on cognitive function in older adults with mild cognitive impairment: A meta-analysis of randomized controlled trials. Am J Geriatr Psychiatry 21: , 1086–1097. |
[24] | Young J , Angevaren M , Rusted J , Tabet N ((2015) ) Aerobic exercise to improve cognitive function in older people without known cognitive impairment. Cochrane Database Syst Rev, CD005381. |
[25] | Zheng G , Xia R , Zhou W , Tao J , Chen L ((2016) ) Aerobic exercise ameliorates cognitive function in older adults with mild cognitive impairment: A systematic review and meta-analysis of randomised controlled trials. Br J Sports Med, doi: 10.1136/bjsports-2015-095699 |
[26] | Saleem M , Bandaru VV , Herrmann N , Swardfager W , Mielke MM , Oh PI , Shammi P , Kiss A , Haughey NJ , Rovinski R , Lanctot KL ((2013) ) Ceramides predict verbal memory performance in coronary artery disease patients undertaking exercise: A prospective cohort pilot study. BMC Geriatr 13: , 135. |
[27] | Swardfager W , Herrmann N , Marzolini S , Saleem M , Shammi P , Oh PI , Albert PR , Daigle M , Kiss A , Lanctot KL ((2011) ) Brain derived neurotrophic factor, cardiopulmonary fitness and cognition in patients with coronary artery disease. Brain Behav Immun 25: , 1264–1271. |
[28] | Swardfager W , Herrmann N , Marzolini S , Saleem M , Kiss A , Shammi P , Oh PI , Lanctot KL ((2010) ) Cardiopulmonary fitness is associated with cognitive performance in patients with coronary artery disease. J Am Geriatr Soc 58: , 1519–1525. |
[29] | Molloy DW , Standish TI ((1997) ) A guide to the standardized Mini-Mental State Examination. Int Psychogeriatr 9: (Suppl 1), 8794; discussion 143–150. |
[30] | Hachinski V , Iadecola C , Petersen RC , Breteler MM , Nyenhuis DL , Black SE , Powers WJ , DeCarli C , Merino JG , Kalaria RN , Vinters HV , Holtzman DM , Rosenberg GA , Wallin A , Dichgans M , Marler JR , Leblanc GG ((2006) ) National Institute of Neurological Disorders and Stroke-Canadian Stroke Network vascular cognitive impairment harmonization standards. Stroke 37: , 2220–2241. |
[31] | Andreazza AC , Kapczinski F , Kauer-Sant’Anna M , Walz JC , Bond DJ , Goncalves CA , Young LT , Yatham LN ((2009) ) 3-Nitrotyrosine and glutathione antioxidant system in patients in the early and late stages of bipolar disorder. J Psychiatry Neurosci 34: , 263–271. |
[32] | Scola G , McNamara RK , Croarkin PE , Leffler JM , Cullen KR , Geske JR , Biernacka JM , Frye MA , DelBello MP , Andreazza AC ((2016) ) Lipid peroxidation biomarkers in adolescents with or at high-risk for bipolar disorder. J Affect Disord 192: , 176–183. |
[33] | Schrag M , Mueller C , Zabel M , Crofton A , Kirsch WM , Ghribi O , Squitti R , Perry G ((2013) ) Oxidative stress in blood in Alzheimer’s disease and mild cognitive impairment: A meta-analysis. Neurobiol Dis 59: , 100–110. |
[34] | Padurariu M , Ciobica A , Hritcu L , Stoica B , Bild W , Stefanescu C ((2010) ) Changes of some oxidative stress markers in the serum of patients with mild cognitive impairment and Alzheimer’s disease. Neurosci Lett 469: , 6–10. |
[35] | Rockwood K , Wentzel C , Hachinski V , Hogan DB , MacKnight C , McDowell I ((2000) ) Prevalence and outcomes of vascular cognitive impairment. Vascular Cognitive Impairment Investigators of the Canadian Study of Health and Aging. Neurology 54: , 447–451. |
[36] | Sachdev P1 , Kalaria R , O’Brien J , Skoog I , Alladi S , Black SE , Blacker D , Blazer DG , Chen C , Chui H , Ganguli M , Jellinger K , Jeste DV , Pasquier F , Paulsen J , Prins N , Rockwood K , Roman G , Scheltens P ; Internationlal Society for Vascular Behavioral and Cognitive Disorders ((2014) ) Diagnostic criteria for vascular cognitive disorders: A VASCOG statement. Alzheimer Dis Assoc Disord 28: , 206–218. |
[37] | Taylor RS , Brown A , Ebrahim S , Jolliffe J , Noorani H , Rees K , Skidmore B , Stone JA , Thompson DR , Oldridge N ((2004) ) Exercise-based rehabilitation for patients with coronary heart disease: Systematic review and meta-analysis of randomized controlled trials. Am J Med 116: , 682–692. |
[38] | Anderson L , Oldridge N , Thompson DR , Zwisler AD , Rees K , Martin N , Taylor RS ((2016) ) Exercise-based cardiac rehabilitation for coronary heart disease: Cochrane systematic review and meta-analysis. J Am Coll Cardiol 67: , 1–12. |
[39] | Butterfield DA , Poon HF , St Clair D , Keller JN , Pierce WM , Klein JB , Markesbery WR ((2006) ) Redox proteomics identification of oxidatively modified hippocampal proteins in mild cognitive impairment: Insights into the development of Alzheimer’s disease. Neurobiol Dis 22: , 223–232. |
[40] | Wang J , Markesbery WR , Lovell MA ((2006) ) Increased oxidative damage in nuclear and mitochondrial DNA in mild cognitive impairment. J Neurochem 96: , 825–832. |
[41] | Butterfield DA , Boyd-Kimball D , Castegna A ((2003) ) Proteomics in Alzheimer’s disease: Insights into potential mechanisms of neurodegeneration. J Neurochem 86: , 1313–1327. |
[42] | Tsai CL , Chen FC , Pan CY , Wang CH , Huang TH , Chen TC ((2014) ) Impact of acute aerobic exercise and cardiorespiratory fitness on visuospatial attention performance and serum BDNF levels. Psychoneuroendocrinology 41: , 121–131. |
[43] | Ohman H , Savikko N , Strandberg TE , Pitkala KH ((2014) ) Effect of physical exercise on cognitive performance in older adults with mild cognitive impairment or dementia: A systematic review. Dement Geriatr Cogn Disord 38: , 347–365. |
[44] | Baker LD , Frank LL , Foster-Schubert K , Green PS , Wilkinson CW , McTiernan A , Plymate SR , Fishel MA , Watson GS , Cholerton BA , Duncan GE , Mehta PD , Craft S ((2010) ) Effects of aerobic exercise on mild cognitive impairment: A controlled trial. Arch Neurol 67: , 71–79. |
[45] | Sultana R , Piroddi M , Galli F , Butterfield DA ((2008) ) Protein levels and activity of some antioxidant enzymes in hippocampus of subjects with amnestic mild cognitive impairment. Neurochem Res 33: , 2540–2546. |
[46] | Radak Z , Taylor AW , Ohno H , Goto S ((2001) ) Adaptation to exercise-induced oxidative stress: From muscle to brain. Exerc Immunol Rev 7: , 90–107. |
[47] | Mazereeuw G , Herrmann N , Oh PI , Ma DW , Wang CT , Kiss A , Lanctot KL ((2016) ) Omega-3 Fatty acids, depressive symptoms, and cognitive performance in patients with coronary artery disease: Analyses from a randomized, double-blind, placebo-controlled trial. J Clin Psychopharmacol 36: , 436–444. |
[48] | Halliwell B , Chirico S ((1993) ) Lipid peroxidation: Its mechanism, measurement, and significance. Am J Clin Nutr 57: , 715S–724S; discussion 724S-725S. |
[49] | Repetto M , Semprine J , Boveris A ((2012) ) Lipid peroxidation: Chemical mechanism, biological implications and analytical determination. In Lipid Peroxidation, Catala A, ed. InTech. doi: 10.5772/45943. Available from: https://www.intechopen.com/books/lipid-peroxidation/lipid-peroxidation-chemical-mechanism-biological-implications-and-analytical-determination. |
[50] | Esterbauer H , Cheeseman KH ((1990) ) Determination of aldehydic lipid peroxidation products: Malonaldehyde and 4-hydroxynonenal. Methods Enzymol 186: , 407–421. |
[51] | Greilberger J , Koidl C , Greilberger M , Lamprecht M , Schroecksnadel K , Leblhuber F , Fuchs D , Oettl K ((2008) ) Malondialdehyde, carbonyl proteins and albumin-disulphide as useful oxidative markers in mild cognitive impairment and Alzheimer’s disease. Free Radic Res 42: , 633–638. |
[52] | Lanctot KL , O’Regan J , Schwartz Y , Swardfager W , Saleem M , Oh PI , Herrmann N ((2014) ) Assessing cognitive effects of anticholinergic medications in patients with coronary artery disease. Psychosomatics 55: , 61–68. |
[53] | MacIntosh BJ , Swardfager W , Robertson AD , Tchistiakova E , Saleem M , Oh PI , Herrmann N , Stefanovic B , Lanctot KL ((2015) ) Regional cerebral arterial transit time hemodynamics correlate with vascular risk factors and cognitive function in men with coronary artery disease. AJNR Am J Neuroradiol 36: , 295–301. |
[54] | Santiago C , Herrmann N , Swardfager W , Saleem M , Oh PI , Black SE , Lanctot KL ((2015) ) White matter microstructural integrity is associated with executive function and processing speed in older adults with coronary artery disease. Am J Geriatr Psychiatry 23: , 754–763. |