Preliminary Study of Plasma Exosomal Tau as a Potential Biomarker for Chronic Traumatic Encephalopathy
Abstract
Background:
Chronic traumatic encephalopathy (CTE) is a tauopathy associated with prior exposure to repetitive head impacts, such as those incurred through American football and other collision sports. Diagnosis is made through neuropathological examination. Many of the clinical features of CTE are common in the general population, with and without a history of head impact exposure, making clinical diagnosis difficult. As is now common in the diagnosis of other neurodegenerative disorders, such as Alzheimer’s disease, there is a need for methods to diagnose CTE during life through objective biomarkers.
Objective:
The aim of this study was to examine tau-positive exosomes in plasma as a potential CTE biomarker.
Methods:
Subjects were 78 former National Football League (NFL) players and 16 controls. Extracellular vesicles were isolated from plasma. Fluorescent nanoparticle tracking analysis was used to determine the number of vesicles staining positive for tau.
Results:
The NFL group had higher exosomal tau than the control group (p < 0.0001). Exosomal tau discriminated between the groups, with 82% sensitivity, 100% specificity, 100% positive predictive value, and 53% negative predictive value. Within the NFL group, higher exosomal tau was associated with worse performance on tests of memory (p = 0.0126) and psychomotor speed (p = 0.0093).
Conclusion:
These preliminary findings suggest that exosomal tau in plasma may be an accurate, noninvasive CTE biomarker.
INTRODUCTION
Chronic traumatic encephalopathy (CTE) is a progressive neurodegenerative disease associated with prior exposure to repetitive head impacts, primarily through contact sports such as boxing and American football [1–9]. Originally termed, “dementia pugilistica” when observed in boxers, CTE is distinguished neuropathologically from other tauopathies, such as Alzheimer’s disease (AD) and frontotemporal lobar degeneration, by the regional distribution of tau aggregates. In CTE, the pathognomonic finding is an abnormal perivascular accumulation of hyperphosphorylated microtubule-associate protein tau (p-tau) as neurofibrillary tangles, astrocytic inclusions, and neurites, distributed irregularly and preferentially at the depths of the cortical sulci [6, 10]. As the disease worsens, the p-tau pathology becomes more widespread, found most prominently in the frontal and temporal lobes, amygdala, hippocampus, and entorhinal cortex [4, 11, 12]. At this time, CTE can only be diagnosed by neuropathological examination.
The clinical presentation of CTE involves a constellation of cognitive (e.g., episodic memory impairment and executive dysfunction), mood (e.g., depression, apathy), and behavioral (e.g., aggression, impulsivity) changes, with dementia often reported as the disease progresses [7]. In some cases, predominantly former boxers, motor signs and symptoms (e.g., gait instability, rigidity) are also seen [12–14]. A purely clinical diagnosis of CTE is difficult, if not impossible, because of the lack of any unique aspects of the clinical course and presentation of CTE, as well as the fact that many of the clinical features are common in the general population, with and without a history of head impact exposure [15]. However, the combination of clinical features and biomarker evidence of specific underlying neuropathology is now common in the diagnosis of other neurodegenerative diseases, such as AD [16–18].
Potential neuroimaging biomarkers for CTE include: volumetric magnetic resonance imaging (MRI) [19–22]; diffusion tensor imaging [23]; MRI detection of specific structural abnormalities common in CTE (e.g., cavum septum pellucidum [24, 25]); functional MRI measuring specific patterns of connectivity [19, 26]; and magnetic resonance spectroscopy [27]. These approaches, however, will likely only serve as proxy biomarkers, rather than specific markers of the underlying p-tau pathology. One emerging method to detect and measure brain p-tau directly involves positron emission tomography (PET) radioligands that are specific for paired helical filament tau [28–31]. However, once refined and validated, PET tau will remain expensive, time-demanding, and relatively invasive.
Cerebrospinal fluid (CSF) measures of amyloid-β peptide, p-tau, and total tau are accepted biomarkers for the diagnosis of AD [32–34]. Although CSF p-tau may prove to be an appropriate biomarker for CTE, its routine use in screening or as a diagnostic test conducted by a primary care physician will be limited by the requirement for a lumbar puncture, a procedure that remains controversial and viewed as invasive, complicated, and time consuming, and is often feared by patients [35]. Therefore, the exploration of potential blood-based biomarkers for other neurodegenerative diseases has grown tremendously in recent years[18, 36–40]. Studies using ultrasensitive single-molecule immunoarray technology to measure blood tau levels have been promising [41–43], though it remains unclear if the measurement of tau in plasma is an accurate marker of brain tau pathology [44]. That is, tau that can be detected in plasma has theoretically been released by damaged axons into the CSF and then leaked from CSF into blood, crossing the blood-brain barrier (BBB). Thus, the measurement of CSF and plasma tau does not necessarily reflect tau within brain cells (i.e., neurons, glia).
Exosomes are nanovesicles released by most cells throughout the body, including the brain, into the extracellular environment through exocytosis of plasma membrane-anchored vesicles [45]. The molecular content or cargo of exosomes directly reflects the content of the cell of origin. They are released into body fluids such as CSF, blood, and urine and are very stable, thus providing the potential to serve as biomarkers for the diagnosis and prognosis of a variety of diseases. Taylor and Doellgast [46] made the initial observation of circulating microvesicles and their diagnostic potential in 1979. The term, “exosomes” was first used in 1981 by Trams and colleagues [47]. Since that time, exosomes have been the focus of growing research, primarily in the area of tumor diagnosis [45, 48]. More recently, investigations of exosomes in neurodegenerative disease have focused on cell-to-cell transmission of pathogenic proteins [49, 50]. Because exosomes readily cross the BBB, their potential as a nanodelivery system from the periphery into the brain for the treatment of neurodegenerative disease is also being explored [51]. Additionally, because exosomes cross the BBB from the brain out to the periphery, with their cargo intact, and because their cargo reflects the intracellular milieu of their point of origin, the measurement of brain-derived exosomes may serve as a direct and noninvasive blood test measuring brain tau pathology [44].
Fiandaca and colleagues isolated exosomes enriched for neural sources through neural cell adhesion molecule (NCAM) antibody in either serum or plasma from patients with clinically diagnosed amnestic mild cognitive impairment (MCI) or AD dementia, patients with frontotemporal dementia, and cognitively healthy controls [52]. They then measured several proteins associated with AD and frontotemporal lobar degeneration pathology, including p-tau and total tau, using ELISA. They report that a profile of these protein levels had high accuracy in classifying AD (MCI or dementia) from controls and frontotemporal dementia from controls. Furthermore, in a subgroup of subjects with measurements at two time-points, from one to ten years after baseline, the exosomal protein levels predicted later clinical decline. More recently, Goetzl and colleagues examined autolysosomal proteins in brain-derived exosomes and found that the exosomal protein levels were able to distinguish patients with AD from case controls and reflected AD pathology up to 10 years before symptom onset [53].
The aim of this study was to establish preliminary support for the use of exosomal tau measurement in plasma as a potential biomarker for CTE. To date, every published case of neuropathologically-confirmed CTE has had a history of repetitive head impacts, such as those experienced in American football [6, 7], indicating that head impact exposure is a necessary (but not sufficient) variable for the development of the disease. We, therefore, studied a group of symptomatic former National Football League (NFL) players with entry criteria selected to enrich the sample with individuals presumably at high risk for developing CTE, based on history of extensive exposure to repetitive head impacts, current symptoms, and age. That group was compared with a Control group comprised of asymptomatic individuals without any history of contact sport involvement, other exposure to repetitive head impacts, or even mild traumatic brain injury (TBI). We hypothesized that all subjects with elevated exosomal tau would be in the NFL group (i.e., no Control subjects would have elevated exosomal tau), but that not all NFL group subjects would have elevated exosomal tau.
MATERIALS AND METHODS
Study design and participants
This case-control study is part of Diagnosing and Evaluating Traumatic Encephalopathy using Clinical Tests (DETECT), a larger project developing potential CTE biomarkers. DETECT includes a sample of former NFL players and a control group of non-contact sport athletes. DETECT study procedures are described elsewhere [24, 54, 55]. The Boston University Medical Campus (BUMC) Institutional Review Board approved all study procedures. Prior to participation, all participants provided written informed consent.
The former NFL players in DETECT met the following inclusion criteria: male; age 40–69; minimum of 12 years of participation in organized tackle football; minimum of 2 years of play in the NFL at positions known to have extensive head impacts based on helmet accelerometer data (i.e., offensive and defensive linemen, linebackers, defensive backs, running backs) [56, 57]; and a self-report (through semi-structured telephone interview) of progressive cognitive, behavioral, and mood symptoms for a minimum of six months prior to participation. Control subjects met the following inclusion criteria: male; age 40–69; and participation in organized non-contact sports for a minimum of four years, with two years at the college level or beyond. Exclusion criteria for Control subjects were: history of military service; participation in organized sports with expected high exposure to head impacts; known or reported history of TBI, including concussion; diagnosis of dementia or other cognitive disorder; or self-reported symptoms of cognitive, mood, or behavioral impairment in the six months prior to entry. For the current study, there were 78 former NFL players and 16 Controls. Demographic characteristics are presented in Table 1. All former NFL players and none of the Controls met proposed diagnostic criteria for traumatic encephalopathy syndrome [13].
Procedures
Each subject was examined over a 2-3 day period at BUMC. Blood was drawn by venipuncture and immediately processed. Plasma samples were stored in 0.75 ml tubes at –80°C, packed in dry ice, and shipped overnight in one batch to the Exosome Sciences lab (Monmouth Junction, NJ). As part of the larger DETECT protocol, subjects were administered a battery of neuropsychological tests by a trained research assistant, and were administered the Hamilton Depression Rating Scale interview by a licensed psychiatrist or clinical psychologist. Subjects also completed several paper-and-pencil self-report measures of mood and behavior. All tests (see Table 2) were selected to measure the clinical features of CTE reported in studies of neuropathologically-confirmed cases [7, 13, 58]. All examiners were blind to the exosome findings at the time of administration and scoring.
Isolation of exosomes
Extracellular vesicles were isolated from the 0.75 ml plasma samples by size exclusion chromatography, performed using 1.0×10 cm columns packed with 2% agarose-based gel (Agarose Bead Technologies) [59, 60]. Plasma samples were applied to the column and it was then run isocratically. The elution was monitored at 280 nm and the void volume material (exosome fraction) was collected and pooled. The exosomal protein for each sample was determined by the Bradford protein assay using duplicate samples [61].
Nanoparticle tracking analysis
The size profiles and concentrations of the chromatographically isolated exosomes were performed using the Nanosight LM10 instrument equipped with a 405 nm laser, sCMOS camera and Nanoparticle Tracking Analysis (NTA) software (version 2.3) [60]. Exosome samples were diluted 1 : 10 in PBS and the samples were injected into the sample chamber. For each sample, two 30-second video clips were created and analyzed to define the vesicle number and size range. In light scattering mode, within the sample field, all vesicles are visualized and counted. Using the dilution of the sample, the NTA software calculates the total number of vesicles in the original sample. Additionally, using the viscosity of the solution and the vesicle Brownian motion, the NTA calculates the size of each vesicle. NTA demonstrated the size range of vesicles in the circulation of NFL and control subjects as 50–200 nm in diameter. This size range is consistent with the reported size of exosomes. Representative vesicle samples were stained for CD63, a tetraspanin marker of exosomes, demonstrating the identity as exosomes. An average of the total number of vesicles was calculated for each sample. To maintain constancy among samples, the NTA post acquisition settings were optimized and kept constant.
Fluorescent nanoparticle tracking analysis was used to determine the number of vesicles staining positive for tau. Fluoresbrite YG 100 nm Microspheres (5.8% CV) (Polysciences Inc., Warrington, PA) with an excitation maxima of 441 nm and emission maxima at 485 nm were routinely examined initially to assess instrument performance. The chromatographically isolated exosomes from all samples were diluted in PBS to the same concentration in a total volume of 100μl. Each exosome sample was incubated with 0.5μg mouse monoclonal antibody for microtubule-associated protein tau (clone D-8, from Santa Cruz SC-166060) at room temperature on a rocker for 2 h. The samples were then incubated with 2μl of anti-mouse Ig labeled with quantum dots (Qdot)-655 at 4°C overnight. Each sample was then diluted in PBS to a final volume of 500μl. These samples were then analyzed used the Nanosight LM10 in fluorescent-mode. To determine the background level, the initial settings for the LM10 were established using known negative samples. Once established, all samples were analyzed using the same settings. For each sample, two 30-second video clips were created and analyzed to define the number of fluorescent vesicles. In fluorescent mode, in order to visualize only those vesicles that have bound the tau antibody and Qdot-labeled second antibody and not unbound Qdots, the level limit of the Nanosight was set to detect only fluorescent spots with diameters greater than 50 nm. The visualized vesicles, within the sample field, are counted by the NTA software and using the dilution of the sample, the software calculates the total number of fluorescent vesicles in the original sample. Additionally, using the viscosity of the solution and the vesicle Brownian motion, the NTA calculates the size of each vesicle.
Statistical analyses
For all statistical tests, a p-value below 0.05 was considered statistically significant. All statistical analyses were conducted using SAS/STAT® Version 9.4 for Windows. The standard deviations of plasma exosomal tau levels were significantly different between the Control and NFL groups (0.2 for Control and 3.0 for NFL, F-test = 265.6, p < 0.0001). Therefore, exosomal tau levels were transformed logarithmically, resulting in considerable stabilization of the variability between the two groups (0.5 for Control and 0.9 for NFL, F-test = 2.71 p = 0.0339); these log-transformed values were used in all subsequent analyses. Group comparisons of age, years of education, body mass index (BMI), total exosome level, and exosomal tau level were performed using independent t-tests. We used analysis of covariance (ANCOVA) to measure the mean difference of exosomal tau levels between groups adjusted for age and BMI (to serve as a control for the possible small amount of tau in muscle). A Receiver Operating Characteristic (ROC) curve was calculated from a simple logistic regression to assess the predictive power of exosomal tau in discriminating between NFL and Control groups. Cross-validation was employed to calculate a 95% confidence interval for the c-statistic which is a summary measure of the ROC curve [62].
Multivariate mixed-effects linear regression analyses were used to model the associations of plasma exosomal tau levels and cognitive, mood, and behavior data within the NFL group. The cognitive, mood, and behavior scores were treated as multivariate dependent variables, while the respective plasma exosomal tau levels, age, and years of education were treated as the independent variables. We allowed for outcome-specific fixed effects and subject- and domain-specific random effects. Hence, we modeled two levels of correlations in our outcomes to account for possible inflation of Type I error: (1) correlations of all test scores from the same subjects, and (2) correlations of all scores within the same domain. These multivariate analyses are more realistic models of the outcomes than using independent regression models for each outcome [63]. Since all information within each subject is utilized, we are able to provide more interpretable and consistent results than simpler statistical models. Based on the results of these mixed effects models, partial correlations between each cognitive, mood, and behavior test score and the log of exosomal tau were calculated adjusted for age and years of education.
RESULTS
Group comparisons on age, education, BMI, total exosomes and exosomal tau are presented in Table 1. The groups did not differ in total plasma exosomes, but the NFL group had significantly higher plasma exosomal tau than the Control group (p < 0.0001).Figure 1 depicts the unadjusted distribution of exosomal tau between the Controls and NFL groups. Parameter estimates of the ANCOVA model are shown in Table 2. Controlling for age and BMI, the NFL group again had significantly higher exosomal tau levels than the Control group (p < 0.0001). Figure 2 shows the ROC curve of the model with exosomal tau predicting classification between groups. The model successfully discriminated between the groups (c-statistic = 0.97, 95% CI = 0.94–1.00). The C-statistic is maximized when the plasma exosomal tau level is set to 0. Using 0 as a threshold resulted in 82% sensitivity, 100% specificity, 100% positive predictive value and 53% negative predictive value. Within the NFL group, plasma exosomal tau levels were significantly correlated with the WAIS-R Digit Symbol test (Fig. 3) and the NAB List Learning test; higher exosomal tau was associated with worse test performance (Table 3). Exosomal tau was not significantly correlated with any of the measures of mood or behavior. Because of the limited amount and variability of exosomal tau in the control group, as well as the small sample size, we do not report correlations between exosomal tau and the cognitive, mood, or behavior measures for the control group.
DISCUSSION
Results suggest that plasma exosomal tau is significantly elevated in a group of symptomatic former NFL players compared to a control group of asymptomatic former non-contact sport athletes without any history of concussion or other TBI. Moreover, the number of tau-positive plasma exosomes was significantly correlated with neuropsychological test performance; the higher the plasma exosomal tau, the worse the performance in the areas of memory and psychomotor speed. The exosomal tau levels had excellent sensitivity, specificity, and positive predictive value for discriminating between groups, though negative predictive value was substantially lower.
It is noteworthy that the plasma exosomal tau levels were only significantly correlated with cognitive functioning (i.e., psychomotor speed and memory) and not with measures of mood and behavior. Although there are many potential interpretations to this finding, the most parsimonious explanation is that elevated neuronal tau is a better marker of the cognitive features of neurodegeneration and not the mood and behavioral features. The cognitive features of CTE have been reported to occur later in the course of the disease than the mood and behavioral features [58]. It is possible that the mood and behavioral features may have multiple potential etiologies, in addition to CTE-associated tau degeneration, whereas the cognitive changes are more consistently due to the tau degeneration. Future research with exosomal tau and neuroimaging biomarkers (including tau PET imaging), should address these possible explanations for our findings.
We hypothesized that elevations in plasma exosomal tau would not be detected in the Control group and would only be detected in the NFL group, whose extensive exposure to repetitive head impacts presumably puts them at risk for CTE. We further hypothesized that not all subjects in the NFL group would have elevated exosomal tau because we would not expect that all former NFL players in the study would have CTE. ROC curve analyses supported both hypotheses, with elevated exosomal tau having 100% positive predictive value but only 53% negative predictive value. Without neuropathological confirmation as a true diagnostic standard, the classification accuracy of a proposed biomarker for disease would not be expected to be very high. The validation of biomarkers for CTE will need to rely on this approach of comparing a high risk group (based on head impact exposure history, clinical features and course) and a control group on the biomarker under study [64]. The combination of a non-invasive blood test for exosomal tau and a clinical evaluation to examine diagnostic criteria for the clinical history and features of CTE [13] may improve diagnostic accuracy.
The methods used to isolate exosomes for this study did not include markers of cell derivation and, therefore, we cannot be completely confident that the exosomes were brain-derived. However, we found no significant between-group difference in the measure of total exosomes. In contrast, we found significant differences and 100% positive predictive value in the measure of exosomal tau. This finding suggests that our approach for exosome isolation yielded brain-derived exosomes, and that our measure of exosomal tau reflected tau derived from brain cells. The examination of exosomal cargo proteins is often used as a method of determining the cells of origin of the exosomes in body fluids [61]. However, it is possible that our methodology may have resulted in a lack of specificity of the derivation of the exosomes. Although tau is most abundant in the brain, it is also found in peripheral nervous system cells, and, to a lesser extent, in muscle [65], kidney, lung, and testis [66]. It is possible, therefore, that some of the tau antibody targeted exosomes from non-brain-derived cells. Furthermore, within the brain, tau is most abundant in neuronal axons [67, 68], though it is also found in somatodendritic compartments [69] and in oligodendrocytes [70]. Therefore, even if the exosomal tau we measured was brain-derived, the approach we used would not differentiate between neuronal and non-neuronal cells. Future research should improve upon the selectivity of exosomal source by initially targeting cellular markers (e.g., through neural adhesion protein), followed by a validation of origin by noncoding RNA for specific cell types (e.g., neurons, glia). Finally, we selected a tau antibody (D-8) which binds to the tau epitope corresponding to amino acids 1–150 mapping at the N-terminus [71]. The antibody is also nonspecific to central nervous system (CNS) tau. Future research should include tau antibodies with greater CNS specificity, as well as specific tau isoforms associated with CTE and neurodegeneration [72], including cis p-tau, believed to be an early driver of neurodegeneration following repeated mild brain injuries [73].
Additional limitations to this study include the relatively small sample size, especially for the control group and the lack of any additional possible biomarker of CTE. Despite these limitations, our findings suggest that the measurement of exosomal tau in plasma may, after additional future research, prove to be an accurate, noninvasive biomarker for CTE. The ability to have a blood test to detect and quantify tau would be a tremendous advancement, not just for the diagnosis of CTE, but for AD and other neurodegenerative diseases [40, 74]. This would not only be useful as a diagnostic and disease progression biomarker, it could be used as a method of enriching participant recruitment and monitoring efficacy for anti-tau therapeutic trials. Because of the inability of this type of test to specify the pattern and location of tau deposition in the brain, it may not be able to differentiate between different tauopathies as a stand-alone test. However, at the very least, a blood test for exosomal tau could serve as an important office-based screen for CTE, with positive results being followed by more extensive multimodal imaging, including PET tau imaging [30].
ACKNOWLEDGMENTS
This study was supported by grants from the National Institutes of Health (R01 NS 078337; R56 NS 078337; P30 AG13846). Christine Baugh is currently supported by the National Institutes of Mental Health under award number T32MH019733. Participant travel was funded by gifts from JetBlue Airlines, the National Football League, and the NFL Players Association. All procedures related to exosome isolation and measurement were conducted at, and fully funded by Exosome Sciences, Inc., a subsidiary of Aethlon Medical, Inc.
Authors’ disclosures available online (http://j-alz.com/manuscript-disclosures/15-1028r2).
REFERENCES
[1] | DeKosky ST , Blennow K , Ikonomovic MD , Gandy S ((2013) ) Acute and chronic traumatic encephalopathies: Pathogenesis and biomarkers. Nat Rev Neurol 9: , 192–200. |
[2] | Jordan BD ((2013) ) The clinical spectrum of sport-related traumatic brain injury. Nat Rev Neurol 9: , 222–230. |
[3] | Kiernan PT , Montenigro PH , Solomon TM , McKee AC ((2015) ) Chronic traumatic encephalopathy: A neurodegenerative consequence of repetitive traumatic brain injury. Semin Neurol 35: , 20–28. |
[4] | McKee AC , Cantu RC , Nowinski CJ , Hedley-Whyte ET , Gavett BE , Budson AE , Santini VE , Lee HS , Kubilus CA , Stern RA ((2009) ) Chronic traumatic encephalopathy in athletes: Progressive tauopathy after repetitive head injury. J Neuropathol Exp Neurol 68: , 709–735. |
[5] | McKee AC , Stein TD , Kiernan PT , Alvarez VE ((2015) ) The neuropathology of chronic traumatic encephalopathy. Brain Pathol 25: , 350–364. |
[6] | McKee AC , Stern RA , Nowinski CJ , Stein TD , Alvarez VE , Daneshvar DH , Lee HS , Wojtowicz SM , Hall G , Baugh CM , Riley DO , Kubilus CA , Cormier KA , Jacobs MA , Martin BR , Abraham CR , Ikezu T , Reichard RR , Wolozin BL , Budson AE , Goldstein LE , Kowall NW , Cantu RC ((2013) ) The spectrum of disease in chronic traumatic encephalopathy. Brain 136: , 43–64. |
[7] | Montenigro PH , Corp DT , Stein TD , Cantu RC , Stern RA ((2015) ) Chronic traumatic encephalopathy: Historical origins and current perspective. Annu Rev Clin Psychol 11: , 309–330. |
[8] | Stein TD , Alvarez VE , McKee AC ((2014) ) Chronic traumatic encephalopathy: A spectrum of neuropathological changes following repetitive brain trauma in athletes and military personnel. Alzheimers Res Ther 6: , 4. |
[9] | Bieniek KF , Ross OA , Cormier KA , Walton RL , Soto-Ortolaza A , Johnston AE , DeSaro P , Boylan KB , Graff-Radford NR , Wszolek ZK , Rademakers R , Boeve BF , McKee AC , Dickson DW ((2015) ) Chronic traumatic encephalopathy pathology in a neurodegenerative disorders brain bank. Acta Neuropathol 130: , 877–889. |
[10] | McKee AC , Cairns NJ , Dickson DW , Folkerth RD , Keene CD , Litvan I , Perl DP , Stein TD , Paul Vonsattel J , Stewart W , Tripodis Y , Crary JF , Bieniek KF , Dams-O’Connor K , Alvarez VE , Gordon WA , the TBI/CTE, group ((2016) ) The first NINDS/NIBIB consensus meeting to define neuropathological criteria for the diagnosis of chronic traumatic encephalopathy. Acta Neuropathol 131: , 75–86. |
[11] | Goldstein LE , Fisher AM , Tagge CA , Zhang XL , Velisek L , Sullivan JA , Upreti C , Kracht JM , Ericsson M , Wojnarowicz MW , Goletiani CJ , Maglakelidze GM , Casey N , Moncaster JA , Minaeva O , Moir RD , Nowinski CJ , Stern RA , Cantu RC , Geiling J , Blusztajn JK , Wolozin BL , Ikezu T , Stein TD , Budson AE , Kowall NW , Chargin D , Sharon A , Saman S , Hall GF , Moss WC , Cleveland RO , Tanzi RE , Stanton PK , McKee AC ((2012) ) Chronic traumatic encephalopathy in blast-exposed military veterans and a blast neurotrauma mouse model. Sci Transl Med 4: , 134ra60. |
[12] | Omalu B , Bailes J , Hamilton RL , Kamboh MI , Hammers J , Case M , Fitzsimmons R ((2011) ) Emerging histomorphologic phenotypes of chronic traumatic encephalopathy in American athletes. Neurosurgery 69: , 173–183. |
[13] | Montenigro PH , Baugh CM , Daneshvar DH , Mez J , Budson AE , Au R , Katz DI , Cantu RC , Stern RA ((2014) ) Clinical subtypes of chronic traumatic encephalopathy: Literature review and proposed research diagnostic criteria for traumatic encephalopathy syndrome. Alzheimers Res Ther 6: , 68. |
[14] | Gardner A , Iverson GL , McCrory P ((2014) ) Chronic traumatic encephalopathy in sport: A systematic review. Br J Sports Med 48: , 84–90. |
[15] | Iverson GL , Gardner AJ , McCrory P , Zafonte R , Castellani RJ ((2015) ) A critical review of chronic traumatic encephalopathy. Neurosci Biobehav Rev 56: , 276–293. |
[16] | McKhann GM , Knopman DS , Chertkow H , Hyman BT , Jack CR Jr , Kawas CH , Klunk WE , Koroshetz WJ , Manly JJ , Mayeux R ((2011) ) The diagnosis of dementia due to Alzheimer’s disease: Recommendations from the National Institute on Aging-Alzheimer’s Association workgroups on diagnostic guidelines for Alzheimer’s disease. Alzheimers Dement 7: , 263–269. |
[17] | Dubois B , Feldman HH , Jacova C , Hampel H , Molinuevo JL , Blennow K , DeKosky ST , Gauthier S , Selkoe D , Bateman R , Cappa S , Crutch S , Engelborghs S , Frisoni GB , Fox NC , Galasko D , Habert MO , Jicha GA , Nordberg A , Pasquier F , Rabinovici G , Robert P , Rowe C , Salloway S , Sarazin M , Epelbaum S , de Souza LC , Vellas B , Visser PJ , Schneider L , Stern Y , Scheltens P , Cummings JL ((2014) ) Advancing research diagnostic criteria for Alzheimer’s disease: The IWG-2 criteria. Lancet Neurol 13: , 614–629. |
[18] | Weiner MW , Veitch DP , Aisen PS , Beckett LA , Cairns NJ , Cedarbaum J , Green RC , Harvey D , Jack CR , Jagust W , Luthman J , Morris JC , Petersen RC , Saykin AJ , Shaw L , Shen L , Schwarz A , Toga AW , Trojanowski JQ ((2015) ) 2014 Update of the Alzheimer’s Disease Neuroimaging Initiative: A review of papers published since its inception. Alzheimers Dement 11: , e1–e120. |
[19] | Koerte IK , Lin AP , Willems A , Muehlmann M , Hufschmidt J , Coleman MJ , Green I , Liao H , Tate DF , Wilde EA , Pasternak O , Bouix S , Rathi Y , Bigler ED , Stern RA , Shenton ME ((2015) ) A review of neuroimaging findings in repetitive brain trauma. Brain Pathol 25: , 318–349. |
[20] | Ng TS , Lin AP , Koerte IK , Pasternak O , Liao H , Merugumala S , Bouix S , Shenton ME ((2014) ) Neuroimaging in repetitive brain trauma. Alzheimers Res Ther 6: , 10. |
[21] | Shenton ME , Hamoda HM , Schneiderman JS , Bouix S , Pasternak O , Rathi Y , Vu MA , Purohit MP , Helmer K , Koerte I , Lin AP , Westin CF , Kikinis R , Kubicki M , Stern RA , Zafonte R ((2012) ) A review of magnetic resonance imaging and diffusion tensor imaging findings in mild traumatic brain injury. Brain Imaging Behav 6: , 137–192. |
[22] | Strain JF , Womack KB , Didehbani N , Spence JS , Conover H , Hart J Jr , Kraut MA , Cullum CM ((2015) ) Imaging correlates of memory and concussion history in retired National Football League athletes. JAMA Neurol 72: , 773–780. |
[23] | Hart J Jr , Kraut MA , Womack KB , Strain J , Didehbani N , Bartz E , Conover H , Mansinghani S , Lu H , Cullum CM ((2013) ) Neuroimaging of cognitive dysfunction and depression in aging retired National Football League players: A cross-sectional study. JAMA Neurol 70: , 326–335. |
[24] | Koerte IK , Hufschmidt J , Muehlmann M , Tripodis Y , Stamm JM , Pasternak O , Giwerc MY , Coleman MJ , Baugh CM , Fritts NG , Heinen F , Lin AP , Stern RA , Shenton ME ((2015) ) Cavum septi pellucidi in symptomatic former professional football players. J Neurotrauma, doi: 10.1089/neu.2015.3880 |
[25] | Gardner RC , Hess CP , Brus-Ramer M , Possin KL , Cohn-Sheehy BI , Kramer JH , Berger MS , Yaffe K , Miller B , Rabinovici GD ((2016) ) Cavum septum pellucidum in retired American pro-football players. J Neurotrauma 33: , 157–161. |
[26] | Hampshire A , MacDonald A , Owen AM ((2013) ) Hypoconnectivity and hyperfrontality in retired American football players. Sci Rep 3: , 2972. |
[27] | Lin AP , Ramadan S , Stern RA , Box HC , Nowinski CJ , Ross BD , Mountford CE ((2015) ) Changes in the neurochemistry of athletes with repetitive brain trauma: Preliminary results using localized correlated spectroscopy. Alzheimers Res Ther 7: , 13. |
[28] | Chien DT , Szardenings AK , Bahri S , Walsh JC , Mu F , Xia C , Shankle WR , Lerner AJ , Su MY , Elizarov A , Kolb HC ((2014) ) Early clinical PET imaging results with the novel PHF-tau radioligand [F18]-T808. J Alzheimers Dis 38: , 171–184. |
[29] | Gandy S , DeKosky ST ((2014) ) [18F]-T807 tauopathy PET imaging in chronic traumatic encephalopathy. F1000Res 3: , 229. |
[30] | Villemagne VL , Fodero-Tavoletti MT , Masters CL , Rowe CC ((2015) ) Tau imaging: Early progress and future directions. Lancet Neurol 14: , 114–124. |
[31] | Villemagne VL , Okamura N ((2014) ) In vivo tau imaging: Obstacles and progress. Alzheimers Dementia 10: , S254–S264. |
[32] | Molinuevo JL , Blennow K , Dubois B , Engelborghs S , Lewczuk P , Perret-Liaudet A , Teunissen CE , Parnetti L ((2014) ) The clinical use of cerebrospinal fluid biomarker testing for Alzheimer’s disease diagnosis: A consensus paper from the Alzheimer’s Biomarkers Standardization Initiative. Alzheimers Dement 10: , 808–817. |
[33] | Kang JH , Korecka M , Figurski MJ , Toledo JB , Blennow K , Zetterberg H , Waligorska T , Brylska M , Fields L , Shah N , Soares H , Dean RA , Vanderstichele H , Petersen RC , Aisen PS , Saykin AJ , Weiner MW , Trojanowski JQ , Shaw LM ((2015) ) The Alzheimer’s Disease Neuroimaging Initiative 2 Biomarker Core: A review of progress and plans. Alzheimers Dement 11: , 772–791. |
[34] | Kang JH , Korecka M , Toledo JB , Trojanowski JQ , Shaw LM ((2013) ) Clinical utility and analytical challenges in measurement of cerebrospinal fluid amyloid-(1-42) and proteins as Alzheimer disease biomarkers. Clin Chem 59: , 903–916. |
[35] | Menéndez-González M ((2014) ) Routine lumbar puncture for the early diagnosis of Alzheimer’s disease. Is it safe? Frontiers Aging Neurosci 6: , 65. |
[36] | Olazaran J , Gil-de-Gomez L , Rodriguez-Martin A , Valenti-Soler M , Frades-Payo B , Marin-Munoz J , Antunez C , Frank-Garcia A , Acedo-Jimenez C , Morlan-Gracia L , Petidier-Torregrossa R , Guisasola MC , Bermejo-Pareja F , Sanchez-Ferro A , Perez-Martinez DA , Manzano-Palomo S , Farquhar R , Rabano A , Calero M ((2015) ) A blood-based,7-metabolite signature for the early diagnosis of Alzheimer’s disease. J Alzheimers Dis 45: , 1157–1173. |
[37] | Wang G , Zhou Y , Huang FJ , Tang HD , Xu XH , Liu JJ , Wang Y , Deng YL , Ren RJ , Xu W , Ma JF , Zhang YN , Zhao AH , Chen SD , Jia W ((2014) ) Plasma metabolite profiles of Alzheimer’s disease and mild cognitive impairment. J Proteome Res 13: , 2649–2658. |
[38] | Voyle N , Baker D , Burnham SC , Covin A , Zhang Z , Sangurdekar DP , Tan Hehir CA , Bazenet C , Lovestone S , Kiddle S , Dobson RJ , AIBL research group ((2015) ) Blood protein markers of neocortical amyloid- burden: A candidate study using SOMAscan technology. J Alzheimers Dis 46: , 947. |
[39] | Apostolova LG , Hwang KS , Avila D , Elashoff D , Kohannim O , Teng E , Sokolow S , Jack CR , Jagust WJ , Shaw L , Trojanowski JQ , Weiner MW , Thompson PM ((2015) ) Brain amyloidosis ascertainment from cognitive, imaging, and peripheral blood protein measures. Neurology 84: , 729–737. |
[40] | O’Bryant SE , Gupta V , Henriksen K , Edwards M , Jeromin A , Lista S , Bazenet C , Soares H , Lovestone S , Hampel H , Montine T , Blennow K , Foroud T , Carrillo M , Graff-Radford N , Laske C , Breteler M , Shaw L , Trojanowski JQ , Schupf N , Rissman RA , Fagan AM , Oberoi P , Umek R , Weiner MW , Grammas P , Posner H , Martins R ((2015) ) Guidelines for the standardization of preanalytic variables for blood-based biomarker studies in Alzheimer’s disease research. Alzheimers Dement 11: , 549–560. |
[41] | Shahim P , Tegner Y , Wilson DH , Randall J , Skillback T , Pazooki D , Kallberg B , Blennow K , Zetterberg H ((2014) ) Blood biomarkers for brain injury in concussed professional ice hockey players. JAMA Neurol 71: , 684–692. |
[42] | Olivera A , Lejbman N , Jeromin A , French LM , Kim HS , Cashion A , Mysliwiec V , Diaz-Arrastia R , Gill J ((2015) ) Peripheral total au in military personnel who sustain traumatic brain injuries during deployment. JAMA Neurol 72: , 1109–1116. |
[43] | Zetterberg H , Wilson D , Andreasson U , Minthon L , Blennow K , Randall J , Hansson O ((2013) ) Plasma tau levels in Alzheimer’s disease. Alzheimers Res Ther 5: , 9. |
[44] | Peskind ER , Kraemer B , Zhang J ((2015) ) Biofluid biomarkers of mild traumatic brain injury: Whither plasma tau. JAMA Neurol 72: , 1103–1105. |
[45] | Properzi F , Logozzi M , Fais S ((2013) ) Exosomes: The future of biomarkers in medicine. Biomark Med 7: , 769–778. |
[46] | Taylor DD , Doellgast GJ ((1979) ) Quantitation of peroxidase-antibody binding to membrane fragments using column chromatography. Anal Biochem 98: , 53–59. |
[47] | Trams EG , Lauter CJ , Salem N Jr , Heine U ((1981) ) Exfoliation of membrane ecto-enzymes in the form of micro-vesicles. Biochim Biophys Acta 645: , 63–70. |
[48] | Taylor DD , Gercel-Taylor C ((2011) ) Exosomes/microvesicles: Mediators of cancer-associated immunosuppressive microenvironments. Semin Immunopathol 33: , 441–454. |
[49] | Guo JL , Lee VM ((2014) ) Cell-to-cell transmission of pathogenic proteins in neurodegenerative diseases. Nat Med 20: , 130–138. |
[50] | Asai H , Tsunoda S , Medalla M , Luebke J , Haydar T , Wolozin B , Butovsky O , Kugler S , Ikezu T ((2015) ) Depletion of microglia and inhibition of exosome synthesis halt tau propagation. Nat Neurosci 18: , 1584–1593. |
[51] | Aryani A , Denecke B ((2014) ) Exosomes as a nanodelivery system: A key to the future of neuromedicine?. Mol Neurobiol, doi: 10.1007/s12035-014-9054-5 |
[52] | Fiandaca MS , Kapogiannis D , Mapstone M , Boxer A , Eitan E , Schwartz JB , Abner EL , Petersen RC , Federoff HJ , Miller BL , Goetzl EJ ((2015) ) Identification of preclinical Alzheimer’s disease by a profile of pathogenic proteins in neurally derived blood exosomes: A case-control study. Alzheimers Dement 11: , 600–607 e601. |
[53] | Goetzl EJ , Boxer A , Schwartz JB , Abner EL , Petersen RC , Miller BL , Kapogiannis D ((2015) ) Altered lysosomal proteins in neural-derived plasma exosomes in preclinical Alzheimer disease. Neurology 85: , 40–47. |
[54] | Stamm JM , Bourlas AP , Baugh CM , Fritts NG , Daneshvar DH , Martin BM , McClean MD , Tripodis Y , Stern RA ((2015) ) Age of first exposure to football and later-life cognitive impairment in former NFL players. Neurology 84: , 1114–1120. |
[55] | Stamm JM , Koerte IK , Muehlmann M , Pasternak O , Bourlas AP , Baugh CM , Giwerc MY , Zhu A , Coleman MJ , Fritts NG , Martin B , Chaisson C , McClean MD , Lin AP , Cantu RC , Tripodis Y , Stern RA , Shenton ME ((2015) ) Age at first exposure to football is associated with altered corpus callosum white matter microstructure in former professional football players. J Neurotrauma 32: , 1768–1776. |
[56] | Crisco JJ , Fiore R , Beckwith JG , Chu JJ , Brolinson PG , Duma S , McAllister TW , Duhaime AC , Greenwald RM ((2010) ) Frequency and locations of head impact exposures in individual collegiate football players. J Athl Train 45: , 549–559. |
[57] | Crisco JJ , Wilcox BJ , Beckwith JG , Chu JJ , Duhaime AC , Rowson S , Duma SM , Maerlender AC , McAllister TW , Greenwald RM ((2011) ) Head impact exposure in collegiate football players. J Biomech 44: , 2673–2678. |
[58] | Stern RA , Daneshvar DH , Baugh CM , Seichepine DR , Montenigro PH , Riley DO , Fritts NG , Stamm JM , Robbins CA , McHale L , Simkin I , Stein TD , Alvarez VE , Goldstein LE , Budson AE , Kowall NW , Nowinski CJ , Cantu RC , McKee AC ((2013) ) Clinical presentation of chronic traumatic encephalopathy. Neurology 81: , 1122–1129. |
[59] | Taylor DD ((2015) ) Isolation and molecular characterization of extracellular vesicles. Methods 87: , 1–2. |
[60] | Taylor DD , Shah S ((2015) ) Methods of isolating extracellular vesicles impact down-stream analyses of their cargoes. Methods 87: , 3–10. |
[61] | Schey KL , Luther JM , Rose KL ((2015) ) Proteomics characterization of exosome cargo. Methods 87: , 75–82. |
[62] | LaValley MP ((2008) ) Logistic regression. Circulation 117: , 2395–2399. |
[63] | Baayen RH , Davidson DJ , Bates DM ((2008) ) Mixed-effects modeling with crossed random effects for subjects and items. J Mem Lang 59: , 390–412. |
[64] | Turner RC , Lucke-Wold BP , Robson MJ , Omalu BI , Petraglia AL , Bailes JE ((2012) ) Repetitive traumatic brain injury and development of chronic traumatic encephalopathy: A potential role for biomarkers in diagnosis, prognosis, and treatment?. Front Neurol 3: , 186. |
[65] | Lubke U , Six J , Villanova M , Boons J , Vandermeeren M , Ceuterick C , Cras P , Martin JJ ((1994) ) Microtubule-associated protein tau epitopes are present in fiber lesions in diverse muscle disorders. Am J Pathol 145: , 175–188. |
[66] | Gu Y , Oyama F , Ihara Y ((1996) ) Tau is widely expressed in rat tissues. J Neurochem 67: , 1235–1244. |
[67] | Lee VM , Goedert M , Trojanowski JQ ((2001) ) Neurodegenerative tauopathies. Annu Rev Neurosci 24: , 1121–1159. |
[68] | Trojanowski JQ , Schuck T , Schmidt ML , Lee VM ((1989) ) Distribution of tau proteins in the normal human central and peripheral nervous system. J Histochem Cytochem 37: , 209–215. |
[69] | Tashiro K , Hasegawa M , Ihara Y , Iwatsubo T ((1997) ) Somatodendritic localization of phosphorylated tau in neonatal and adult rat cerebral cortex. Neuroreport 8: , 2797–2801. |
[70] | Klein C , Kramer EM , Cardine AM , Schraven B , Brandt R , Trotter J ((2002) ) Process outgrowth of oligodendrocytes is promoted by interaction of fyn kinase with the cytoskeletal protein tau. J Neurosci 22: , 698–707. |
[71] | Esteves-Villanueva JO , Trzeciakiewicz H , Loeffler DA , Martic S ((2015) ) Effects of tau domain-specific antibodies and intravenous immunoglobulin on tau aggregation and aggregate degradation. Biochemistry 54: , 293–302. |
[72] | Ren Y , Sahara N ((2013) ) Characteristics of tau oligomers. Front Neurol 4: , 102. |
[73] | Kondo A , Shahpasand K , Mannix R , Qiu J , Moncaster J , Chen CH , Yao Y , Lin YM , Driver JA , Sun Y , Wei S , Luo ML , Albayram O , Huang P , Rotenberg A , Ryo A , Goldstein LE , Pascual-Leone A , McKee AC , Meehan W , Zhou XZ , Lu KP ((2015) ) Antibody against early driver of neurodegeneration cis P-tau blocks brain injury and tauopathy. Nature 523: , 431–436. |
[74] | Schneider P , Hampel H , Buerger K ((2009) ) Biological marker candidates of Alzheimer’s disease in blood, plasma, and serum. CNS Neurosci Ther 15: , 358–374. |
[75] | Wechsler D ((1981) ) Wechsler Adult Intelligence Scale-Revised, Psychological Corporation, San Antonio. |
[76] | Reitan RM ((1992) ) Trail Making Test: Manual for Administration and Scoring, Reitan Neuropsychology Laboratory. |
[77] | Grant D , Berg E ((1981) ) Wisconsin Card Sorting Test, Psychological Assessment Resources, Lutz, FL. |
[78] | Stern RA , Javorsky DJ , Singer EA , Singer NG , Duke LM , Somerville JA , Thompson JA , Kaplan E ((1999) ) Boston Qualitative Scoring System (BQSS) for the Rey-Osterrieth Complex Figure, Psychological Assessment Resources, Odessa, FL. |
[79] | Roth RM , Isquith PK , Gioia GA ((2005) ) BRIEF-A: Behavior Rating Inventory of Executive Function–Adult Version: Professional Manual, Psychological Assessment Resources, Lutz, FL. |
[80] | Stern R , White T ((2003) ) The Neuropsychological Assessment Battery (NAB), Psychological Assessment Resources, Lutz, FL. |
[81] | Hamilton M ((1960) ) A rating scale for depression. J Neurol Neurosurg Psychiatry 23: , 56–61. |
[82] | Beck A , Ward C , Mendelson M , Mock J , Erbaugh J ((1961) ) An inventory for measuring depression. Arch Gen Psychiatry 4: , 561–571. |
[83] | Beck AT , Weissman A , Lester D , Trexler L ((1974) ) The measurement of pessimism: The hopelessness scale. J Consult Clin Psychol 42: , 861–865. |
[84] | Barratt ES ((1965) ) Factor analysis of some psychometric measures of impulsiveness and anxiety. Psychol Rep 16: , 547–554. |
[85] | Buss AH , Durkee A ((1957) ) An inventory for assessing different kinds of hostility. J Consult Psychol 21: , 343. |
Figures and Tables
Fig.1
Unadjusted distribution of plasma exosomal tau between Control and NFL groups.
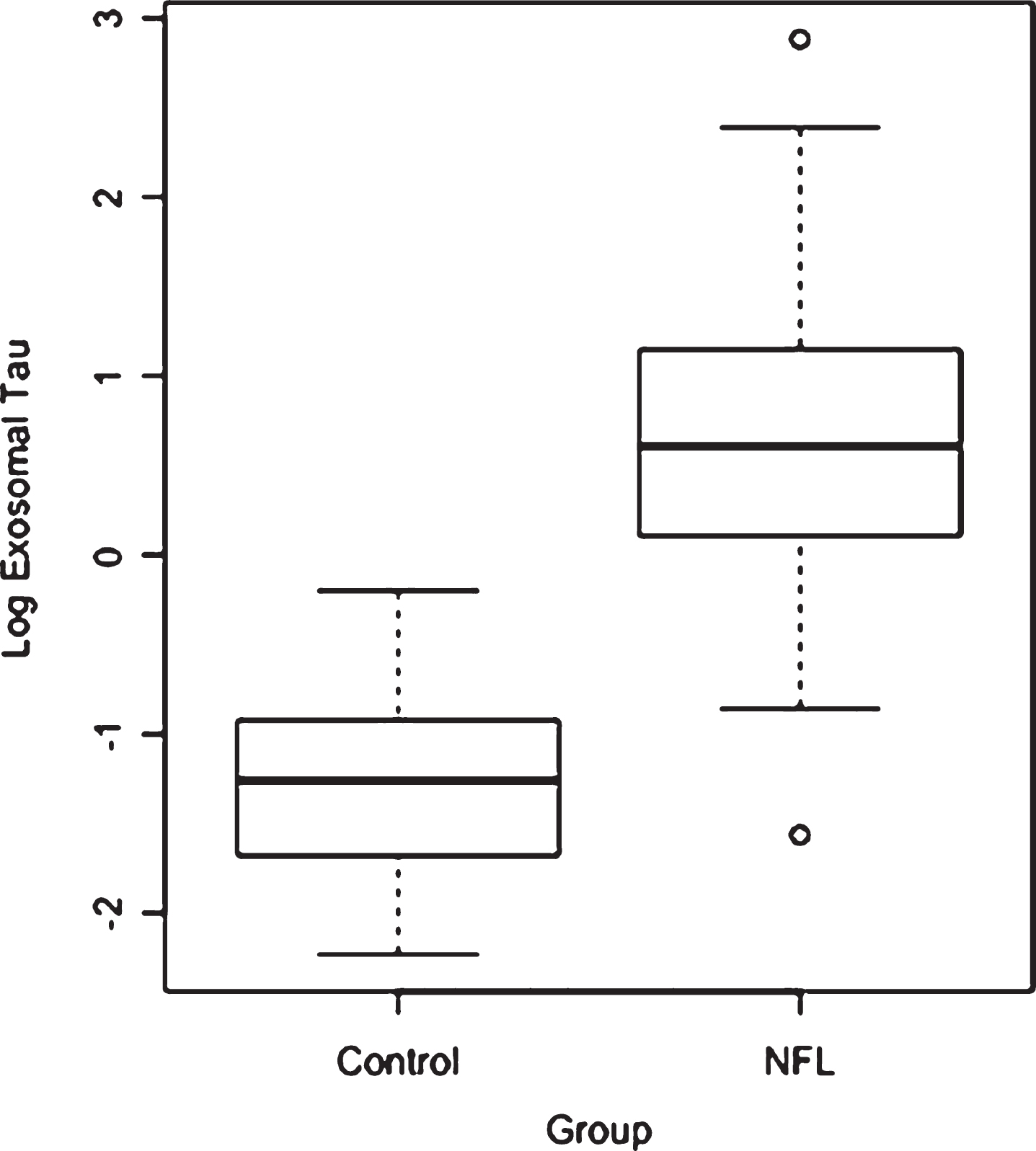
Fig.2
Receiver operating characteristics (ROC) curve with plasma exosomal tau predicting classification between Control and NFL groups. The model successfully discriminated between the groups (c-statistic = 0.97, 95% CI = 0.94–1.00).
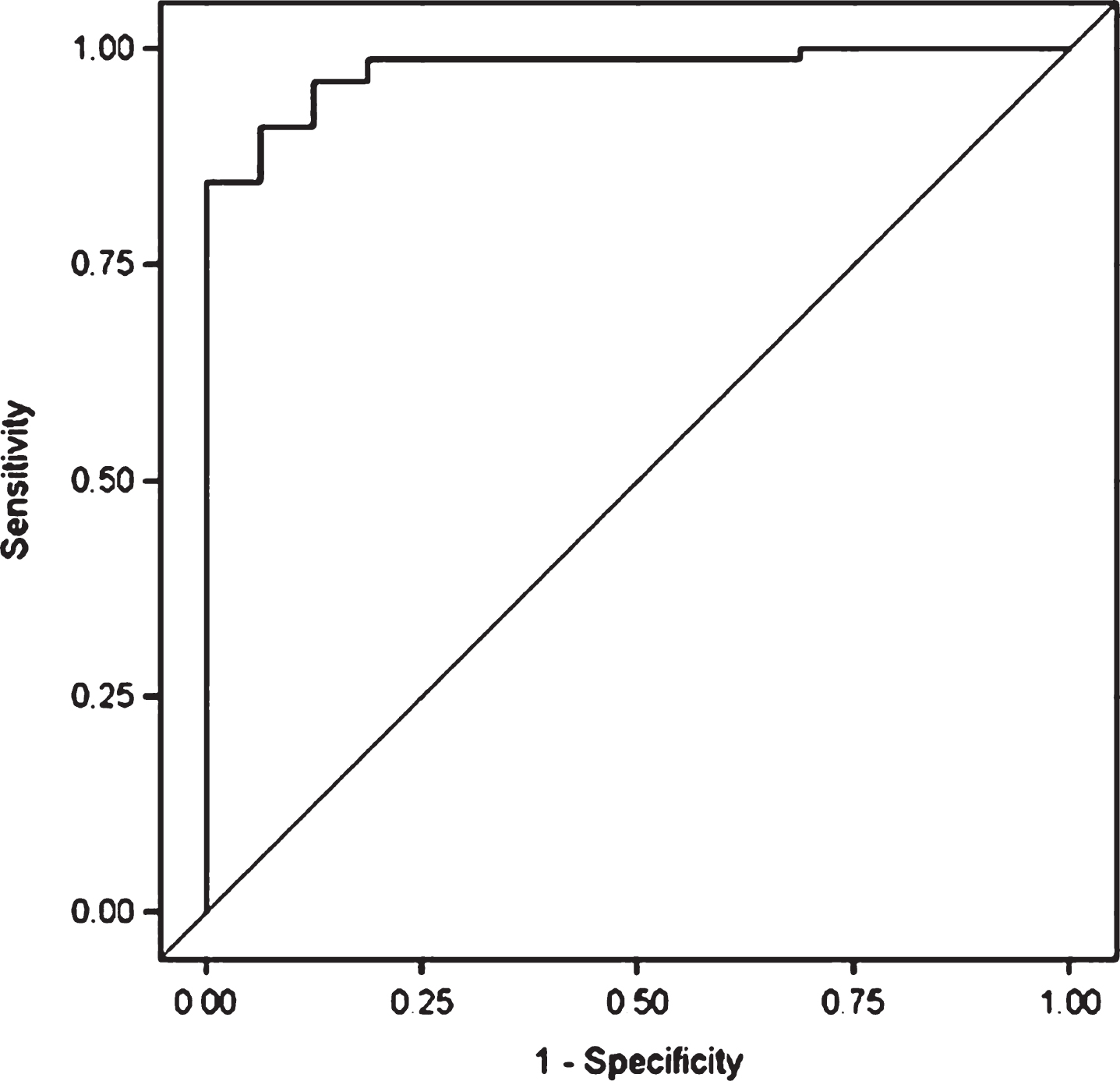
Fig.3
Scatter plot and regression line for the relationship between log exosomal tau and the WAIS-R Digit Symbol raw score for the NFL group (higher Digit Symbol scores reflect better performance).
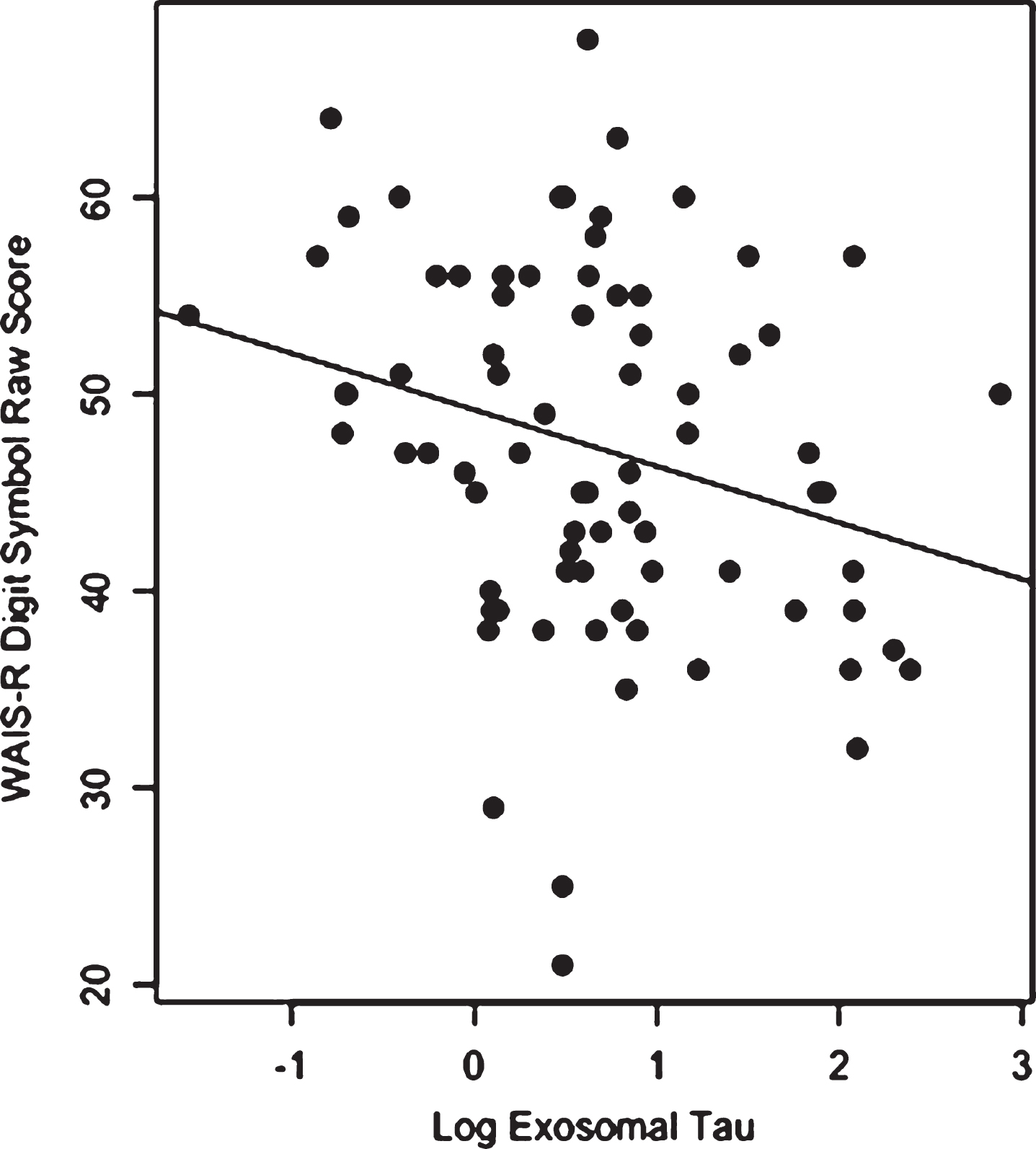
Table 1
Group comparisons on age, education, body mass index, total plasma exosomes, and plasma exosomal tau
Variable | Control Group | NFL Group | t-test | p-value |
(N = 16) | (N = 78) | |||
Age | 56.9 (7.2) | 54.5 (8.0) | 1.12 | 0.2674 |
Education Years | 17.6 (2.0) | 16.4 (0.9) | 2.34 | 0.0320 |
Body Mass Index | 28.2 (3.5) | 32.1 (4.6) | –3.80 | 0.0019 |
Exosomes (per ml) | 6.2 (3.4) | 6.7 (4.3) | –0.49 | 0.6232 |
Exosomal Tau* | –1.3 (0.5) | 0.7 (0.9) | –11.75 | <0.0001 |
*All tau-positive exosome levels (exosomes/ml) are log transformed.
Table 2
Parameter estimates of ANCOVA for exosomal tau
Predictor | Estimate | Standard | t-test | p-value |
Error | ||||
Difference of control minus | –1.93 | 0.24 | –8.05 | <0.0001 |
NFL exosomal tau levels | ||||
Age | 0.01 | 0.01 | 1.20 | 0.2351 |
Body Mass Index | 0.01 | 0.02 | 0.34 | 0.7319 |
Table 3
Relationship between log exosomal tau and measures of cognition, mood, and behavior from mixed effects regression analysis adjusted for age and education as well as for associations of multiple tests per subject and correlations of tests within the same domain for the NFL group only
Domain and Measure | Partial | Beta | Standard | t-test | p-value |
Correlation | Estimate | Error | |||
Psychomotor Speed | |||||
Wechsler Adult Intelligence Scale-Revised [78] Digit Symbol Test, Raw Score | –0.33 | –3.01 | 1.16 | –2.60 | 0.0093 |
Trail Making Test [79] Part A, T-Score | –0.18 | –2.03 | 1.52 | –1.33 | 0.1826 |
Executive Functioning | |||||
Trail Making Test [79] Part B, T-Score | –0.03 | –0.11 | 2.15 | –0.05 | 0.9589 |
Wisconsin Card Sorting Test [80] Percent Errors, T-score | –0.09 | –0.74 | 1.28 | –0.58 | 0.5605 |
Rey-Osterreith Complex Figure –Boston Qualitative Scoring System (BQSS) [81] Organization Score, T-score | 0.09 | 1.69 | 2.06 | 0.82 | 0.4117 |
Behavior Rating Inventory of Executive Function–Adult Version (BRIEF-A) [82] Metacognition Index, T-score | 0.01 | 0.34 | 1.75 | 0.20 | 0.8434 |
Learning and Memory | |||||
BQSS [81] Immediate Presence &Accuracy, T-score | –0.14 | –1.14 | 1.32 | –0.86 | 0.3879 |
BQSS [81] Delayed Presence &Accuracy, T-score | –0.19 | –1.74 | 1.44 | –1.21 | 0.2258 |
Neuropsychological Assessment Battery (NAB) [83] List Learning Test List A Immediate Recall, T-score | –0.31 | –2.84 | 1.14 | –2.50 | 0.0126 |
NAB [83] List Learning Test List A Short Delay Recall, T-score | –0.29 | –3.41 | 1.63 | –2.09 | 0.0365 |
NAB [83] List Learning Test List A Long Delay Recall, T-score | –0.30 | –4.35 | 1.78 | –2.44 | 0.0147 |
Visuospatial Skills | |||||
NAB [83] Map Reading Test, T-score | 0.11 | 1.38 | 1.25 | 1.10 | 0.2709 |
Language | |||||
NAB [83] Naming Test, T-Score | –0.12 | –1.41 | 1.46 | –0.97 | 0.3336 |
Mood | |||||
Hamilton Depression Rating Scale [84], Total Score | 0.16 | 1.43 | 0.99 | 1.43 | 0.1517 |
Beck Depression Inventory [85], Total score | 0.13 | 1.63 | 1.49 | 1.09 | 0.2753 |
Beck Hopelessness Scale [86], Total Score | 0.18 | 0.92 | 0.72 | 1.28 | 0.2024 |
Behavior | |||||
Barratt Impulsivity Scale [87], Total Score | –0.05 | –0.21 | 1.95 | –0.11 | 0.9141 |
Buss-Durkee Hostility Scale [88], Total Score | –0.04 | –0.39 | 1.65 | –0.24 | 0.8121 |
BRIEF-A Behavioral Regulation Index [82], T-score | 0.11 | 1.63 | 1.64 | 1.00 | 0.3187 |