Pathological Markers of Alzheimer’s Disease and Related Dementia in the Rhesus Macaque Amygdala
Abstract
Rhesus macaques develop amyloid-β (Aβ) plaques during old age, but it is unclear how extensively they express other pathological hallmarks of dementia. Here we used immunohistochemistry to examine expression of phosphorylated tau (pTau) protein and cytoplasmic inclusions of TAR DNA binding protein 43 kDa (TDP-43) within the amygdala of young and old males, and also in old surgically-menopausal females that were maintained on regular or obesogenic diets. Only one animal, a 23-year-old female, showed pTau expression and none showed TDP-43 inclusions. What genetic and/or environmental factors protect macaques from expressing more severe human neuro-pathologies remains an interesting unresolved question.
INTRODUCTION
Amyloid-β (Aβ) plaques and hyperphosphorylated tau (pTau) protein represent two pathological hallmarks of Alzheimer’s disease (AD), a primary cause of dementia in humans [1, 2]. Two other major forms of dementia in the elderly, limbic-predominant age-related TDP-43 proteinopathy (LATE-NC) and frontotemporal lobar degeneration (FTLD), involve TAR DNA binding protein 43 kDa (TDP-43). Normally, this protein plays an important role in critical cell functions such as transcriptional repression, pre-mRNA splicing, mRNA transport, microRNA maturation and translational regulation. However, TDP-43 becomes pathological when abnormal amounts or forms of this protein accumulate as cytoplasmic inclusions in frontal and temporal lobe neurons [3–7].
Slow progress in development of therapies for these human dementias stems in part from a lack of animal models that naturally develop similar pathologies. One animal model with potential value is the rhesus macaque (Macaca mulatta). This long-lived primate shows similar brain organization and development to that of humans, and like women, adult female rhesus macaques menstruate and eventually undergo menopause [8–11]. Importantly, unlike humans, rhesus macaques can be maintained under tightly controlled environmental conditions, and tissues can be obtained with essentially no postmortem interval. Such features make the rhesus macaque a desirable animal model in which to study genetic and/or environmental factors that contribute to normal and pathological human aging.
Like humans, rhesus macaques show an age-related increase in Aβ plaque density [12–16], which becomes especially evident within their amygdala after ∼20 years of age [17]. Furthermore, this Aβ plaque density has been shown to be significantly lower in old surgically menopausal (i.e., ovariectomized) females if they receive estradiol hormone replacement therapy (ERT) [17]; a finding that is consistent with the idea that hormone replacement may help to reduce Aβ in postmenopausal women [18]. In contrast, few rhesus macaque studies have focused on pTau [19, 20] or TDP-43 [21], and although these proteins have been detected during old age, they do not appear to be clearly linked to neuronal pathology or dementia. Therefore, the aim of the present study was to examine the time course of neuronal pTau and TDP-43 expression in the rhesus macaque, using brain sections from the same rhesus macaques that were recently used in our Aβ plaque study [17]. Our focus was on the amygdala, a brain area that is involved in learning and memory, is highly susceptible to amyloidosis [14], and shows a high expression of estrogen receptors [22–24]. We hypothesized that the developmental time course of both pTau and TDP-43 pathology could be advanced or delayed by manipulation of diet and/or sex-steroid environment, thereby helping to lay a foundation for novel therapeutic approaches to human dementia.
MATERIALS AND METHODS
Animals
The study was performed on paraformaldehyde-fixed rhesus macaque brains, previously collected from unrelated research projects that were approved by the ONPRC Institutional Animal Care and Use Committee. Details of the animal group compositions are depicted in Table 1. Experiment 1: pTau and TDP-43 expression across age. Experiment 2: effects of ovariectomy (∼48 months) and ERT on pTau and TDP-43 expression; experimental details previously reported in [25]. Experiment 3: effects of ovariectomy (∼30 months) and ERT on pTau and TDP-43 expression in females maintained on a high-fat, high-sugar, Western-style diet (WSD); experimental details previously reported in [26, 27].
Table 1
Composition of Rhesus macaque treatment groups
Treatment | Age (y) | |
EXPERIMENT 1 | Young males (n = 6) | 11.2±1.05 |
Old males (n = 6) | 24.8±0.80*** | |
EXPERIMENT 2 | Old OVX female (n = 7) | 23.3±0.60 |
Old OVX+E females (n = 5) | 25.5±1.20 | |
EXPERIMENT 3 | Old OVX+WSD females (n = 6) | 21.5±0.50 |
Old OVX+E+WSD females (n = 8) | 20.8±0.52 |
Values represent means (±SEMs) and the number of animals per group is indicated in parentheses. OVX, ovariectomized; OVX+E, ovariectomized plus estradiol supplementation in the form of subcutaneous elastomer capsules containing crystalline estradiol that produced circulating estradiol levels similar to those observed during the follicular phase of the menstrual cycle; WSD, Western-style diet, which provided calories from 36% fat, 44% carbohydrates (including 18.5% sugars), and 18% protein; for comparison, regular monkey chow provided calories from 13% fat, 69% complex carbohydrates (including 6% sugars), and 18% protein. ***p< 0.001 (Student’s t-test).
Immunohistochemistry (IHC)
IHC staining for pTau was performed on 30-μm-thick coronal amygdala sections, using mouse antibody AT8 (BioLegend, San Diego, CA, USA) at 1 : 500 dilution, and a standard avidin-biotin-peroxidase procedure (VECTASTAIN ABC kit; Vector Laboratories, Burlingame, CA, USA) with 3,3′-diaminobenzadine tetrahydrochloride (Sigma-Aldrich, St. Louis, MO, USA) as the chromogen. As positive controls, amygdala sections from an AD patient and from a very old macaque (a 29-year-old female Japanese macaque, Macaca fuscata) were also stained for pTau. Immunohistochemical staining for TDP-43 was similarly performed but used rabbit anti-TDP-43 primary antibody (cat# 10782-2-AP, Proteintech, Rosemont, IL, USA) at 1 : 1200 dilution. See Supplementary Material for IHC details.
Imaging and analysis
For each animal and each primary antibody, five sections, spanning the entire amygdala at ∼900-μm intervals, were selected for analysis. Digitized images were examined by an investigator who was blind as to animal treatment and identity, and positively-stained cells that had a neuronal morphology were counted.
Tissue collection and protein extraction for Western blot analysis
Human tissue was obtained from the Oregon Brain Bank and also from the Banner Sun Health Research Institute’s Brain & Body Donation Program. Rhesus macaque tissue was obtained from a 32-year-old female through the ONPRC Tissue Distribution Program. The human control sample came from an 82-year-old man with no dementia and negative for frontotemporal lobe dementia (FTLD) neuropathology. The human FTLD sample came from an 86-year-old woman with a history of dementia and confirmed FTLD neuropathology.
Protein extraction was performed using the subcellular protein fractionation kit for tissues (Thermo Fisher, Waltham, MA, USA), following the manufacturer’s protocol. In brief, 100-200 mg tissue was homogenized using glass Dounce homogenizer in 1-2 ml ice cold CEB buffer. After passing the solution through a provided strainer and centrifuged at 500 g for 5 min, the supernatant was kept as a cytoplasmic fraction (C). The pellet was resuspended in ice-cold MEB buffer and incubated on ice for 10 min. After a 5-min centrifugation at 3000 g, the supernatant was kept as a membrane fraction (M). The pellet was resuspended in ice cold NEB buffer and incubated on ice for 30 min with gentle mixing. After a 5-min centrifugation at 5000 g, the supernatant was kept as a soluble nuclear fraction (SN). The pellet was resuspended in room temperature NEB, containing micrococcal nuclease and CaCl2, and incubated at room temperature for 30 min. After a 5-min centrifugation at 16000 g, the supernatant was kept as a chromatin-bound nuclear fraction (CB). The final pellet was resuspended in room temperature PEB buffer and incubated at room temperature for 10 min. After a 5-min centrifugation at 16000 g, the supernatant was kept as a cytoskeletal fraction (Ck). All buffers contained HALT Protease Inhibitor Cocktail and HALT Phosphatase Inhibitor Cocktail (Thermo Fisher). Each fraction was immediately flash frozen and stored at -80°C. Protein concentration in each fraction was determined using the Micro BCA protein Assay Kit (Thermo Fisher), following the manufacturer’s instructions.
Western blot (TDP-43)
Western blot analysis was used to validate the widely-used clinical TDP-43 antibody for use in rhesus macaques. Protein lysates (10μg) were subjected to SDS-PAGE on 12% Bis-Tris polyacrylamide gels (Thermo Fisher), electro-transferred on nitrocellulose or polyvinylidene difluoride (PVDF) membranes (Thermo Fisher), blocked for 1 h at room temperature with a solution of 5% non-fat dry milk in Tris Buffered Saline with Teen 20 (TBST) and probed overnight at 4°C in the presence of primary antibodies (Supplementary Material) in TBST. Molecular weights were estimated by running 7 ml Seeblue Plus2 pre-stained protein ladder (LC5925, Thermo Fisher). For protein detection, the membranes were incubated in horseradish peroxidase-conjugated secondary antibodies (Thermo Fisher) diluted in TBST for 1 h at room temperature and West Dura chemiluminescence (Thermo Fisher). Chemiluminescence signal was detected and digitalized using a FluorChem System (Bio-Techne, Minneapolis, MN, USA).
RESULTS
Neuronal pTau staining was detected in the amygdala of the AD patient (Fig. 1A), and in the very old macaque (Fig. 1B), thus serving as a positive antibody control. Importantly, out of all the rhesus macaques examined in Experiments 1-3 (i.e., a total of 38 brains), positive pTau immunostaining was detected in the amygdala and adjacent entorhinal cortex of only one animal, an ovariectomized 23-year-old female (Fig. 1C). However, it needs to be emphasized that even in this individual very few positively labeled neurons were detected (mean: 3 per section in the amygdala and 5 per section in the entorhinal cortex), and based on cell morphology there was no obvious staining of glial cells. Interestingly, this same 23-year-old female showed the most extensive staining of extracellular Aβ plaques in the amygdala (Fig. 1D), as previously reported [17].
Fig. 1
Intraneuronal pTau expression in humans and nonhuman primates. A) Representative immuno-positive pTau staining in the amygdala of a control AD patient, and B) a control very old (29 years) female Japanese macaque. C) Representative pTau neuronal staining in the sole experimental rhesus macaque (a 23-year-old ovariectomized female), showing positive staining of a neuron in a pre-tangle state; none of the other 37 animals showed any positive pTau staining in the amygdala. D) Extensive staining of extracellular Aβ plaques in the amygdala of the same 23-year-old ovariectomized female. In summary, pTau pathology in the rhesus macaques was rare and did not approach the severity of that observed in human AD. Scale bars = 50μm for panels A-C, and 100μm for panel D.
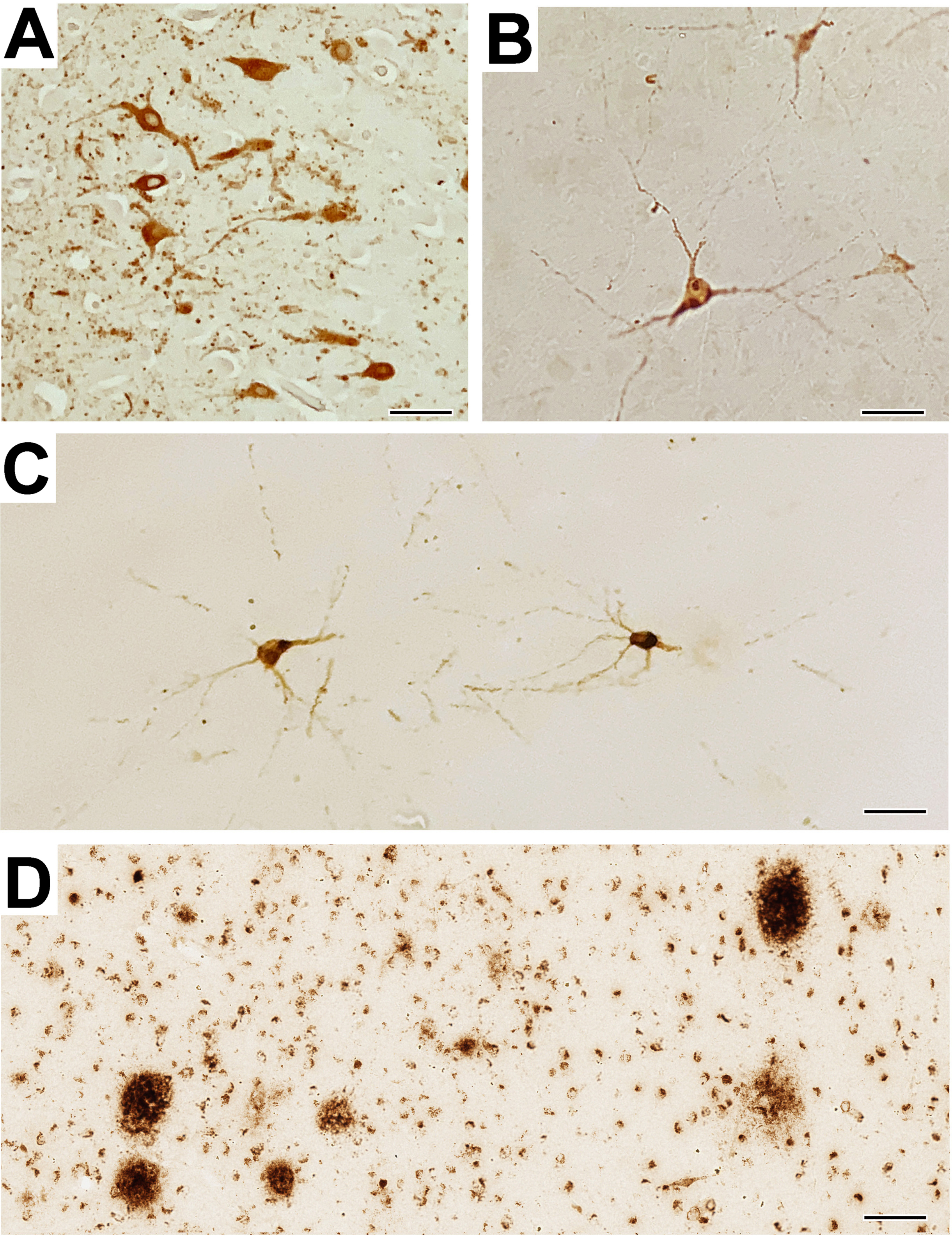
Western blot analysis for TDP-43 confirmed a single band predominantly present in the chromatin bound (CB) and cytoskeletal (Ck) fractions. While the TDP-43 signal in rhesus macaques resembled that of the control human sample, it is worth noting that the FTLD patient showed diminished TDP-43 associated with the CB fraction (Fig. 2A). TDP-43 immunohistochemical staining was detected in the amygdala of the FTLD patient and served as an additional positive control (Fig. 2B). Although TDP-43 was evident in the nucleus and cytoplasm of some cells (i.e., indicative of normal TDP-43 expression), numerous C-shaped cytoplasmic inclusions that are characteristic of advanced FTLD pathology were also present. Positive TDP-43 staining was also clearly detected in the rhesus macaque amygdala, both in the nucleus and in the cytoplasm (Fig. 2C). In contrast to the human FTLD patient; however, no cytoplasmic inclusions were detected in any of the animals, regardless of their age, sex, diet, or hormonal status.
Fig. 2
Intraneuronal TDP-43 expression in humans and nonhuman primates. A) Representative subcellular distribution of TDP43 in the frontal lobe revealed by western blots analysis: Subcellular fractionation of human and monkey frontal lobe shows that TDP43 immunoreactivity is enriched in the nuclear and cytoskeletal fractions. Moreover, while TDP43 is found highly associated with the chromatin bound fraction in the monkey and non-pathological human sample (Control), this association is reduced in a human subject with confirmed FTLD neuropathology. Subcellular fractions are: cytoplasmic (C), membrane (M), soluble nuclear (SN), chromatin bound (CB) and cytoskeletal (Ck). Enrichment of each fraction was confirmed by detecting proteins markers of subcellular compartments. C: Glyceraldehyde 3-phosphate dehydrogenase (GAPDH, MW = 38 KDa), M: Syntaxin (STX1A, MW = 33 KDa), SN: NK2 homeobox 1 (NKX2.1, MW = 42 KDa), CB: Histone H3 (H3, MW = 18 KDa), Ck: Vimentin (Vim = 57KDa). B,C) Immuno-positive TDP-43 staining in the amygdala of a human subject with confirmed FTLD neuropathology and in a representative rhesus macaque, respectively. In the former case, there is reduced staining in cell nuclei compared to the cytoplasm, which shows numerous C-shaped TDP-43 inclusions. In contrast, >95% of the TDP-43 staining in the rhesus macaque is present in both the nucleus and cytoplasm and there are no obvious TDP-43 cytoplasmic inclusions. In summary, none of the rhesus macaques showed TDP-43 pathology approaching the severity of that observed in human FTLD. Scale bars = 50μm for panels B-C, and each inset depicts a 2.5x enlargement.
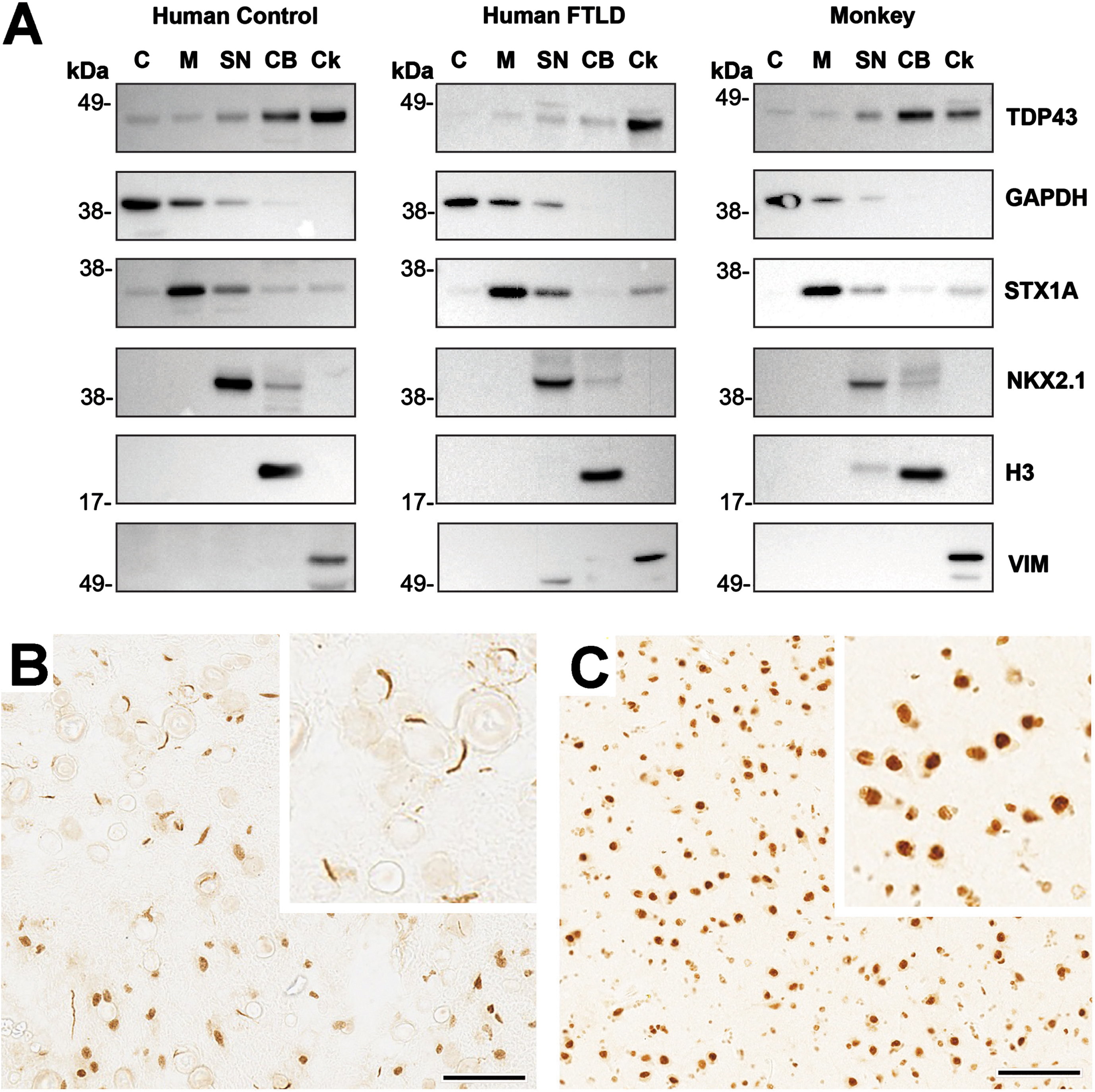
DISCUSSION
Rhesus macaques do not develop full-blown AD, LATE-NC, or FTLD, but like humans they do show an age-related increase in the expression of Aβ plaques in cognitive brain areas [12–17]. They therefore have value as translational animal models in which to examine potential causes of these human dementias. In the case of AD, expression of intraneuronal pTau protein is considered to be a more advanced biomarker of the pathology [1, 2], but its expression in old rhesus macaques has not been widely studied [28, 29] and does not appear to approach the level of severity seen in AD [30]. Similarly, although there appears to be some synergy in the expression of pTau and TDP-43 in human dementia, up to now only one study has examined the expression of TDP-43 protein in the brains of rhesus macaques and it did not find abnormalities resembling those seen in LATE-NC or FTLD [21, 31].
In the present study we sought to examine two environmental factors that may contribute to these between-species differences. Specifically, we hypothesized that maintenance of rhesus macaques on a WSD, instead of their standard monkey chow might advance the timing of pTau and TDP-43 pathology, especially if the animals also had reduced circulating estrogen levels; i.e., because women have a higher risk of developing AD than men [32, 33], one of our goals was to more closely mimic a diet and hormonal status that is typical of postmenopausal women living in the West. We previously examined Aβ plaque density of old rhesus macaques, focusing on the amygdala—an estrogen receptor rich area of the brain [23, 24, 34] that is particularly susceptible to neurodegenerative diseases [35]. Importantly, Aβ plaque density was found to be significantly greater in old ovariectomized rhesus macaques when compared to age-matched ovariectomized animals that had undergone estradiol replacement therapy [17]. In the present study, however, we failed to disclose any advancement of pTau or TDP-43 pathology in the amygdala of these same previously-examined animals. Regardless of their age, sex, diet, or hormonal status, there was no obvious advancement of neuronal pTau expression in the amygdala. Relative to the very old 29-year-old control, only one 23-year-old ovariectomized, untreated animal showed pTau expression, and only in a few neurons; but even these did not show the same degree of pathology as those observed in the amygdala of an AD patient control. Interestingly, this one animal was the same one that showed the most intense Aβ plaque density in our previous study [17], an observation that is consistent with the view that Aβ plaques play a causal role in the eventual development of pTau pathology [1, 2, 36].
TDP-43 pathology typically involves accumulation of hyper-phosphorylated and ubiquinated forms of the molecule in the cytoplasm and its nuclear depletion. Fragmentation of TDP-43 then leads to formation of toxic and aggregate-prone fragments that manifest as cytoplasmic inclusions [5, 31]. However, in none of the rhesus macaques examined were such inclusions detected, regardless of the animal’s sex, age, or hormonal status.
Taken together, the present results confirm that development of pTau in the rhesus macaque brain occurs much later in life than development of Aβ plaques, which already become evident at 20 years of age [12–16], and that cytoplasmic TDP-43 inclusions that are characteristic of LATE-NC and FTLD do not occur naturally during the normal lifespan of rhesus macaques. Moreover, neither of these pathological markers appears to be advanced by attenuating circulating estrogen levels or by maintenance of the animals on a WSD, suggesting that neither of these environmental factors is likely to be a primary cause. In retrospect, the lack of severe pTau or TDP-43 pathology in the amygdala of old rhesus macaques is not altogether surprising. Although previous studies showed some cognitive benefit when the ovariectomized females received estradiol hormone replacement therapy [25, 37], the untreated control animals did not show cognitive deficits approaching the severity associated with human dementia; also, the necropsies were performed several months later after the in vivo studies had been completed. Similarly, in the previous in vivo study involving young and old males [38], there was no obvious age-associated impairment of cognitive function. Taken together, these results emphasize that rhesus macaques do not develop dementia during their normal life span, nor do they express severe forms of the brain pathology that characterizes AD, LATE-NC or FTLD in humans. Additional studies would be necessary to elucidate the exact reasons for why old rhesus macaques do not develop dementia, despite their relatively long lifespan. However, because macaques can be maintained under tightly controlled environmental conditions, they could serve as valuable experimental models in which to investigate factors that contribute to development of the underlying pathologies (e.g., genetics, epigenetics, diet, hormonal environment, environmental toxins, etc.) and in which to test potential therapeutic interventions [11, 39–41].
ACKNOWLEDGMENTS
We would like to thank Dr. Randy Woltjer of the Oregon Brain Bank at OHSU (Portland, OR, USA) for providing human brain sections for use in immunohistochemistry, and Dr. Thomas Beach from the Banner Sun Health Research Institute Brain & Body Donation Program (Sun City, AZ, USA) for providing frozen human biological materials for use in Western blot analysis.
FUNDING
This work was supported by National Institutes of Health Grants: P30 AG066518, P51 OD011092, R24 OD011895, RF1 AG062220, and S10 OD025002.
CONFLICT OF INTEREST
The authors declare that the research was conducted in the absence of any commercial or financial relationships that could be construed as a potential conflict of interest.
DATA AVAILABILITY
All data generated during this study are included in this article or are available on reasonable request.
SUPPLEMENTARY MATERIAL
[1] The supplementary material is available in the electronic version of this article: https://dx.doi.org/10.3233/ADR-230184.
REFERENCES
[1] | Busche MA , Hyman BT , ((2020) ) Synergy between amyloid-β and tau in Alzheimer’s disease. Nat Neurosci 23: , 1183–1193. |
[2] | Jack CR Jr ((2022) ) Advances in Alzheimer’s disease research over thepast two decades. Neurol 21: , 866–869. |
[3] | de Boer EM , Orie VK , Williams T , Baker MR , De Oliveira HM , Polvikoski T , Silsby M , Menon P , van den Bos M , Halliday GM , van denBerg LH , Van Den Bosch L , van Damme P , Kiernan MC , van Es MA , Vucic S ((2020) ) TDP-43 proteinopathies: A new wave of neurodegenerativediseases. J Neurol Neurosurg Psychiatry 92: , 86–95. |
[4] | Sephton CF , Cenik C , Kucukural A , Dammer EB , Cenik B , Han Y , Dewey CM , Roth FP , Herz J , Peng J , Moore MJ , Yu G ((2011) ) Identification ofneuronal RNA targets of TDP-43-containing ribonucleoproteincomplexes. J Biol Chem 286: , 1204–1215. |
[5] | Nelson PT , Lee EB , Cykowski MD , Alafuzoff I , Arfanakis K , Attems J. et al. ((2023) ) LATE-NC staging in routine neuropathologic diagnosis:An update. Acta Neuropathol 145: , 159–173. |
[6] | Neumann M , Perneel J , Cheung S , Van den Broeck M , Nygaard H , Hsiung GR , Wynants S , Heeman B , Rademakers R , Mackenzie IRA ((2023) ) Limbic-predominant age-related TDP-43 proteinopathy (LATE-NC) isassociated with abundant TMEM106B pathology. Acta Neuropathol 146: , 163–166. |
[7] | Nilaver BI , Urbanski HF ((2023) ) Mechanisms underlying TDP-43pathology and neurodegeneration: An updated mini-review. FrontAging Neurosci 15: , 1142617. |
[8] | Downs JL , Urbanski HF ((2006) ) Neuroendocrine changes in the agingreproductive axis of female rhesus macaques (Macacaca mulatta). Biol Reprod 75: , 539–546. |
[9] | Messaoudi I , Urbanski HF , Kohama SG (2011) Integrative models of aging in the nonhuman primate. In Monkeys: Biology, Behavior and Disorders, Williams RM, ed. Nova Science Publishers, New York, pp. 1–54. |
[10] | Luna SL , Brown DI , Kohama SG , Urbanski HF ((2020) ) Lack of effect ofshort-term DHEA supplementation on the perimenopausal ovary. Biol Reprod 103: , 1209–1216. |
[11] | Stonebarger GA , Bimonte-Nelson HA , Urbanski HF ((2021) ) The rhesusmacaque as a translational model for neurodegeneration andAlzheimer’s disease. Front Aging Neurosci 13: , 734173. |
[12] | Heilbroner PL , Kemper TL ((1990) ) The cytoarchitectonic distributionof senile plaques in three aged monkeys. Acta Neuropathol 81: , 60–65. |
[13] | Martin LJ , Sisodia SS , Koo EH , Cork LC , Dellovade TL , Weidemann A , Beyreuther K , Masters C , Price DL ((1991) ) Amyloid precursor protein in aged nonhuman primates. Proc Natl Acad Sci U S A 88: , 1461–1465. |
[14] | Uno H , Walker LC ((1993) ) The age of biosenescence and the incidence of cerebral beta-amyloidosis in aged captive rhesus monkeys. Ann NY Acad Sci 695: , 232–235. |
[15] | Sloane JA , Pietropaolo MF , Rosene DL , Moss MB , Peters A , Kemper T , Abraham CR ((1997) ) Lack of correlation between plaque burden and cognition in the aged monkey. Acta Neuropathol 94: , 471–478. |
[16] | Stonebarger GA , Urbanski HF , Woltjer RL , Vaughan KL , Ingram DK , Schultz PL , Calderazzo SM , Siedeman JA , Mattison JA , Rosene DL , Kohama SG ((2020) ) Amyloidosis increase is not attenuated by long-termcalorie restriction or related to neuron density in the prefrontalcortex of extremely aged rhesus macaques. Geroscience 42: , 1733–1749. |
[17] | Appleman ML, Thomas JL, Weiss AR, Nilaver BI, Cervera-Juanes R, Kohama SG, Urbanski HF ((2023) ) Effect of hormone replacement therapy on amyloid beta (Aβ) plaque density in the rhesus macaque amygdala. Front Aging Neurosci 15: , 1326747. |
[18] | German-Castelan L , Shanks HRC , Gros R , Saito T , Saido TC , Saksida LM , Bussey TJ , Prado MAM , Schmitz TW , Prado VF ; Australian Imaging Biomarkers and Lifestyle flagship study of ageing (2023) Sex-dependent cholinergic effects on amyloid pathology: A translational study. Alzheimers Dement, doi: 10.1002/alz.13481. |
[19] | Paspalas CD , Carlyle BC , Leslie S , Preuss TM , Crimins JL , Huttner AJ , van Dyck CH , Rosene DL , Nairn AC , Arnsten AFT ((2018) ) The agedrhesus macaque manifests Braak stage III/IV Alzheimer’s-likepathology. Alzheimers Dement 14: , 680–691. |
[20] | Arnsten AFT , Datta D , Leslie S , Yang S-T , Wang M , Nairn AC ((2019) ) Alzheimer’s-like pathology in aging rhesus macaques: Uniqueopportunity to study the etiology and treatment of Alzheimer’sdisease. Proc Soc Natl Acad Sci U S A 116: , 26230–26238. |
[21] | Darricau M , Canron MH , Bosc M , Arotcarena ML , Quang ML , Dehay B , Bezard E , Planche V ((2021) ) Lack of limbic-predominant age-relatedTDP-43 encephalopathy (LATE) neuropathological changes in agedmacaques with memory impairment. Neurobiol Aging 107: , 53–56. |
[22] | Shughrue PJ , Lane MV , Merchenthaler I ((1997) ) Comparativedistribution of estrogen receptor-alpha and -beta mRNA in the ratcentral nervous system. J Comp Neurol 388: , 507–525. |
[23] | Pau CY , Pau KY , Spies HG ((1998) ) Putative estrogen receptor beta andalpha mRNA expression in male and female rhesus macaques. MolCell Endocrinol 146: , 59–68. |
[24] | Gundlah C , Kohama SG , Mirkes SJ , Garyfallou VT , Urbanski HF , Bethea CL ((2000) ) Distribution of estrogen receptor beta (ERβ) mRNA in hypothalamus, midbrain and temporal lobe of spayed macaque: Continued expression with hormone replacement. Mol Brain Res 76: , 191–204. |
[25] | Kohama SG , Renner L , Landauer N , Weiss AR , Urbanski HF , Park B , Voytko ML , Neuringer M ((2016) ) Effect of ovarian hormone therapy oncognition in the aged female rhesus macaque. J Neurosci 36: , 10416–10424. |
[26] | Purnell JQ , Urbanski HF , Kievit P , Roberts CT , Bethea CL ((2019) ) Estradiol replacement timing and obesogenic diet effects on bodycomposition and metabolism in postmenopausal macaques. Endocrinology 160: , 899–914. |
[27] | Urbanski HF , Mueller K , Bethea CL ((2017) ) Effect of an obesogenicdiet on circadian activity and serum hormones in old monkeys. Endocr Connect 6: , 380–383. |
[28] | Arnsten AFT , Datta D , Preuss TM ((2021) ) Studies of aging nonhuman primates illuminate the etiology of early-stage Alzheimer’s-like neuropathology: An evolutionary perspective. , e. Am J Primatol 83: , 23254. |
[29] | Datta D , Leslie SN , Wang M , Morozo YM , Yang S , Mentone S , Zeiss C , Duque A , Rakic P , Horvath TL , van Dyck CH , Nairn AC , Arnsten AFT ((2021) ) Age-related calcium dysregulation linked with tau pathologyand impaired cognition in non-human primates. AlzheimersDement 17: , 920–932. |
[30] | Braak H , Alafuzoff I , Arzberger T , Kretzschmar H , Del Tredici K ((2006) ) Staging of Alzheimer disease-associated neurofibrillarypathology using paraffin sections and immunocytochemistry. ActaNeuropathol 112: , 389–404. |
[31] | Young AL , Vogel JW , Robinson JL , McMillan CT , Ossenkoppele R , Wolk DA , Irwin DJ , Elman L , Grossman M , Lee VMY , Lee EB , Hansson O ((2023) ) Data-driven neuropathological staging and subtyping of TDP-43proteinopathies. Brain 146: , 2975–2988. |
[32] | Ferretti MT , Iulita MF , Cavedo E , Chiesa PA , Schumacher Dimech A , Santuccione Chadha A , Baracchi F , Girouard H , Misoch S , Giacobini E , Depypere H , Hampel H ; Women’s Brain Project and the AlzheimerPrecision Medicine Initiative ((2018) ) Sex differences in Alzheimerdisease - the gateway to precision medicine. Nat Rev Neurol 14: , 457–469. |
[33] | Zhu D , Montagne A , Zhao Z ((2021) ) Alzheimer’s pathogenic mechanisms and underlying sex difference. Cell Mol Sci 78: , 4907–4920. |
[34] | Ostlund H , Keller E , Hurd YL ((2003) ) Estrogen receptor gene expression in relation to neuropsychiatric disorders. Ann NY Acad Sci 1007: , 54–63. |
[35] | Nelson PT , Abner EL , Patel E , Anderson S , Wilcock DM , Kryscio RJ , Van Eldik LJ , Jicha GA , Gal Z , Nelson RS , Nelson BG , Gal J , Azam MT , Fardo DW , Cykowski MD ((2018) ) The amygdala as a locus of pathologicmisfolding in neurodegenerative diseases. J. Neuropathol ExpNeurol 77: , 2–20. |
[36] | Crary JF ((2016) ) Primary age-related tauopathy and the amyloid cascadehypothesis: The exception that proves the rule? J NeurolNeuromedicine 1: , 53–57. |
[37] | Zimmerman B , Kundu P , Liu Z , Urbanski HF , Kroenke CD , Kohama SG , Bethea CL , Raber J ((2020) ) Longitudinal effects of immediate anddelayed estradiol on cognitive performance in a spatial maze andhippocampal volume in menopausal macaques under an obesogenic diet. Front Neurol 11: , 539. |
[38] | Weiss A , Urbanski HF ((2023) ) Effect of short-term androgensupplementation on cognitive performance in older male rhesusmacaques. Neurobiol Aging 132: , 246–249. |
[39] | Latimer CS , Shively CA , Keene CD , Jorgensen MJ , Andrews RN , Register TC , Montine TJ , Wilson AM , Neth BJ , Mintz A , Maldjian JA , Whitlow CT , Kaplan JR , Craft S ((2019) ) A nonhuman primate model of earlyAlzheimer’s disease pathologic change: Implications for diseasepathogenesis. Alzheimers Dement 15: , 93–105. |
[40] | Beckman D , Chakrabarty P , Ott S , Dao A , Zhou E , Janssen WG , Donis-Cox K , Muller S , Kordower JH , Morrison JH ((2021) ) A noveltau-based rhesus monkey model of Alzheimer’s pathogenesis. Alzheimers Dement 17: , 933–945. |
[41] | Beckman D , Morrison JH ((2021) ) Towards developing a rhesus monkey modelof early Alzheimer’s disease focusing on women’s health. Am JPrimatol 83: , e23289. |