Seasonal Variations in Vitamin D Levels and the Incident Dementia Among Older Adults Aged ≥60 Years in the UK Biobank1
Abstract
Background:
Limited knowledge exists regarding the association between dementia incidence and vitamin D insufficiency/deficiency across seasons.
Objective:
This study aimed to evaluate the impact of seasonal serum vitamin D (25(OH)D) levels on dementia and its subtypes, considering potential modifiers.
Methods:
We analyzed 193,003 individuals aged 60–73 at baseline (2006–2010) from the UK Biobank cohort, with follow-up until 2018. 25(OH)D were measured at baseline, and incident dementia cases were identified through hospital records, death certificates, and self-reports.
Results:
Out of 1,874 documented all-cause dementia cases, the median follow-up duration was 8.9 years. Linear and nonlinear associations between 25(OH)D and dementia incidence across seasons were observed. In multivariable-adjusted analysis, 25(OH)D deficiency was associated with a 1.5-fold (95% CIs: 1.2–2.0), 2.2-fold (1.5–3.0), 2.0-fold (1.5–2.7), and 1.7-fold (1.3–2.3) increased incidence of all-cause dementia in spring, summer, autumn, and winter, respectively. Adjusting for seasonal variations, 25(OH)D insufficiency and deficiency were associated with a 1.3-fold (1.1–1.4) and 1.8-fold (1.6–2.2) increased dementia incidence, respectively. This association remained significant across subgroups, including baseline age, gender, and education levels. Furthermore, 25(OH)D deficiency was associated with a 1.4-fold (1.1–1.8) and 1.5-fold (1.1–2.0) higher incidence of Alzheimer’s disease and vascular dementia, respectively. These associations remained significant across all subgroups.
Conclusions:
25(OH)D deficiency is associated with an increased incidence of dementia and its subtypes throughout the year.
INTRODUCTION
Dementia is a neurocognitive disorder that is associated with a significant functional deficit in language, behavior, and cognition [1, 2]. Globally, this disorder presents a formidable challenge, affecting an estimated 55 million individuals and adding nearly 10 million new cases annually [3]. In 2019, dementia was attributed to 12.5% of all deaths in England and Wales [4]. Financially, the worldwide cost of dementia surged from $818 billion in 2015 to approximately $1.3 trillion by 2019 [3]. These figures underscore the substantial burden that dementia imposes on global healthcare systems. Importantly, cognitive decline may commence years prior to the manifestation of clinical symptoms [5], offering a potential window for preventive interventions. Given the absence of effective treatments to halt the progression of dementia, identifying key modifiable risk factors remains a critical imperative.
Recently, as there is no established treatment to halt the progression of dementia, understanding modifiable factors like dietary intake becomes crucial for both prevention and insight into its pathogenesis. There has been an increasing number of studies investigating the potential role of vitamin D in the development of dementia however, the findings have been inconsistent so far [6–9]. A 30-year prospective cohort study of 10,186 Danish people found a relationship between low serum vitamin D levels and an elevated incidence of Alzheimer’s disease (AD), yet not the development of vascular dementia [10]. Data from the Three-City Bordeaux cohort of 916 French adults aged ≥65 demonstrated that vitamin D at baseline was associated with an increased risk of incident dementia over 12 years [11]. Conversely, the Rotterdam Study of 6,087 adults aged ≥55 years demonstrated no significant association between the incidence of dementia and serum vitamin D deficiency [12]. Amid strong evidence for seasonal fluctuations in vitamin D exposure, multivariable models were adjusted for the season of serum collection in only a handful of studies [10–12]. Given the association of vitamin D levels with recognized dementia risk factors, including stroke, hypertension, cardiovascular disease, obesity, diabetes, and depression, which are key contributors to the development of dementia [7, 13–18], it is of great importance to investigate the interaction of vitamin D and dementia risk factors with the incidence of dementia.
Using the UK Biobank, we sought to identify cut-off points for vitamin D insufficiency and deficiency across seasons that were associated with incident dementia. We also aimed to examine whether the association between vitamin D insufficiency and deficiency and incident dementia differed across subgroups of important dementia risk factors and whether the association was mediated by newly developed chronic conditions.
METHODS
Study population
This study utilized the UKB cohort study data, which included >500,000 United Kingdom (UK) population ranging in age from 40 to 73 years old at baseline. Participants were recruited from the UK during the period spanning from 2006 to 2010 [19]. The design and population details have been explained elsewhere [19]. A total of 502,505 subjects, whose ages ranged from 40 to 73 years, were recruited at the baseline. Individuals with aged ≥60 years were screened for analysis due to the higher prevalence of dementia within this specific age group. This UKB Study was ethically approved. During the recruitment phase, all individuals provided informed consent via electronic signatures. The current investigation was carried out under the UKB resource application number 62443.
The UK Biobank Study’s ethical approval had been granted by the National Information Governance Board for Health and Social Care and the NHS North West Multicenter Research Ethics Committee. All participants provided informed consent through electronic signature at recruitment.
Vitamin D
Serum 25-hydroxyvitamin D (25(OH)D), a proxy for vitamin D status, was used as the indicator of vitamin D levels and measured using a chemiluminescence immunoassay (DiaSorin Liaison XL, DiaSorin Ltd., UK) between 2006 and 2010. The assay for 25OHD had an analytical sensitivity (lower detection limit) of 10 nmol/L representing the lowest measurable analyte level that can be distinguished from zero. Quality control was conducted by the UK Biobank central team. The average coefficients of variation of 25OHD derived from internal quality control samples ranged from 5.0% to 6.1%. External quality assurance results showed that 100% of participated distributions (n = 108) were acceptable (assurance scheme registration: RIQAS Immunoassay Specialty 1).
Ascertainment of incident dementia
All-cause dementia cases in the UK Biobank Study were ascertained using hospital inpatient records, death registers, and self-reported data. Data on admissions and diagnoses were used to identify dementia cases with a primary/secondary diagnosis using the international classification diseases (ICD) coding system (detailed in Supplementary Table 1). Additional cases were defined as underlying/contributory cause of death being dementia through linkage to death register data. Dementia was also defined by reporting a diagnosis of dementia during follow-up. The earliest recorded date regardless of sources was used as the onset date of dementia. Person-years were calculated from the date of baseline assessment to the date of onset dementia, date of death, or the end of follow-up (1 March 2018 for England and Wales and 1 March 2017 for Scotland), whichever came first.
Covariates
Age (years), sex (females, males), ethnicity (Whites, non-whites), education (0–5 years, 6–12 years,≥13 years), and income (<18,000£, 18,000–30,999£, 31,000–51,999£, 52,000–100,000£, >100,000£) were self-reported. Sleep duration was assessed with the survey item “About how many hours sleep do you get in every 24 h?”. Questions about walking, moderate physical activity, and vigorous physical activity, which were similar to those used in the short form of the International Physical Activity Questionnaire [20], were used to estimate excess metabolic equivalent (MET)-hours/week of physical activity during work and leisure time. A healthy diet score was computed based on seven commonly eaten food groups following recommendations on dietary priorities for cardiometabolic health [21] with a higher score representing a healthier diet. A higher healthy diet score has been shown to be associated with a lower risk of dementia [22].
Systemic conditions at baseline were based on self-reported data or interviews. Participants were asked whether they had ever been told by a doctor that they had certain common medical conditions, including heart attack, angina, stroke, hypertension, and diabetes. Depression was recorded during the interview with a research nurse. Newly developed systemic conditions during follow-up (before the onset of dementia) were identified using hospital inpatient records and self-reported data (Supplementary Table 1). Body mass index (BMI) was computed based on the weight in kilograms divided by height in meters, and obesity was defined as BMI≥30 kg/m2 [23].
Statistical analysis
Data of baseline characteristics were expressed as frequency (percentage) and means±standard deviations (SDs). To examine the seasonal effect on the 25(OH)D, the regression splines method was used to smooth the relationship between 25(OH)D and the day of the year when the serum was collected (0–365). Hazard ratio curves for 25(OH)D with incident dementia were fitted using the Cox regression model with penalized spline smoothing adjusted for age, gender, and the day of the year when serum was collected [24]. This analysis was conducted for summer, autumn, winter, and spring separately. Both linear and non-linear relationships were tested and cubic regression splines with four knots were examined. The cut-off points for the two knots with lower 25(OH)D levels adjusted for age, gender, and the day of the year when the serum was collected were used to define insufficiency and deficiency for each season.
Cox proportional hazard regression models were used to examine the association of 25(OH)D insufficiency and deficiency with incident dementia. The following models were tested: 1) age and gender; 2) model 1 plus the day of the year when serum was collected, ethnicity, education, income, diet score, vitamin D supplement, smoking, alcohol consumption, sleep, physical activity, BMI, cholesterol, glycosylated hemoglobin, cystatin C, depression, hypertension, diabetes, heart disease, and chronic kidney disease at baseline. We then analyzed the association of 25(OH)D insufficiency and deficiency with incident dementia stratified by important factors such as age, gender, education, physical activity, diet score, smoking, depression, hypertension, diabetes, heart disease, and chronic kidney disease.
The potential mediation effects of newly developed systemic conditions during follow-up (before onset dementia) on the association between lower levels of 25(OH)D (insufficiency and deficiency combined) and incident dementia were estimated using Cox proportional hazards regression models [25].
We also examined the association between quintiles of 25(OH)D by month when serum was collected and incident dementia. The association of 25(OH)D insufficiency (25–49.9 nmol/L) and deficiency (≥50 nmol/L) defined by non-season specific cut-off points with incident dementia was then analyzed.
In further analysis, we examined association of 25(OH)D insufficiency (25–49.9 nmol/L) and deficiency (≥50 nmol/L) with incident dementia subtypes, including AD and vascular dementia.
Data analyses were conducted using SAS 9.4 for Windows (SAS Institute Inc.) and all p values were two-sided with statistical significance set at <0.05.
RESULTS
Population selection and baseline characteristics
Baseline data were collected among 502,505 participants. After excluding individuals with missing data on 25(OH)D (n = 54,155), with age younger than 60 years (n = 255,213), or with prevalent dementia (n = 134), 193,003 adults (51.3% females) aged 60–73 years (mean±SD: 64.2±2.9) were included in the final analysis. Women were more likely to have low income and education, lower levels of physical activity and higher diet quality than men. Men had higher BMI, cystatin C, and glycosylated hemoglobin than women. Men had a higher prevalence of diabetes, stroke, heart disease, and depression than women (all p-values<0.05, Table 1).
Table 1
Baseline characteristics of participants according to 25(OH)D status
Women (n = 98,984) | Men (n = 94,019) | p | |
Age (y) | 64.0±2.9 | 64.3±2.9 | <0.0001 |
Ethnicity | 0.0974 | ||
Whites | 95,757 (96.7) | 90,823 (96.6) | |
Non-whites | 3227 (3.3) | 3196 (3.4) | |
Education | <0.0001 | ||
0–5 y | 23,060 (23.3) | 27,084 (28.8) | |
6–12 y | 47,036 (47.5) | 41,293 (43.9) | |
≥13 y | 27,471 (27.8) | 24,263 (25.8) | |
Missing | 1,417 (1.4) | 1,379 (1.5) | |
Household income (£) | <0.0001 | ||
<18,000 | 29,050 (29.3) | 24,182 (25.7) | |
18,000–30,999 | 25,130 (25.4) | 25,934 (27.6) | |
31,000–51,999 | 13,885 (14.0) | 18,851 (20.1) | |
52,000–100,000 | 5,844 (5.9) | 10,100 (10.7) | |
>100,000 | 1,333 (1.3) | 2,467 (2.6) | |
Unknown | 8,367 (8.5) | 2,373 (2.5) | |
Not answered | 1,537 (15.5) | 10,112 (10.8) | |
Physical activity (MET-min/week) | 2,700±2,200 | 2,800±2,600 | <0.0001 |
Diet score | 4.3±1.3 | 3.6±1.4 | <0.0001 |
Vitamin D supplementation | 3,080 (3.1) | 1,931 (2.1) | <0.0001 |
25(OH)D (nmol/L) | |||
Spring | 45.6±20.2 | 45.3±19.5 | 0.0712 |
Summer | 56.8±19.1 | 60.4±20.0 | <0.0001 |
Autumn | 53.9±19.8 | 56.1±20.4 | <0.0001 |
Winter | 44.2±19.8 | 42.9±19.0 | <0.0001 |
Combined | 50.4±20.4 | 51.4±21.0 | <0.0001 |
Sleep duration (h) | 7.2±1.2 | 7.3±1.1 | <0.0001 |
Alcohol consumption | <0.0001 | ||
Never | 6,746 (6.8) | 2,407 (2.6) | |
Previous | 4,018 (4.1) | 3,333 (3.5) | |
Current | 88,011 (88.9) | 88,085 (93.7) | |
Missing | 209 (0.2) | 194 (0.2) | |
Smoking | <0.0001 | ||
Never | 56,414 (57.0) | 39,070 (41.6) | |
Former | 35,442 (35.8) | 45,169 (48.0) | |
Current | 6,562 (6.6) | 9,192 (9.8) | |
Missing | 566 (0.6) | 588 (0.6) | |
BMI (kg/m2) | 27.4±4.9 | 27.86±4.0 | <0.0001 |
Cholesterol (mmol/L) | 5.9±1.0 | 5.26±1.1 | <0.0001 |
Glycosylated hemoglobin (mmol/mol) | 37.1±5.9 | 37.5±7.5 | <0.0001 |
Cystatin C (mg/L) | 0.9±0.2 | 1.0±0.2 | <0.0001 |
Diabetes | 4,236 (4.3) | 7,713 (8.2) | <0.0001 |
Stroke | 1,775 (1.8) | 2,845 (3.0) | <0.0001 |
Heart disease | 4,318 (4.4) | 10,765 (11.4) | <0.0001 |
Hypertension | 33,629 (34.0) | 37,192 (39.6) | <0.0001 |
Depression | 5,518 (5.6) | 3,339 (3.6) | <0.0001 |
Kidney failure | 52 (0.1) | 63 (0.1) | 0.19 |
Kidney stone | 505 (0.5) | 1312 (1.4) | 0.0449 |
Data are mean (standard deviation), or N (%). BMI, body mass index; MET, metabolic equivalent. *T-test was used to test the difference of continuous variables across subgroups of 25(OH)D status and Chi-square for categorical variables.
Incidence of dementia
Over 1,721,817 person-years of follow-up (median [interquartile range] length of follow-up: 8.9 [8.3–9.5] years), 1874 cases of incident all-cause dementia were documented.
25(OH)D by seasons
The proportion of blood sampling for spring, summer, autumn, and winter was 28.9%, 26.9%, 24.1%, and 20.1%, respectively. There was significant variation across seasons with the mean 25(OH)D for spring, summer, autumn, and winter as 45.4 nmol/L, 58.6 nmol/L, 54.9 nmol/L, and 43.5 nmol/L, respectively. Similar trends were found in women and men but higher 25(OH)D in summer and autumn and lower 25(OH)D in winter and early spring was observed in men than women (Fig. 1).
Fig. 1
Nonparametric smoothing plot with 95% confidence interval of fitted 25(OH)D against day of year. The day of year began from the first date of summer (1 June in United Kingdom).
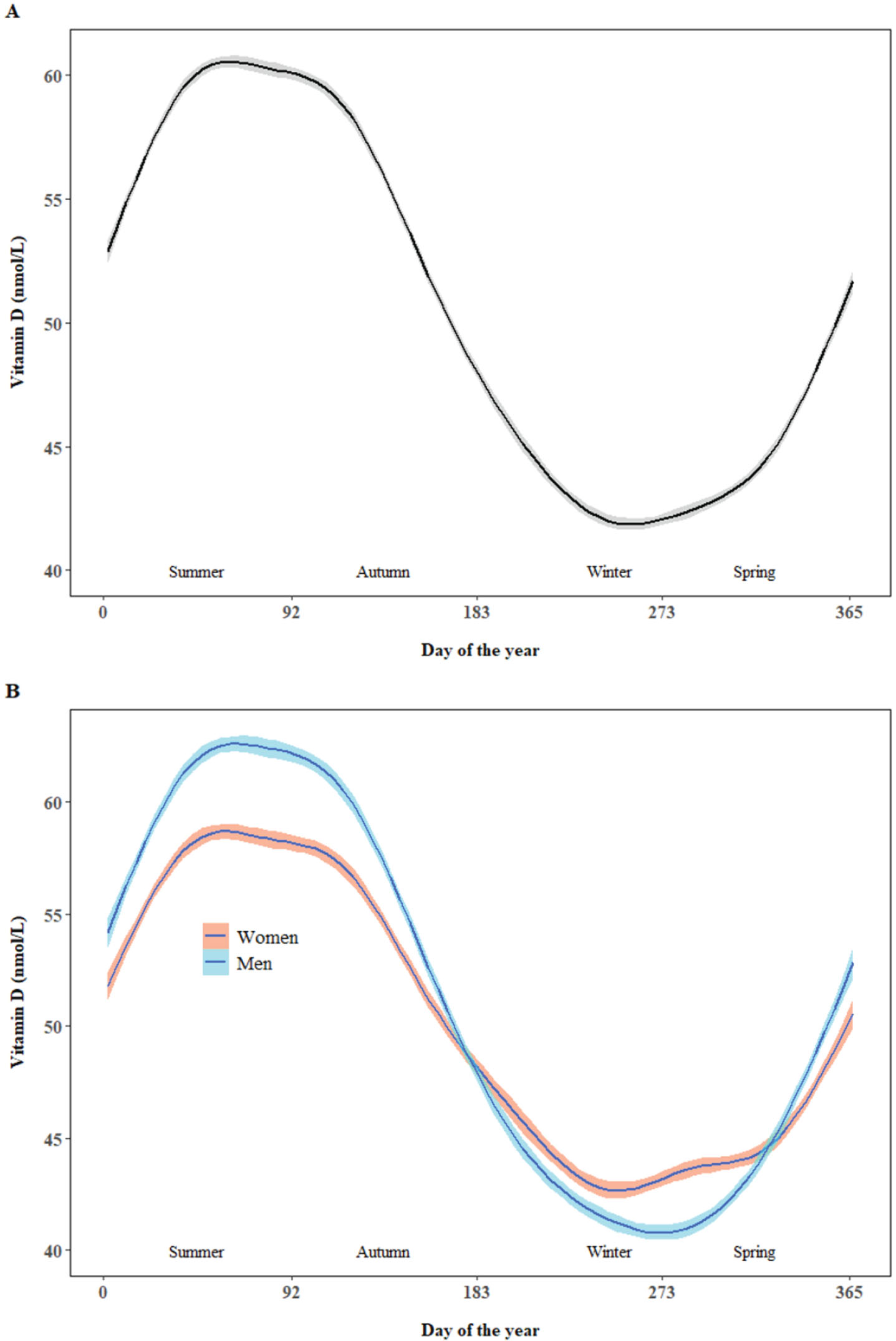
Hazard ratio curves for 25(OH)D with incident dementia across seasons
The risk of incident dementia increased with decreasing 25(OH)D adjusted for age, gender, and the day of the year when serum was collected (Fig. 2), and similar trends were observed for all seasons (Supplementary Figure 1). The cut-off points for 25(OH)D insufficiency and deficiency were 35.2 nmol/L and 17.8 nmol/L, respectively, for spring, and 50.4 nmol/L and 28.0 nmol/L for summer, 46.2 nmol/L and 24.0 nmol/L for autumn, and 33.4 nmol/L and 16.9 nmol/L for winter. Both linear and non-linear relationships between 25(OH)D and incident dementia were observed for spring, summer, and autumn (all p-values<0.05). Linear (p = 0.0002) but not non-linear relationship (p = 0.13) was found for winter. The prevalence of 25(OH)D insufficiency and deficiency was 29.9% and 5.0%, respectively.
Fig. 2
Hazard ratio curves for 25(OH)D with incident dementia. The curves were fitted using the Cox regression model with penalized spline smoothing adjusted for age, gender, and the day of the year when serum was collected. The solid black line represents the estimated hazard ratio for incident dementia, the dashed black line represents the 95% CI (Confidence Interval), and the red line represents when the hazard ratio is 1. Panels A, B, C, and D represent the curve for spring, summer, autumn, and winter, respectively. Both linear and non-linear relationships were tested and cubic regression splines with four knots were examined.
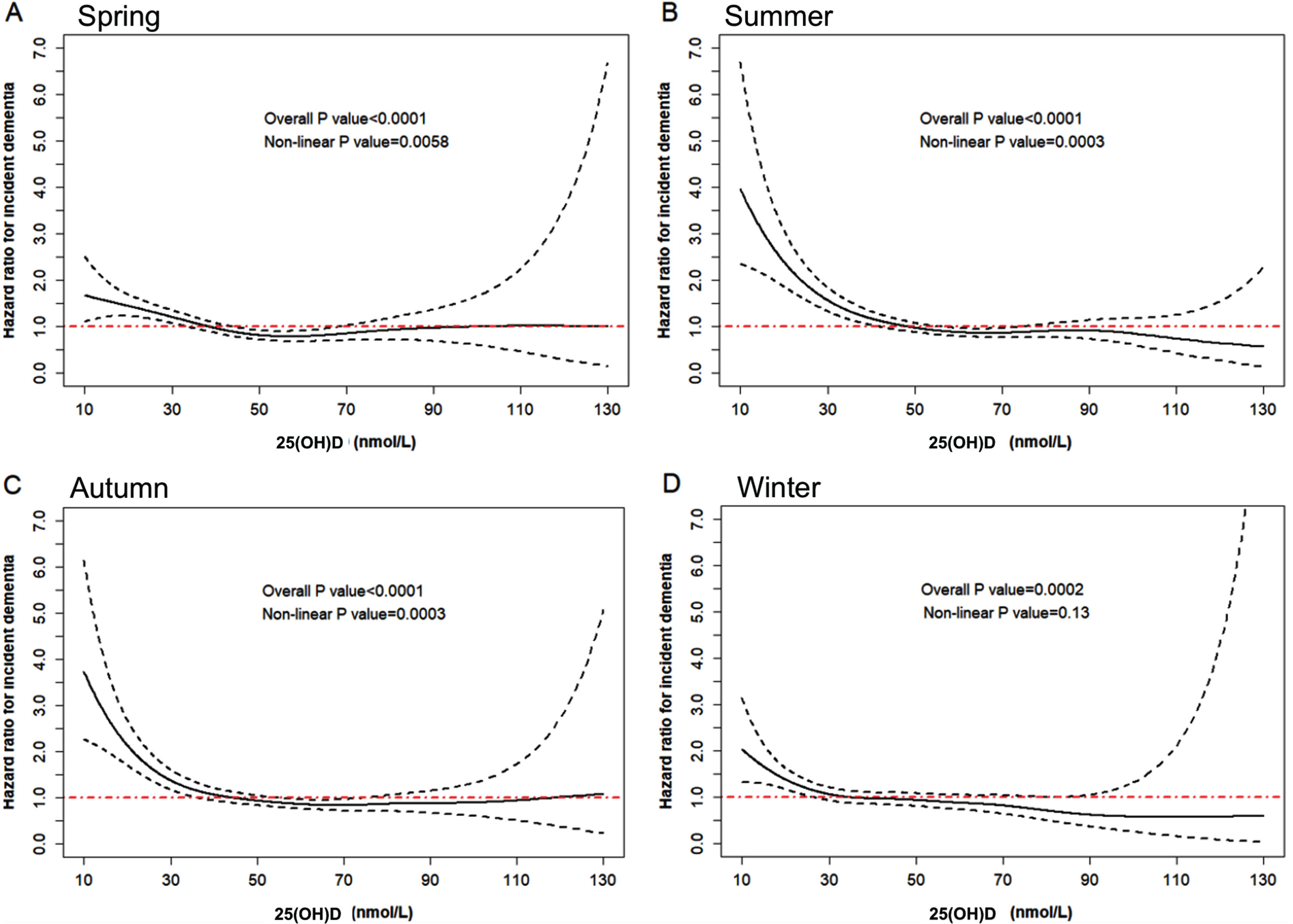
As shown in Supplementary Table 2, individuals with 25(OH)D deficiency were more likely to be females, have low income and education, and be non-whites. 25(OH)D deficiency was associated with higher BMI, cystatin C, and glycosylated hemoglobin, and lower physical activity and diet score. 25(OH)D deficiency was also associated with a higher prevalence of diabetes, obesity, stroke, heart disease, and depression at baseline.
25(OH)D insufficiency and deficiency and incident dementia
There was a lower probability of survival free of dementia during follow-up in individuals with vitamin insufficiency or deficiency (Fig. 3). 25(OH)D insufficiency (HR (95% CI): 1.5 (1.3–1.8)) and deficiency (1.6 (1.1–2.3)) tested in spring were both associated with an increased risk of incident dementia (Table 2). The multivariable-adjusted HR (95% CI) for incident dementia associated with 25(OH)D insufficiency and deficiency was 1.3 (1.1–1.5) and 1.9 (1.4–2.6) for summer, respectively. The corresponding numbers for autumn were 1.1 (0.9–1.4) and 2.1 (1.5–2.9), respectively. 25(OH)D insufficiency tested in winter was associated with an increased risk of incident dementia before (HR (95% CI): 1.3 (1.0–1.6)) but not after adjustment for all covariates (1.2 (0.9–1.5)). 25(OH)D deficiency tested in winter was independently associated with an increased risk of incident dementia (1.7 (1.1–2.5)). The multivariable-adjusted HR (95% CI) for incident dementia associated with 25(OH)D insufficiency and deficiency with seasons combined was 1.3 (1.1–1.4 and 1.84 (1.6–2.2), respectively. Similar results were observed when 25(OH)D insufficiency and deficiency were defined using non-season-specific cut-off points (Supplementary Table 3).
Fig. 3
Kaplan-Meier curves for rates of all-cause dementia by 25(OH)D status. The cut-off points for 25(OH)D insufficiency and deficiency were 35.2 nmol/L and 17.8 nmol/L, respectively, for spring, 50.4 nmol/L and 28.0 nmol/L for summer, 46.2 nmol/L and 24.0 nmol/L for autumn, and 33.4 nmol/L and 16.9 nmol/L for winter. The cut-off points were estimated based on the knots generated in Fig. 2.
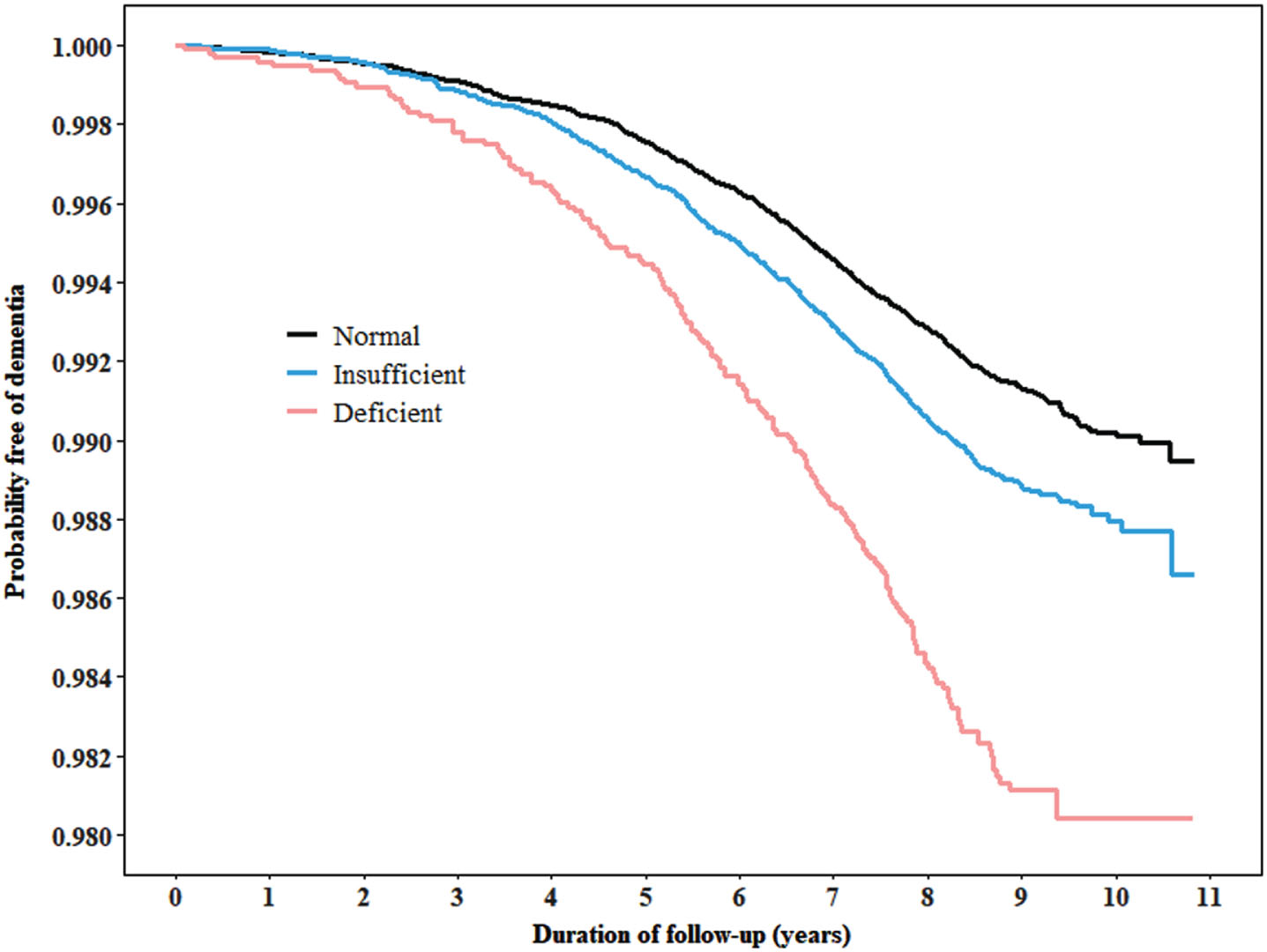
Table 2
The risk for incident dementia associated with 25(OH)D by seasons
25(OH)D | ||||
Normal | Insufficient | Deficient | p-trend | |
Spring | ||||
Range (nmol/L) | 10.0–17.8 | 17.9–35.2 | ≥35.3 | |
Events | 281 | 186 | 33 | |
Person-years | 319,338 | 146,930 | 24,354 | |
Incidence | 0.9 | 1.3 | 1.4 | |
HR (95% CI), Model 1 | 1.0 | 1.5 (1.2–1.8) | 1.6 (1.1–2.3) | <0.0001 |
HR (95% CI), Model 2 | 1.0 | 1.5 (1.3–1.8) | 1.6 (1.1–2.3) | <0.0001 |
Summer | ||||
Range (nmol/L) | 10.0–28.0 | 28.1–50.4 | ≥50.5 | |
Events | 314 | 179 | 55 | |
Person-years | 302,662 | 137,969 | 22,775 | |
Incidence | 1.0 | 1.3 | 2.41 | |
HR (95% CI), Model 1 | 1.0 | 1.3 (1.1–1.6) | 2.5 (1.9–3.3) | <0.0001 |
HR (95% CI), Model 2 | 1.0 | 1.3 (1.1–1.5) | 1.9 (1.4–2.6) | <0.0001 |
Autumn | ||||
Range (nmol/L) | 10.0–24.0 | 24.1–46.2 | ≥46.3 | |
Events | 263 | 140 | 52 | |
Person-years | 272,827 | 125,247 | 20,621 | |
Incidence | 1.0 | 1.1 | 2.5 | |
HR (95% CI), Model 1 | 1.0 | 1.2 (1.0–1.5) | 2.7 (2.0–3.7) | <0.0001 |
HR (95% CI), Model 2 | 1.0 | 1.1 (0.9–1.4) | 2.1 (1.5–2.9) | <0.0001 |
Winter | ||||
Range (nmol/L) | 10.0–16.9 | 17.0–33.4 | ≥33.5 | |
Events | 217 | 122 | 32 | |
Person-years | 227,625 | 10,4421 | 17,049 | |
Incidence | 1.0 | 1.2 | 1.9 | |
HR (95% CI), Model 1 | 1.0 | 1.3 (1.0–1.6) | 2.1 (1.4–3.0) | 0.0002 |
HR (95% CI), Model 2 | 1.0 | 1.2 (0.9–1.5) | 1.7 (1.1–2.5) | 0.0246 |
Combined | ||||
Events | 1,075 | 627 | 172 | |
Person-years | 1,122,451 | 514,566 | 84,800 | |
Incidence | 1.0 | 1.2 | 2.0 | |
HR (95% CI), Model 1 | 1.0 | 1.3 (1.2–1.5) | 2.2 (1.9–2.6) | <0.0001 |
HR (95% CI), Model 2 | 1.0 | 1.3 (1.1–1.4) | 1.8 (1.6–2.2) | <0.0001 |
Incidence of dementia represents number of cases per 1000 person-years. Hazard ratio (95% CI) for incident dementia associated with 25(OH)D status was estimated using Cox proportional regression models. Model 1 was adjusted for age and gender; Model 2 was adjusted for model 1 plus the day of the year when serum was collected, ethnicity, education, income, diet score, 25(OH)D supplement, smoking, alcohol consumption, sleep, physical activity, BMI, cholesterol, glycosylated hemoglobin, cystatin C, depression, hypertension, diabetes, heart disease, and chronic kidney disease at baseline.
Vitamin D deficiency tested in spring (HR (95% CI): 1.4 (1.1–1.8)), summer (2.0 (1.4–2.9)) or autumn (1.8 (1.3–2.4)) but not winter (1.1 (0.8–1.4)) was associated with an increased risk of AD. The multivariable-adjusted HR (95% CI) for incident AD associated with vitamin D deficiency with seasons combined was 1.4 (1.2–1.6) (Supplementary Table 4). Vitamin D deficiency tested in summer, autumn, or winter but not spring was associated with an increased risk of vascular dementia. In the multivariable analysis, vitamin D deficiency with seasons combined was associated with an increased risk of vascular dementia (HR (95% CI): 1.3 (1.1–1.6), Supplementary Table 5).
Moderation analysis
The association between low levels of 25(OH)D (insufficiency/deficiency) and incident dementia was stronger in individuals with lower education (HR (95% CI) for 0–5 years: 1.6 (1.4–1.9), 6–12 years: 1.2 (1.0–1.4),≥13 years: 1.3 (1.0–1.6); p-value for interaction =0.0442). No significant interaction for other factors was observed (Fig. 4).
Fig. 4
Low levels of 25(OH)D and incident dementia stratified by important factors. Cox regression models were used to estimate the hazard ratio (95% CI) for incident dementia associated with low levels of vitamin D. Low levels of 25(OH)D refer to 25(OH)D insufficiency and deficiency combined.
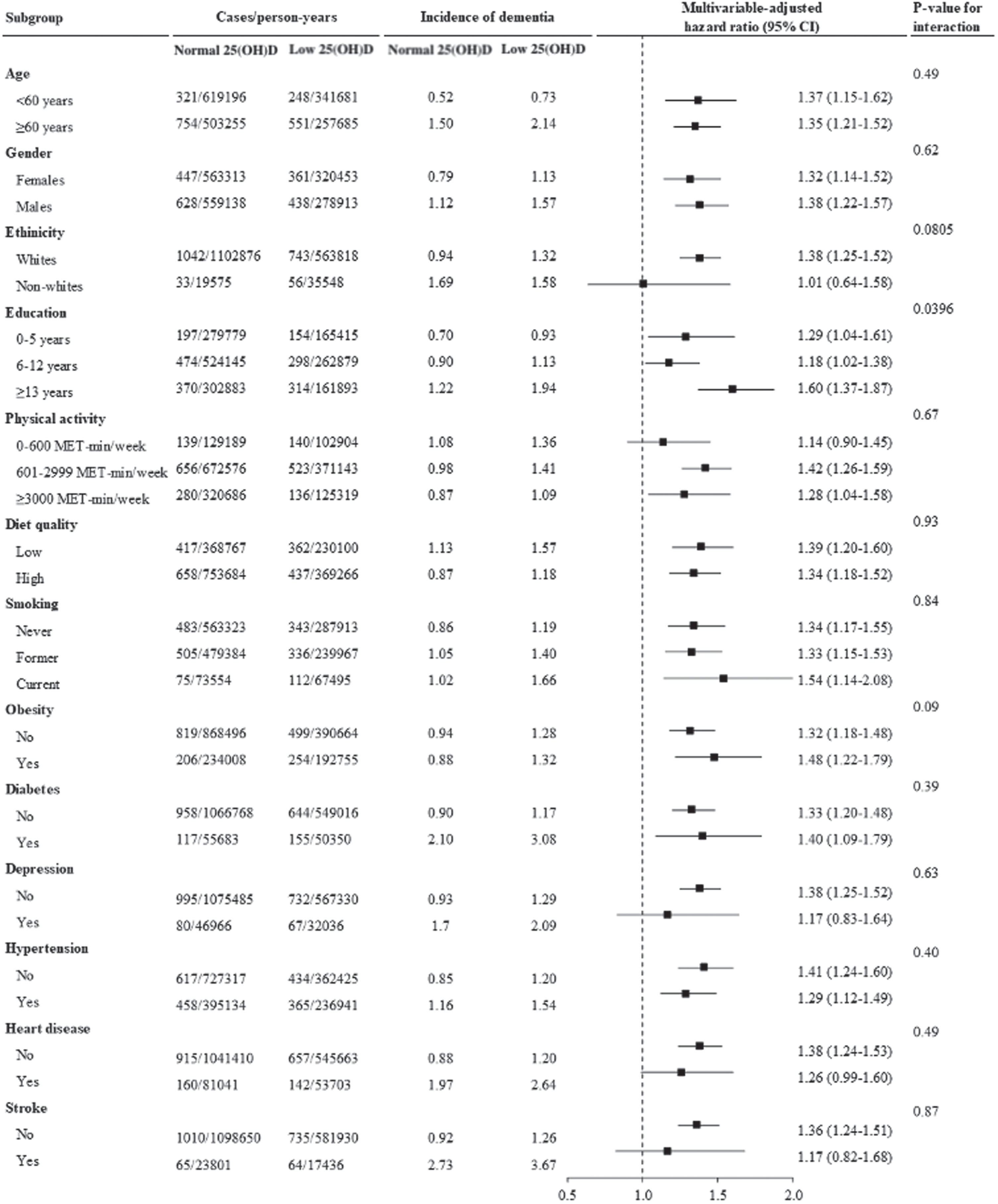
Mediation analysis
Percentage of the total effect of the association between low levels of 25(OH)D (insufficiency/deficiency) and incident dementia explained by stroke, depression, diabetes, and heart disease identified during follow-up was 5.9%, 4.7%, 3.8%, and 2.3%, respectively. These conditions together explained 12.9% of the association (Supplementary Figure 2).
Sensitivity analysis
Individuals in the first and secondary quintiles of month-specific 25(OH)D had a higher risk of incident dementia compared with those in the fifth quintile (HR (95% CI): 1.6 (1.4–1.8) for the first quintile, 1.2 (1.1–1.4) for the secondary quintile). Similar results were observed across seasons (Supplementary Table 6).
DISCUSSION
In this large population of older adults aged 60 years or over, there was significant variation in 25(OH)D between seasons. The cut-off points for 25(OH)D insufficiency and deficiency for summer and autumn were significantly higher than for spring and winter. 25(OH)D insufficiency and deficiency determined according to these cut-off points were both associated with an increased risk of incident dementia and this association was significant across seasons. Newly developed stroke, depression, diabetes, and heart disease mediated the association between low levels of 25(OH)D and incident dementia.
25(OH)D deficiency and insufficiency is a public health challenge globally [26]. Our research is consistent with previous studies demonstrating that there was significant seasonal variation in 25(OH)D [27]. The average 25(OH)D for summer was 15 nmol/L higher than that for winter in our study highlighting the importance of sunlight exposure as the major source of 25(OH)D for most adults [28]. Moreover, dietary intake is another determinant of 25(OH)D status. Previous study has found that the highest tertile of dietary 25(OH)D consumption is associated with a 27% dementia hazard reduction [29]. While meat is an important source of 25(OH)D but also is a risk factor for AD [30]. It is critical to obtain an adequate amount of 25(OH)D from selective dietary sources and sunlight exposure especially in winter and spring.
There are several potential mechanisms for the association between 25(OH)D deficiency and an increased risk of dementia. Age, gender, depression, hypertension, diabetes, heart disease, and chronic kidney disease may confound the association between 25(OH)D and incident dementia. All these covariates were adjusted for in our multivariable analysis suggesting the potential role of 25(OH)D on the development of dementia via other mechanisms. Evidence showed 25(OH)D and 25(OH)D receptors being related to mitochondrial gene transcription and energy metabolism in the human brain [31], and previous vitro and in vivo work has demonstrated that 25(OH)D has an important role in brain development and function [32]. 25(OH)D may be involved in the clearance of amyloid-β [33], and an optimal 25(OH)D level corresponds to lower tau protein levels in the cerebrospinal fluid, suggesting a reduced dementia risk [34]. In addition, 25(OH)D sufficiency was associated with reduced inflammation and oxidative stress [35, 36], which provides a rationale for the inverse association between 25(OH)D and dementia. Moreover, the deficiency is associated with increased dementia-related conditions’ risks, including hypertension [37], diabetes [13, 17], cardiovascular disease and depression [14, 18]. further underscores the protective potential of 25(OH)D against the development of dementia.
Our study is in line with two previous prospective cohort studies demonstrating that 25(OH)D deficiency was associated with an increased risk of incident dementia [10, 11]. However, other prospective studies showed that 25(OH)D was not significantly associated with the incidence of dementia [7, 12, 38–40]. A meta-analysis involving these cohort studies demonstrated that 25(OH)D deficiency was associated with an increased risk of dementia (pooled relative risk (95% CI): 1.30 (1.1–1.5) [9]. The inconsistent findings may be due to the difference in population and mean age between cohorts in these studies. Although the season was adjusted for in some of these studies, misclassification of 25(OH)D deficiency cannot be solved as season-specific cut-off points were not used. Our study is the first to demonstrate that there was a significant non-linear relationship between 25(OH)D and incident dementia. We also estimated the cut-off points for 25(OH)D deficiency for each season with much lower levels in spring and winter than in summer and autumn. This suggests the definition of 25(OH)D insufficiency and deficiency should be season specific.
Our findings indicate that 25(OH)D deficiency is more strongly associated with an increased risk of dementia during spring and winter compared to autumn and summer. This pattern aligns with seasonal variations in sunlight exposure, which is a primary source of vitamin D. Notably, lower sunlight exposure in winter and spring correlates with reduced 25(OH)D levels, underscoring the importance of vitamin D supplementation during these seasons for dementia prevention.
As 25(OH)D deficiency is associated with an increased risk of hypertension, diabetes, cardiovascular disease, and depression [7, 13–16], these conditions may moderate or mediate the association between 25(OH)D deficiency and incident dementia. Our study showed that the association between 25(OH)D and incident dementia did not differ between individuals with and without these conditions. Our further analysis also demonstrated that stroke, depression, diabetes, and heart disease identified during follow-up were significant mediators for the association between 25(OH)D and incident dementia. These newly developed conditions together explained 12.9% of the total effect, but the association between 25(OH)D deficiency and incident dementia remained significant. However, no such study has been published so that more research is needed to warrant our findings.
To our knowledge, this is the first study to estimate the cut-off points for 25(OH)D insufficiency and deficiency for each season and their associations with the incidence of dementia. Our study also uniquely examined the potential mediation effects of newly developed well-known dementia risks on the association between 25(OH)D and incident dementia. The present study also has several limitations. Firstly, some cases of all-cause dementia might not be captured in the medical records or death registers. However, previous research has shown that there is a good agreement between dementia case ascertainment with primary care records [41]. Because of the observational design of our study, causal relationships cannot be established based on our findings. The predominant proportion of participants in our study were Caucasian, therefore the cut-off points for 25(OH)D insufficiency and deficiency may be unlikely to apply in other ethnic groups. Finally, our analysis was restricted to a subgroup of the UK Biobank cohort who were aged 60–73 years old. Thus, our findings may not be generalized to the whole population with a wide age range.
Conclusion
We found seasonal differences in cut-off points of 25(OH)D insufficiency and deficiency that were associated with an increased risk of incident dementia in older adults residing in the UK. Newly developed stroke, depression, diabetes, and heart disease mediated the association between 25(OH)D and incident dementia.
AUTHOR CONTRIBUTIONS
Jiahao Liu (Writing – original draft); Eddy Roccati, PhD (Data curation); Yutong Chen, BSc (Writing – review & editing); Zhuoting Zhu, MD, PhD (Project administration); Wei Wang, MD, PhD (Investigation); Mingguang He, MD, PhD (Funding acquisition; Resources); Xianwen Shang, MPH, PhD (Conceptualization; Formal analysis; Visualization; Writing – review & editing).
ACKNOWLEDGMENTS
The authors have no acknowledgments to report.
FUNDING
The present work was supported by the Fundamental Research Funds of the State Key Laboratory of Ophthalmology, Project of Investigation on Health Status of Employees in Financial Industry in Guangzhou, China (Z012014075), Science and Technology Program of Guangzhou, China (202002020049). Mingguang He receives support from the University of Melbourne at Research Accelerator Program and the CERA Foundation. The Centre for Eye Research Australia receives Operational Infrastructure Support from the Victorian State Government. Mingguang He also receives support from the Australian Government (MRFF: MRFAI000035).
CONFLICT OF INTEREST
The authors have no conflict of interest to report.
DATA AVAILABILITY
Restrictions apply to the availability of these data. Data was obtained from UK Biobank and are available https://www.ukbiobank.ac.uk with the permission of UK Biobank.
SUPPLEMENTARY MATERIAL
[1] The supplementary material is available in the electronic version of this article: https://dx.doi.org/10.3233/ADR-230077.
REFERENCES
[1] | Rossor M , Collinge J , Fox N , Mead S , Mummery C , Rohrer J , Schott J , Warren J ((2016) ) Dementia and cognitive impairment. In Neurology: A Queen Square Textbook, Second Edition, Clarke C, Howard R, Rossor M, Shorvon S, eds. John Wiley & Sons, Ltd., pp. 289–336. |
[2] | Dursun E , Gezen-Ak D ((2019) ) Vitamin D basis of Alzheimer’s disease: From genetics to biomarkers. Hormones 18: , 7–15. |
[3] | Prince M , Wimo A , Guerchet M , Ali G-C , Wu Y-T , Prina M ((2015) ) World Alzheimer Report 2015. The global impact of dementia: An analysis of prevalence, incidence, cost and trends. Alzheimer’s Disease International, London. |
[4] | Rushton R ((2020) ) Dementia and Alzheimer’s disease deaths including comorbidities, England and Wales: 2019 registrations. Office for National Statistics. |
[5] | Wang X , Huang W , Su L , Xing Y , Jessen F , Sun Y , Shu N , Han Y ((2020) ) Neuroimaging advances regarding subjective cognitive decline in preclinical Alzheimer’s disease. Mol Neurodegener 15: , 1–27. |
[6] | Balion C , Griffith LE , Strifler L , Henderson M , Patterson C , Heckman G , Llewellyn DJ , Raina P ((2012) ) Vitamin D, cognition, and dementia: A systematic review and meta-analysis. Neurology 79: , 1397–1405. |
[7] | Zhang D , Cheng C , Wang Y , Sun H , Yu S , Xue Y , Liu Y , Li W , Li X ((2020) ) Effect of vitamin D on blood pressure and hypertension in the general population: An update meta-analysis of cohort studies and randomized controlled trials. Prev Chronic Dis 17: , E03. |
[8] | Gezen-Ak D , Dursun E ((2023) ) Vitamin D, a secosteroid hormone and its multifunctional receptor, vitamin D receptor, in Alzheimer’s type neurodegeneration. J Alzheimers Dis 95: , 1273–1299. |
[9] | Chai B , Gao F , Wu R , Dong T , Gu C , Lin Q , Zhang Y ((2019) ) Vitamin D deficiency as a risk factor for dementia and Alzheimer’s disease: An updated meta-analysis. BMC Neurol 19: , 284. |
[10] | Afzal S , Bojesen SE , Nordestgaard BG ((2014) ) Reduced 25-hydroxyvitamin D and risk of Alzheimer’s disease and vascular dementia. Alzheimers Dement 10: , 296–302. |
[11] | Feart C , Helmer C , Merle B , Herrmann FR , Annweiler C , Dartigues JF , Delcourt C , Samieri C ((2017) ) Associations of lower vitamin D concentrations with cognitive decline and long-term risk of dementia and Alzheimer’s disease in older adults. Alzheimers Dement 13: , 1207–1216. |
[12] | Licher S , de Bruijn R , Wolters FJ , Zillikens MC , Ikram MA , Ikram MK ((2017) ) Vitamin D and the risk of dementia: The Rotterdam Study. J Alzheimers Dis 60: , 989–997. |
[13] | Barbarawi M , Zayed Y , Barbarawi O , Bala A , Alabdouh A , Gakhal I , Rizk F , Alkasasbeh M , Bachuwa G , Manson JE ((2020) ) Effect of Vitamin D supplementation on the incidence of diabetes mellitus. J Clin Endocrinol Metab 105: , dgaa335. |
[14] | Shi H , Chen H , Zhang Y , Li J , Fu K , Xue W , Teng W , Tian L ((2020) ) 25-Hydroxyvitamin D level, vitamin D intake, and risk of stroke: A dose-response meta-analysis. Clin Nutr 39: , 2025–2034. |
[15] | Gholami F , Moradi G , Zareei B , Rasouli MA , Nikkhoo B , Roshani D , Ghaderi E ((2019) ) The association between circulating 25-hydroxyvitamin D and cardiovascular diseases: A meta-analysis of prospective cohort studies. BMC Cardiovasc Disord 19: , 248. |
[16] | Li H , Sun D , Wang A , Pan H , Feng W , Ng CH , Ungvari GS , Tao L , Li X , Wang W , Xiang YT , Guo X ((2019) ) Serum 25-hydroxyvitamin D levels and depression in older adults: A dose-response meta-analysis of prospective cohort studies. Am J Geriatr Psychiatry 27: , 1192–1202. |
[17] | Dawson-Hughes B , Staten MA , Knowler WC , Nelson J , Vickery EM , LeBlanc ES , Neff LM , Park J , Pittas AG ((2020) ) Intratrial exposure to vitamin D and new-onset diabetes among adults with prediabetes: A secondary analysis from the vitamin D and type 2 diabetes (D2d) study. Diabetes Care 43: , 2916–2922. |
[18] | Musazadeh V , Keramati M , Ghalichi F , Kavyani Z , Ghoreishi Z , Alras KA , Albadawi N , Salem A , Albadawi MI , Salem R ((2022) ) Vitamin D protects against depression: Evidence from an umbrella meta-analysis on interventional and observational meta-analyses. Pharmacol Res 187: , 106605. |
[19] | Sudlow C , Gallacher J , Allen N , Beral V , Burton P , Danesh J , Downey P , Elliott P , Green J , Landray M , Liu B , Matthews P , Ong G , Pell J , Silman A , Young A , Sprosen T , Peakman T , Collins R ((2015) ) UK biobank: An open access resource for identifying the causes of a wide range of complex diseases of middle and old age. PLoS Med 12: , e1001779. |
[20] | IPAQ Research Committee ((2005) ), Guidelines for data processing and analysis of the International Physical Activity Questionnaire (IPAQ)-short and long forms. |
[21] | Mozaffarian D ((2016) ) Dietary and policy priorities for cardiovascular disease, diabetes, and obesity: A comprehensive review. Circulation 133: , 187–225. |
[22] | Lourida I , Hannon E , Littlejohns TJ , Langa KM , Hyppönen E , Kuzma E , Llewellyn DJ ((2019) ) Association of lifestyle and genetic risk with incidence of dementia. JAMA 322: , 430–437. |
[23] | World Health Organization (WHO) ((2000) ) Obesity: Preventing and managing the global epidemic. Report of a WHO consultation. WHO, Geneva. |
[24] | Cadarso-Suarez C , Meira-Machado L , Kneib T , Gude F ((2010) ) Flexible hazard ratio curves for continuous predictors in multi-state models: An application to breast cancer data. Stat Model 10: , 291–314. |
[25] | Lin DY , Fleming TR , De Gruttola V ((1997) ) Estimating the proportion of treatment effect explained by a surrogate marker. Stat Med 16: , 1515–1527. |
[26] | Holick MF ((2017) ) The vitamin D deficiency pandemic: Approaches for diagnosis, treatment and prevention. Rev Endocr Metab Disord 18: , 153–165. |
[27] | Hypponen E , Boucher BJ , Berry DJ , Power C ((2008) ) 25-hydroxyvitamin D, IGF-1, and metabolic syndrome at 45 years of age: A cross-sectional study in the 1958 British Birth Cohort. Diabetes 57: , 298–305. |
[28] | Macdonald HM , Mavroeidi A , Fraser WD , Darling AL , Black AJ , Aucott L , O’Neill F , Hart K , Berry JL , Lanham-New SA , Reid DM ((2011) ) Sunlight and dietary contributions to the seasonal vitamin D status of cohorts of healthy postmenopausal women living at northerly latitudes: A major cause for concern? Osteoporos Int 22: , 2461–2472. |
[29] | Zhao C , Tsapanou A , Manly J , Schupf N , Brickman AM , Gu Y ((2020) ) Vitamin D intake is associated with dementia risk in the Washington Heights-Inwood Columbia Aging Project (WHICAP). Alzheimers Dement 16: , 1393–1401. |
[30] | Zhang H , Greenwood DC , Risch HA , Bunce D , Hardie LJ , Cade JE ((2021) ) Meat consumption and risk of incident dementia: Cohort study of 493,888 UK Biobank participants. Am J Clin Nutr 114: , 175–184. |
[31] | Gezen-Ak D , Alaylıoúglu M , Yurttaş Z , Čamoúglu T , Şengül B , İşler C , Kına ÜY , Keskin E , Atasoy İL , Kafardar AM ((2023) ) Vitamin D receptor regulates transcription of mitochondrial DNA and directly interacts with mitochondrial DNA and TFAM. J Nutr Biochem 116: , 109322. |
[32] | Cui X , Gooch H , Petty A , McGrath JJ , Eyles D ((2017) ) Vitamin D and the brain: Genomic and non-genomic actions. Mol Cell Endocrinol 453: , 131–143. |
[33] | Koyama A , Okereke OI , Yang T , Blacker D , Selkoe DJ , Grodstein F ((2012) ) Plasma amyloid-β as a predictor of dementia and cognitive decline: A systematic review and meta-analysis. Arch Neurol 69: , 824–831. |
[34] | Soares JZ , Valeur J , Šaltytė Benth J , Knapskog A-B , Selbæk G , Bogdanovic N , Pettersen R ((2022) ) Associations between intrathecal levels of vitamin D, cytokines, and core biomarkers of Alzheimer’s disease: A cross-sectional study. J Alzheimers Dis 89: , 825–834. |
[35] | Brouwer-Brolsma EM , de Groot LC ((2015) ) Vitamin D and cognition in older adults: An update of recent findings. Curr Opin Clin Nutr Metab Care 18: , 11–16. |
[36] | Mansournia MA , Ostadmohammadi V , Doosti-Irani A , Ghayour-Mobarhan M , Ferns G , Akbari H , Ghaderi A , Talari HR , Asemi Z ((2018) ) The effects of vitamin D supplementation on biomarkers of inflammation and oxidative stress in diabetic patients: A systematic review and meta-analysis of randomized controlled trials. Horm Metab Res 50: , 429–440. |
[37] | Mirhosseini N , Vatanparast H , Kimball SM ((2017) ) The association between serum 25 (OH) D status and blood pressure in participants of a community-based program taking vitamin D supplements. Nutrients 9: , 1244. |
[38] | Karakis I , Pase MP , Beiser A , Booth SL , Jacques PF , Rogers G , DeCarli C , Vasan RS , Wang TJ , Himali JJ , Annweiler C , Seshadri S ((2016) ) Association of serum vitamin D with the risk of incident dementia and subclinical indices of brain aging: The Framingham Heart Study. J Alzheimers Dis 51: , 451–461. |
[39] | Knekt P , Sääksjärvi K , Järvinen R , Marniemi J , Männistö S , Kanerva N , Heliövaara M ((2014) ) Serum 25-hydroxyvitamin d concentration and risk of dementia. Epidemiology 25: , 799–804. |
[40] | Olsson E , Byberg L , Karlström B , Cederholm T , Melhus H , Sjögren P , Kilander L ((2017) ) Vitamin D is not associated with incident dementia or cognitive impairment: An 18-y follow-up study in community-living old men. Am J Clin Nutr 105: , 936–943. |
[41] | Sibbett RA , Russ TC , Deary IJ , Starr JM ((2017) ) Dementia ascertainment using existing data in UK longitudinal and cohort studies: A systematic review of methodology. BMC Psychiatry 17: , 239. |