ABA Supplementation Rescues IRS2 and BDNF mRNA Levels in a Triple-Transgenic Mice Model of Alzheimer’s Disease
Abstract
Insulin resistance underlies Alzheimer’s disease (AD) by affecting neuroinflammation and brain-derived neurotrophic factor (BDNF) expression. Here, we evaluated the effect of early and late-start abscisic acid (ABA) intervention on hippocampal BDNF, tumor necrosis factor α (TNFα), and insulin receptors substrates (IRS) 1/2 mRNA levels in a triple-transgenic mice model of AD. Transgenic mice displayed lower BDNF and IRS2, equal IRS1, and higher TNFα expression compared to wild-type mice. Late ABA treatment could rescue TNFα and increased IRS1/2 expression. However, early ABA administration was required to increase BDNF expression. Our data suggests that early intervention with ABA can prevent AD, via rescuing IRS1/2 and BDNF expression.
INTRODUCTION
Alzheimer’s disease (AD) is the most common type of dementia, and it is characterized by progressive memory loss, cognitive impairment, and functional decline. The main histopathological hallmarks of AD are the accumulation of extracellular amyloid-β peptide (Aβ) and hyperphosphorylated tau protein in intracellular neurofibrillary tangles that correlate with significant glucose hypometabolism. Scientific evidence has demonstrated that the onset of neurodegeneration occurs decades before the first clinical symptoms appear [1]. At these early stages, the neuroinflammatory status and insulin resistance are key players at the onset of the disease, as they can cause irreversible neuronal damage [2]. Late-onset AD has an idiopathic etiology; however, several risk factors have been identified, and metabolic syndrome leading to type II diabetes are considered major risks factors for AD [3]. One of the well-studied targets of insulin resistance is tau phosphorylation, and the presence of hyperphosphorylated tau in the patient blood serum is a significant predictive biomarker of AD [4]. Insulin signaling starts by the binding to the insulin receptor, and it is mediated by the insulin receptor substrates isoforms 1 and 2 (IRS1 and IRS2). We previously reported reduced levels of hippocampal IRS2 mRNA in an animal model of metabolic syndrome that also displayed cognitive impairment [5]. Furthermore, human studies have shown that IRS2 mRNA and protein expression are significantly decreased in the hippocampus and temporal [6] and prefrontal cortexes [7] of AD patients compared to control subjects. The administration of molecules with anti-inflammatory capability can rescue the IRS2 mRNA and protein levels, as shown with ABA administration to rats with metabolic syndrome [5], and curcumin in a rat model of AD [8]. IRS2 rescuing, in all cases, correlates with cognitive function improvement.
Interestingly, insulin signaling is strongly linked with brain-derived neurotrophic factor (BDNF), a key neurotrophic factor for neuronal survival and plasticity [9]. In fact, diabetic patients display distinctly reduced BDNF serum levels compared to non-diabetic controls [10]. Low BDNF expression is strongly correlated with high brain inflammatory status [11]. Not only is the BDNF function reduced in AD, but it is also considered a fundamental target for disease management, given its fundamental role in neuroprotection [12].
Abscisic acid (ABA) is a phytohormone with insulin sensitizing and anti-inflammatory properties. In our previous studies, we evaluated two ABA treatments in a 3xTg model of AD, an early-start (duration of 5 months) and late-start (duration of 3 months). We observed that, early-start treatment was required to effectively ameliorate memory impairment and prevent microglia switching to a reactive state [13]. To better understand ABA mechanism of action, we hypothesized that ABA could enhance BDNF and IRS1/IRS2 expression levels, thereby improving insulin signaling, and neurotrophic response, thus preventing neurodegeneration.
METHODS
Mice and treatment
In this study, frozen brains from the mice used in a preceding study [13], where analyzed. In that former report, wild type and transgenic mice had been divided randomly into three experimental groups: Control (no ABA); ABA 3M; and ABA 5M. All mice were sacrificed at 8 months of age. ABA (purchased from Fernandez-Rapado, Spain) was added to the drinking water (20 mg/L) for 3 (ABA 3M) or 5 months (ABA 5M), respectively.
The procedures followed directive 86/609/EEC of the European Community on the protection of animals used for experimental and other scientific purposes. The experiments were approved by the Ethics Committee of the University Jaume I (approval number 2014/VSC/PEA00209).
RNA extraction and cDNA synthesis
Hippocampus were dissected from frozen brains and total RNA extracted (n = 5–9) using the RNeasy LipidTissue Mini Kit (Qiagen), according to the manufacturer’s protocol. Briefly, tissue was disrupted and homogenized in QIAzol Lysis Reagent. After 5 min incubation at room temperature (15–25°C), chloroform was added to the homogenate, centrifuged (12,000×g, 15 min at 4°C) and the upper, aqueous phase, transferred to a new tube with 1 volume of 70% of ethanol. Sample was inoculated into a RNeasy Mini spin column and after several washes, genomic DNA removed using DNase I, (Life Technologies, Carlsbad, CA, USA), for 30 min at 37°C. Finally, the RNA was eluted in RNAse-free water and concentration and purity evaluated with a nanodrop (Thermo Scientific Nanodrop 2000c). 1μg of RNA was used for reverse transcription using (Prime- Script™ RT Reagent Kit (Takara Bio Inc., Shiga, Japan). The mixture was incubated at 37°C for 15 min and heated at 85°C for 5 min to terminate reaction. DNA concentration was evaluated as above and subsequently stored at –20°C.
Real-time quantitative polymerase chain reaction
Primers were designed using the Primer3 software tool (https://primer3.ut.ee/, accessed in November 2018) (Table 1). RT-PCR was performed using Maxima SYBR Green/ROX qPCR Master Mix (2X) (Applied Biosystems Life Technologies, Carlsbad, CA, USA) as in [14]. Briefly, the reaction started with one cycle at 98°C (10 min), followed by 40 cycles at 98°C (10 s), 60°C (10 s), and 72°C (20 s). Each sample was tested in triplicate and normalized to the housekeeping gene GAPDH. Relative gene expression was calculated using 2–ΔΔCt, using the wild type treated with vehicle as control group.
Table 1
Primer sequences
Gene | Forward Primer (5′–3′) | Reverse Primer (5′–3′) |
IRS1 | CCTGACATTGGAGGTGGGTC | TGGGGATCTTCTGGGCCATA |
IRS2 | GCAGCCAGGAGACAAGAACT | AGCGCTTCACTCTTTCACGA |
BDNF | TACCTGGATGCCGCAAACAT | AGTTGGCCTTTGGATACCGG |
TNF α | GACCCTCACACTCAGATCA | TGCTACGACGTGGGCTACG |
GAPDH | TGCCCCCATGTTTGTGATG | TGGTGGTGCAGGATGCATT |
Statistics
Data were expressed as mean±SEM using Graph Pad Prism version 8. Data were subjected to an unpaired Student t-test, two-tail, with a confidence of 95%, for the identification of the statistical differences between means. Gaussian distribution was monitored with the Shapiro–Wilk normality test.
RESULTS
ABA treatment reduces inflammatory cytokine TNFα while increasing BDNF mRNA expression in hippocampi of triple-transgenic mice
We first evaluated the levels of cytokine tumor necrosis factor α (TNFα) to confirm the inflammatory status of the 3xTg mice and the effect of ABA (Fig. 1A). We have previously shown that the microglia morphology in 3xTg mice is less ramified, corresponding to an M1/M2 status, compared to the age-matched controls. However, M1 and M2 differ in their cytokine profile, being M1 pro-inflammatory with higher levels of TNFα.
Fig. 1
ABA treatment (A) reduces hippocampal TNF and (B) increases BDNF expression as measured by qPCR. Data are represented as the mean±of at least 5–9 independent subjects in triplicate. Data were analyzed by Student t-test (*p < 0.05, **p < 0.01, and ****p < 0.0001 for ABA effect; # #p < 0.01 and # # #p < 0.001 for genotype effect).
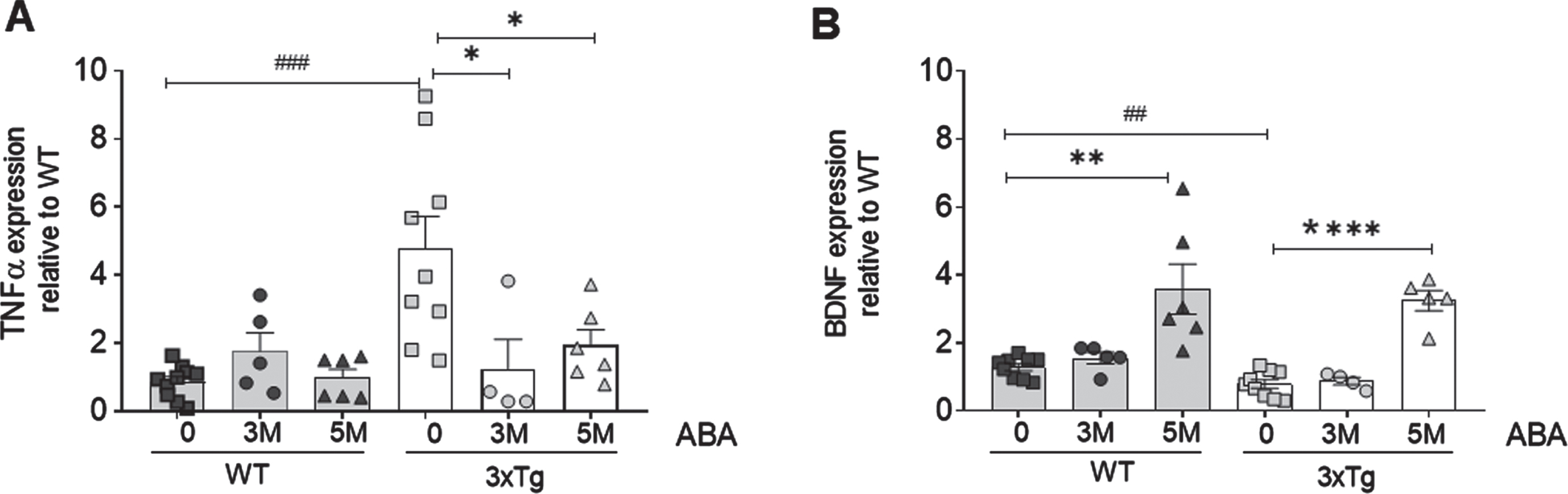
Here we show that, as expected, at the age of 8 months, untreated 3xTg mice have significantly higher levels of TNFα than wild-type animals (***p = 0.0004; t = 4.34; df = 17). Confirming our previous effect on inflammatory microglia, ABA administration significantly prevented the increase in the TNFα mRNA levels in the 3xTg mice hippocampi, both at 3M ABA (*p = 0.042; t = 2.298; df = 11) and 5 M ABA (*p = 0.036; t = 2.33; df = 13) treatment.
Next, we measured the BDNF mRNA (Fig. 1B), given its relevance in neuron survival and activity, and the evidence showing that BDNF activity is compromised in AD models [15]. We confirmed that untreated 3xTg mice had reduced hippocampal BDNF mRNA levels compared to wild type (**p = 0.009; t = 2.268; df = 16). Next, we evaluated the potential benefit of ABA treatment and found that; late-start of ABA administration (3M ABA) did not increase the BDNF mRNA levels. However, 3xTg mice that received an early-start of ABA treatment (5M ABA) displayed hippocampal BDNF levels significantly higher than untreated 3xTg mice (****p < 0.0001; t = 8.865; df = 12). Interestingly, we found that this mode of ABA administration also increased the hippocampal BDNF in the wild-type mice (**p = 0.002; t = 3.79; df = 13).
ABA treatment increases mRNA levels of insulin substrate receptors 1 and 2
Next, we determined the levels of IRS1 and IRS2 at 3 and 5 months of ABA treatment (Fig. 2). We observed that, at 8 months of age, the IRS2 levels (Fig. 2A) were significantly lower in the hippocampi of the 3xTg mice compared to the wild type (# #p = 0.0075; t = 3.34; df = 10). Early-start ABA treatment (5M ABA) rescued the levels of hippocampal IRS2 mRNA expression in the 3xTg mice compared to untreated mice (*p = 0.043; t = 2.35; df = 9). The late-start ABA treatment (3M ABA) increased the expression but did not reach statistical significance. In the wild-type mice, 3M ABA treatment induced a significant increase in the IRS2 mRNA expression (***p = 0.0003; t = 5.372; df = 8). However, the increases in IRS2 mRNA obtained with the 5M ABA did not reach statistical significance. On the contrary, the IRS1 levels were not different in the untreated 3xTg mice compared to the wild-type mice at 8 months of age. In the wild-type mice, ABA treatment significantly increased the IRS1 mRNA levels, both the short (*p = 0.0215; t = 2.642; df = 12) and long (*p = 0.0311; t = 2.44; df = 12) administration, while in the 3xTg mice, 3M ABA did not induce a significant effect, but 5M ABA induced significant IRS1 mRNA increase (*p = 0.0115; t = 2.980; df = 12).
Fig. 2
ABA administration increases IRS mRNA expression in hippocampi as measured by qPCR. A) IRS1 expression increases in WT mice as soon as 3 months of ABA treatment, but in 3xTg mice, only at 5 months. B) IRS2 increases in WT animals at 3 months of treatment in WT animals. Data are represented as the mean±of at least 5–9 independent subjects in triplicate. Data were analyzed by Student t-test (*p < 0.05 and **p < 0.01 for ABA effect, and # #p < 0.01 for genotype effect).
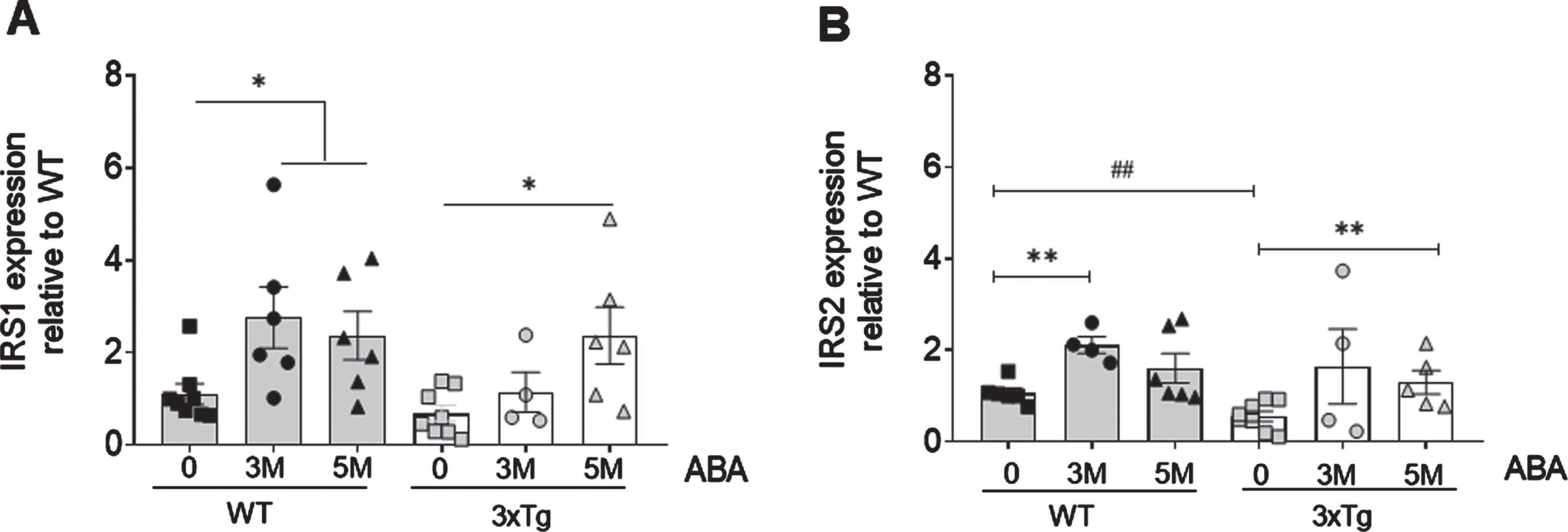
DISCUSSION
In this study, we confirmed that the 8-month-old 3xTg mice, a model of AD, displayed higher mRNA levels of TNFα and lower mRNA levels of BDNF than the wild type controls. We made use of our previously published model [13], where we had treated mice in two administration ways: the late-start and short ABA treatment; (3M-ABA) starting at 5 months of age; and early-start and longer ABA treatment (5M-ABA), starting at 3 months of age. 3M ABA was sufficient to significantly reduce the TNFα mRNA levels in the 3xTg mice. However, 5M ABA was required to increase BDNF mRNA levels. The relationship between BDNF and inflammation is bidirectional, and other studies have shown that reductions in inflammatory markers are a consequence of BDNF increase [16], in our study reduction of inflammation does not depend on BDNF mRNA levels. Because our previous studies have shown that memory improvement by ABA in this model requires early intervention [13], the present data are in line with the fact that lowering inflammation is not sufficient, but it is likely a mandatory step to rescue BDNF and cognitive function.
The mechanism by which ABA exerts its effects has not been completely elucidated, and some studies indicate that ABA may be a peroxisome proliferator-activated receptor gamma (PPAR-γ) agonist (brain tissue [17]; T-cells [18]); or indirectly ABA may activate PPAR-γ expression via LANC-2 (microglia cells [19]). However, other studies indicate that ABA’s function may be PPAR-γ-independent (macrophages) [20]. This discrepancy may be due to the different cell types analyzed, and it requires further research to fully elucidate ABA mechanism of action. Our data would be in accordance with ABA as a PPAR-γ agonist or activator as PPAR-γ can decrease the TNFα mRNA levels through NFkB activation and, in parallel, activate the BDNF promoter [21]. Moreover, this action is associated with improving brain injury, as demonstrated in a neuropsychiatric systemic lupus erythematosus [22], and with alleviating memory impairment in rodent models of neuroinflammation, either by LPS administration [23] or metabolic syndrome [14].
Furthermore, we analyzed the IRS1 and IRS2 mRNA levels, given the importance of insulin resistance in AD. We found that the IRS2 (but not IRS1) mRNA levels were reduced in the 8-month-old 3xTg mice compared to the wild-type controls. This is concomitant with the reduction in spatial memory that we had formerly observed in this model [13]. These data agree with the hypothesis stating that IRS2 deficiency could be the link between type II diabetes [24] and AD (for review, see [25, 26]). Moreover, these findings are in accordance with our previous studies in which we found that the IRS2, but not IRS1, gene expression was reduced in the brain of a rat metabolic syndrome model [14]. Interestingly, we also found that physiological aging is accompanied by a reduction in both the IRS1 and IRS2 levels [27], which agrees with the notion that AD accelerates aging pathology.
Although some investigations suggest that a reduction in IRS2 [28], and specifically in IGF1 R/IRS2 signaling [29, 30], can ameliorate AD, our current data are in line with other studies showing that IRS2 decline correlates with impaired spatial memory and emotional responses [31]. Furthermore, our findings agree with the pivotal role of IRS2 in NMDA-receptor-dependent synaptic transmission [32]. Moreover, the beneficial effects observed with ABA administration are supported by those reported for exendin (an anti-diabetic molecule) administration in a mice model of AD [33]. Furthermore, thiazolidinediones, which are known as PPAR-γ agonists, also increase IRS2 [34] and IRS1 [35] expression, which supports that ABA action mechanism may involve PPAR-γ. However, there is no evidence suggesting that PPAR-γ directly activates the IRS2 promoter, but it indirectly interacts with transcription factors that bind and regulate the IRS2 promoter (for example, transcription factor Sp1) [36]. Likewise, the IRS1 promoter can be activated by Sp1 [37], and we indeed observed that ABA also increased the IRS1 gene expression, both in the wild-type and 3xTg mice, which could agree with common activating mechanisms. Because 3xTg mice do not show brain IRS1 expression alteration compared to the wild type, it is unclear whether the ABA effect on IRS1 contributes to the improvement in the 3xTg mice memory function.
Interestingly, 3M ABA treatment elevated the IRS1 and IRS2 mRNA levels in the wild-type mice, whereas 5M ABA treatment were required to increase both IRSs expression in 3xTg mice, suggesting either a slower promoter activation or an inflammatory status that may hamper effective mRNA increase in these mice.
Finally, further studies are required to understand whether early intervention or the long exposure time is the key factor in ABA administration.
In conclusion, our data support that early ABA exposure is a promising intervention to reduce inflammation-induced brain damage in AD models, effectively targeting the regulation of key molecules (BDNF, IRS1/2), that are altered in AD.
ACKNOWLEDGMENTS
The authors want to thank the generous donations from the Association of Alzheimer Families, AFA, Castello.
FUNDING
This research was funded by university competitive grants; codes: UJI-B2018-01 and UJI-B2021-21 to A.M.S.-P.
CONFLICT OF INTEREST
The authors declare no conflicts of interest.
DATA AVAILABILITY
The data is available from a UJI repository (DOI: https://dx.doi.org/10.6035/UJI_B201801).
REFERENCES
[1] | Beason-Held LL , Goh JO , An Y , Kraut MA , O’Brien RJ , Ferrucci L , Resnick SM ((2013) ) Changes in brain function occur years before the onset of cognitive impairment. J Neurosci 33: , 18008–18014. |
[2] | Vinuesa A , Pomilio C , Gregosa A , Bentivegna M , Presa J , Bellotto M , Saravia F , Beauquis J ((2021) ) Inflammation and insulin resistance as risk factors and potential therapeutic targets for Alzheimer’s disease. Front Neurosci 15: , 653651. |
[3] | Berlanga-Acosta J , Guillén-Nieto G , Rodríguez-Rodríguez N , Bringas-Vega ML , García-del-Barco-Herrera D , Berlanga-Saez JO , García-Ojalvo A , Valdés-Sosa MJ , Valdés-Sosa PA ((2020) ) Insulin resistance at the crossroad of Alzheimer disease pathology: A review. Front Endocrinol (Lausanne) 11: , 560375. |
[4] | Qin W , Li F , Jia L , Wang Q , Li Y , Wei Y , Li Y , Jin H , Jia J ((2022) ) Phosphorylated tau 181 serum levels predict Alzheimer’s disease in the preclinical stage. Front Aging Neurosci 14: , 900773. |
[5] | Sánchez-Sarasúa S , Moustafa S , García-Avilés Á , López-Climent MF , Gómez-Cadenas A , Olucha-Bordonau FE , Sánchez-Pérez AM ((2016) ) The effect of abscisic acid chronic treatment on neuroinflammatory markers and memory in a rat model of high-fat diet induced neuroinflammation. Nutr Metab (Lond) 13: , 73. |
[6] | Moloney AM , Griffin RJ , Timmons S , O’Connor R , Ravid R , O’Neill C ((2010) ) Defects in IGF-1 receptor, insulin receptor and IRS-1/2 in Alzheimer’s disease indicate possible resistance to IGF-1 and insulin signalling. Neurobiol Aging 31: , 224–243. |
[7] | Steen E , Terry BM , Rivera EJ , Cannon JL , Neely TR , Tavares R , Xu XJ , Wands JR , De La Monte SM ((2005) ) Impaired insulin and insulin-like growth factor expression and signaling mechanisms in Alzheimer’s disease - Is this type 3 diabetes? J Alzheimers Dis 7: , 63–80. |
[8] | Das TK , Chakrabarti SK , Zulkipli IN , Hamid MRWA ((2019) ) Curcumin ameliorates the impaired insulin signaling involved in the pathogenesis of Alzheimer’s disease in rats. J Alzheimers Dis Rep 3: , 59–70. |
[9] | Edelmann E , Leßmann V , Brigadski T ((2014) ) Pre- and postsynaptic twists in BDNF secretion and action in synaptic plasticity. Neuropharmacology 76: , 610–627. |
[10] | Moosaie F , Mohammadi S , Saghazadeh A , Dehghani F , Id NR ((2023) ) Brain-derived neurotrophic factor in diabetes mellitus: A systematic review and meta- analysis. PLoS One 18: , e0268816. |
[11] | Porter GA , O’Connor JC ((2022) ) Brain-derived neurotrophic factor and inflammation in depression: Pathogenic partners in crime? World J Psychiatry 12: , 77–97. |
[12] | Gao L , Zhang Y , Sterling K , Song W ((2022) ) Brain-derived neurotrophic factor in Alzheimer’s disease and its pharmaceutical potential. Transl Neurodegener 11: , 4. |
[13] | Espinosa-Fernández V , Mañas-Ojeda A , Pacheco-Herrero M , Castro-Salazar E , Ros-Bernal F , Sánchez-Pérez AM ((2019) ) Early intervention with ABA prevents neuroinflammation and memory impairment in a triple transgenic mice model of Alzheimer’s disease. Behav Brain Res 374: , 112106. |
[14] | Ribes-Navarro A , Atef M , Sánchez-Sarasúa S , Beltrán-Bretones MT , Olucha-Bordonau F , Sánchez-Pérez AM ((2019) ) Abscisic Acid supplementation rescues high fat diet-induced alterations in hippocampal inflammation and IRSs expression. Mol Neurobiol 56: , 454–464. |
[15] | Choi SH , Bylykbashi E , Chatila ZK , Lee SW , Pulli B , Clemenson GD , Kim E , Rompala A , Oram MK , Aronson J , Zhang C , Miller SJ , Lesinski A , Chen JW , Kim DY , Praag H Van , Spiegelman BM , Gage FH , Tanzi RE ((2018) ) Exercise on cognition in an Alzheimer’s mouse model. Science 361: , eaan8821. |
[16] | Du Q , Zhu X , Si J ((2020) ) Angelica polysaccharide ameliorates memory impairment in Alzheimer’s disease rat through activating BDNF/TrkB/CREB pathway. Exp Biol Med (Maywood) 245: , 1–10. |
[17] | Kooshki R , Anaeigoudari A , Abbasnejad M , Askari-Zahabi K , Esmaeili-Mahani S ((2021) ) Abscisic acid interplays with PPARγ receptors and ameliorates diabetes-induced cognitive deficits in rats. Avicenna J Phytomed 11: , 247–257. |
[18] | Guri AJ , Evans NP , Hontecillas R , Bassaganya-Riera J ((2011) ) T cell PPAR γ is required for the anti-inflammatory efficacy of abscisic acid against experimental IBD. J Nutr Biochem 22: , 812–819. |
[19] | Maixner DW , Christy D , Kong L , Viatchenko-Karpinski V , Horner KA , Hooks SB , Weng HR ((2022) ) Phytohormone abscisic acid ameliorates neuropathic pain via regulating LANCL2 protein abundance and glial activation at the spinal cord. Mol Pain 18: , 17448069221107781. |
[20] | Bassaganya-Riera J , Guri AJ , Lu P , Climent M , Carbo A , Sobral BW , Horne WT , Lewis SN , Bevan DR , Hontecillas R ((2011) ) Abscisic acid regulates inflammation via ligand-binding domain-independent activation of peroxisome proliferator-activated receptor γ. J Biol Chem 286: , 2504–2516. |
[21] | Kariharan T , Nanayakkara G , Parameshwaran K , Bagasrawala I , Ahuja M , Abdel-Rahman E , Amin AT , Dhanasekaran M , Suppiramaniam V , Amin RH ((2015) ) Central activation of PPAR-gamma ameliorates diabetes induced cognitive dysfunction and improves BDNF expression. Neurobiol Aging 36: , 1451–1461. |
[22] | Li X , Xu S , Liu J , Zhao Y , Han H , Li X , Wang Y ((2022) ) Treatment with 1,25-dihydroxyvitamin D3 delays choroid plexus infiltration and BCSFB injury in MRL/lpr mice coinciding with activation of the PPARγ/NF-κB/TNF-α pathway and suppression of TGF-β/Smad signaling. Inflammation 46: , 556–572. |
[23] | Beheshti F , Hosseini M , Hashemzehi M , Soukhtanloo M , Khazaei M , Naser Shafei M ((2019) ) The effects of PPAR-γ agonist pioglitazone on hippocampal cytokines, brain-derived neurotrophic factor, memory impairment, and oxidative stress status in lipopolysaccharidetreated rats. Iran J Basic Med Sci 22: , 940–948. |
[24] | Brady MJ ((2004) ) IRS2 takes center stage in the development of type 2 diabetes. J Clin Invest 114: , 886–888. |
[25] | Athanasaki A , Melanis K , Tsantzali I , Stefanou MI , Ntymenou S , Paraskevas SG , Kalamatianos T , Boutati E , Lambadiari V , Voumvourakis KI , Stranjalis G , Giannopoulos S , Tsivgoulis G , Paraskevas GP ((2022) ) Type 2 diabetes mellitus as a risk factor for Alzheimer’s disease: Review and meta-analysis. Biomedicines 10: , 778. |
[26] | Rojas M , Chávez-Castillo M , Bautista J , Ortega Á , Nava M , Salazar J , Díaz-Camargo E , Medina O , Rojas-Quintero J , Bermúdez V ((2021) ) Alzheimer’s disease and type 2 diabetes mellitus: Pathophysiologic and pharmacotherapeutics links. World J Diabetes 12: , 745–766. |
[27] | Sánchez-Sarasúa S , Meseguer-Beltrán M , García-Díaz C , Beltrán-Bretones MT , ElMlili N , Sánchez-Pérez AM ((2022) ) IRS1 expression in hippocampus is age-dependent and is required for mature spine maintenance and neuritogenesis. Mol Cell Neurosci 118: , 103693. |
[28] | Killick R , Scales G , Leroy K , Causevic M , Hooper C , Irvine EE , Choudhury AI , Drinkwater L , Kerr F , Al-Qassab H , Stephenson J , Yilmaz Z , Giese KP , Brion JP , Withers DJ , Lovestone S ((2009) ) Deletion of Irs2 reduces amyloid deposition and rescues behavioural deficits in APP transgenic mice. Biochem Biophys Res Commun 386: , 257–262. |
[29] | Tanokashira D , Fukuokaya W , Taguchi A ((2019) ) Involvement of insulin receptor substrates in cognitive impairment and Alzheimer’s disease. Neural Regen Res 14: , 1330–1334. |
[30] | Freude S , Hettich MM , Schumann C , Stöhr O , Koch L , Köhler C , Udelhoven M , Leeser U , Müller M , Kubota N , Kadowaki T , Krone W , Schroder H , Brüning JC , Schubert M ((2009) ) Neuronal IGF-1 resistance reduces Aβ accumulation and protects against premature death in a model of Alzheimer’s disease. FASEB J 23: , 3315–3324. |
[31] | Tanokashira D , Wang W , Maruyama M , Kuroiwa C , White MF , Taguchi A ((2021) ) Irs2 deficiency alters hippocampus-associated behaviors during young adulthood. Biochem Biophys Res Commun 559: , 148–154. |
[32] | Costello DA , Claret M , Al-Qassab H , Plattner F , Irvine EE , Choudhury AI , Giese KP , Withers DJ , Pedarzani P ((2012) ) Brain deletion of insulin receptor substrate 2 disrupts hippocampal synaptic plasticity and metaplasticity. PLoS One 7: , e31124. |
[33] | Bomfim TR , Forny-germano L , Sathler LB , Brito-moreira J , Houzel J , Decker H , Silverman MA , Kazi H , Melo HM , Mcclean PL , Holscher C , Arnold SE , Talbot K , Klein WL , Munoz DP , Ferreira ST , Felice FG De ((2012) ) An anti-diabetes agent protects the mouse brain from defective insulin signaling caused by Alzheimer’s disease–associated Aβ oligomers. J Clin Invest 122: , 1339–1353. |
[34] | Smith U , Gogg S , Johansson A , Olausson T , Rotter V , Svalstedt B ((2001) ) Thiazolidinediones (PPARγ agonists) but not PPAR α agonists increase IRS-2 gene expression in 3T3-L1 and human adipocytes 1. FASEB J 15: , 215–220. |
[35] | Hammarstedt A , Smith U ((2003) ) Thiazolidinediones (PPARγ ligands) increase IRS-1, UCP-2 and C/EBPα expression, but not transdifferentiation, in L6 muscle cells. Diabetologia 46: , 48–52. |
[36] | Singh AK , Battu A , Mohareer K , Hasnain SE , Ehtesham NZ ((2010) ) Transcription of human resistin gene involves an interaction of Sp1 with peroxisome proliferator- activating receptor gamma (PPARγ). PLoS One 5: , e9912. |
[37] | Panno ML , Mauro L , Marsico S , Bellizzi D , Rizza P , Morelli C , Salerno M , Giordano F , Ando S ((2006) ) Evidence that the mouse insulin receptor substrate-1 belongs to the gene family on which the promoter is activated by estrogen receptor α through its interaction with Sp1. J Mol Endocrinol 36: , 91–105. |