Candesartan Effectively Preserves Cognition in Senescence Accelerated Mouse Prone 8 (SAMP8) mice
Abstract
Background:
Alzheimer’s disease (AD) has become a worldwide crisis with no effective therapeutic options. The medications currently available for AD are only palliative; their effect is temporary, and they are associated with unfavorable side effects. Even the newest medication aducanumab, granted accelerated FDA approval in 2021, failed to show cognitive benefits in clinical trials and continued approval requires verification in subsequent clinical trials. There is an urgent need for safe and effective therapies to preserve cognition and effectively manage AD. Generally, a new drug product takes several years for FDA approval and exceeds 2.5 billion dollars in research and development, with most new drug products never even reaching the market. This has led to a recent shift for repurposing/repositioning existing FDA-approved medications, to new therapeutic indications.
Objective:
To investigate the effects of long-term treatment with candesartan, an FDA-approved angiotensin-II type-1 receptor blocker (ARB), on the development of cognitive impairment associated with premature aging.
Methods:
Candesartan was given at a dose of 1 mg/kg/d in an AD model of senescence-accelerated mouse prone-8 (SAMP8) and senescence-accelerated mouse resistant (SAMR1) mice. Oral treatment with candesartan or vehicle was started, in 2-month-old mice and administered continuously for 4-months.
Results:
Low-dose candesartan prevented the development of cognitive impairment, otherwise associated with accelerated aging, in SAMP8 mice, by reducing inflammation and nitro-oxidative stress. Candesartan did not affect the cognitive function of control SAMR1 mice.
Conclusion:
Early ARB treatment might be beneficial in preventing age-related cognitive deficits in AD-prone individuals.
INTRODUCTION
Dementia is most often the result of Alzheimer’s disease (AD), an irreparable, incurable neurodegenerative condition that increases exponentially despite all attempts to overcome it. AD is currently the only leading cause of death that continues to rise, with total costs surpassing cardiovascular disease and cancer combined [1]. Unfortunately, all the medications currently approved for AD do not reverse or delay the disease’s progressive nature. Such agents serve only as palliative measures, their efficacy is reduced over time, and they are often associated with unfavorable side effects [1].
Even the newest medication aducanumab (Aduhelm), a monoclonal anti-amyloid antibody available as an intravenous infusion and recently granted accelerated FDA approval in 2021 [2]. This agent was authorized for use based merely on its ability to reduce amyloid-β (Aβ), a “surrogate endpoint” deemed likely to predict clinical benefit, and not on actual “functional” criteria like cognition. It is also associated with a high incidence (≥10%) of adverse reactions, including but not limited to headaches, brain edema, and microhemorrhages. Continued FDA approval of this agent may be contingent upon verifying clinical benefit in confirmatory trials [2]. This is especially important given the disappointing results of this and similarly novel drug products targeting Aβ in exorbitant, high-profile clinical trials [3].
All efforts to develop new and more effective treatments for AD have been unsuccessful thus far and have led a large portion of the research community to investigate licensed drugs with potential pharmacological effects applicable to neurodegenerative diseases. This strategy of repurposing existing medications currently approved by the FDA to new therapeutic indications, also referred to as “drug repurposing,” has been successful in many other areas, including cancer and cardiovascular diseases, and effectively minimizes wasted time, effort, resources, and cost [1, 4].
Angiotensin II type 1 receptor (AT1R) blockers (ARBs) are a class of drugs used for the prevention and treatment of cardiovascular disease and have also been shown to protect against cognitive impairment in various clinical and experimental settings [1]. We and others have shown that the positive effect of ARBs on cognition occurs directly by selectively blocking the AT1R and indirectly, by allowing an unopposed stimulation of the neuroprotective AT2R pathway, by the unbound Ang II [1, 5, 6].
While ARBs share a distinctive imidazole pharmacophore responsible for their common “class effect,” this group is chemically heterogeneous. This is mainly the result of the different substituents, which result in varying physicochemical properties and pharmacokinetic profiles. Candesartan is an FDA-approved ARB indicated for the treatment of hypertension in both adults and children and also for heart failure [7]. It has the strongest AT1R blocking properties of its class [8] and was selected for our study based on its myriad of advantages, including but not limited to its ability to cross the blood-brain barrier (BBB) [9] in addition to the extensive data supporting its benefits in cognition [1].
This ARB effectively preserved cognitive function in young [5] and aged [10] hypertensive animals post-ischemic insult. Even when drug initiation was delayed [5, 10]. The cognitive benefits of candesartan went far beyond its effect on BP, as these were also seen with low sub-hypotensive doses [5]. Candesartan also prevented the cognitive deterioration resulting from traumatic brain injuries [11, 12], as well as that induced by chronic stress [13] and diabetes [14]. This current study is the first to investigate the long-term effects of candesartan on the development of cognitive impairment associated with premature aging.
The greatest known risk factor for AD is aging, and the majority (∼50%) of people with AD are age 65 and older. In this study, we chose to use senescence-accelerated mouse prone 8 (SAMP8) and senescence-resistant control (SAMR1) mice. The SAMP8 is a spontaneous mouse model that displays age-related learning and memory disorders similar to AD [15–17]. The phenotype of this particular inbred strain (SAMP8) closely mirrors many of the complex cognitive symptoms, behavioral traits, and neuropathological features of individuals with sporadic AD (sAD) [15–17]. SAMP8 animals undergo accelerated brain/CNS aging and possess a relatively short lifespan (average ≈ 9.7 months) with an early onset of cognitive deficits and emotional disturbances [16]. Therefore, it is particularly well suited to study the “transitional switch” between aging and AD. This model of cognitive aging is not only well established; it has even been suggested to represent the complexity of the human disease much more accurately than any other model, including transgenic mouse lines, because of the multifactorial nature of clinical AD [16, 18]. The SAMR1 strain was used to serve as a normal aging control. This strain has a background similar to SAMP8 but does not develop the accelerated brain/CNS aging or cognitive symptoms seen in SAMP8 animals and is the representative control strain for pre-clinical studies employing SAMP8 animals [15–17]. Oral treatment, with candesartan or vehicle, was started in 2-month-old animals of both sexes (an early stage at which there are no apparent cognitive differences between the strains) and continued for 4 months [16]. Cognitive function was monitored using spatial learning, short-term/working memory, and long-term reference memory (aspects of cognition known to diminish with advanced age) tests.
MATERIALS AND METHODS
Experimental design
A total of 50 young adults, 2 months old, SAMP8/SAMR1 mice of both sexes, breeding pairs obtained from (Envigo “Formerly Harlan” Laboratories, Inc.) were housed under standard conditions (temperature 21–25°C, humidity 45–50%) on a 12:12 h light-dark cycle. Male and female animals were housed in separate cages. These animals had free access to food and water and were randomly assigned to receive daily candesartan cilexetil (TCV-116) or vehicle (5% Arabic gum) for 4 months before starting experiments. Treatments were incorporated into the drinking water and adjusted according to the animals’ average daily intake of water, which was monitored periodically to provide them with a 1 mg/kg/d dose of candesartan (Fig. 1), a dose previously established to provide cognitive benefits in aged animals [10, 19]. Treatments were prepared in a blinded manner as previously described [10]. The complete set of data was reported and included in the final data analyses for each animal that survived and completed the full course of treatment and all the necessary behavioral tests required for this study. Experiments were conducted by the U.S Public Health Service Policy on Humane Care and Use of Laboratory Animal guidelines [10]. Moreover, all procedures were approved by the Institutional Animal Care and Use Committee (IACUC, Protocol # 20-0204.0) at the University of Tennessee Health Sciences Center. Functional (cognitive/behavioral) outcomes were assessed using validated, reproducible tests and reported by the most recent ARRIVE guidelines 2.0 (Animal Research: Reporting of in Vivo Experiments-Updated guidelines for reporting animal research) [20, 21].
Fig. 1
Schematic Depiction: Overall Experimental Design. This was a long-term pre-clinical study designed to investigate the effects of continuous treatment with Candesartan on cognitive function, inflammatory mediators, and oxidative biomarkers in an age accelerated model of sAD.
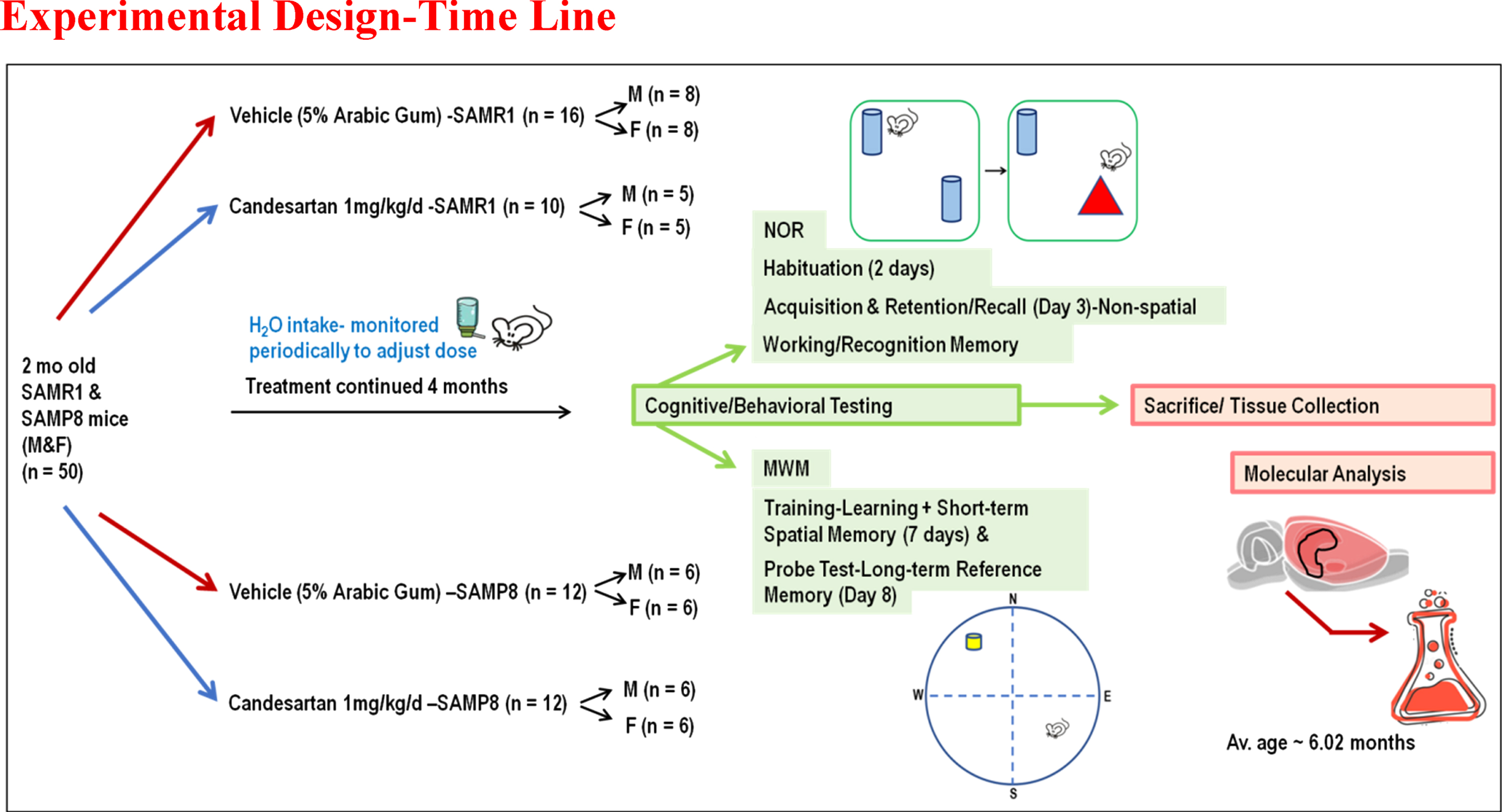
Memory, cognitive, and neurobehavioral testing
AD is fundamentally a clinical diagnosis, with functional (cognitive/neurobehavioral) outcomes serving as the primary criteria (cornerstone for diagnosis) in clinical practice [1, 22]. Moreover, large clinical studies currently focus on behavioral and functional outcomes as the main measure of therapeutic success [1, 22]. Our focus, therefore, centers on pre-clinical applicable measures, using tests established to simulate those employed in the clinic. Behavioral tests were initiated 4 months after continuous treatment and performed under standard conditions during the animal’s natural active cycle to ensure reliable results [23]. The testing sequence was selected based on recommendations of a neurobehavioral specialist in a way designed to minimize task interference, such that tasks with aversive components, i.e., Morris Water Maze (MWM) training sessions and probe test, used for assessing spatial learning and long-term/reference memory respectively, were conducted after the non-aversive Novel Object Recognition (NOR) test of non-spatial working memory [23].
The Novel Object Recognition (NOR) test
The NOR test evaluated non-spatial, short-term working memory [24]. This test is based on the natural tendency of an animal to explore and interact with a novel object more than a familiar one. It consists of two trials separated by a retention period and preceded by a habituation phase. The latter was conducted before the start of the test to allow animals to acclimatize to their arena. On the designated test day, each animal was first introduced into the arena (standard size 45×45×35 cm), containing 2 identical sample objects (acquisition/sample trial), and allowed to explore these for 10 min. Following sample object exposure, the animal was returned to its home cage for a 1-h retention period. The 2nd preference/test trial (5 min), which follows the retention period, was conducted the same way as the 1st trial, except that a new/novel object replaced one of the familiar/sample objects. All tests were recorded, and video tracked by Etho-Vision XT 7 automated tracking system (Noldus, Leesburg, VA, USA). The time spent exploring each object during the preference/test trial was computed by the software and used to calculate the 1) Discrimination index (DI), which is the difference in exploration time for the different objects divided by the total time of exploration, as well as the 2) Recognition index (RI), which is the time spent exploring the novel object relative to the total time of exploration, both of which are established indicators of working memory.
T N and T F are the times spent exploring the novel and familiar object, respectively. The greater the value for DI or RI, the better the memory [5, 10, 24, 26].
The Morris Water Maze (MWM)
The MWM was used to assess spatial learning and long-term/reference memory, and motor performance. All water maze tests were conducted in a large circular pool of water, 120 cm in diameter, 55 cm in height, filled to a depth of 35±1 cm with water, made opaque using non-toxic white tempera paint (Crayola) and maintained at 25±2°C. The pool was separated into quadrants designated northeast (NE), northwest (NW), southeast (SE), and southwest (SW), based on the 4 equally spaced cardinal points N (North), S (South), E (East), and W (West) around the edge of the pool. These quadrants (NW) contained a transparent escape platform (10.5 cm diameter), submerged 1.5 cm below the water surface and obscured from view. The platform remained in the same quadrant during the entire experiment. Visual extra-maze cues were mounted to aid spatial navigation. Learning/acquisition and spatial reference memory/consolidation were assessed with daily training sessions followed by a standard place probe test. The probe test was conducted 24 h after the last daily training session; all procedures were kept the same, but the platform was removed, and mice were allowed to swim for 60 s to find it. All animals’ swim patterns were monitored, and video recorded by ANY-maze (Stoelting, Wood Dale, IL, USA) computerized tracking software. The tracking software calculated the desired assessment parameters, which were evaluated and compared between treatment groups at different time points. Performance in the training sessions was assessed by the mean escape latency time to find the platform (s), which is inversely proportional to cognitive performance. Spatial reference memory/ consolidation was assessed by measuring the number of entries into the target quadrant and the time spent in the target quadrant/zones (higher values indicate better outcomes) [10, 27].
BP measurements (SAM animals)
Systolic blood pressure (SBP) and mean arterial pressure (MAP) were measured in conscious animals by a non-invasive tail-cuff plethysmography technique using the CODA® HT System with 6 activated channels (Kent Scientific, Torrington, CT, USA). BP was measured at the same time of day in both the control and experimental groups to avoid circadian effects. During blood measurement, mice were restrained on heating pads maintained at 35–37°C. According to the manufacturer’s protocol, heart rate and blood pressure were continuously recorded using volume pressure recording (VPR) sensor technology, with 10 successive measurements taken to calculate an average value [28].
Animal sacrifice and tissue collection
Animals were anesthetized, and their brains were collected for biochemical determinations/quantitative assays as described previously [10, 27].
Protein expression
Protein expression of a standard panel of inflammatory mediators (IFN-γ, IL-1β, IL-2, IL-4, IL-5, IL-6, KC/GRO, IL-10, IL-12p70, TNF-α) was achieved using a species-specific MSD V-plex, multi-array proinflammatory electrochemiluminescence detection kit (Catalog # K15048D-1) This kit has been used by multiple groups [29–32], and was used for the simultaneous measurement of multiple inflammatory biomarkers in hippocampal lysates according to the manufacturer’s protocol.
Western blotting
Brain/tissue samples were homogenized and processed for western blotting, as previously described [27]. The primary antibodies included TRX (1:1,000; Cell signaling) and β-actin (1:2,000 A5316, Sigma) with the respective horseradish peroxidase-conjugated secondary antibody (1:10,000, Sigma Sigma-Aldrich, St. Louis, MO). Protein levels and integrated density/area were analyzed using Image J software (NIH, Bethesda, MD) and normalized to β-actin/loading control [27].
Slot/dot blot analysis
Mouse hippocampal lysates containing equivalent amounts of total protein (20μg) were spotted onto a nitrocellulose membrane, enclosed within a slot blot microfiltration unit as described in the previous report with slight modifications [27]. The primary antibodies specific for the desired proteins are either anti-nitrotyrosine (1:1000, Millipore, Saint Louis, MO, USA, catalog#05-233) or anti-4-hydroxynonenal (4-HNE) (1:1000, StressMarq Biosciences Victoria, BC V8N, Canada; catalog# SMC-511), with their respective horseradish, peroxidase-conjugated 2° antibodies (1:10,000, Sigma Sigma-Aldrich, St. Louis, MO) were used. Protein levels and integrated density/area were analyzed using Image J software (NIH, Bethesda, MD) and normalized to total protein [33, 34].
Statistical analysis
All statistical analyses were completed using the GraphPad/Prism 8.0 software, with statistical significance assessed at an alpha level of 0.05, unless otherwise specified. Cognitive/functional outcomes, molecular parameters, and behavioral data/measures among the experimental groups were evaluated by one-way analysis of variance (ANOVA) at specific time points, followed by Tukey-Kramer multiple comparison tests to examine post hoc pair-wise differences. Descriptive statistics for behavioral data measures in animals, within-group, and at various indicated measurement times were determined and expressed as mean±SEM. For MWM learning/training sessions, a 2-way repeated-measures ANOVA/lot led model, with Greenhouse-Geisser correction, was used to examine differences in learning patterns between the 4 groups over time [10].
RESULTS
Candesartan did not affect the body weight trajectory of SAMR1 or SAMP8 mice
Animal body weight remained relatively stable, for each strain, throughout the full duration of the study. Although SAMP8 animals showed overall lower body weight than age matched SAMR1 animals, this trend was not affected by candesartan treatment in either of the 2 strains at any time (Fig. 2A). Moreover, mean fluid intake was consistent between groups throughout the study and ranged between 0.15–0.2 mL/g/day.
Fig. 2
Effect of strain (SAMR1 versus SAMP8) and long-term candesartan treatment on (A) body weight, (B, C) blood pressure, and (D, E) motor performance. Repeated measures ANOVA/mixed models were used to examine differences in body weights between the 4 groups over time, with statistical significance for post hoc pair-wise comparisons, denoted by *p < 0.05. Systolic and mean arterial blood pressures were obtained by non-invasive tail-cuff plethysmography, and differences between the groups were assessed by one-way analysis of variance with a Tukey-Kramer multiple comparisons test used to examine post hoc pair-wise differences. This was also used to compare differences in swim speeds, and total distance traveled on the MWM test for motor performance (n ≈ 10 animals/group,±SEM, *p < 0.05).
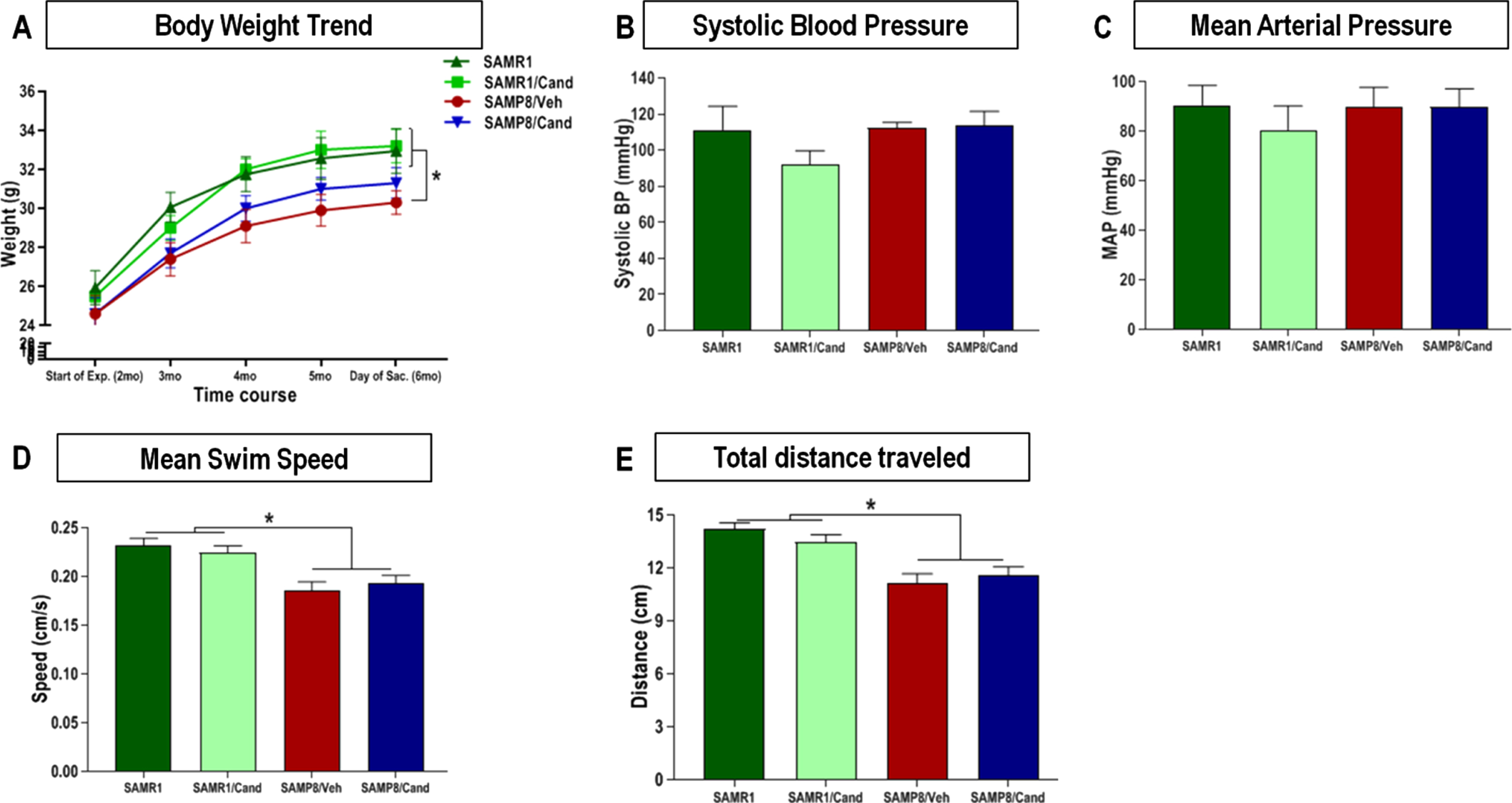
Candesartan did not affect the BP of SAMR1 or SAMP8 mice
There were no differences in blood pressure between SAMR1 and SAMP8 animals at 6 months of age. Moreover, SAMP8 and SAMR1 animals treated long-term with candesartan (1 mg/kg/day) had similar systolic blood pressure (SBP) and mean arterial pressure (MAP) readings (Fig. 2B, C), as measured by non-invasive tail-cuff plethysmography as those of vehicle-treated controls. BP ranges were very similar among all 4 groups, irrespective of strain or treatment.
Candesartan did not affect the motor function of SAMR1 or SAMP8 mice
All 4 groups appeared to have dexterous limb movement and a relatively comparable overall motor performance, including an ability to effortlessly climb the escape platform in addition to ambulating an open arena with excellent coordination and appropriate gait. Nevertheless, SAMP8 animals displayed significantly lower swim speeds and total distance traveled (Fig. 2D, E) on the MWM probe test compared with age matched SAMR1animals. This was in line with the findings of others [35] and was not affected by candesartan.
Candesartan prevented declines in the non-spatial, short-term working memory of SAMP8 mice
SAMP8 animals had significantly lower recognition and discrimination indices, on the novel object recognition test, compared to age matched SAMR1 animals (Fig. 3). This defect in non-spatial working memory was not apparent in SAMP8 animals continually treated with candesartan. These candesartan-treated animals showed a higher affinity for the novel object during the preference test and had RI and DI values similar to those of control SAMR1 animals, indicating preserved non-spatial working memory. Although candesartan resulted in benefits in SAMP8 animals, it had no significant effects on the non-spatial working memory of control SAMR1 mice.
Fig. 3
Effect of long-term candesartan treatment on non-spatial, short-term working memory in SAMR1 and SAMP8 mice. A) Recognition index (RI) - time spent exploring the novel object relative to the total time of exploration. B) Discrimination index (DI) – the difference in exploration time for the different objects to the total time of exploration. C) Heat Maps illustrating relative time spent in the various locations during the retention test. The novel object is located within the white-rimmed area. TF and TN are the times spent interacting with the familiar and novel object, respectively (n ≈ 10 animals/group,±SEM, *p < 0.05).
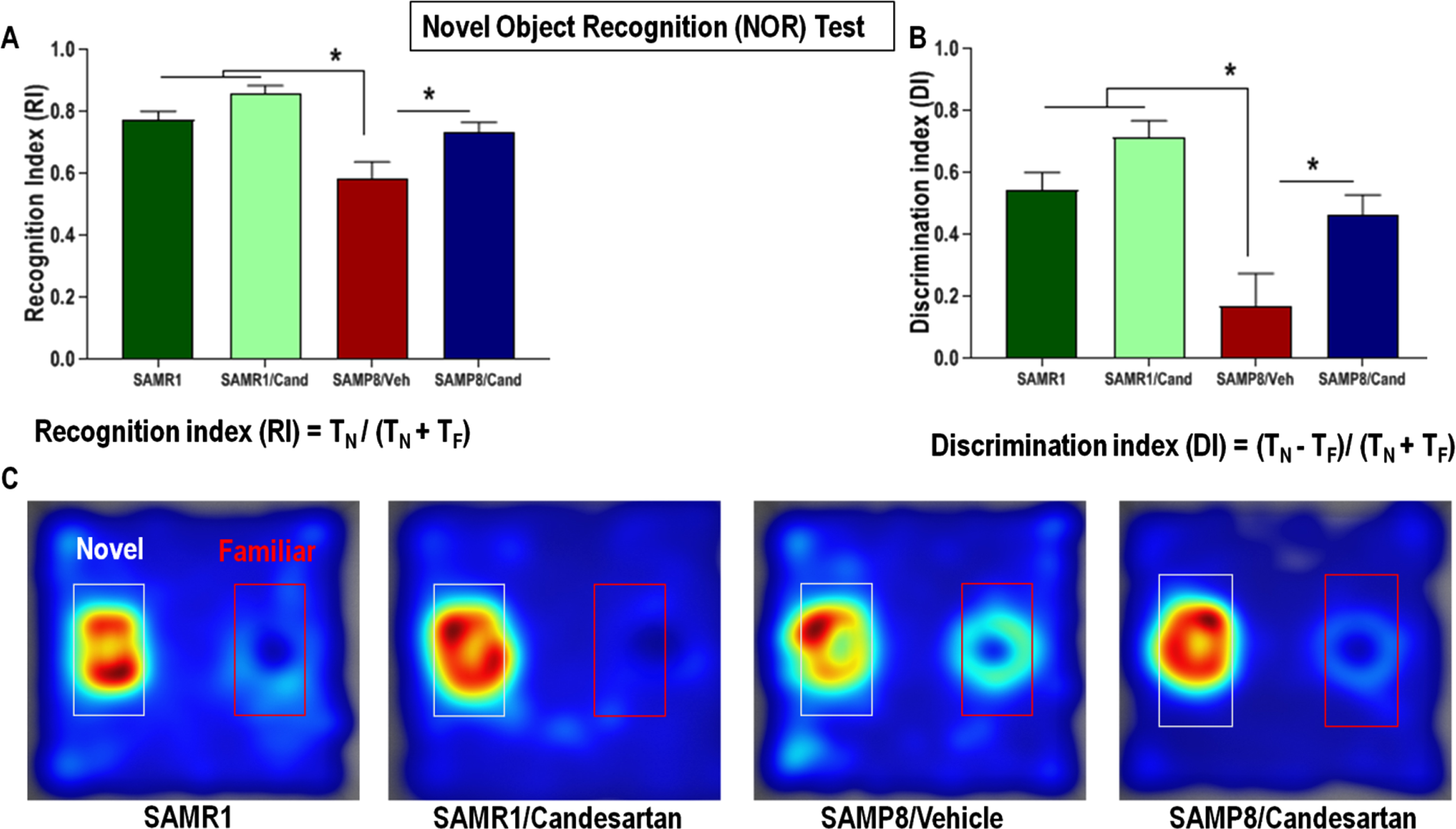
Candesartan facilitated learning & improved the spatial working memory of SAMP8 mice
SAMP8 animals showed severely impaired learning & short-term working memory. This was indicated by significantly higher mean escape latencies on MWM training days 3, 4, 5, and 6 compared to control SAMR1 mice (Fig. 4A). Such cognitive deficits were not apparent in SAMP8 animals continually treated with candesartan. These animals acquired the paradigm within the first few sessions and demonstrated the classic learning curve, characterized by a gradual reduction in the mean escape latency over the 6-day training period, with significantly improved latencies on the final days of training compared to untreated animals. Most of the candesartan treated SAMP8 animals showed target latencies that were not much different from those of control SAMR1 animals at any point in time. Candesartan treatment did not affect the learning and memory of control SAMR1 mice.
Fig. 4
Effect of long-term candesartan treatment on learning, spatial working memory, and long-term/ reference memory retrieval in SAMR1 and SAMP8 mice. A) Learning and spatial working memory were evaluated by animals’ learning curve, the number of sessions it took for animals to acquire the paradigm and use the surrounding cues for spatial navigation of the maze to locate the hidden platform during the training period. The animal is introduced into the maze from various positions and relies on its working memory to reach the target. Long-term reference memory was determined by the probe test. Performance was evaluated by measuring the (B) time spent in the target quadrant as well as (C) number of target entries. D) Heat maps illustrating relative time spent in the various locations during the probe test (n ≈ 10 animals/group). Symbols and error bars indicate mean±SEM. One-way ANOVA or repeated-measures ANOVA mixed models were used to examine differences in outcomes between the 4 groups over time for the 6-day training, with statistical significance for post hoc pair-wise comparisons, denoted by *p < 0.05.
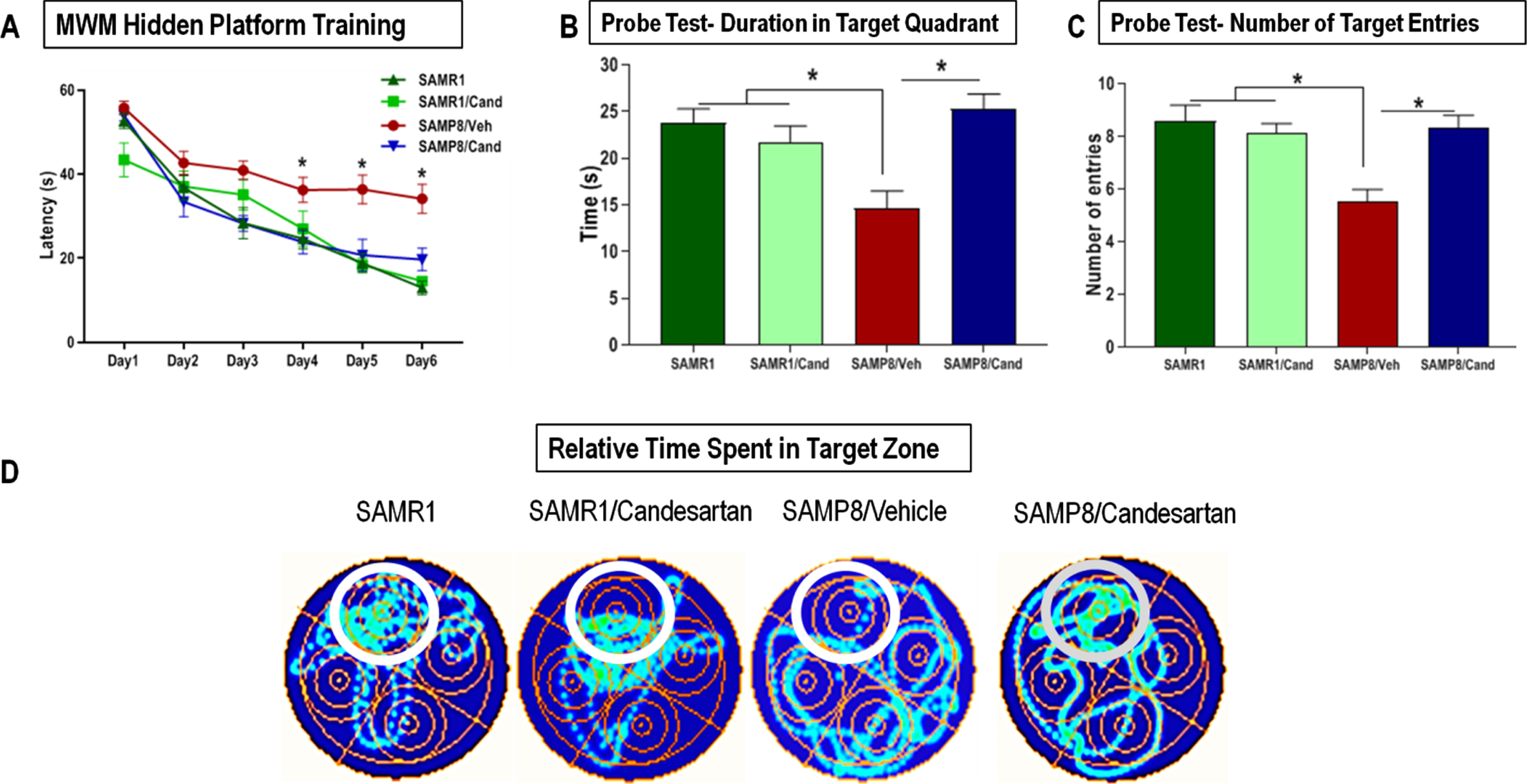
Candesartan promoted long-term/reference memory consolidation in SAMP8 mice
To assess long-term/reference memory consolidation, an MWM probe test was conducted 24 hours after the final training session. Untreated SAMP8 animals failed to remember the location of the escape platform and hence spent significantly less time (cumulative duration) in the target quadrant compared to both candesartan treated SAMP8 animals and SAMR1 controls (Fig. 4B). These animals also showed a considerably lower number of target entries than all other groups (Fig. 4C). On the other hand, the overall performance of SAMP8 animals treated with candesartan was no different from controls. SAMR1 animals treated with or without candesartan performed similarly on the MWM probe test, indicating a lack of effect of candesartan on the cognitive function of SAMR1 mice.
Candesartan mitigated the oxidative stress and inflammation associated with accelerated aging in SAMP8 mice
SAMP8 animals showed significantly lower expression levels of the antioxidant thioredoxin-1 (TRX1) (Fig. 5A) with a consequential increase in oxidative stress markers (nitrotyrosine, 4-HNE) in the hippocampus compared to age-matched SAMR1 animals (Fig. 5B, C). These animals also showed overall elevations in the level of proinflammatory cytokines IL-1β, KC-GRO, TNFα, IL-6, and IL-12p70 from a standard panel (Fig. 5D-H), a specific pattern of inflammatory cytokine expression that is similar to that noted for other models of AD [36, 37], These negative associations were not apparent in SAMP8 animals treated continually with candesartan.
Fig. 5
Effect of long-term candesartan treatment on markers of oxidative stress and inflammation in SAMR1 and SAMP8 mice. Western blot and dot blot analyses for oxidative markers (A) TRX, (B) nitrotyrosine, and (C) 4HNE, respectively, along with comprehensive multiplex analyses for quantification of proinflammatory cytokine levels as part of a standard panel (D) IL-1β, (E) KC/GRO, (F) TNF-α, (G) IL-6, and (H) IL-12p70 in SAMR1 and SAMP8 animals treated with or without candesartan (n ≈ 10 animals/group,±SEM. *p < 0.05).
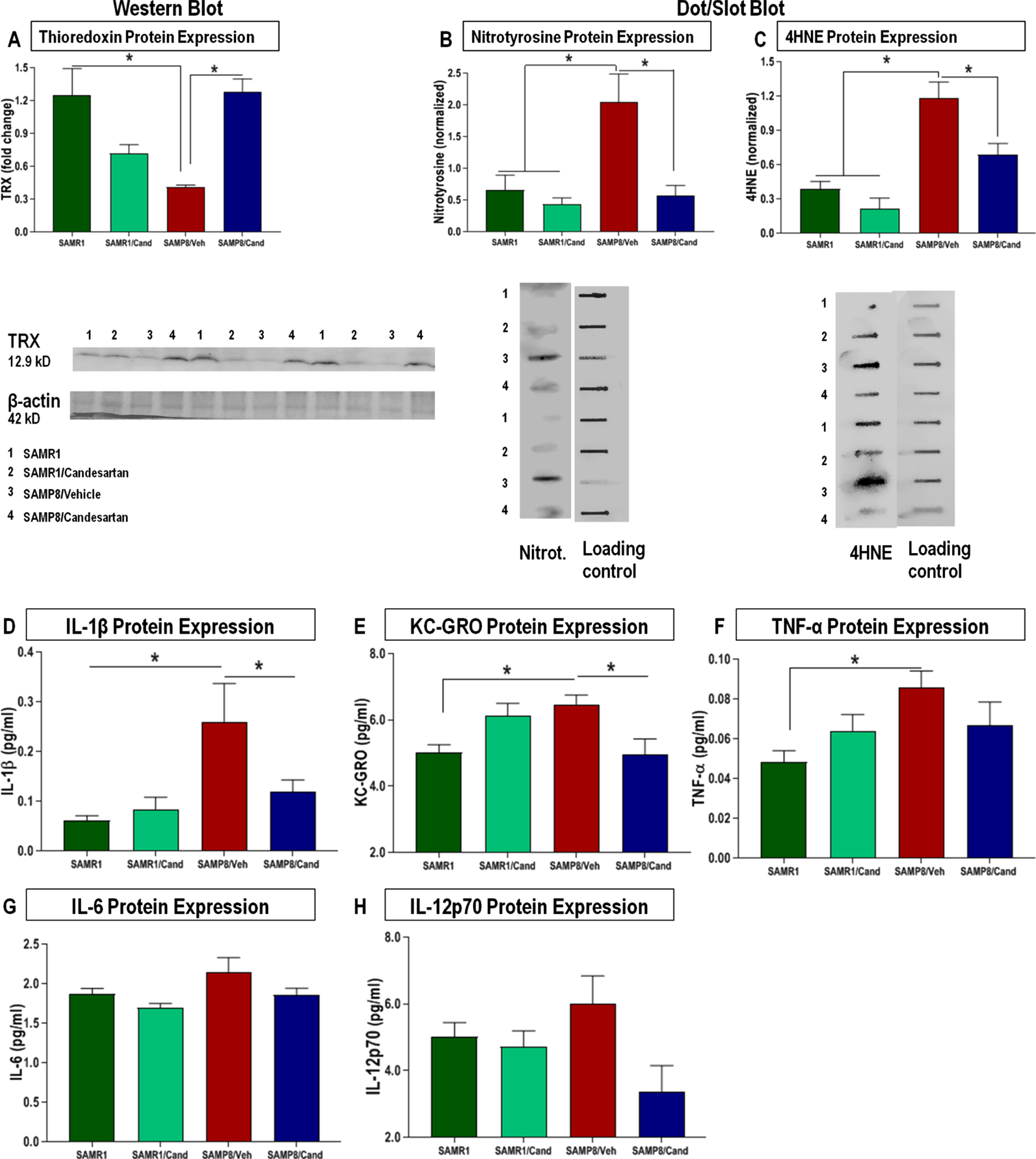
DISCUSSION
This was the first long-term (4 months) pre-clinical study to evaluate the effects of candesartan on cognitive function and relevant molecular markers in SAMP8 and SAMR1 animals of both sexes. AD is a chronic, progressive disease that generally requires long-term, if not life-long treatment. As is the case with most chronic conditions, including cardiovascular diseases like hypertension and coronary artery disease (common in older individuals). Therefore, for a medication to be deemed safe and effective in preclinical trials (animal models), it should be given in such a way to best simulate its intended use in actual patients. We utilized this unique strain as it precisely depicts several of the complex behavioral, biochemical, and pathological features of sAD, including progressive cognitive decline, chronic, low-grade neuroinflammation, elevated oxidative stress, and disturbances in synaptic plasticity [17, 38]. Indeed, this strain follows the clinical AD-like trajectory much more closely than any other animal model [17, 38]. We also noticed a particularly interesting observation, apparent in male SAMP8 animals. These animals displayed continued aggressive behavior that appeared to worsen with age. These animals would fight incessantly and make harsh noises. They were put under strict observation, but when the aggression got out of hand, signs of blood in the cage, or flesh wounds to their back/buttocks, they had to be separated and singly housed. This is comparable to some of the behavioral, affective symptoms common in patients with AD [39, 40]. Such symptoms, now classified as behavioral and psychological symptoms of dementia, directly impact social functioning and quality of life, often resulting in early institutionalization [40, 41]. It is essential to note that the major obstacle limiting the translatability of basic research has been the lack of an appropriate animal model that effectively replicates what takes place clinically. Most rodent models of AD utilize transgenic animals with specific gene mutations. This does not come close to accurately simulating the true complexity and multifactorial nature of the human disease, which directly results from multiple intricate interactions among genetic, epigenetic, and environmental factors [38, 40]. Most pre-clinical/experimental studies also tend to avoid female animals, given the complexity of the female estrous cycle and the lack of robust memory changes in female animals compared to males [42, 43]. We believe that this is another important reason for failed translation, especially considering the high prevalence of AD in female patients [42, 43]. We included animals of both sexes in our study and incorporated both functional cognitive/ behavioral and molecular outcomes to assess drug efficacy.
Growing evidence suggests that renin-angiotensin system (RAS) modulators support cognitive function in various animal models age-related Impairment in learning but not memory in SAMP8 female mice [1]. However, little is known about their long-term effects in the unique SAMP8; age accelerated model of spontaneous AD. In the current study, we extend these findings by confirming the positive effects of candesartan, a special type of RAS modulator/ARB in the SAMP8 animal model. Candesartan, indicated for use in the prevention and treatment of cardiovascular disease, has the greatest documented BBB permeability [9] AT1R selectivity and strongest antagonist properties of all agents in its class [8]. It was also selected based on these properties and the extensive data supporting its benefits in cognition [1, 5, 6, 10, 13, 14, 44, 45].
We observed no differences in BP between the two strains (SAMR1 and SAMP8) of mice at 6 months. This was comparable to what others found in SAMR1 and SAMP8 animals ranging from 2–7 months of age and was consistent regardless of the technique used to determine BP; the tail-cuff method [46] and continuous BP telemetry [47] gave similar results. However, in the study, we initiated oral treatment with candesartan in SAMR1 and SAMP8 animals to show that candesartan, given at a daily dose of 1 mg/kg, does not affect the BP of either strain of animals. BP readings as measured by non-invasive tail-cuff plethysmography were very similar among all four groups, irrespective of strain or treatment.
Our results also show that SAMP8 animals had lower body weights than age matched SAMR1 animals. This was consistent with other findings [48] and was not significantly affected by the candesartan (ARB) treatment.
SAMP8 animals displayed significant cognitive decline compared with age-matched SAMR1 controls, which is similar to the findings of others [35, 37]. These animals failed to attain an adequate level of performance during MWM training sessions, which are known to evaluate learning, consolidation, and short-term spatial working memory. They also performed significantly worse on the probe test of hippocampal-dependent long-term reference memory than age-matched SAMR1 controls animals. This was also true with the NOR test, as with other studies [38, 49]. This NOR test is relatively quick and easy to implement, making it widely used for assessing cognitive deficits in pre-clinical research [1].
However, we show that candesartan prevented this cognitive decline, improved consolidation, and expedited memory retrieval in SAMP8 animals without affecting cognitive function in SAMR1 animals. Indeed, SAMP8 animals treated with candesartan showed superior performance on all cognitive tests than vehicle-treated animals. Their level of performance did not differ from that of healthy SAMR1 controls on any of the cognitive measures. They performed similarly to healthy age-matched SAMR1 controls, with evident long-term retention (entered the target zone more frequently and spent more time in the target quadrant) and rapid retrieval (low latencies) not seen with their vehicle-treated counterparts.
Candesartan-treated animals also maintained adequate brain levels of TRX in the hippocampus compared to those treated with vehicles. This is an important finding, given the significant role of TRX, a multifunctional ROS scavenger protein and neuroprotective compound, abundant in healthy neurons but greatly reduced in the hippocampal region of patients with AD [50, 51]. Such reduced levels of TRX correlated with increased production of oxidative products, mainly 4HNE., a product of lipid peroxidation and neurodegeneration as well as nitrotyrosine, both of which result in cumulative oxidative damage to cellular structures [50, 51]. We found that animals treated long-term with candesartan not only had significantly lower levels of these harmful oxidative by-products compared to vehicle-treated animals, but this was also true with the inflammatory markers IL-1β, KC-GRO, IL-6, and TNF-α. These markers, which are known to increase with age in the brains of SAMP8 mice [52], showed a consistently lower trend in animals treated with candesartan.
Therefore, candesartan appears to preserve cognition in this model at least in part by reducing inflammation and oxidative damage. This is further supported by previous findings noting that candesartan promotes microglial polarization from the M1 (pro-inflammatory) to the M2 (anti-inflammatory) phenotype at least in part by a TLR4/NF-κB-dependent signaling pathway [53]. Where it not only reduced the expression of M1 and increased the expression of M2 markers, but it also regulated the neuroinflammatory response directly by reducing the release of proinflammatory cytokines TNF-α and IL-1β and increasing anti-inflammatory cytokines, which exert neuroprotective effects [53]. Candesartan may also preserve cognition by maintaining BBB integrity and function. Studies showed that ARBs reduce BBB permeability independently of blood pressure changes [54]. This is because ARBs block the action of angiotensin II, which otherwise stimulates the production of proinflammatory cytokines and activates matrix metalloproteinases (MMPs), which mediate TJ disruption and increase BBB permeability, ultimately leading to cognitive dysfunction. Therefore ARBs like candesartan, by its antioxidant and anti-inflammatory properties, resulting in inhibition in MMP-9 expression, thereby restoring TJs, claudins/ occludins, and collagen-IV (essential components required for proper BBB function) [54].
Conclusion
This proof-of-concept study indicates that continuous candesartan treatment preserves cognition and improves long-term functional capacity in the SAMP8 mouse model of AD. Considering that aging is the single greatest risk factor for AD, the present study results suggest that candesartan may preserve cognition in older individuals at high risk of developing AD [55]. Further investigations are needed to confirm whether candesartan treatment could be promising for age-associated dementia and AD mouse models (5XFAD, rTg4510, and APP/PS1). Although the exact molecular mechanism is not completely understood, it appears to be a result of candesartan’s anti-inflammatory and anti-oxidative activity. Therefore, further studies are needed to look at possible mechanistic effects of candesartan, antioxidant and anti-inflammatory signaling, including nuclear factor-erythroid factor 2-related factor 2 (Nrf2).
Summary
The most important finding of our investigation is that continuous administration of candesartan effectively prevented the development of cognitive impairment in an age accelerated model of spontaneous AD. This was independent of effects on other functional outcomes, including blood pressure or motor function, and was consistent across a series of blinded tests, assessing different aspects of cognition. These animals’ behavioral manifestations and cognitive deterioration were typical of clinical AD, characterized by impairments in spatial learning, short-term working memory, and long-term reference memory. Such cognitive deficits were not apparent in animals treated long-term with candesartan, which showed much more favorable inflammatory profiles and lower levels of nitro-oxidative stress. These results suggest that early ARB treatment might be beneficial in preventing age-related cognitive deficits in AD-prone individuals and could be an emerging candidate for the treatment of other diseases linked to dementia.
ACKNOWLEDGMENTS
We acknowledge UTHSC Bridge funding support.
FUNDING
This study was supported by the National Institute of Health [R01-NS097800 (TI)] and startup funds (TI) Department of Anatomy and Neurobiology, UTHSC, Memphis, TN, supported this study.
CONFLICT OF INTEREST
The authors declare they have no conflict of interests.
SUPPLEMENTARY MATERIAL
[1] The supplementary material is available in the electronic version of this article: https://dx.doi.org/10.3233/ADR-220016.
REFERENCES
[1] | Ahmed HA , Ishrat T ((2020) ) The Brain AT2R — a potential target for therapy in Alzheimer’s disease and vascular cognitive impairment: A comprehensive review of clinical and experimental therapeutics. Mol Neurobiol 57: , 3458–3484. |
[2] | Saeedi M , Mehranfar F ((2022) ) Challenges and approaches of drugs such as Memantine, Donepezil, Rivastigmine, and Aducanumab in the treatment, control, and management of Alzheimer’s disease. Recent Pat Biotechnol, doi: 10.2174/1872208316666220302115901 |
[3] | Huang L , Chao S , Hu C ((2020) ) Clinical trials of new drugs for Alzheimer’s disease. J Biomed Sci 27: , 18–30. |
[4] | Ashburn TT , Thor KB ((2004) ) Drug repositioning: Identifying and developing new uses for existing drugs. Nat Rev Drug Discov 3: , 673–683. |
[5] | Ahmed HA. Ishrat T , Pillai B. Fouda AY , Sayed MA , Eldahshan W. Waller JL , Ergul A Fagan SC ((2018) ) RAS modulation prevents progressive cognitive impairment after experimental stroke: A randomized, blinded preclinical trial. J Neuroinflammation 15: , 229–245. |
[6] | Tota S , Kamat PK , Awasthi H , Singh N , Raghubir R , Nath C , Hanif K ((2009) ) Candesartan improves memory decline in mice: Involvement of AT1 receptors in memory deficit induced by intracerebral streptozotocin. Behav Brain Res 199: , 235–240. |
[7] | ((2015) ) Candesartan [package Insert]. AstraZeneca LP, Wilmington, DE. |
[8] | Gradman AH ((2002) ) AT1-receptor blockers: Differences that matter. J Hum Hypertens 16: , S9–S16. |
[9] | Unger T ((2003) ) Inhibiting angiotensin receptors in the brain: Possible therapeutic implications. Curr Med Res Opin 19: , 449–451. |
[10] | Ahmed HA , Ishrat T , Pillai B , Bunting KM , Patel A , Vazdarjanova A , Waller JL , Arbab AS , Ergul A , Fagan SC ((2018) ) Role of angiotensin system modulation on progression of cognitive impairment and brain MRI changes in aged hypertensive animals - A randomized double-blind pre-clinical study. Behav Brain Res 346: , 29–40. |
[11] | Villapol S , Yaszemski AK , Logan TT , Sanchez-Lemus E , Saavedra JM , Symes AJ ((2012) ) Candesartan, an angiotensin II AT(1)-receptor blocker and PPAR-gamma agonist, reduces lesion volume and improves motor and memory function after traumatic brain injury in mice. Neuropsychopharmacology 37: , 2817–2829. |
[12] | Timaru-Kast R , Wyschkon S , Luh C , Schaible EV , Lehmann F , Merk P , Werner C , Engelhard K , Thal SC ((2012) ) Delayed inhibition of angiotensin II receptor type 1 reduces secondary brain damage and improves functional recovery after experimental brain trauma. Crit Care Med 40: , 935–944. |
[13] | Braszko JJ , Wincewicz D ((2013) ) Candesartan prevents impairment of recall caused by repeated stress in rats. Psychopharmacology (Berl) 225: , 421–428. |
[14] | Tsukuda K , Mogi M , Li JM , Horiuchi M ((2007) ) Amelioration of cognitive impairment in the type-2 diabetic mouse by the angiotensin II type-1 receptor blocker candesartan. Hypertension 50: , 1099–1105. |
[15] | Fernández A , Quintana E , Velasco P , Moreno-Jimenez B , de Andrés B , Gaspar ML , Liste I , Vilar M , Mira H , Cano E. ((2021) ) Senescent accelerated prone 8 (SAMP8) mice as a model of age dependent neuroinflammation. J Neuroinflammation 18: , 75. |
[16] | Pallas M ((2012) ) Senescence-accelerated mice P8: A tool to study brain aging and Alzheimer’s disease in a mouse model. ISRN Cell Biol 2012: , 917167. |
[17] | Sun J , Mamo JCL , Takechi R , Owada Y , Nicolazzo J , Pan Y ((2021) ) Learning deficits occurs prior to memory retrieval impairment in female senescence accelerated mouse (SAMP8). Alzheimers Dement 17: (Suppl 12), e058418. |
[18] | Pang KCH , Miller JP , Fortress A , Mcauley JD ((2006) ) Age-related disruptions of circadian rhythm and memory in the senescence-accelerated mouse (SAMP8). Age (Dordr) 28: , 283–296. |
[19] | Kozak W , Kozak A , Johnson MH , Elewa HF , Fagan SC ((2008) ) Vascular Protection with candesartan after experimental acute stroke in hypertensive rats: A dose-response study. J Pharmacol Exp Ther 326: , 773–782. |
[20] | Kilkenny C , Browne W , Cuhill IC , Emerson M , Altman DG ((2010) ) Improving bioscience research reporting: The ARRIVE Guidelines for Reporting Animal Research. PLoS Biol 8: , 6–10. |
[21] | Percie du Sert N , Hurst V , Ahluwalia A , Alam S , Avey MT , Baker M , Browne WJ , Clark A , Cuthill IC , Dirnagl U , Emerson M , Garner P , Holgate ST , Howells DW , Karp NA , Lazic SE , Lidster K , MacCallum CJ , Macleod M , Pearl EJ , Petersen OH , Rawle F , Reynolds P , Rooney K , Sena ES , Silberberg SD , Steckler T , Würbel H ((2020) ) The ARRIVE guidelines 2.0: Updated guidelines for reporting animal research. PLoS Biol 18: , e3000410. |
[22] | McKhann GM , Knopmanc DS , Chertkowd H , Hymanf BT , Jack CR Jr. , Kawash CH , Klunkk WE , Koroshetzl WJ , Manlym JJ , Mayeuxm R , Mohsp RC , Morrisq JC , Rossorr MN , Scheltens P , Carrillo MC , Thies B , Weintraub S , Phelps CH ((2011) ) The diagnosis of dementia due to Alzheimer’s disease: Recommendations from the National Institute on Aging-Alzheimer’s Association workgroups on diagnostic guidelines for Alzheimer’s disease. Alzheimers Dement 7: , 263–269. |
[23] | Ahmed HA , Ishrat T , Pillai B , Bunting KM , Vazdarjanova , Waller JL , Ergul A , Fagan SC ((2019) ) Angiotensin receptor (AT2R) agonist C21 prevents cognitive decline after permanent stroke in aged animals—A randomized double-blind pre-clinical study. Behav Brain Res 359: , 560–569. |
[24] | Ma J , Xiong JY , Hou WW , Yan HJ , Sun Y , Huang SW , Jin L , Wang Y , Hu WW , Chen Z ((2012) ) Protective effect of carnosine on subcortical ischemic vascular dementia in mice. CNS Neurosci Ther 18: , 745–753. |
[25] | Wietrzych M , Meziane H , Sutter A , Ghyselinck N , Chapman PF , Chambon P , Krezel W ((2005) ) Working memory deficits in retinoid X receptor gama-deficient mice. Learn Mem 12: , 318–326. |
[26] | Antunes M , Biala G ((2012) ) The novel object recognition memory: Neurobiology, test procedure, and its modifications. Cogn Process 13: , 93–110. |
[27] | Ahmed HA , Ismael S , Mirzahosseini G , Ishrat T ((2021) ) Verapamil prevents development of cognitive impairment in an aged mouse model of sporadic Alzheimer’s disease. Mol Neurobiol 58: , 3374–3387. |
[28] | Singh P , Song C , Young D , Shubha R , Gonzalez FJ , Malik KU ((2020) ) Central CYP1B1 (cytochrome P450 1B1)-estradiol metabolite 2-methoxyestradiol protects from hypertension and neuroinflammation in female mice. Hypertension 75: , 1054–1062. |
[29] | Manimala J , Gupte P , Kaur A , Wong K , Anderson A , Stewart D , Oberoi P ((2014) ) Development and validation of a 10-plex mouse proinflammatory cytokine V-PLEX®. Panel. MSD Poster AAPS 2014, San Diego. |
[30] | Rong J , Xiong CY , Rong DY , Hu BR ((2021) ) Meso-scale discovery assay detects the changes of plasma cytokine levels in mice after low or high LET ionizing irradiation. Biomed Environ Sci 34: , 540–551. |
[31] | Gally F , Rao D , Schmitz C , Kelley L , Yeager ME , Perraud A-L ((2017) ) The TRPM2 ion channel contributes to cytokine hyperproduction in a mouse model of down syndrome. Biochim Biophys Acta Mol Basis Dis 1864: , 126–143. |
[32] | Ding M , Cavallin A , Hermansson N , Berntsson P , Jinton L , Blomqvist SR ((2018) ) Comparing flow cytometry QBeads PlexScreen assays with other immunoassays for determining multiple analytes. SLAS Discov 23: , 676–686. |
[33] | Abouhish H , Thounaojam MC , Jadeja RN , Gutsaeva DR , Powell FL , Khriza M , Martin PM , Bartoli M ((2020) ) Inhibition of HDAC6 attenuates diabetes-induced retinal redox imbalance and microangiopathy. Antioxidants 9: , 599–617. |
[34] | Ishrat T , Mohamed IN , Pillai B , Soliman S , Fouda A , Ergul A , El-Remessy AB , Fagan SC ((2015) ) Thioredoxin interacting protein: A novel target for neuroprotection in experimental thromboembolic stroke in mice. Mol Neurobiol 51: , 766–778. |
[35] | Piccarducci R , Pietrobono D , Pellegrini C , Daniele S , Fornai M , Antonioli L , Martini C ((2019) ) High levels of β-amyloid, tau, and phospho-tau in red blood cells as biomarkers of neuropathology in senescence-accelerated mouse. Oxid Med Cell Longev 2019: , 5030475. |
[36] | Flores J , Noël A , Leblanc AC , Lynham J ((2018) ) Caspase-1 inhibition alleviates cognitive impairment and neuropathology in an Alzheimer’s disease mouse model. Nat Commun 9: , 3916. |
[37] | Chen W , Liang T , Zuo W , Wu X , Shen Z , Wang F , Peng G ((2018) ) Neuroprotective effect of 1-Deoxynojirimycin on cognitive impairment, β-amyloid deposition, and neuroinflammation in the SAMP8 mice. Biomed Pharmacother 106: , 92–97. |
[38] | Carter TA , Greenhall JA , Yoshida S , Fuchs S , Helton R , Swaroop A , Barlow C ((2005) ) Mechanisms of aging in senescence-accelerated mice. Genome Biol 6: , R48. |
[39] | Zhao Q-F , Tan L , Wang H-F , Jiang T , Tan M-S , Tan L , Yu J-T ((2016) ) The prevalence of neuropsychiatric symptoms in Alzheimer’s disease: Systematic review and meta-analysis. J Affect Disord 190: , 264–271. |
[40] | Vasilipoulou F , Companys-Alemany J , Canudas A , Palomera-Ávalos V , Ortuño-Sahagún D , Pallàs M , Griñán-Ferré C ((2020) ) Anxiety, impaired social and aggressive behaviour correlates with cognitive and molecular changes in older SAMP8 males. Preprints 2020: , 2020110336. |
[41] | Bidzan L , Bidzan M , Pąchalska M ((2012) ) Aggressive and impulsive behavior in Alzheimer’s disease and progression of dementia. Med Sci Monit 18: , CR182–189. |
[42] | Beery AK , and Zucker I ((2011) ) Sex bias in neuroscience and biomedical research annaliese. Neurosci Biobehav Rev 35: , 565–572. |
[43] | Flood JF , Farr SA , Fran E. Kaiser FE , Morley JE ((1995) ) Age-related impairment in learning but not memory in SAMP8 female mice. Pharmacol Biochem Behav 50: , 661–664. |
[44] | Sánchez-Lemus E , Honda M , Saavedra JM ((2012) ) Angiotensin II AT1 receptor blocker candesartan prevents the fast up-regulation of cerebrocortical benzodiazepine-1 receptors induced by acute inflammatory and restraint stress. Behav Brain Res 232: , 84–92. |
[45] | Trofimiuk E , Wielgat P , Braszko J ((2018) ) Candesartan, angiotensin II type 1 receptor blocker is able to relieve age-related cognitive impairment. Pharmacol Rep 70: , 87–92. |
[46] | Han J , Hosokawa M , Umezawa M , Yagi H , Matsushita T , Higuchi K , Takeda T ((1998) ) Age-related changes in blood pressure in the senescence-accelerated mouse (SAM): Aged SAMP1 mice manifest hypertensive vascular disease. Lab Anim Sci 48: , 256–263. |
[47] | Reed AL , Tanaka A , Sorescu D , Liu H , Jeong E-M , Sturdy M , Walp ER , Dudley SC , Sutliff RL ((2011) ) Diastolic dysfunction is associated with cardiac fibrosis in the senescence-accelerated mouse Diastolic dysfunction is associated with cardiac fibrosis in the senescence-accelerated mouse. Am J Physiol Heart Circ Physiol 301: , H824–H831. |
[48] | Sawano E , Negishi T , Aoki T , Murakami M ((2013) ) Alterations in local thyroid hormone signaling in the hippocampus of the SAMP8 mouse at younger ages: Association with delayed myelination and behavioral abnormalities. J Neurosci Res 91: , 382–392. |
[49] | López-Ramos JC , Jurado-Parras MT , Sanfeliu C , Acuña-Castroviejo D , Delgado-García JM ((2012) ) Learning capabilities and CA1-prefrontal synaptic plasticity in a mice model of accelerated senescence. Neurobiol Aging 33: , 627.e13–627.e26. |
[50] | Lovell MA , Xie C , Gabbita SP , Markesbery WR ((2000) ) Decreased thioredoxin and increased thioredoxin reductase levels in Alzheimer’s disease brain. Free Radic Biol Med 28: , 418–427. |
[51] | Guo YZ , Chenghong W , Chunyang H , Yufei LJ , Chu H ((2021) ) Thioredoxin-1 is a target to attenuate Alzheimer-like pathology in diabetic encephalopathy by alleviating endoplasmic reticulum stress and oxidative stress. Front Physiol 12: , 651105. |
[52] | Akiguchi I , PallàsM , Budka H , Akiyama H , Ueno M , Han J , Yagi H , Nishikawa T , Chiba Y , Sugiyama H , Takahashi R , Unno K , Higuchi K , Hosokawa K ((2017) ) SAMP8 mice as a neuropathological model of accelerated brain aging and dementia: Toshio Takeda’s legacy and future directions. Neuropathology 37: , 293–305. |
[53] | Qie S , Ran Y , Lu X , Su W , Li W , Xi Ji , Gong W , Liu Z ((2020) ) Candesartan modulates microglia activation and polarization via NF-κ B signaling pathway. Int J Immunopathol Pharmacol 34: , 2058738420974900. |
[54] | Pelisch N , Hosomi N , Ueno M , Nakano D , Hitomi H , Mogi M , Shimada k , Kobori H , Horiuchi M , Sakamoto H , Matsumoto M , Kohno M , Nishiyama A ((2011) ) Blockade of AT1 receptors protects the BBB and improves cognition in Dahl salt-sensitive hypertensive rats. Am J Hypertens 24: , 362–368. |
[55] | Guerrero A , De Strooper B , Arancibia-Cárcamo IL ((2021) ) Cellular senescence at the crossroads of inflammation and Alzheimer’s disease. Trends Neurosci 44: , 714–727. |