Borrelidin from Saltern-Derived Halophilic Nocardiopsis sp. Dissociates Amyloid-β and Tau Fibrils
Abstract
Background:
Alzheimer’s disease (AD) is characterized by the aggregation of two pathological proteins, amyloid-β (Aβ) and tau, leading to neuronal and cognitive dysfunction. Clearance of either Aβ or tau aggregates by immunotherapy has become a potential therapy, as these aggregates are found in the brain ahead of the symptom onset. Given that Aβ and tau independently and cooperatively play critical roles in AD development, AD treatments might require therapeutic approaches to eliminate both aggregates together.
Objective:
We aimed to discover a chemical drug candidate from natural sources for direct dissociation of both insoluble Aβ and tau aggregates through in vitro assessments.
Methods:
We isolated four borrelidin chemicals from a saltern-derived halophilic actinomycete strain of rare genus Nocardiopsis and simulated their docking interactions with Aβ fibrils. Then, anti-cytotoxic, anti-Aβ, and anti-tau effects of borrelidins were examined by MTT assays with HT22 hippocampal cell line, thioflavin T assays, and gel electrophoresis.
Results:
When HT22 cells were exposed to Aβ aggregates, the treatment of borrelidins alleviates the Aβ-induced toxicity. These anti-cytotoxic effects can be derived from the inhibitory functions of borrelidins against the Aβ aggregation as shown in thioflavin T and gel electrophoretic analyses. Among them, especially borrelidin, which exhibits the highest probability of docking, not only dissociates Aβ aggregates but also directly regulates tau aggregation.
Conclusion:
Borrelidin dissociates insoluble Aβ and tau aggregates together and our findings support the view that it is possible to develop an alternative chemical approach mimicking anti-Aβ or anti-tau immunotherapy for clearance of both aggregates.
INTRODUCTION
Alzheimer’s disease (AD) is the most common type of dementia characterized by cognitive impairments associated with neurotoxicity [1, 2]. Abnormal aggregation of the extracellular amyloid-β (Aβ) and the intracellular tau is considered to be the ma-jor culprit of the AD pathology and a key target for treatments [3, 4]. Because such protein aggrega-tion induces neuronal death and interferes the syn-aptic function in the brain, several current disease-modifying treatments in phase III aim to clear the aggregates or prevent the production of them [5, 6]. However, none of these drugs have been approved for clinical use yet [6]. The possible reason of the failure is that most of drug candidates were designed to target only one among the two pathogenic proteins, Aβ and tau. It is important to remove both proteins simultaneously due to an extensive crosstalk between Aβ and tau during the development of AD pathology [7]. Synergistic interactions between Aβ and tau aggregates result in accelerated neurodegeneration and cognitive impairment compared to their individual contributions on disease progression [8]. It leads to shift attention to a new approach that simultaneously regulates two pathogenic proteins of AD. Currently, two small molecules with a dual targeting mechanism, Nilotinib and Posiphen, are undergoing phase II clinical trials; however, both drug candidates regulate Aβ and tau indirectly. Therefore, we focused on natural product borrelidins, isolated from a halophilic actinomycete living in hypersaline environments, and assessed its therapeutic activities against two pathogenic proteins of AD.
Here, we report the possibility of borrelidin as a small molecule mimicking anti-Aβ and anti-tau anti-body drug candidates to clear the toxic aggregates. Borrelidin (1) and borrelidins C-E (2–4), were obt-ained from a saltern-derived halophilic actinomycete strain belonging to the rare genus Nocardiopsis (Fig. 1A) [9]. These small molecules were previously reported for their anti-cancer and anti-bacterial functions. Likewise, several studies have shown that borrelidins, especially 1, possess therapeutic effects against many diseases; however, the effects on neurodegenerative diseases have not been reported yet [9–13]. In this study, the docking models of 1–4 to Aβ fibrils presented the potential interactions between Aβ and borrelidins mostly formed within the hydrophobic pockets of Aβ. The comparative structure-activity analysis suggested that the hydroxyl groups in the cyclopentane ring are responsible for the varied activities of the borrelidin derivatives on Aβ aggregation by affecting solvent accessibility of the ligands. Also, we investigated the potency of 1–4 on Aβ aggregates-induced neuronal cell death by MTT assay. To further assess their activities, inhibition of Aβ aggregation by 1–3 was examined through Thioflavin T (ThT) and Photo-Induced Cross-Linking of Unmodified Proteins (PICUP) assay. Among these molecules, the most potent candidate 1 was additionally examined on its dissociation mechanism for Aβ and tau in vitro.
Fig. 1
Docking simulation of borrelidins. A) Chemical structures of borrelidin (1) and borrelidins C-E (2–4) and (B) binding simulation of each compound to Aβ aggregates in docking model.
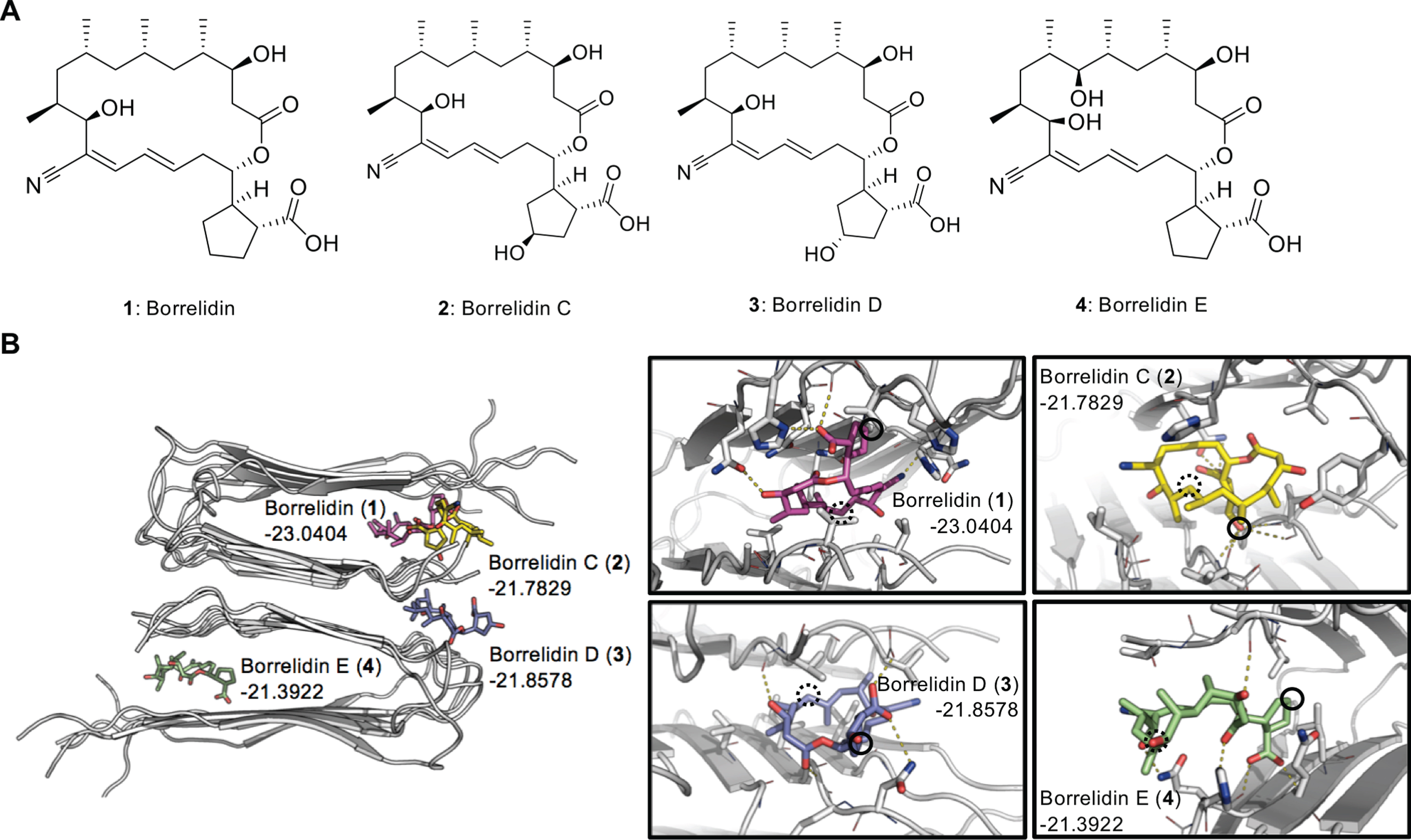
MATERIALS AND METHODS
MTT assay
Borrelidins (1–4) were obtained as previously reported [9]. Aβ42 synthesis and MTT assay using HT22 murine hippocampal neuronal cells were performed as previously described [14–17]. Cells (2×104 cells/well) were treated with 10μM Aβ42 with 1–4 (20, 100μM) and incubated for 24 h at 37°C. After the incubation, Aβ-induced cytotoxicity was measured by absorbance as previously reported [16, 17].
Docking simulation
The docking of 1–4 to Aβ42 were described previously [17, 18]. Briefly, the two-staged docking procedure was sequentially performed using the three-dimensional conformers of each ligand. The numbers of conformers considered for docking were 61, 42, 75, and 51 for 1–4, respectively. The low-resolution global docking was first performed by PatchDock software [19] to estimate potential binding sites in the Aβ structure (PDB ID 2LMO). Of the low-resolution docking structures, the top 500 were used for the secondary all-atom refinement by Rosetta software [20]. Docking structures were refined by the customized Rosetta ligand docking protocol, consisted of five rounds of docking/minimization, two low-repulsive followed by three normal. The docking conformation with the lowest binding energy was finally selected as the most plausible model. The binding energy was estimated by the Rosetta energy difference between ligand-bound and unbound structure.
Aβ inhibition and disaggregation
10 mM Aβ42 were dissolved in DMSO and diluted with distilled water to make 25μM Aβ solutions. To measure the inhibition effect of 1–3 on Aβ aggregation, monomeric Aβ42 with 1 mM of each compound was incubated for five days at 37°C. For Aβ disaggregation, 0.1 mM of Aβ42 in 10% DMSO and 90% distilled water was aggregated for three days at 37°C. After the pre-incubation, 1 mM of compound was added to aggregates and incubated for additional three days at 37°C. To observe the inhibition or dissociating effect of compound, all samples were loaded into the half-area 96-well black plate. 75μL of ThT solution (5μM in 50 mM glycine buffer, pH 8.5) was added to each well [21]. Fluorescence of Aβ-bound ThT was measured at 450 nm (excitation) and 485 nm (emission) using SpectraMax M2 microplate reader (Molecular Devices).
Tau inhibition and disaggregation
Tau K18 fragment (0.5 mg/mL in PBS) was used in these assays. For tau inhibition assay, 1 mM of compound was added to tau containing 0.1 mg/mL of Heparin and 100μM of DTT, aggregation inducers. Mixed samples were incubated for five days at 37°C. For disaggregation assay, tau containing inducers was aggregated for two days at 37°C. After the pre-incubation, 1 mM of compound was added to aggregates and incubated for additional three days at 37°C. To evaluate the inhibition and disaggregation effect of compound, the fluorescence intensity was measured by ThT assay as described above.
PICUP and SDS-PAGE
To visualize inhibitory effect of compounds on Aβ aggregation, all samples were prepared under the identical conditions as used in ThT assay. For Aβ cross-linking, 10 mM Ru(Bpy) and 200 mM APS were dissolved in buffer A (0.1 M sodium phosphate, pH 7.4), and diluted with the same buffer to make 1 mM and 20 mM, respectively. Then, 1μL of Ru(Bpy) and APS were added to 10μL of each incubated sample. The mixtures were irradiated by visible light, and the reaction was quenched by adding 3μL of 5X sample buffer containing 5% reducing agent. Then, samples were boiled for 5 min at 95°C and separated by SDS-PAGE electrophoresis. After gel running, different size of Aβ peptides was visualized by silver staining according to the manufacturer’s instructions (GE Healthcare).
Statistical analysis
Graphs were obtained with GraphPad Prism 7 and statistical analysis were performed with Student’s t-tests and one-way ANOVA, followed by Bonferroni’s post hoc comparisons tests (*p < 0.05, **p < 0.01, ***p < 0.001). The error bars in all data represent the SEM.
RESULTS
Borrelidins bind to Aβ aggregates in docking model
As the abnormal aggregation of Aβ and tau exacerbate AD, the direct interaction between these pathological proteins and drug candidate is needed to dissociate the toxic aggregates. In this regard, we initially performed exhaustive low-resolution docking simulation to find the most plausible docking model of 1–4 to the Aβ structure. These low-resolution docking structures were then refined by Rosetta ligand optimization protocol with all-atom Rosetta energy function (REF15) [22]. The final docking model for each ligand was selected by the calculated binding energy.
Among the docking models, 1 showed the lowest binding energy (–23.04 by Rosetta Energy Unit) and 2–4 showed undistinguishable binding energies (Fig. 1B). The docking structures of 1, 2, and 4 had similar binding modes that commonly packed in the hydrophobic patch formed by the beta-strand assembly, whereas 3 was located between the beta-strand assemblies. Notably, the docking model of 1 was more sequestered inside the beta-strands than the others, which might be more effective in terms of preventing further Aβ aggregation.
The chemical structures of 1 and 2 are very similar except for the hydroxyl group (solid line circle in Fig. 1B) attached to the cyclopentane ring. In the docking model of 1, the cyclopentane ring showed tight hydrophobic contacts to the Aβ structure without the hydroxyl group attachment. However, 2 was docked more towards the exterior surface, probably due to the presence of the hydroxyl group in the cyclopentane ring. The hydroxyl group in 2 formed hydrogen bonds to the main-chain atoms of Val39.
On the other hand, the chemical structure of 4 is almost similar to 1 except for another hydroxyl group attached to the carbon chain (dotted line circle in Fig. 1B). The carbon chains in 4 faced outside, but the same part in all other ligands without the hydroxyl group was tightly packed into the hydrophobic patches in the Aβ structure. Moreover, the docking model of 3 showed a distinctive binding mode where the hydroxyl group was fully exposed to the solvent. Accordingly, the two hydroxyl groups might be responsible for the varied activities of the borrelidin derivatives on Aβ aggregation.
Borrelidins reduce Aβ-induced cytotoxicity
Since the possibility of binding between Aβ and borrelidins was confirmed through the docking model, we performed additional biochemical assays to investigate the effects of 1–4 on Aβ and tau aggregates. To evaluate their effects on Aβ-oligomer-induced neuronal cell death, we performed MTT assay using HT22, murine hippocampal cell line. Aβ were pre-aggregated at 37°C for 24 hours to form oligomers. We observed about 60% decrease in cell viability due to the toxicity induced by pre-formed aggregates. However, when the cells were treated with 1–4, a significant increase in cell viability was observed (Fig. 2A). 1 and 2 attenuated the Aβ oligomer-induced neuronal death dose dependently, and about 100% of viability as non-treated cell was shown at higher concentration (100μM) of both compounds. 4 showed the significant change in only higher concentration. These findings indicated that both concentrations (20 and 100μM) of 1–3 reduced the neurotoxicity induced by Aβ oligomers.
Fig. 2
The effects of borrelidins, especially borrelidin (1), against Aβ and tau pathology in vitro. A) The effects of each compound on Aβ-induced cell death were measured by MTT assay in HT22 neuronal cell line. Cell viability was normalized to non-treated cells (100%). B, C) The inhibition effects of borrelidin (1) and borrelidin C, D (2, 3) were presented by ThT assay (B) and PICUP followed by SDS-PAGE gel (C). Fluorescence intensity was normalized to Aβ aggregates (100%, 5 days incubation). D) Aβ disaggregation effect of borrelidin (1) by ThT assay. Fluorescence intensity was normalized to pre-formed Aβ aggregates (100%, 3 days incubation). E, F) Tau inhibition (E) and disaggregation effects (F) of borrelidin (1) by ThT assay. Fluorescence intensity was normalized to tau aggregates (100%, 5 days incubation) and pre-formed tau aggregates (100%, 2 days incubation), respectively. Cpd, compound; aggr., aggregates. All data were represented as mean±SEM. MTT and ThT assays were analyzed by Student’s t-tests and One-way ANOVA followed by Bonferroni post-hoc comparison tests (*p≤0.05 and ***p≤0.001).
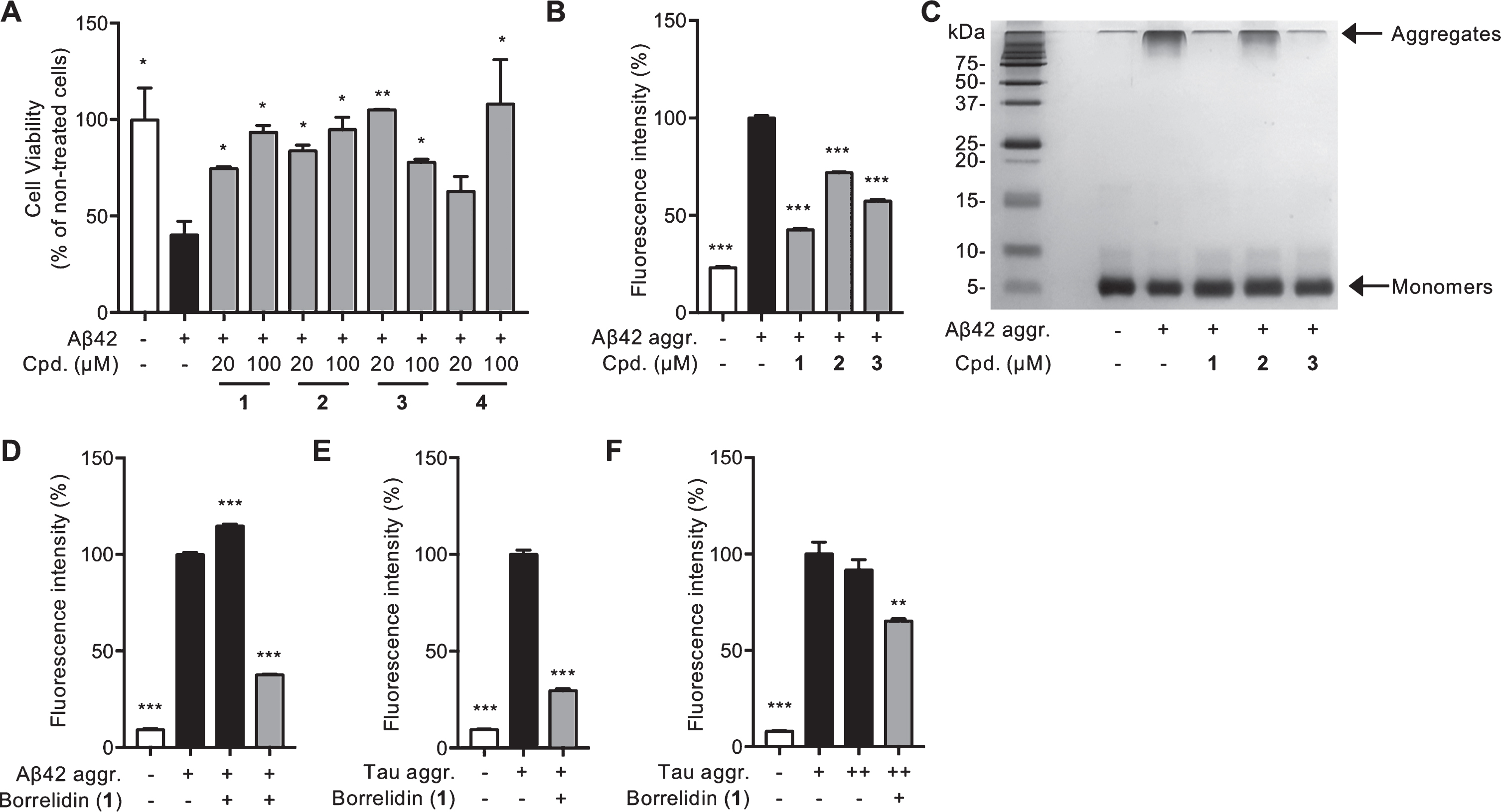
Borrelidins inhibit Aβ aggregation
Next, we quantified the Aβ fibril formation through ThT fluorescence intensity [23] to examine the protective mechanisms of borrelidins against neuronal death by Aβ aggregates. All compounds significantly inhibited the Aβ aggregation, especially, 1 exhibited the highest effect against Aβ aggregation, reducing ThT-positive aggregates by more than 50% (Fig. 2B). However, it is difficult to identify the effects on oligomers or protofibrils by ThT assays. Thus, all samples were visualized after covalently cross-linking of proteins to fix the original states of aggregates [24]. Through the silver stained SDS-PAGE gel, we discovered that all compounds, especially 1 and 3, remarkably decreased the formation of Aβ fibrils in size greater than 250 kDa (Fig. 2C), corresponding to ThT results (Fig. 2B). It might be because the conversion of aggregates to the monomers (4.5 kDa) by the compounds, resulting in increase of Aβ monomers when incubated with each compound.
Borrelidin dissociates pre-formed Aβ aggregates and also regulates tau pathology
Overall, 1 showed the highest possibility; interaction with Aβ aggregates, leading to the decrease of neuronal cell death via an inhibition of Aβ aggregation. However, the clearing of toxic pre-formed aggregates is considered more effective way because these accumulation starts decades in the brain before onset of symptoms. Thus, we examined whether 1, which was regarded as the most potent inhibitor, also has a clearance effect on pre-existing Aβ aggregates. We observed the levels of Aβ aggregates measured by ThT fluorescence intensity were notably reduced when incubated with 1, indicating that it could dissociate pre-existing Aβ aggregates (Fig. 2D).
As aggregation of tau proteins are also contributed to neuronal dysfunction and eventually neuronal death, we confirmed that the inhibitory effects of 1 on tau aggregation. Tau K18 fragment containing repeat domain was used in this assay. After tau incubation with 1, we found that the level of tau aggregation decreased by more than 50% in ThT assay (Fig. 2E). Moreover, ThT fluorescence intensity was also reduced when 1 was incubated with pre-aggregated tau, implying that this small molecule has inhibitory effect on tau aggregation and also can dissociate tau aggregates (Fig. 2F).
DISCUSSION
Here we report that borrelidin, a natural product derived from Nocardiopsis sp. isolated from a solar saltern, can dissociate Aβ and tau fibrils in vitro, leading to attenuate neuronal cell death induced by toxic aggregates (Fig. 3). Previously, we reported that reduced Aβ aggregates and hyperphosphorylated tau together mediated by a small molecule necrostatin-1 led to inhibition of neuronal cell death, and eventually alleviation of cognitive impairments in AD model mouse [25]. Thus, our in vitro data suggests borrelidin may be potential to improve cognitive function in vivo and such possibility makes borrelidin more beneficial than necrostatin-1 in that it dissociates both Aβ and tau aggregates.
Fig. 3
The mechanism of action of borrelidin. The inhibition of the aggregation of both protein aggregates and dissociates toxic aggregates by borrelidin.
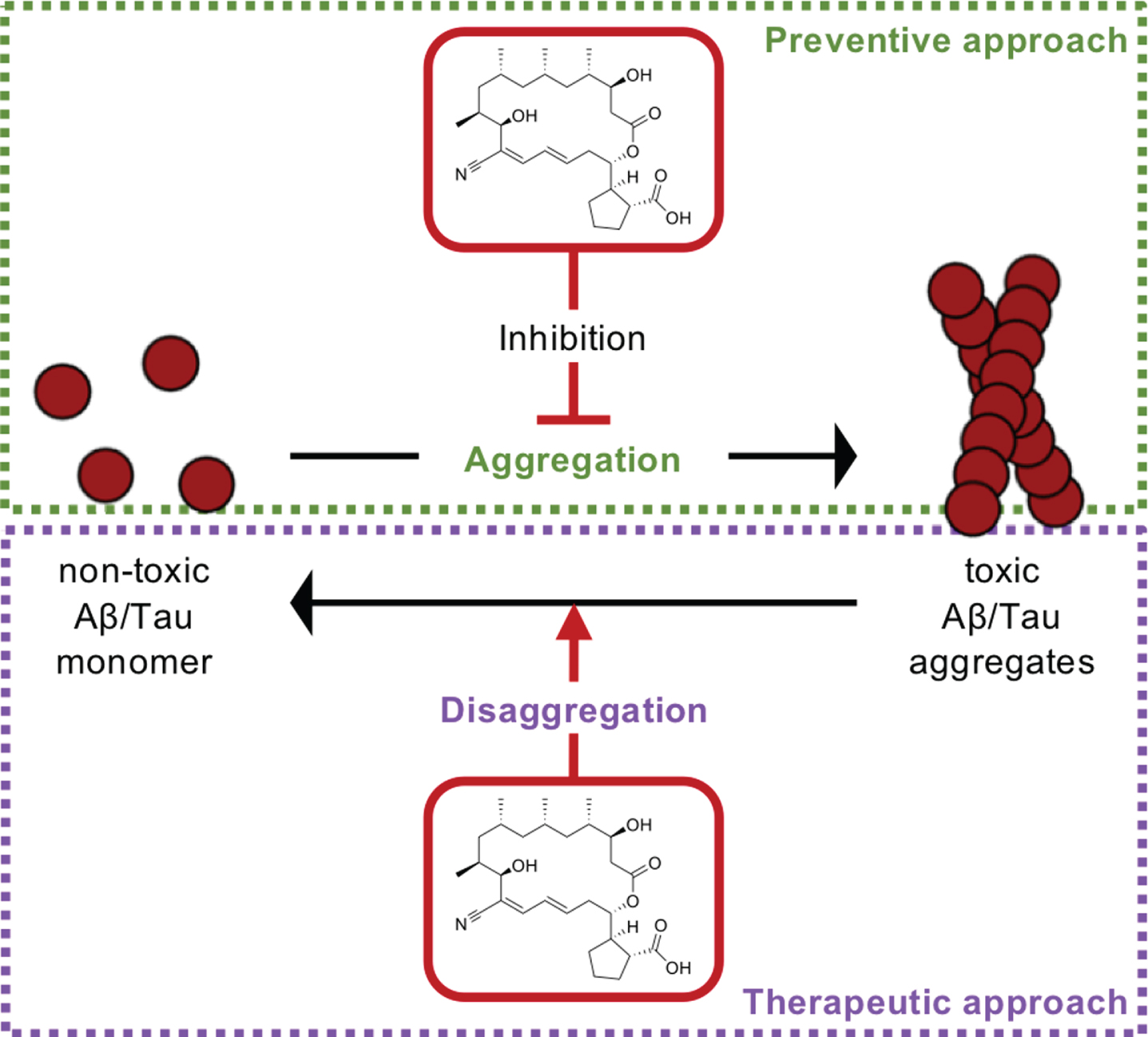
Given that AD is a chronic disorder that may require a long-term administration of treatments, safety is one of the major issues for AD drugs. Such property can be solved by searching for drug candidates among natural products such as borrelidin. It is not common to find a single small natural product effective on both pathogenic proteins of AD, Aβ and tau, with additional anti-neurotoxic activity. Although further animal studies are required to warrant the therapeutic potency of borrelidin in vivo, the discovery of the effects of borrelidins, especially 1, on toxic Aβ and tau aggregates highlights that saltern-derived secondary metabolites could serve as chemical reservoirs providing bioactive compounds with pharmaceutical potential.
CONFLICT OF INTEREST
The authors have no conflict of interest to report.
ACKNOWLEDGMENTS
This work was supported by Yonsei University (2017-22-0072) and National Research Foundation of Korea (NRF-2015R1A6A3A04058568, NRF-2017M3A9E2058046, NRF-2018R1A4A1021703, NRF-2015R1A6A3A04058568, and NRF-2020R1F1A1076240) funded by the Ministry of Science and ICT. This research was also supported by the Collaborative Genome Program (20180430) of the Korea Institute of Marine Science and Technology Promotion (KIMST) funded by the Ministry of Oceans and Fisheries, Korea.
REFERENCES
[1] | Terry RD , Masliah E , Salmon DP , Butters N , DeTeresa R , Hill R , Hansen LA , Katzman R ((1991) ) Physical basis of cognitive alterations in Alzheimer’s disease: Synapse loss is the major correlate of cognitive impairment, Ann Neurol 30: , 572–580. |
[2] | Fox NC , Scahill RI , Crum WR , Rossor MN ((1999) ) Correlation between rates of brain atrophy and cognitive decline in AD, Neurology 52: , 1687–1689. |
[3] | Sobow T , Flirski M , Liberski PP ((2004) ) Amyloid-beta and tau proteins as biochemical markers of Alzheimer’s disease, Acta Neurobiol Exp 64: , 53–70. |
[4] | Hong-Qi Y , Zhi-Kun S , Sheng-Di C ((2012) ) Current advances in the treatment of Alzheimer’s disease: Focused on considerations targeting Abeta and tau, Transl Neurodegener 1: , 21–32. |
[5] | Parihar MS , Brewer GJ ((2010) ) Amyloid-beta as a modulator of synaptic plasticity, J Alzheimers Dis 22: , 741–763. |
[6] | Cummings J , Lee G , Ritter A , Sabbagh M , Zhong K ((2019) ) Alzheimer’s disease drug development pipeline: 2019, Alzheimers Dement (N Y) 5: , 272–293. |
[7] | Nisbet RM , Polanco JC , Ittner LM , Gotz J ((2015) ) Tau aggregation and its interplay with amyloid-beta, Acta Neuropathol 129: , 207–220. |
[8] | Pascoal TA , Mathotaarachchi S , Shin M , Benedet AL , Mohades S , Wang SQ , Beaudry T , Kang MS , Soucy JP , Labbe A , Gauthier S , Rosa-Neto P , Alzheimer’s Disease Neuroimaging Initiative ((2017) ) Synergistic interaction between amyloid and tau predicts the progression to dementia, Alzheimers Dement 13: , 644–653. |
[9] | Kim J , Shin D , Kim SH , Park W , Shin Y , Kim WK , Lee SK , Oh KB , Shin J , Oh DC ((2017) ) Borrelidins C-E: New antibacterial macrolides from a saltern-derived halophilic nocardiopsis sp, Mar Drugs 15: , 166–176. |
[10] | Habibi D , Ogloff N , Jalili RB , Yost A , Weng AP , Ghahary A , Ong CJ ((2012) ) Borrelidin, a small molecule nitrile-containing macrolide inhibitor of threonyl-tRNA synthetase, is a potent inducer of apoptosis in acute lymphoblastic leukemia, Invest New Drug 30: , 1361–1370. |
[11] | Otoguro K , Ui H , Ishiyama A , Kobayashi M , Togashi H , Takahashi Y , Masuma R , Tanaka H , Tomoda H , Yamada H , Omura S ((2003) ) In vitro and in vivo antimalarial activities of a non-glycosidic 18-membered macrolide antibiotic, borrelidin, against drug-resistant strains of Plasmodia, J Antibiot 56: , 727–729. |
[12] | Tsuchiya E , Yukawa M , Miyakawa T , Kimura KI , Takahashi H ((2001) ) Borrelidin inhibits a cyclin-dependent kinase (CDK), Cdc28/Cln2, of Saccharomyces cerevisiae, J Antibiot 54: , 84–90. |
[13] | Wakabayashi T , Kageyama R , Naruse N , Tsukahara N , Funahashi Y , Kitoh K , Watanabe Y ((1997) ) Borrelidin is an angiogenesis inhibitor; Disruption of angiogenic capillary vessels in a rat aorta matrix culture model, J Antibiot 50: , 671–676. |
[14] | Choi JW , Kim HY , Jeon M , Kim DJ , Kim Y ((2012) ) Efficient access to highly pure beta-amyloid peptide by optimized solid-phase synthesis, Amyloid 19: , 133–137. |
[15] | Lee S , Kim Y ((2015) ) Anti-amyloidogenic approach to access amyloid-(1-42) in Fmoc solid-phase synthesis, Bull Korean Chem Soc 36: , 2147–2149. |
[16] | Kim HY , Kim Y , Han G , Kim DJ ((2010) ) Regulation of in vitro Abeta1-40 aggregation mediated by small molecules, J Alzheimers Dis 22: , 73–85. |
[17] | Yang SH , Lee DK , Shin J , Lee S , Baek S , Kim J , Jung H , Hah JM , Kim Y ((2017) ) Nec-1 alleviates cognitive impairment with reduction of Abeta and tau abnormalities in APP/PS1 mice, EMBO Mol Med 9: , 61–77. |
[18] | Yang SH , Shin J , Shin NN , Hwang JH , Hong SC , Park K , Lee JW , Lee S , Baek S , Kim K , Cho I , Kim Y ((2019) ) A small molecule Nec-1 directly induces amyloid clearance in the brains of aged APP/PS1 mice, Sci Rep 9: , 4183–4193. |
[19] | Schneidman-Duhovny D , Inbar Y , Nussinov R , Wolfson HJ ((2005) ) PatchDock and SymmDock: Servers for rigid and symmetric docking.W, Nucleic Acids Res 33: , 363–367. |
[20] | Lemmon G , Meiler J ((2012) ) Rosetta ligand docking with flexible XML protocols, Methods Mol Biol 819: , 143–155. |
[21] | Hasegawa K , Yamaguchi I , Omata S , Gejyo F , Naiki H ((1999) ) Interaction between Abeta(1-42) and Abeta(1-40) in Alzheimer’s beta-amyloid fibril formation in vitro, Biochemistry 38: , 15514–15521. |
[22] | Alford RF , Leaver-Fay A , Jeliazkov JR , O’Meara MJ , DiMaio FP , Park H , Shapovalov MV , Renfrew PD , Mulligan VK , Kappel K , Labonte JW , Pacella MS , Bonneau R , Bradley P , Dunbrack RL , Das R , Baker D , Kuhlman B , Kortemme T , Gray JJ ((2017) ) The rosetta all-atom energy function for macromolecular modeling and design, J Chem Theory Comput 13: , 3031–3048. |
[23] | Wolfe LS , Calabrese MF , Nath A , Blaho DV , Miranker AD , Xiong Y ((2010) ) Protein-induced photophysical changes to the amyloid indicator dye thioflavin T, Proc Natl Acad Sci U S A 107: , 16863–16868. |
[24] | Rahimi F , Maiti P , Bitan G ((2009) ) Photo-induced cross-linking of unmodified proteins (PICUP) applied to amyloidogenic peptides. J Vis Exp, e1071-1073. |
[25] | Yang SH , Lee DK , Shin J , Lee S , Baek S , Kim J , Jung H , Hah JM , Kim Y ((2017) ) Nec-1 alleviates cognitive impairment with reduction of Abeta and tau abnormalities in APP/PS1 mice, EMBO Mol Med 9: , 61–77. |