Modelling Modifiable Predictors of Age-Related Cognitive Decline: Exercise, Aortic Stiffness, and the Importance of Physical Fitness
Abstract
Background:
Previous modelling found that fitness and aortic stiffness both independently predicted spatial working memory (SWM) performance in older people. There is also evidence that greater engagement in moderate intensity exercise contributes to better cognitive performance, potentially working through improving fitness and aortic stiffness.
Objective:
To investigate the effect of exercise on the previously established relationships between fitness, aortic stiffness, and SWM, and whether these associations differ between older adults of higher and lower fitness.
Methods:
One hundred and two residents of independent living facilities, aged 60–90 (M = 77.5, SD = 6.9) participated in the study. Measures included computerized cognitive assessment, the Six-Minute Walk fitness test, the CHAMPS physical activity questionnaire, and aortic pulse wave analysis. Multiple structural equation models were used to test hypotheses.
Results:
Overall, exercise levels had a small additional effect in predicting SWM, working exclusively through fitness, although this was only true for those of lower than average fitness. Additionally, it was found that while fitness was the most important factor in predicting SWM in those of lower fitness, aortic stiffness was the strongest predictor in those of higher fitness.
Conclusion:
Fitness and aortic stiffness are strong predictors of cognition in older people, and greater engagement in exercise predicted better cognition in those who were of lower fitness. Fitter older people may benefit more from interventions which target aortic stiffness in order to preserve cognitive performance as they age, while those who are less fit may benefit most from improving fitness first, including through increased physical activity.
INTRODUCTION
The increase in global life expectancy is bringing with it an associated dramatic rise in age-associated illnesses, in particular serious cognitive decline and dementias such as Alzheimer’s disease (AD). In addition to the obvious devastating personal impacts of dementia, on both the affected individuals and their families, serious cognitive decline also has significant societal and economic costs. In particular, it is a major factor in the ever-increasing cost of age care, as the majority of permanent aged-care residents have some form of dementia [1]. In 2015 there were approximately 47 million people living with dementia globally, with an estimated economic impact of USD $818 billion [2]. Worldwide, with over 140 million people predicted to be living with dementia by 2050, dementia is considered to be the greatest social and health care challenge of the 21st century [2].
While declining cognition is considered a normal part of aging, more rapid or severe decline is a major risk factor for dementia [3].As there are currently no viable long-term pharmacological treatment options available, it is important that focus is placed on intervention strategies that address modifiable risk factors in accelerated cognitive decline and dementia. Of these modifiable risk factors, cardiovascular health [2, 4, 5] and physical fitness [6–9] have emerged as important targets, with increased exercise/physical activity considered a primary intervention methodology [2, 8, 9].
As outlined in the “vascular hypothesis” of AD, there is clear evidence that vascular health is inexorably linked to brain health and resultant cognitive functioning [4, 5, 10]. One area of particular focus in recent years is the stiffening of the central thoracic arteries (primarily the aorta), which is highly associated with increasing age [11]. These large elastic arteries play a major role in buffering the brain from the pulsatile output from the heart. Over the lifespan, these arteries progressively dilate and stiffen [12, 13], resulting in increased peak pressures being transmitted to downstream vascular beds. As the brain requires a continuous and high flow of blood, there is significantly less secondary vasoconstrictive arterial resistance in the brain than other tissues. As a result, the cerebral microvasculature is more exposed to increases in maximal pressures that of other tissue [11, 14]. Prolonged exposure to higher pressures is proposed to result in damage to these small vessels, resulting in eventual neuronal loss and, consequently, a degradation of cognitive ability [15–17]. While this relationship between higher aortic stiffness and greater cognitive decline has been supported by the bulk of the extant literature, much of the research in this area is considered statistically weak [17] and overly reliant on low-sensitivity measures of cognition such the Mini-Mental State Examination (MMSE) [16]. It is also still unclear whether the stiffening of the aorta associated with increasing age can be readily ameliorated [12]; however, there is some evidence that exercise, particularly involving moderate-to-vigorous types of physical activity, may lower central arterial stiffness in middle-aged and older people [18].
Lower levels of physical fitness have been shown to correlate with poorer cognition [19, 20] and higher risk of dementia in older people. Large population studies have found that, along with hearing loss, inactivity and low levels of physical fitness are the predominant modifiable lifestyle factors associated with increased rates of dementia, exceeding other primary areas of focus such as obesity, diabetes, hypertension, and smoking [21, 22]. In an older cohort, individuals with the most cognitive impairment were found to have the lowest levels of cardio-respiratory fitness and least engagement in physical activity [23]. A meta-analysis of MRI studies also concluded that, although the effect sizes were small, lower levels of physical fitness and activity are associated with lower white matter volume, and increased white matter lesion volume and severity [24]. These changes in white matter have, in turn, been associated with poorer cognitive function [25–27]. Interim analysis of the large Dr’s Extra intervention study, involving 1,335 older people, found that moderate to high levels of fitness predicted > 50% lower incidence of memory impairment than low fitness; however, it failed to show any group effect of exercise on cognition [28]. While a number of studies have found that exercise, in particular exercise of at least moderate intensity, may reduce the cognitive decline associated with aging [29, 30], intervention trial-based evidenced to support the efficacy of exercise in preventing cognitive decline or dementia is, at best, low in strength [31].
It has been proposed that physical activity may improve fitness and subsequently improve cognition in older individuals of lower fitness, while maintaining fitness and therefore maintaining cognitive function in those with moderate to high levels of fitness [32, 33], suggesting possible boundary conditions for the efficacy of exercise and fitness in improving cognitive performance in the elderly. This proposed boundary effect is also supported by the findings of a recent study of middle-aged office workers. This research found that those with low level of fitness performed worse in a number of cognitive assessments; however, there was no difference in performance between those of moderate and high fitness levels [34].
Our recently published study [35] derived a structural equation model based on data from the Lifestyle Intervention in Independent Living Aged Care (LIILAC) trial [36]. This model showed that, while there was no direct relationship between them (contrary to expectations), both better fitness (assessed using the Six Minute Walk (6 MW) Test) and lower aortic stiffness (assessed via carotid-femoral pulse wave velocity, CFPWV) independently predicted better Spatial Working Memory (SWM) performance in older people and, in conjunction with BMI and sex, explained 33% of the total SWM variance. Additionally, in combination, fitness and aortic stiffness totally mediated the strong effect of age on cognitive performance [35].
The current study extended the investigation of our previous model on the relationships between fitness, CFPWV, and SWM, by investigating the effects that physical activity and levels of fitness would have on the model. In particular, the effect of energy expenditure in moderate-to-vigorous physical activity (MVPA) on this model was examined. As illustrated in the conceptual model shown in Fig. 1, it was hypothesized that higher MVPA would predict better spatial working memory performance both directly, as well as indirectly via its effect on fitness and arterial stiffness. Additionally, the differential relationships amongst these variables were examined for people at lower and higher levels of fitness. It was hypothesized that the relationship between moderate-to-vigorous physical activity and spatial working memory would be primarily mediated by Six-Minute Walk score in the case of people with lower fitness and by carotid-femoral pulse wave velocity in the case of people with higher fitness.
Fig.1
Conceptual model. BMI, body mass index; MVPA, moderate-to-vigorous physical activity; 6 MW, Six-Minute Walk test; CFPWV, carotid-femoral pulse wave velocity; SWM, Spatial Working Memory.
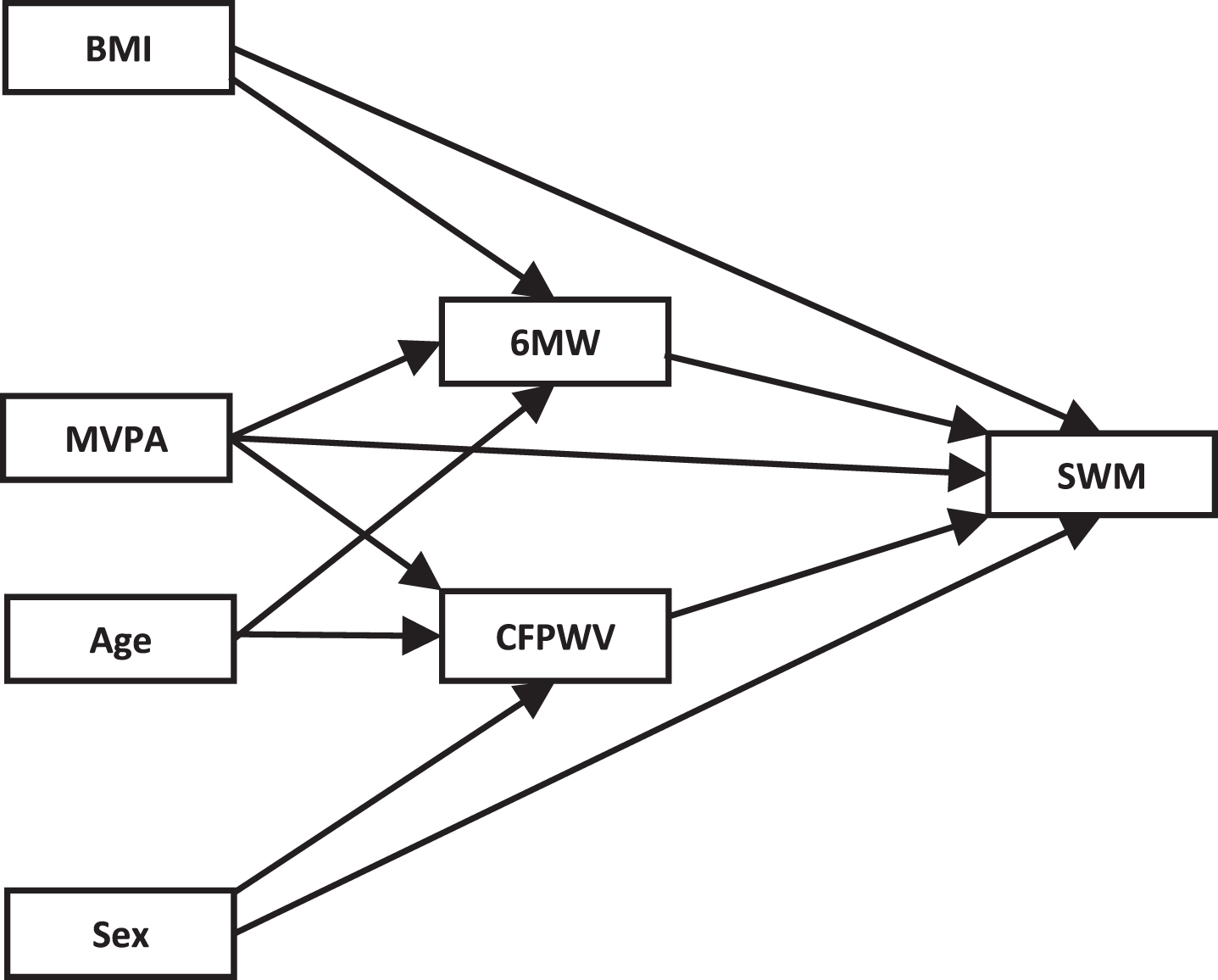
METHODS
Cross-sectional baseline data from the LIILAC trial [36] were used to further interrogate the previously established model of the relationships between fitness, aortic stiffness, and spatial working memory performance in older people.
Ethics
Ethical approval for this trial was obtained from the Swinburne University Human Research Ethics Committee – SUHREC 2013/057.The trial was also registered with the Australian New Zealand Clinical Trials Registry – ACTRN12614001133628. All procedures were conducted in accordance with the Declaration of Helsinki (as revised in 2004).
Participants
One hundred and two individuals, aged 60 to 90 (M = 77.5, SD = 6.9), living in independent living accommodation within retirement communities, participated in the trial. All participants in the LIILAC trial tested at baseline were included in this analysis; however, three participants did not complete the fitness assessment. The 73 females and 29 males in this study lived in their own residence within the community and were able to carry out their daily living activities without external support. All testing was conducted onsite at the residential community facilities.
To more accurately reflect the aging population, the use of medications, other than those drugs considered to be either illicit or cognitively enhancing, was not an exclusion criterion, as long as the dosages were stable for at least six months. However, prospective participants were excluded from the study if they scored less than 24 on the MMSE, indicating possible cognitive impairment; or more than nine on the Geriatric Depression Scale (GDS), indicating a significant level of depressive symptoms. Participants were also excluded if they had uncorrected visual impairment that limited their ability to perform the trial-based assessments, significant psychological or neurological disorders, or lacked the ability to speak and read English fluently. Finally, the ability to walk independently and safely, as assessed by their physician, was also a requirement for participation.
Cardiovascular assessment
Cardiovascular assessments were carried out with the SphygmoCor® XCEL device (AtCor Medical, Sydney, Australia). Participants initially rested for five minutes in a supine position. Brachial systolic, diastolic, and pulse pressures were then measured via a cuff affixed to the upper left arm. Central (aortic) blood pressures were also automatically derived by the device during this process. For greater accuracy measurements were taken three times. The first was discarded, as this had the greatest chance of being artificially elevated due to apprehension and ‘white-coat syndrome’, and the final results were taken as the average of the second and third measurements.
To derive the carotid-femoral pulse wave velocity (CFPWV) measure of aortic stiffness, a cuff was placed high on the left leg to capture the femoral pulse waveform, while the carotid waveform was captured using a hand-held tonometer. The distance between these two sites was measured and then divided by the pulse transit time to calculate the CFPWV. This is considered the ‘gold standard’ method of central arterial stiffness assessment [37].
Fitness and exercise assessment
Six-Minute Walk test
Fitness/functional capacity was assessed using the Six-Minute Walk test (6 MW) [38]. Participants were encouraged to walk back and forth between two markers placed 10 m apart as many times as possible, for a continuous period of 6 min. The total distance covered, to the nearest meter was then recorded [39]. This submaximal test of aerobic capacity [40] has been shown to be a simple, reliable and practical method of evaluating fitness, particularly in those that may have physical limitations [41, 42].
Community healthy activities model program for seniors
Exercise was assessed using the Community Healthy Activities Model Program for Seniors (CHAMPS) Physical Activity Questionnaire for Older Adults [43], a tool designed specifically to assess the physical activity of older adults. The CHAMPS was designed to assess appropriate types of activities for older people, facilitate accurate reporting, reduce socially desirable responding as much as possible, and be sensitive to change. This self-report activity questionnaire asked participants about 40 activities engaged in over the last four weeks. Calorific expenditure for each activity was then calculated using the summary of metabolic weights with selected items adjusted for older adults, with moderate-to-vigorous intensity physical activity related activates being classified as the subset with metabolic equivalent of task (MET) values≥3. Scoring was conducted using the amendments described in the revised codebook [44], which corrected for an omission error in the original scoring codebook [43], used to calculate the weekly expenditure of calories in moderate-to-vigorous physical activity (MVPA). The CHAMPS has been found to be a valid and reliable method of evaluating physical activity in older adults [45, 46].
Cognitive assessment
Cognitive performance was assessed using the Swinburne University Computerised Cognitive Assessment Battery (SUCCAB), which is well-validated against age [47] and sensitive to interventions [48–50]. This battery consists of the following tests, known to be sensitive to cognitive changes commonly associated with aging [51–53]: Simple Reaction Time, Choice Reaction Time, Immediate Recognition, Congruent Stroop, Incongruent Stroop, Spatial Working Memory, Contextual Memory, and Delayed Recognition. Detailed descriptions of the component tasks of the SUCCAB can be found in earlier papers by Pipingas and colleagues [47, 49, 54].
The SUCCAB was administered on an external screen attached to a laptop, to reduce distraction and ensure appropriate visibility. Participants were instructed to respond as fast and as accurately as possible using a 4-button response box. Each task was preceded by a truncated practice session to ensure they understood the nature of the test and how to appropriately respond.
The average response time and percentage accuracy for each task were calculated. To account for the well-known phenomena of older people sacrificing speed for accuracy, a performance score was then calculated by dividing the accuracy by the response time, with higher scores reflecting overall better performance. In order to better reflect general cognitive domains, rather than specific battery test scores, these performance scores were then combined into the following four broader cognitive domain scores, reflecting the Cattell-Horn-Carroll Broad Abilities model [55]: Reaction and Decision Speed (RDS), Cognitive Processing (CogP), Spatial Working Memory (SWM), and Visual Processing (VisP). This process has been detailed in the previous paper by Kennedy et al. [35].
Statistical analysis
The participants were divided into two groups with higher and lower levels of fitness, based on a median split of the 6 MW score. Independent samples t-tests were used to compare the characteristics of participants with higher and lower levels of fitness. These data were also used to further interrogate the previously established model of the relationships between fitness, aortic stiffness and spatial working memory performance in older people using structural equation modelling, with invariance testing used to compare the relationships between these variables for people with higher and lower levels of fitness. This allows a holistic comparison of the factors influencing SWM in participants with higher and lower levels of fitness. Goodness of fit statistics used for the structural equation modelling included the chi-square statistic and the normed chi-square. As recommended by Hu and Bentler [56], adequate model fit requires normed chi-square between 1 and 2. Invariance was assessed using a chi-squared statistic to compare the fitted models for the two groups. The structural models for each group were then refined with the removal of non-significant paths. The analysis was conducted using IBM SPSS and AMOS version 25. A G-Power analysis showed that, with the relatively small sample sizes for the two groups, only large effect sizes (f2 = 0.35) can be detected.
RESULTS
Overall means and standard deviations for the demographic, cardiovascular, cognitive, and exercise variables/measures are presented in Table 1 for the combined and separate fitness groups.
Table 1
Demographic, cardiovascular, cognitive and exercise characteristics – including fitness group differences
Total (n = 102) | Lower Fitness (n = 49) | Higher Fitness (n = 50) | |
Demographics &Morphometrics | |||
Age, y | 77.5 (6.9) | 79.9 (5.9) ** | 75.1 (7.0) ** |
Education, y | 12.7 (3.5) | 12.3 (3.7) | 13.2 (3.3) |
MMSE | 28.49 (1.34) | 28.61 (1.34) | 28.42 (1.34) |
BMI, kg/m2 | 28.29 (4.69) | 29.35 (4.54)∧ | 27.06 (4.28)∧ |
% males | 39.73 | 41.43 | 42.86 |
Cardiovascular | |||
BSBP, mmHg | 135.72 (15.90) | 137.50 (17.85) | 135.00 (13.73) |
DBP, mmHg | 70.35 (10.27) | 68.96 (10.82) | 72.20 (9.24) |
BPP, mmHg | 65.38 (12.63) | 68.54 (13.95)∧ | 62.80 (10.74)∧ |
CSBP, mmHg | 123.73 (14.53) | 124.04 (16.59) | 122.34 (12.31) |
CPP, mmHg | 51.88 (10.68) | 53.21 (12.01)∧ | 48.96 (8.97)∧ |
CFPWV, m/sec | 11.51 (1.94) | 11.70 (1.71) | 11.39 (2.15) |
Cognitive Domains | |||
RDS | 0.03 (0.79) | –0.09 (0.79) | 0.17 (0.78) |
CogP | 0.00 (1.00) | –0.75 (0.91) | 0.03 (1.09) |
VisP | 0.00 (0.86) | –0.04 (0.81) | 0.05 (0.94) |
SWM | 0.00 (1.00) | –0.29 (0.82)* | 0.28 (1.11)* |
Fitness &Exercise | |||
6 MW, m | 356.16 (75.59) | 307.58 (53.33) ** | 421.59 (45.57) ** |
MVPA, cal/week | 1511.30 (1841.59) | 1253.13 (1518.88) | 1824.93 (2131.10) |
Mean (SD) reported. Education, years of formal education; MMSE, Mini-Mental State Examination; BMI, body mass index; BSBP, brachial systolic blood pressure; DBP, diastolic blood pressure; BPP, brachial pulse pressure; CSBP, central systolic blood pressure; CPP, central pulse pressure; AP, augmented pressure; AI, augmented index; CFPWV, carotid-femoral pulse wave velocity; RDF, Reaction and Decision Speed; CogP, Cognitive Processing; VisP, Visual Processing; SWM, Spatial Working Memory; 6 MW, Six-Minute Walk test; MVPA, moderate-to-vigorous physical activity. Significant differences between lower and higher fitness groups indicated: ∧p≤0.05, *p≤0.01, **p≤0.001.
Correlations among the model variables are shown in Table 2 for the combined and separate fitness groups. A path model, as shown in Fig. 2, was developed to investigate the effect of the inclusion of quantity of moderate exercise into the original model previously developed by Kennedy et al. [35]. This model included all participants and showed a good fit, χ2 (8) = 8.393, p = 0.396, normed chi-square = 1.049. Standardized direct, indirect and total effect sizes for the model are shown in Table 3. Similarly to the original model, both 6 MW and CFPWV have a large direct effect on SWM, with the effect of the 6 MW being 28% stronger than that of CFPWV. The addition of MVPA added little to the overall model, with the adjusted model explaining only an additional 1% of the variation in SWM.
Table 2
Correlations among model variables for the Overall, Lower Fitness and Higher Fitness groups
BMI | MVPA | Age | Sex | 6MW | CFPWV | |
Overall | ||||||
MVPA | –0.149 | |||||
Age | –0.038 | –0.274 ∧ | ||||
Sex | –0.065 | –0.259 ∧ | –0.083 | |||
6MW | –0.339 ** | 0.243 ∧ | –0.382 ** | –0.003 | ||
CFPWV | –0.086 | –0.034 | 0.308 * | –0.325** | –0.036 | |
SWM | 0.082 | 0.228 ∧ | –0.367 ** | –0.237 ∧ | 0.374** | –0.238 ∧ |
Lower Fitness | ||||||
MVPA | 0.006 | |||||
Age | –0.345 ∧ | –0.105 | ||||
Sex | 0.184 | –0.134 | –0.210 | |||
6MW | –0.235 | 0.291 ∧ | –0.274 | 0.051 | ||
CFPWV | –0.280 | 0.165 | 0.051 | –0.352 ∧ | 0.085 | |
SWM | 0.144 | –0.007 | –0.306 ∧ | –0.153 | 0.271 | –0.162 |
Higher Fitness | –0.181 | |||||
Age | 0.145 | –0.288 | ||||
Sex | –0.369 * | –0.348 ∧ | –0.020 | |||
6MW | –0.230 | 0.128 | –0.140 | –0.030 | ||
CFPWV | 0.010 | –0.134 | 0.507 ** | –0.300 ∧ | –0.007 | |
SWM | 0.156 | 0.311 ∧ | –0.292 ∧ | –0.315 ∧ | 0.257 | –0.269 |
Pearson’s correlation coefficients reported. 6 MW, Six-Minute Walk test distance; CFPWV, carotid-femoral pulse wave velocity; MVPA, moderate-to-vigorous physical activity; BMI, body mass index; SWM, Spatial Working Memory. ∧p≤0.05, *p≤0.01, **p≤0.001.
Fig.2
Overall model for the physical fitness and arterial stiffness contribution to the variability in SWM with the addition of moderate exercise. Standardized regression coefficients (β) are shown for each path and the percentage of variation explained is shown for each dependent variable. BMI, body mass index; MVPA, moderate-to-vigorous physical activity; 6 MW, Six-Minute Walk test; CFPWV, carotid-femoral pulse wave velocity; SWM, Spatial Working Memory.
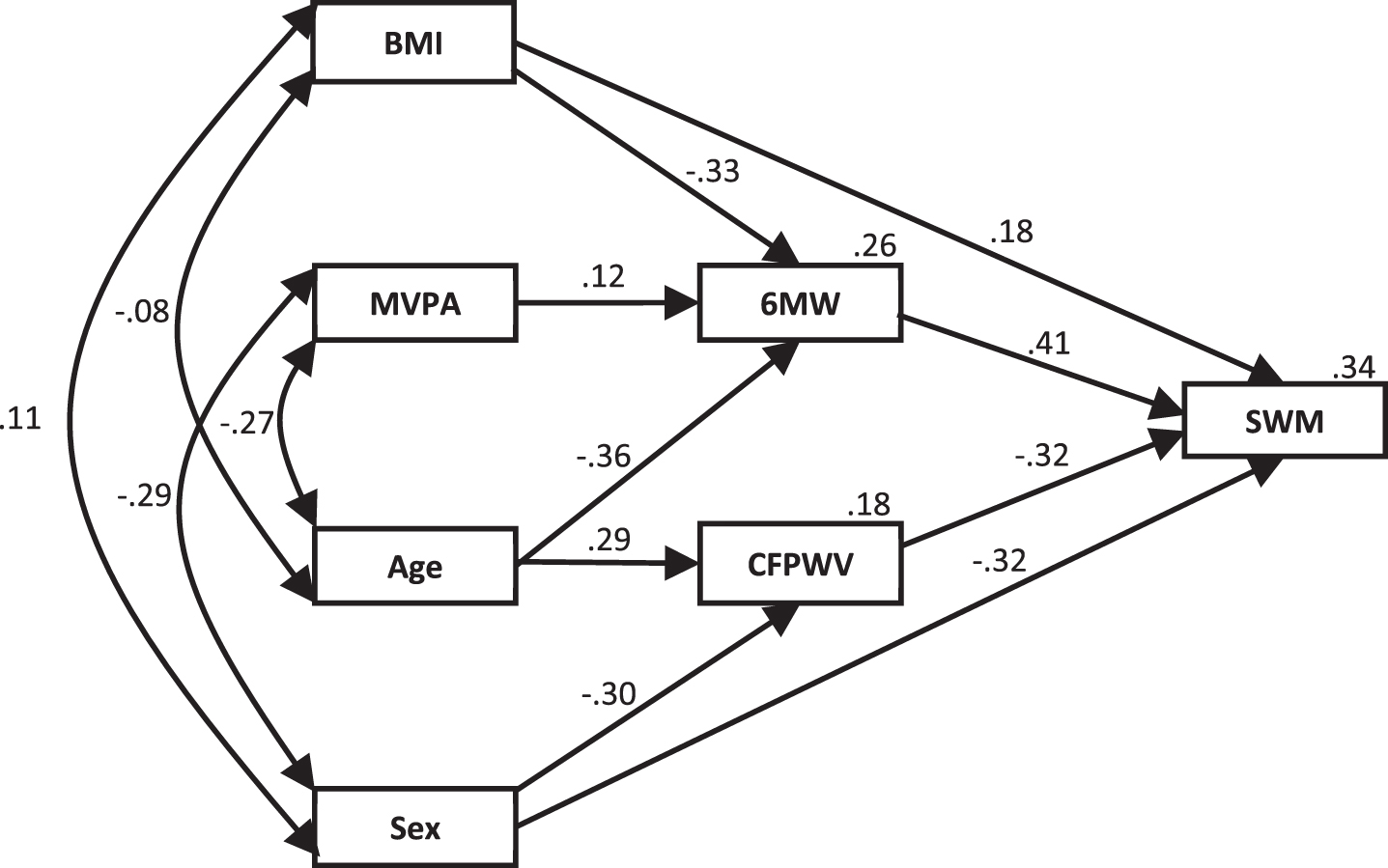
Table 3
Standardized effect sizes from Fig. 2 for SWM
Direct | Indirect | Total | |
6MW | 0.415 | 0.000 | 0.415 |
CFPWV | –0.316 | 0.000 | –0.316 |
MVPA | 0.000 | 0.051 | 0.051 |
Age | 0.000 | –0.214 | –0.241 |
Sex | –0.320 | 0.096 | –0.224 |
BMI | 0.178 | –0.137 | 0.041 |
BMI, body mass index; MVPA, moderate-to-vigorous physical activity; 6 MW, Six-Minute Walk test; CFPWV, carotid-femoral pulse wave velocity; SWM, Spatial Working Memory.
To explore whether level of fitness differentially influenced the contribution the variables in this expanded SWM model separately for the two fitness groups. Significant differences were found for the estimated parameters of these fitted models, χ2 (9) = 17.795, p = 0.038.
The Lower-Fitness model, Fig. 3, showed good fit, χ2 (14) = 19.433, p = 0.149, normed chi-square = 1.388, once non-significant paths were removed. This model explained 10% of the variation in SWM, with 6 MW being the main predictor and CFPWV no longer significantly contributing to the model. BMI, age and MVPA were all significant contributors to the model, however their effects on SWM were all totally mediated by 6 MW. The direct effect of sex on SWM remained significant, although the strength of this relationship was approximately halved in comparison to the overall model. Standardized direct, indirect and total effect sizes for the model are shown in Table 4.
Fig.3
Lower-fitness model for the physical fitness and arterial stiffness contribution to the variability in SWM with the addition of moderate exercise. Standardized regression coefficients (β) are shown for each path and the percentage of variation explained is shown for each dependent variable. Dotted lines indicate non-significant pathways that were significant in the overall model. BMI, body mass index; MVPA, moderate-to-vigorous physical activity; 6 MW, six-minute walk test; CFPWV, carotid-femoral pulse wave velocity; SWM, spatial working memory.
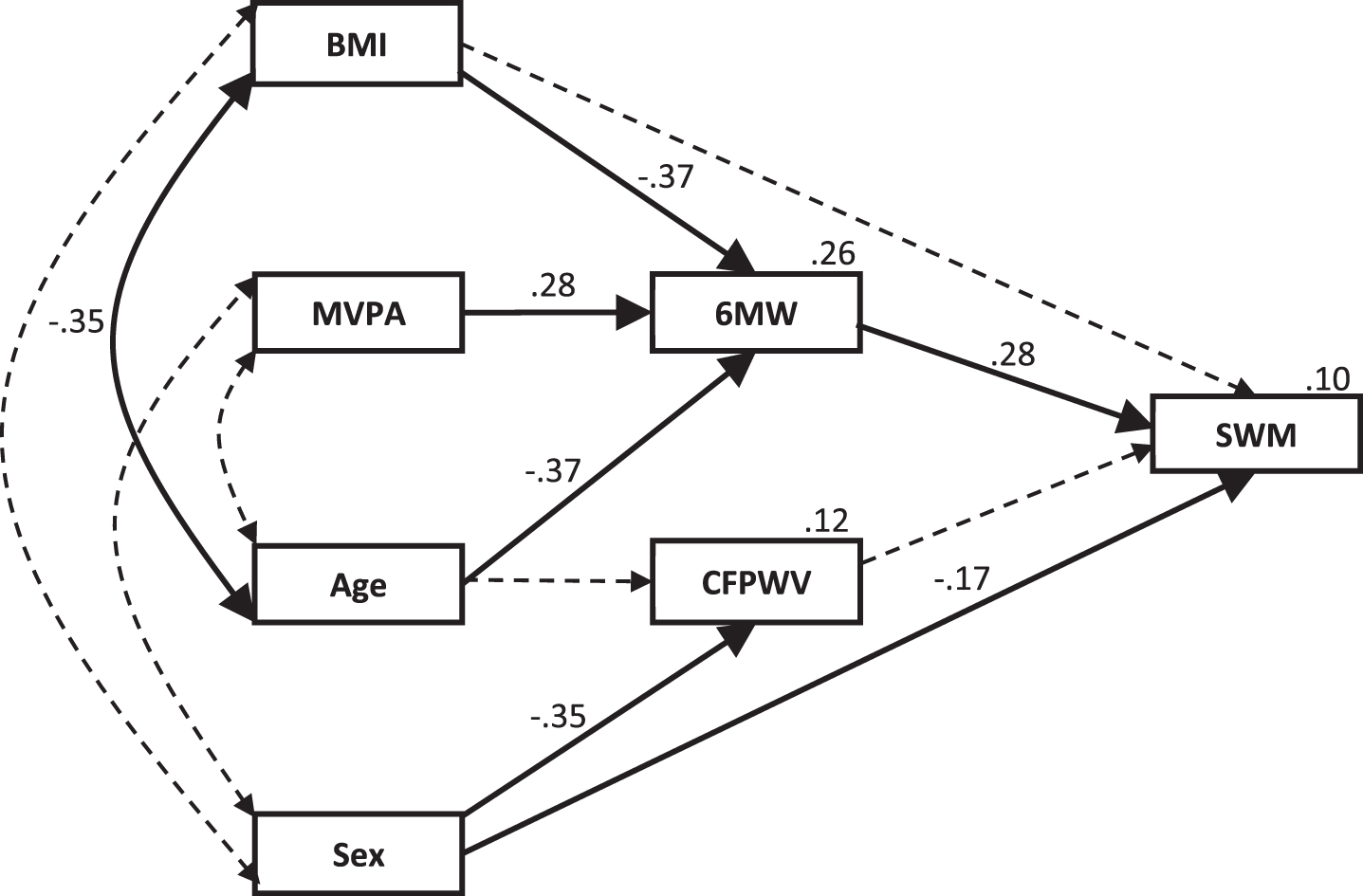
Table 4
Standardized effect sizes from Fig. 3 for SWM
Direct | Indirect | Total | |
6MW | 0.277 | 0.000 | 0.277 |
CFPWV | 0.000 | 0.000 | 0.000 |
MVPA | 0.000 | 0.076 | 0.076 |
Age | 0.000 | –0.104 | –0.104 |
Sex | –0.167 | 0.000 | –0.167 |
BMI | 0.000 | –0.103 | –0.103 |
BMI, body mass index; MVPA, moderate-to-vigorous physical activity; 6 MW, Six-Minute Walk test; CFPWV, carotid-femoral pulse wave velocity; SWM, Spatial Working Memory.
The Higher-Fitness model, Fig. 4, also showed good fit, χ2 (14) = 14.871, p = 0.387, normed chi-square = 1.062, once non-significant paths were removed. This model explained 31% of the variation in SWM. As opposed to the Lower-Fitness model, CFPWV strongly predicted SWM in this model, with CFPWV totally mediating the effect of age and partially mediating the effect of sex. 6 MW also remained a significant predictor of SWM. However, BMI, MVPA, and age were no longer significant predictors of 6 MW, nor did they contribute significantly, either directly or indirectly, to the variation in SWM. Sex showed the strongest of all direct relationships with SWM in this model. Standardized direct, indirect and total effect sizes for the model are shown in Table 5.
Fig.4
Higher-fitness model for the physical fitness and arterial stiffness contribution to the variability in SWM with the addition of moderate exercise. Standardized regression coefficients (β) are shown for each path and the percentage of variation explained is shown for each dependent variable. Dotted lines indicate non-significant pathways that were significant in the overall model. BMI, body mass index; MVPA, moderate-to-vigorous physical activity; 6 MW, six-minute walk test; CFPWV, carotid-femoral pulse wave velocity; SWM, spatial working memory.
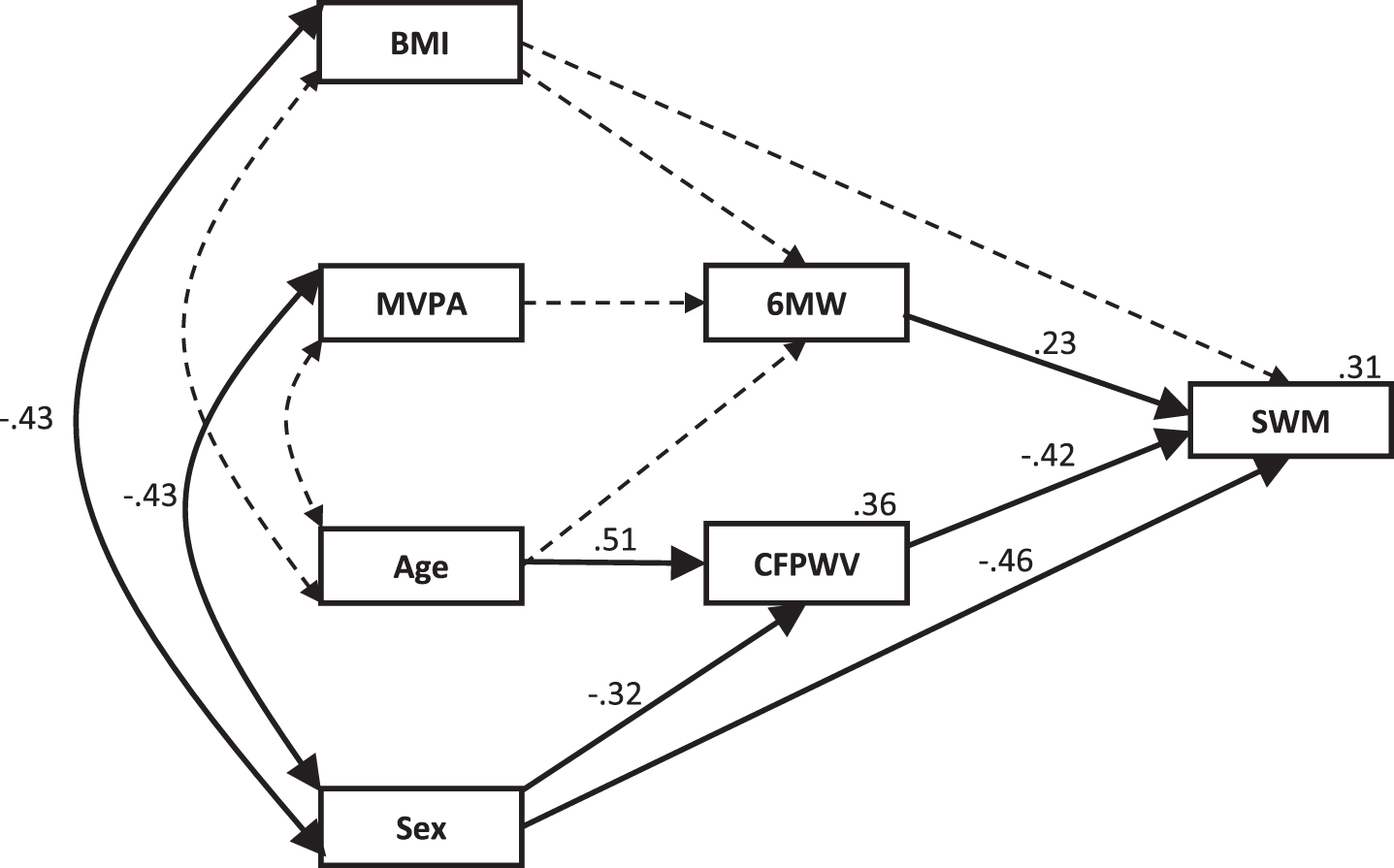
Table 5
Standardized effect sizes from Fig. 4 for SWM
Direct | Indirect | Total | |
6MW | 0.235 | 0.000 | 0.235 |
CFPWV | –0.416 | 0.000 | –0.416 |
MVPA | 0.000 | 0.000 | 0.000 |
Age | 0.000 | –0.211 | –0.211 |
Sex | –0.457 | 0.135 | –0.323 |
BMI | 0.000 | 0.000 | 0.000 |
BMI, body mass index; MVPA, moderate-to-vigorous physical activity; 6 MW, Six-Minute Walk test; CFPWV, carotid-femoral pulse wave velocity; SWM, Spatial Working Memory.
DISCUSSION
Previous modelling of data from the LIILAC trial found that both higher fitness and lower aortic stiffness independently predicted better SWM in older people [35]. This study further investigated the effects of fitness and aortic stiffness on cognitive performance, in particular the effect that physical activity has on the previously developed model. The first hypothesis, that higher MVPA would predict better SWM, both directly and indirectly through fitness and arterial stiffness, was partially supported. Greater engagement in physical activity did indirectly explain better SWM through its effect on fitness, although the overall effect size (0.051) was very small. However, contrary to expectations, physical activity had no significant direct effect on SWM, nor indirectly through any relationship with aortic stiffness. This means that the small effect that greater engagement in moderate-to-vigorous physical activity had on predicting better SWM performance in older people, may be totally explained by higher levels of physical activity predicting better fitness.
Secondly, when the relationships in this model were examined differentially between participants of lower-fitness and those of higher-fitness, physical activity only predicted SWM through its effect on fitness for less fit people, which was in line with expectations. It was also found that the effect of age on SWM was completely explained by its relationship with fitness in this group, with aortic stiffness no longer a significant predictor of SWM. However, contrary to expectations, for participants with higher levels of fitness, there was no significant effect on physical activity on SWM, either directly, or indirectly through aortic stiffness, nor was there any significant effect through fitness. Additionally, the effect of age on SWM was no longer partially explained by its effect on fitness, but was solely mediated by its strong relationship to aortic stiffness (0.51). These results are in line with the other recent findings which proposed that moderate-to-vigorous physical activity has a beneficial effect on cognition in older people up to a certain point, after which the returns on greater energy expenditure diminish [33].
These findings suggest that there is a small, but tangible advantage in older, cognitively intact people engaging in more physical activity of at least moderate intensity to preserve cognitive function. As this relationship is not evident in this study for those of moderate to high levels of fitness, this effect may be limited to those of lower fitness. It should be noted, however, that the current study is limited by its cross-sectional nature and by the relatively small sample size. Additionally, although current fitness was measured objectively, we relied on subjective reports of engagement in physical activity over the previous month only. However, the CHAMPS has been validated against accelerometer measurements in the Seniors Quality of Life longitudinal study. In particular the MVPA showed the highest association with accelerometry minutes of corresponding intensity in seniors. The CHAMPS has, however, has been shown to require further refinement for lower sedentary and low-to-light levels of physical activity [46].
Current fitness and short-term engagement in physical activity may not accurately represent the engagement in exercise and levels of fitness across different stages of participants’ lives, nor the time-courses over which these may affect cognitive outcomes later in life. There is also a realistic probability that the rate of aortic stiffening may be reduced in those who maintain higher levels of exercise and physical fitness over long periods of time, particularly from middle age [18, 57].
Conversely, a strength of this study was the involvement of a cohort residing in independent living aged care communities, with testing conducted at the participants’ facility of residence rather than in a university, laboratory, or hospital setting. As well as affording an element of ecological validity, this allowed for a reduction in the potential for influences of variation in social isolation and living conditions, as well as capturing a broader sample of the independently living older community, as participants who were unable or unwilling to travel were able to participate. Also, given that the majority of older people in developed countries take some form of medication [58, 59], the inclusion of people on stable doses of medications allowed the study to reflect a more typical aging population than many studies which are more restrictive in their medication inclusion criteria.
Overall, the structural models derived from this study reinforce the predominant assertion that maintaining physical fitness is a factor in maintaining cognitive health in older people. However, these findings also indicate that increased engagement in moderate-vigorous levels of physical activity may not be an overly important aspect of the maintenance of physical and cognitive health, particularly in those who are already moderately fit. Furthermore, while aortic stiffness was not a significant contributor to SWM for those of lower fitness, it was the most important potentially modifiable factor in predicting SWM performance for fitter people. This suggests that future interventions aiming to ameliorate the rate of cognitive decline in the elderly may benefit from an increased focus on attempting to reduce aortic stiffness, particularly in fitter older adults who may not receive as much cognitive benefit from further increasing their fitness level. While neither current fitness nor engagement in higher levels of physical activity had any effect on aortic elasticity in this study, there is some evidence from other studies that dietary and pharmacological interventions may be successful in improving aortic health [60, 61].
In summary, while the overall model shows that the influence of age on SWM performance can be totally explained by a combination of its effect on fitness and aortic stiffness, these findings from the fitness level models suggest that interventions to preserve cognitive function may need to be prioritized differentially depending an individual’s level of fitness. Less fit older individuals should potentially prioritize improving their fitness level, at least in part by engaging in more physical activity, to preserve their cognitive function. However, fitter older people would likely benefit by interventions which have the potential to reduce aortic stiffness. Further investigation involving larger longitudinal and interventional studies in this area, including additional factors which may influence fitness and aortic stiffness, would further elucidate the relationships established in these models and potentially lead to better intervention strategies to reduce the rate of cognitive decline and risk of dementias such as AD in older people.
CONFLICT OF INTEREST
The authors declare that the research was conducted in the absence of any commercial or financial relationships that could be construed as a potential conflict of interest.
ACKNOWLEDGMENTS
The resources of Centre for Human Psychopharmacology, Swinburne University of Technology, Melbourne, have been used to conduct this study. Collection and storage of biological sample were provided by Dorevitch Pathology. Access to participants and facilities was provided by Australian Unity Ltd, BaptCare Ltd, Churches of Christ in Queensland Ltd., and Catholic Homes Ltd. This study would not be possible without these supporters, and it is greatly appreciated by the Swinburne LIILAC team.
Additional review and advice on the development and structure of this manuscript was provided by Mr. Roy Groncki and Mr. Jeffery Reddan.
REFERENCES
[1] | Australian Institute of Health and Welfare ((2017) ) Care needs in residential aged care, AIHW, Canberra. |
[2] | Livingston G , Sommerlad A , Orgeta V , Costafreda SG , Huntley J , Ames D , Ballard C , Banerjee S , Burns A , Cohen-Mansfield J , Cooper C , Fox N , Gitlin LN , Howard R , Kales HC , Larson EB , Ritchie K , Rockwood K , Sampson EL , Samus Q , Schneider LS , Selbæk G , Teri L , Mukadam N ((2017) ) Dementia prevention, intervention, and care. Lancet 390: , 2673–2734. |
[3] | Deary IJ , Corley J , Gow AJ , Harris SE , Houlihan LM , Marioni RE , Penke L , Rafnsson SB , Starr JM ((2009) ) Age-associated cognitive decline. Br Med Bull 92: , 135–152. |
[4] | de la Torre JC ((2004) ) Is Alzheimer’s disease a neurodegenerative or a vascular disorder? Data, dogma, and dialectics. Lancet Neurol 3: , 184–190. |
[5] | van Norden AGW , van Dijk EJ , de Laat KF , Scheltens P , Olderikkert MGM , de Leeuw FE ((2012) ) Dementia: Alzheimer pathology and vascular factors: From mutually exclusive to interaction. Biochim Biophys Acta 1822: , 340–349. |
[6] | Barnes DE , Yaffe K , Satariano WA , Tager IB ((2003) ) A longitudinal study of cardiorespiratory fitness and cognitive function in healthy older adults. J Am Geriatr Soc 51: , 459–465. |
[7] | Edwards MK , Loprinzi PD ((2017) ) Combined associations of sedentary behavior and cardiorespiratory fitness on cognitive function among older adults. Int J Cardiol 229: , 71–74. |
[8] | Stimpson NJ , Davison G , Javadi A-H ((2018) ) Joggin’ the Noggin: Towards ahysiological understanding of exercise-induced cognitive benefits. Neurosci Biobehav Rev 88: , 177–186. |
[9] | Segaert K , Lucas SJE , Burley CV , Segaert P , Milner AE , Ryan M , Wheeldon L ((2018) ) Higher physical fitness levels are associated with less language decline in healthy ageing. Sci Rep 8: , 1–10. |
[10] | de la Torre JC ((2002) ) Alzheimer disease as a vascular disorder: Nosological evidence. Stroke 33: , 1152–1162. |
[11] | Cavalcante JL , Lima JAC , Redheuil A , Al-Mallah MH ((2011) ) Aortic stiffness: Current understanding and future directions. J Am Coll Cardiol 57: , 1511–1522. |
[12] | Wilkinson IB , McEniery CM ((2012) ) Arteriosclerosis: Inevitable or self-inflicted? Hypertension 60: , 3–5. |
[13] | Najjar SS , Scuteri A , Lakatta EG ((2005) ) Arterial aging: Is it an immutable cardiovascular risk factor? Hypertension 46: , 454–462. |
[14] | O’Rourke MF , Safar ME ((2005) ) Relationship between aortic stiffening and microvascular disease in brain and kidney: Cause and logic of therapy. Hypertension 46: , 200–204. |
[15] | Stone J , Johnstone DM , Mitrofanis J , O’Rourke M ((2015) ) The mechanical cause of age-related dementia (Alzheimer’s disease): The brain is destroyed by the pulse. J Alzheimers Dis 44: , 355–373. |
[16] | Singer J , Trollor JN , Baune BT , Sachdev PS , Smith E ((2014) ) Arterial stiffness, the brain and cognition: A systematic review. Ageing Res Rev 15: , 16–27. |
[17] | van Sloten TT , Protogerou AD , Henry RMA , Schram MT , Launer LJ , Stehouwer CDA ((2015) ) Association between arterial stiffness, cerebral small vessel disease and cognitive impairment: A systematic review and meta-analysis. Neurosci Biobehav Rev 53: , 121–130. |
[18] | Kennedy G , Hardman RJ , MacPherson H , Scholey AB , Pipingas A ((2017) ) How does exercise reduce the rate of age-associated cognitive decline? A review of potential mechanisms. J Alzheimers Dis 55: , 1–18. |
[19] | Kramer AF , Erickson KI , Colcombe SJ ((2006) ) Exercise, cognition, and the aging brain. J Appl Physiol 101: , 1237–1242. |
[20] | Niemann C , Godde B , Staudinger UM , Voelcker-Rehage C ((2014) ) Exercise-induced changes in basal ganglia volume and cognition in older adults. Neuroscience 281: , 147–163. |
[21] | Norton S , Matthews FE , Barnes DE , Yaffe K , Brayne C ((2014) ) Potential for primary prevention of Alzheimer’s disease: An analysis of population-based data. Lancet Neurol 13: , 788–794. |
[22] | Ashby-Mitchell K , Burns R , Shaw J , Anstey KJ ((2017) ) Proportion of dementia in Australia explained by common modifiable risk factors. Alzheimers Res Ther 9: , 1–8. |
[23] | Stuckenschneider T , Askew CD , Rüdiger S , Polidori MC , Abeln V , Vogt T , Krome A , Olde Rikkert M , Lawlor B , Schneider S ((2018) ) Cardiorespiratory fitness and cognitive function are positively related among participants with mild and subjective cognitive impairment. J Alzheimers Dis 62: , 1865–1875. |
[24] | Sexton CE , Betts JF , Demnitz N , Dawes H , Ebmeier KP , Johansen-Berg H ((2016) ) A systematic review of MRI studies examining the relationship between physical fitness and activity and the white matter of the ageing brain. Neuroimage 131: , 81–90. |
[25] | Vibha D , Tiemeier H , Mirza SS , Adams HHH , Niessen WJ , Hofman A , Prasad K , Lugt A van der , Vernooij MW , Ikram MA ((2018) ) Brain volumes and longitudinal cognitive change: A population-based study. Alzheimer Dis Assoc Disord 32: , 43–49. |
[26] | Jiang J , Paradise M , Liu T , Armstrong NJ , Zhu W , Kochan NA , Brodaty H , Sachdev PS , Wen W ((2018) ) The association of regional white matter lesions with cognition in a community-based cohort of older individuals. Neuroimage Clin 19: , 14–21. |
[27] | Reddan JM , Macpherson H , White DJ , Scholey A , Pipingas A ((2018) ) Examining the relationship between nutrition and cerebral structural integrity in older adults without dementia. Nutr Res Rev 32: , 79–98. |
[28] | Komulainen P , Kivipelto M , Lakka TA , Savonen K , Hassinen M , Kiviniemi V , Hänninen T , Rauramaa R ((2010) ) Exercise, fitness and cognition – A randomised controlled trial in older individuals: The DR’s EXTRA study. Eur Geriatr Med 1: , 266–272. |
[29] | Northey JM , Cherbuin N , Pumpa KL , Smee DJ , Rattray B ((2018) ) Exercise interventions for cognitive function in adults older than 50: A systematic review with meta-Analysis. Br J Sports Med 52: , 154–160. |
[30] | Peven JC , Grove GA , Jakicic JM , Alessi MG , Erickson KI ((2018) ) Associations between short and long bouts of physical activity with executive function in older adults. J Cogn Enhanc 2: , 137–145. |
[31] | Brasure M , Desai P , Davila H , Nelson VA , Calvert C , Jutkowitz E , Butler M , Fink HA , Ratner E , Hemmy LS , McCarten JR , Barclay TR , Kane RL ((2018) ) Physical activity interventions in preventing cognitive decline and alzheimer-type dementia a systematic review. Ann Intern Med 168: , 30–38. |
[32] | Farrell SW , Abramowitz AR , Willis BL , Barlow CE , Weiner M , Falkowski J , Leonard D , Pavlovic A , Defina LF ((2018) ) The relationship between cardiorespiratory fitness and montreal cognitive assessment scores in older adults. Gerontology 64: , 440–445. |
[33] | Gregory MA , Gill DP , Petrella RJ ((2013) ) Brain health and exercise in older adults. Curr Sports Med Rep 12: , 256–271. |
[34] | Pantzar A , Jonasson LS , Ekblom Ö , Boraxbekk CJ , Ekblom MM ((2018) ) Relationships between aerobic fitness levels and cognitive performance in Swedish office workers. Front Psychol 9: , 1–9. |
[35] | Kennedy G , Meyer D , Hardman RJ , Macpherson H , Scholey AB , Pipingas A ((2018) ) Physical fitness and aortic stiffness explain the reduced cognitive performance associated with increasing age in older people. J Alzheimers Dis 63: , 1307–1316. |
[36] | Hardman RJ , Kennedy G , Macpherson H , Scholey AB , Pipingas A ((2015) ) A randomised controlled trial investigating the effects of Mediterranean diet and aerobic exercise on cognition in cognitively healthy older people living independently within aged care facilities: The Lifestyle Intervention in Independent Living Aged Care (LIILAC) study protocol [ACTRN12614001133628]. Nutr J 14: , 53. |
[37] | Mattace-Raso FUS , Hofman A , Verwoert GC , Wittemana JCM , Wilkinson I , Cockcroft J , McEniery C , Yasmina , Laurent S , Boutouyrie P , Bozec E , Hansen TW , Torp-Pedersen C , Ibsen H , Jeppesen J , Vermeersch SJ , Rietzschel E , de Buyzere M , Gillebert TC , van Bortel L , Segers P , Vlachopoulos C , Aznaouridis C , Stefanadis C , Benetos A , Labat C , Lacolley P , Stehouwer CDA , Nijpels G , Dekker JM , Ferreira I , Twisk JWR , Czernichow S , Galan P , Hercberg S , Pannier B , Guérin A , London G , Kennedy Cruickshank J , Anderson SG , Paini A , Rosei EA , Muiesan ML , Salvetti M , Filipovsky J , Seidlerova J , Dolejsova M ((2010) ) Determinants of pulse wave velocity in healthy people and in the presence of cardiovascular risk factors: ‘Establishing normal and reference values.’. Eur Heart J 31: , 2338–2350. |
[38] | Bean JF , Kiely DK , Leveille SG , Herman S , Huynh C , Fielding R , Frontera W ((2002) ) The 6-minute walk test in mobility-limited elders: What is being measured? J Gerontol A Biol Sci Med Sci 57: , M751–M756. |
[39] | Troosters T , Gosselink R , Decramer M ((1999) ) Six minute walking distance in healthy elderly subjects. Eur Respir J 14: , 270–274. |
[40] | Steffen T , Hacker T , Mollinger L ((2002) ) Age and gender-related test performance in community-dwelling elderly people: Six-Minute Walk Test, Berg Balance Scale, Timed Up & Go Test, and Gait Speeds. Phys Ther 82: , 128–137. |
[41] | Chatterjee AB , Rissmiller RW , Meade K , Paladenech C , Conforti J , Adair NE , Haponik EF , Chin R ((2009) ) Reproducibility of the 6-minute walk test for ambulatory oxygen prescription. Respiration 79: , 121–127. |
[42] | Young J , Angevaren M , Rusted J , Tabet N ((2015) ) Aerobic exercise to improve cognitive function in older people without known cognitive impairment. Cohrane Database Syst Rev 4: , 1–145. |
[43] | Stewart AL , Mills KM , King AC , Haskell WL , Gillis D , Ritter PL ((2001) ) CHAMPS physical activity questionnaire for older adults: Outcomes for interventions. Med Sci Sports Exerc 33: , 1126–1141. |
[44] | CHAMPS Community Health Activity Model Program for Seniors, Revised Codebook for CHAMPS Physical Activity Measures. |
[45] | Harada ND , Chiu V , King AC , Stewart AL ((2001) ) An evaluation of three self-report physical activity instruments for older adults. Med Sci Sport Exerc 33: , 962–970. |
[46] | Hekler EB , Buman MP , Haskell WL , Conway TL , Cain KL , Sallis JF , Saelens BE , Frank LD , Kerr J , King AC ((2012) ) Reliability and validity of CHAMPS self-reported sedentary-to-vigorous intensity physical activity in older adults. J Phys Act Health 9: , 225–236. |
[47] | Pipingas A , Harris E , Tournier E , King R , Kras M , Stough CK ((2010) ) Assessing the efficacy of nutraceutical interventions on cognitive functioning in the elderly. Curr Top Nutraceutical Res 8: , 79–88. |
[48] | Simpson T , Camfield D , Pipingas A , Macpherson H , Stough C ((2012) ) Improved processing speed: Online computer-based cognitive training in older adults. Educ Gerontol 38: , 445–458. |
[49] | Macpherson H , Ellis KA , Sali A , Pipingas A ((2012) ) Memory improvements in elderly women following 16 weeks treatment with a combined multivitamin, mineral and herbal supplement: A randomized controlled trial. Psychopharmacology (Berl) 220: , 351–365. |
[50] | Pase MP ((2014) ) PhD Thesis: Modifiable hemodynamic predictors of cognitive ageing. |
[51] | Thorne DR ((2006) ) Throughput: A simple performance index with desirable characteristics. Behav Res Methods 38: , 569–573. |
[52] | Brebion G ((2001) ) Language processing, slowing, and speed/accuracy trade-off in the elderly. Exp Aging Res 27: , 137–150. |
[53] | Smith GA , Brewer N ((1995) ) Slowness and age: Speed-accuracy mechanisms. Psychol Aging 10: , 238–247. |
[54] | Pipingas A , Silberstein RB , Vitetta L , Rooy C Van , V E , Young JM , Frampton CM , Sali A , Nastasi J ((2008) ) Improved cognitive performance after dietary supplementation with a Pinus radiata bark extract formulation. Phyther Res 22: , 1168–1174. |
[55] | McGrew KS ((2009) ) CHC theory and the human cognitive abilities project: Standing on the shoulders of the giants of psychometric intelligence research. Intelligence 37: , 1–10. |
[56] | Hu L , Bentler PM ((1999) ) Cutoff criteria for fit indexes in covariance structure analysis: Conventional criteria versus new alternatives. Mutidisciplinary J 6: , 1–55. |
[57] | Ahmadi-Abhari S , Sabia S , Shipley MJ , Kivimäki M , Singh-Manoux A , Tabak A , McEniery C , Wilkinson IB , Brunner EJ ((2017) ) Physical activity, sedentary behavior, and long-term changes in aortic stiffness: The Whitehall II study. J Am Heart Assoc 6: , e005974. |
[58] | Kaufman DW , Kelly JP , Rosenberg L , Anderson TE , Mitchell AA ((2002) ) Recent patterns of medication use in the ambulatory adult population of the United States: The Slone survey. JAMA 287: , 337–344. |
[59] | Elliott R ((2006) ) Problems with medication use in the elderly: An Australian perspective. J Res Pharm Pract 36: , 58–66. |
[60] | Ong K-T , Delerme S , Pannier B , Safar ME , Benetos A , Laurent S , Boutouyrie P ((2011) ) Aortic stiffness is reduced beyond blood pressure lowering by short-term and long-term antihypertensive treatment: A meta-analysis of individual data in 294 patients. J Hypertens 29: , 1034–1042. |
[61] | Boutouyrie P , Lacolley P , Briet M , Regnault V , Stanton A , Laurent S , Mahmud A ((2011) ) Pharmacological modulation of arterial stiffness. Drugs 71: , 1689–1701. |