Long-Term Non-Invasive Treatment via Intranasal Administration of Nerve Growth Factor Protects the Human Brain in Frontotemporal Dementia associated with Corticobasal Syndrome: A Pilot Study
Abstract
Background:
Nerve growth factor (NGF) is known for playing a critical protective role on a number of brain neurons in mammals, including humans. NGF can be delivered to the CNS via nasal route and has a neuroprotective action in case of neurodegenerative diseases.
Objective:
The aim of this study is to investigate for the first time whether purified NGF can play a neuroprotective role on human brain neurons affected by neurodegenerative diseases when administered via nasal route.
Methods:
Two female patients, both affected by frontotemporal dementia (FTD) associated with corticobasal syndrome (CBS) at different stages of disease progression, received a daily intranasal NGF spray for one year. Clinical/neurological aspects were observed over time. The follow-up study was performed using 18 FDG PET.
Results:
This case study seems to demonstrate that IN-NGF slows down the common decline caused by FTD/CBS.
Conclusions:
These findings suggest the potential neuroprotective role of IN-NGF administered in case of neurodegenerative diseases.
INTRODUCTION
Nerve growth factor (NGF) is the first discovered and best characterized member of the neurotrophin family [1], known for playing a critical protective role in the development and survival of sympathetic, sensory and basal forebrain cholinergic neurons in mammals, including humans [2–4]. For over three decades, studies conducted on NGF have been focusing on its biochemical and molecular characterization. Since the discovery by Rita Levi-Montalcini, other researchers demonstrated the potential therapeutic role of NGF in the treatment of human diseases in the central nervous system (CNS) and peripheral nervous system (PNS) [5–7], and some trials have shown encouraging results. Inevitable loss of neurons and synapses in neurodegenerative diseases occurs over time, leading to cognitive decline. NGF plays a neuroprotective role for basal forebrain cholinergic neurons, improves memory, and reduces cognitive deficits in animal models of Alzheimer’s disease [3, 8–10]. NGF does not cross the blood-brain barrier if injected subcutaneously or intravenously; therefore, an alternative delivery method is required. Intranasal delivery of NGF has so far been sufficiently investigated in animal models [11–21] and only recently in humans, as demonstrated in a recent study on intranasal administration of NGF in a brain injury [22]. Some of these studies demonstrated that NGF has the ability to rescue recognition memory deficits in mice [16] and to slow amyloid neurodegeneration [12]. Intranasal administration is the most effective and non-invasive way to deliver NGF to the CNS [11, 17–19]; NGF has a neuroprotective action in Alzheimer’s and Parkinson’s diseases, as showed by several studies in animal models and humans [10, 12, 16, 24–27]. Furthermore, recent studies have shown an active link between the nasal pathway and the spinal cord in the delivery of NGF to the CNS, thus demonstrating the neuroprotective ability of NGF to support injured neurons in a model of spinal cord injury [13–15, 21]. Different ways of direct delivery of NGF to the CNS have been investigated in humans and animal models, including direct CNS infusion [26, 28], gene therapy approaches [24–25], cell-based delivery using stem cells [29], and application of an encapsulated cell biodelivery device [30]. All these approaches have the restriction of being invasive. Hence, the aim of this study is to investigate for the first time whether purified NGF would play a neuroprotective role on human brain neurons affected by neurodegenerative diseases when administered via nasal cavity.
What is nerve growth factor?
NGF is the founding member of the neurotrophins family of proteins. In 1953, while working in the Victor Hamburger laboratory at Washington University, Rita Levi-Montalcini discovered that sarcoma tissue in mice produced a soluble factor that promoted the growth of nearby sensory and sympathetic ganglia [1, 5]. Working on a team with Stanley Cohen, they isolated the substance responsible for this phenomenon and named it nerve growth factor. In the CNS, the greatest amount of NGF is produced in the following brain areas: cortex, hippocampus, the basal ganglia, thalamus, and the pituitary gland; significant quantities of this neurotrophin are also produced in other areas, including spinal cord and in the retina [31]. Many cells of the immune-hematopoietic system also produce and utilize NGF: keratinocytes, fibroblasts, and mast cells [32]. Furthermore, the largest source of NGF is inside the mouse salivary glands [33]. The biological action of NGF is mediated by two NGF-receptors, the historically recognized high-affinity receptor trkANGFR, having a tyrosine kinase activity, and the low-affinity transmembrane glycoprotein, a non-selective pan-neurotrophin receptor p75NTR that regulates signaling through trkANGFR [34]. The effect of NGF on target cells depends on the ratio of these two receptors co-distributed on cell surface [35]. More recent studies have shown a novel interaction between trkANTR and CD2-associated protein (CD2AP) during NGF-induced signaling. These data place CD2AP as a major intracellular signaling molecule coordinating NGF signaling to regulate collateral sprouting and structural plasticity of intact adult axons [36]. Moreover, CD2AP would seem to be a genetic risk factor for Alzheimer’s disease [37].
Frontotemporal dementia
Frontotemporal dementia (FTD) is a neurodegenerative disease characterized by atrophy and progressive loss of nerve cells in the frontal and temporal lobes of the brain resulting in a gradual and progressive decline in behavior and/or language [38–39]. It was previously known as Pick’s disease and considered rare [38–39]. FTD is now one of the most common forms of dementia in persons younger than 65 years [40]. About 55% of patients have FTD with ubiquitin-positive inclusions while 45% have FTD with tau-positive inclusions. FTD with Pick’s bodies is still called Pick’s disease [41]. Recent studies have identified the presence of TAR DNA–binding protein (TDP-43), which may be the primary disease protein underlying the ubiquitin-positive cases [42]. The most common neuropathologic substrates of frontotemporal lobar degeneration (FTLD) are FTLD-tau, FTLD-TDP, and FTLD-FUS. The three genes most commonly associated with FTD are C9ORF72, MAPT, and GRN [38, 43, 44].
There are three subtypes/variant of frontotemporal dementia: 1) behavioral variant FTD; 2) Primary progressive aphasia (PPA): nonfluent/agrammatic variant, semantic variant, logopenic variant (a recent variant usually associated with Alzheimer’s disease) [23, 45–47]; and 3) Motor associated disorders FTD: corticobasal syndrome (CBS), progressive supranuclear palsy, and FTD with motor neuron disease.
This study analyzes the effects of intranasal NGF administration in two cases of FTD associated with CBS at different stages of disease.
Corticobasal syndrome
CBS is a progressive neurological disorder that may involve the motor system, cognition or both [48–49]. Typically, it starts as a movement disorder, with affected individuals showing a unilateral paucity of movement and muscle rigidity with or without a tremor. Previously considered as a distinct clinicopathologic entity [50], then termed corticobasal degeneration (CBD) [51]. The pathology of CBD is predicted antemortem in only 25% to 56% of cases [52–53]. Clinicopathologic studies have since revealed that the originally described clinical features of CBD, now called CBS, are often due to other pathologies. CBS is often associated with FTD and progressive aphasia [54], and the extrapyramidal-apractic syndrome of CBS often appears in FTD and PPA [49, 54–55]. From recent studies, 4 CBD phenotypes emerged: CBS, frontal behavioral-spatial syndrome, nonfluent/agrammatic variant of primary progressive aphasia, and progressive supranuclear palsy syndrome [56].
Motor signs of CBS [49] include akinesia/bradykinesia, rigidity, tremor, and limb dystonia. The cognitive associated signs [49] are alien hand syndrome, apraxia, acalculia, and visual-spatial impairment. CBS language associated signs [49] include progressive aphasia.
METHODS
The rationale of nasal route delivery
Nasal delivery is easy and non-invasive and the olfactory mucosa is in direct contact with the brain and cerebrospinal fluid by the olfactory and trigeminal pathways [57].
An accurate literature review shows that NGF (and other large molecules) can reach neurons of the CNS, particularly the brain, via nasal route [11, 17–19]. These studies (Frey II et al.) led to a new non-invasive solution of reaching the CNS via nasal route. Subsequent studies about intranasal delivery of NGF and others molecules NGF-like to the brain have reinforced the concept [15, 16, 20, 21]. The anatomical connection between the nasal cavity and central nervous system structures consists of two possible routes. One is associated with the peripheral olfactory system connecting the nasal passage with the olfactory bulbs and rostral brain regions; the other goes through the peripheral trigeminal system connecting the nasal passage with the brainstem and spinal cord region [15, 21, 57, 58]. Following these tracks and driven by the need to use a non-invasive method of delivering NGF to the CNS, we tested the effect of intranasal administration of purified NGF on two patients, both affected by FTD/CBS but at different stages of disease progression (early stage; middle stage).
NGF purification and administration
The NGF was purified in our laboratory from submaxillary salivary gland of the adult male mice, following Bocchini and Angeletti method and stored at –70°C until used [59]. Patients received 10 μl of NGF (concentration 200 μg/ml) dissolved in physiological saline solution daily. 5 μl per each nostril daily (via nasal spray) for a 1-year period (range 12–18 months). For further details of purification see: Lambiase et al. [60].
Study design, setting, and treatment intensity
The subjects included four female patients affected by FTD/CBS, aged 58 to 64 years and with an average duration of FTD/CBS diagnosis of 8±4 years. Informed consent was provided by their caregivers. The study was approved by the Maria Rosaria Maglione Foundation Human Research Protection Program Ethics Committee (Protocol-no: 1405-NGF-07). History, neurological examination, review of magnetic resonance brain imaging, and PET-scan within recent years, clinical dementia rating, Mini-Mental State Examination (MMSE), Simpson-Angus scale, and verbal fluency test were performed. Two female patients at different stages of FTB/CBS disease (early stage; middle stage) received NGF via nasal route daily (1 spray per nostril once daily over a 12–18 months period, two daily sprays) and no other drug was administered. Memantine and idebenone therapy was discontinued 6 months before the beginning of NGF-treatment in the experimental NGF-study Group (Case 1; Case 2), in order to be clearly and sufficiently convinced that the observed effect was due to the action of NGF. The control group consisted of two female FTD/CBS patients (early stage; middle stage) treated using memantine and idebenone therapy only. During the treatment, purified NGF was stored at +4°C for daily use. Clinical/neurological aspects were observed over time. The authors have limited the long-term administration of NGF to once daily, 1 spray per nostril (two daily sprays) in the morning to avoid the undesired effects (as reported in Table 1).
Table 1
Dose-dependent adverse effects
Dosage Testing: 5 μl of NGF per spray | 2 daily sprays (1 spray per nostril) | 4 daily sprays (2 sprays per nostril) | 6 daily sprays (3 sprays per nostril) |
Mild Rhinitis (in Case 1) | Mild Rhinitis (in Case 1) | Rhinitis (in Case 1) | |
Slight Rigidity (in Case 1) | Rigidity (in both Cases) | ||
Moderate Psychomotor agitation (in both Cases) |
Dosage testing: The long-term administration of NGF was limited only 1 time daily, 1 spray per nostril (two daily sprays) in the morning to avoid the undesired effects. Patients received 10 μl of NGF (concentration 200 μg/ml) dissolved in physiological saline solution daily, 5 μl of NGF per each nostril daily for 1 year period. Increasing dosage some adverse effects have occurred as reported in Table 1. All adverse effects were attenuated 3-4 h after onset.
Case 1
Start of IN-NGF-Study (2008): Female aged 63 in middle stage of FTD/CBS. Clinical Dementia Rating (CDR) scores 2.0 = moderate. Non-L-dopa responsive extrapyramidal syndrome on the right side (upper limb>lower limb) without tremor and with stiffness and shakiness of the right arm, paraphasias (progressive aphasia), akinesia, poor coordination, apraxia, agraphia, acalculia, visual-spatial deficit. Start of disease (2000): short-term memory deficit, hesitant and halting speech, dysgraphia, dyscalculia, non-L-dopa responsive extrapyramidal syndrome on the right side (upper limb>lower limb) without tremor and with stiffness and shakiness of the right arm. Anti-varicella zoster virus antibodies high, VZV/IgG = 780 mIU/ml (reference values:<135 mIU/mL = negative;>165 mIU/mL = positive); VZV/IgM = negative. Heart arrhythmia in treatment with beta-blockers. Advanced disease: rigidity at 4 limbs, myoclonus, epileptic crisis, mutism. Duration of disease: 18 years (2000-present).
Case 2
Start of IN-NGF-Study (2008): Female aged 58 in early stage of FTD/CBS. CDR scores 1.0 = mild. Non-L-dopa responsive extrapyramidal syndrome on the right side with a mild right hand tremor, dysgraphia, dyscalculia, speech disorder (progressive aphasia). Start of disease (2004): short-term memory deficit, hesitant and halting speech, dysgraphia, dyscalculia, non-L-dopa responsive extrapyramidal syndrome on the right side. Advanced disease: rigidity at 4 limbs, myoclonus, mutism. Absence of other associated pathologies. Duration of disease: 14 years (2004-present).
Control group
Two female FTD/CBS patients treated using memantine and idebenone therapy only. Case 3: Start NFG-study (2008): Aged 60, in early stage (CDR scores 1.0 = mild) of FTD/CBS. Signs and symptoms overlapping with Case 2. Duration of disease: 2004-present. Case 4: Start NGF-study (2008): Aged 64, in middle stage (CDR scores 2.0 = moderate) of FTD/CBS. Signs and symptoms overlapping with Case 1. Duration of disease: 2000–2014. Absence of other associated pathologies in both patients.
Amyloid PET-scan was negative in all patients.
Search antibodies included: anti-VZV, anti-HSV-1, anti-HSV-2, anti-HSV-6, anti-CMV, anti-EBV.
Search result: High values of anti-VZV in Case 1 (no previous history of encephalitis), no other antibodies in all patients (NGF-treated and untreated). The author’s guidelines always require the search for antiviral antibodies, according to the new trends in literature [61–63]. In recent years, evidence of the microbial origin of various chronic inflammatory disorders, including several neurodegenerative, neuropsychiatric and other systemic disorders, has been steadily growing. New and historical observations provide evidence of an association between Alzheimer’s disease and certain infectious agents. The same hypothesis could be applied to all neurodegenerative diseases.
PET-scan study
Follow-up was performed using 18 FDG PET-scan. Exam technique: PET images were performed on an empty stomach 30 min after intravenous injection of 300 MBq 18 FDG. Images of tracer distribution were acquired over a 30–60 min period. The tomographic images were acquired in the axial, coronal and sagittal sections with a thickness of 4.25 mm. Flame scale indicates FDG use/100 g tissue/min. Red color indicates more 18 FDG-use than blue.
Statistical analysis
Statistical evaluations were performed using the GraphPad 5 software (GraphPad Software Inc., San Diego, CA, USA) and the data were expressed as mean±SEM. Comparison of pre-treatment and post-treatment rates (MMSE and Verbal Fluency test) were made using paired t-tests, with a significance level of p-value<0.05. PET statistics: Regionally specific effects were compared using linear contrasts. The resulting voxel values represented a parametric mapping of t-statistics, SPM (t), which were transformed into the unit normal distribution SPM (z) and thresholded at t = 2.12, p < 0.05. Resulting foci were characterized in peak height (u). The significance of each region was estimated based on the probability that the observed peak height could have occurred by chance p (Zmax >u).
RESULTS
Clinical aspects
The long-term treatment via IN-NGF showed an enhancement of brain performances in both cases, improving cognitive and motor abilities. Patients were more responsive, had slight improvement in speech and in motor rigidity. A greater responsiveness (mood and eye opening) was observed during the first 15–20 days of each monthly treatment. Over time the denaturation of the molecule pushed down neurological performances (mood and eye opening).
Cognitive assessment
Cognitive dysfunction in patients affected by FTD/CBS does not always change linearly, but the patients who participated to this study showed a cognitive decline which resulted linear to the intermediate stage of the disease. MMSE scores before NGF-treatment showed a mean annual rate of decline 4.0±2.0 points per year, based on pre-treatment assessment over a 14 months period (range 12–18 months). In the post-treatment phase, the mean rate of MMSE decline was 2.0±1.0 points per year in Case 2; a patient in an early stage of the disease who showed a 50% reduction of MMSE decline compared to the pre-treatment MMSE rate, i.e., a reversal of usual decline in neurodegenerative diseases. In Case 1, a patient in a middle stage of disease progression, the post NGF-treatment MMSE score was steady, unchanged and showed no decline compared with the pre-treatment MMSE score, i.e., a slow down of disease progression, considering the average rate of decline before NGF treatment. During the first 3 months of stopping NGF-treatment, a decrease in levels of attention and mood tone was observed. After 1 year of stopping NGF-treatment and no other conventional medication, there was a general worsening of the patients. MMSE scores worsened again with a mean annual rate of decline 4.0±2.5 points per year, based on post-treatment assessment over a 14 months period (range 12–18 months). This marked a clear return to pre-treatment conditions. Comparison between pre-treatment and post-treatment decline rates of the MMSE were made using paired t-tests, with a significance level of p < 0.05. The hypothesis of the positive effect due to NGF is also suggested by the case of two other patients (in the control group) who were treated with memantine and idebenone therapy and did not show similar improvements. In these untreated NGF patients, the average MMSE decline rate continued to decrease 4.0±2.7 points per year.
Rigidity assessment
Rigidity assessment was performed using the Simpson-Angus Scale, currently used to assess pseudoparkinsonism. The severity grades of each item are rated using a 5-point scale. We focused only on the evaluation of elbow rigidity. The elbow joints are separately bent at right angles and passively extended and flexed, with the subject’s biceps observed and simultaneously palpated. The resistance to this procedure has also been rated. Post-treatment assessment (after a 14-month period of NGF-treatment) showed a slight improvement in rigidity: a reduction of 1.0 in both patients, compared with pre-treatment assessment over a 14 months period (range 12–18 months). In Case 1 (patient in middle stage), rigidity improved from marked to moderate. In Case 2 (patient in early stage), rigidity improved from moderate to slight. The control group did not show similar improvements. After 1 year of stopping NGF-treatment and no other medication, there was a clear return to pre-treatment conditions.
Speech assessment
Post-treatment Verbal Fluency Test (after 14 months NGF-treatment) showed a score improvement of 2.0±1.0 points in NGF-treated patients, compared to the pre-treatment score (range 12–18 months). Comparison of pre-treatment and post-treatment improvement rates were made using paired t-tests, with a significance level of p < 0.05. In detail, Case 1 (middle stage) showed improvement in word recruitment and a decrease in phonemic paraphasias; e.g., “wap” for wrap or “triger” for tiger or “deks” for desk. Case 2 (early stage) showed improvement in fluency and word recruitment. The control group did not show similar improvements. After 1 year of stopping NGF-treatment and no other medication, speech assessment showed a score worsening of 2.0±1.5 points. This marked a clear return to pre-treatment conditions.
Dose-dependent adverse effect
The following undesirable effects were observed: 1) temporary adverse effects with one spray administration per nostril once daily (two daily sprays): mild rhinitis; and 2) temporary/dose-dependent adverse effects in case of more than one spray per nostril daily: rhinitis, moderate psychomotor agitation, rigidity of the four limbs.
Dosage testing
Nasal administration once daily (1 spray per nostril) did not give significant side effects, except for a sporadic/mild rhinitis in a single case (Case 1). Conversely, increasing the dosage caused some side effects (as reported in Table 1). All adverse effects were attenuated within 3-4 h maximum following the administration. No side effects were observed in a 18–24 months period of follow-up after the last dose of NGF was administered.
PET-scan results
Before IN-NGF was administrated to the patients, PET-scans showed a reduction of FDG uptake in fronto-temporal areas (left>right), basal ganglia, cerebellum and occipital lobe in all patients (Figs. 1A and 2A). After 3 months of NGF treatment, the PET-study shows a significant increase in FDG-uptake compared to the first scans (p < 0.05) (Figs. 1B and 2B). After 1 year of NGF treatment, the PET-scans show a further enhancement of brain metabolic activity, compared to the previous PET (p < 0.05) and particularly in the following brain areas: fronto-temporal lobes, cingulate gyrus, thalamus, basal ganglia, para-hippocampal and para-olfactory area, cerebellum, dentate nucleus, occipital lobes, i.e., a reversal of usual decline in neurodegenerative diseases (Figs. 1C and 2C). One year after the interruption of NGF treatment (and no other medication), PET-images show a clear deterioration of the global framework, compared to the previous PETs. The brain areas show a significant reduction of the activities (Figs. 1D and 2D). Both NGF-treated patients showed a similar 18 FDG-PET evolution.
Fig.1
18 FDG PET-scan coronal sections. Matching of representative coronal sections showed a significant increase in the brain metabolism during NGF-treatment (10 μl NGF daily). Flame scale indicates FDG use/100 g tissue/min. Red color indicates more FDG-use than blue. A) Before treatment, note the FDG-uptake reduction in the following brain areas: frontotemporal lobes left>right, basal ganglia, cerebellum and occipital lobes. B) After 3 months of NGF-treatment (5 μl NGF per nostril daily), note a significant increase in FDG-uptake (p < 0.05) in the following brain areas: frontotemporal lobes, thalamus, basal ganglia, occipital lobes, cerebellum, and dentate nucleus. C) After 1 year of NGF-treatment (5 μl NGF per nostril daily), the PET scans showed further enhancement of FDG-uptake (p < 0.05) in the same brain areas as in (B) and also in the parahippocampal areas. D) After 1 year of stopping NGF-treatment, the brain areas showed a significant reduction in global activities.
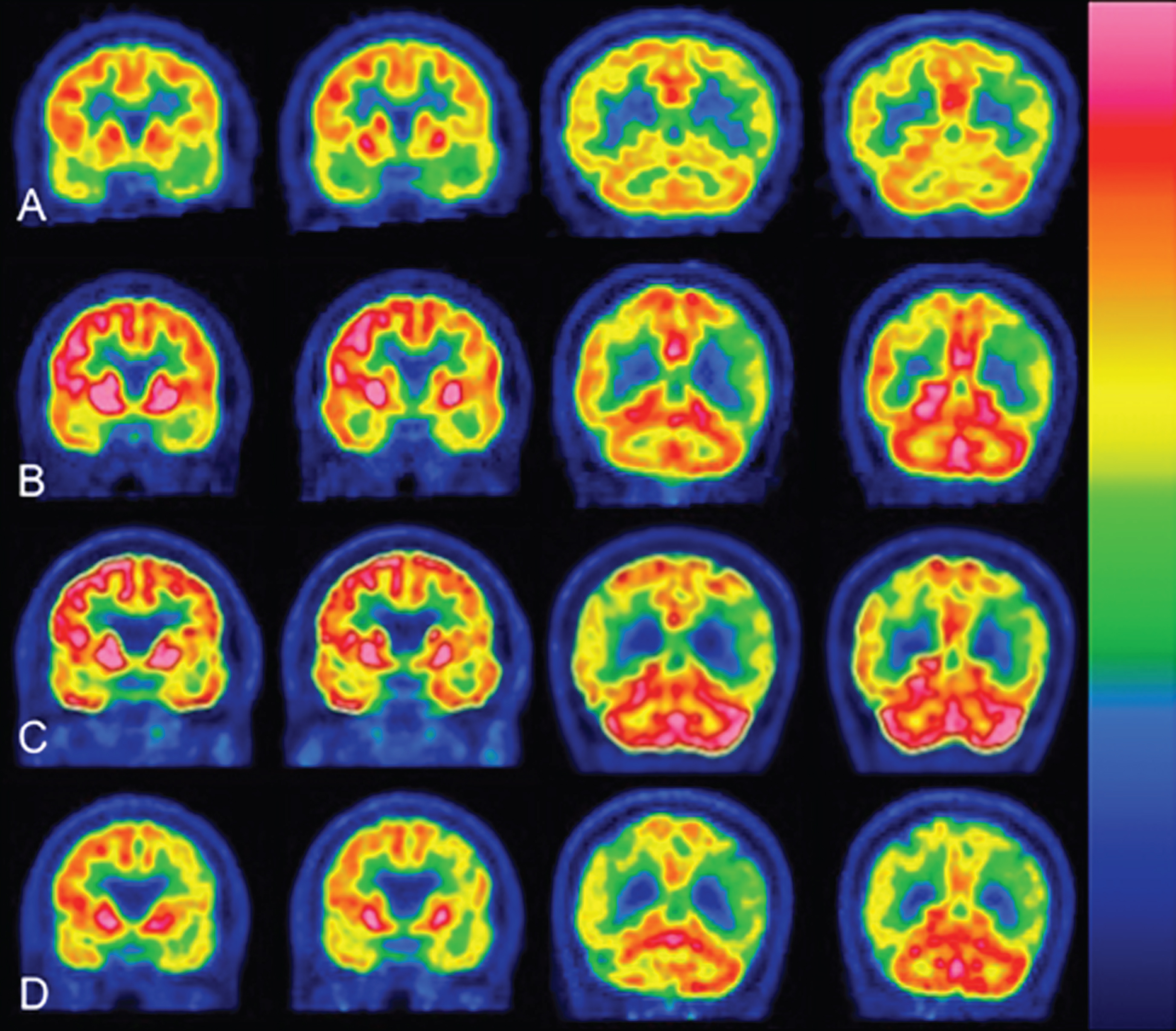
Fig.2
18 FDG PET-scan sagittal sections. Matching of representative sagittal sections showed a significant increase in the brain metabolism during NGF-treatment (10 μl NGF daily). Flame scale indicates FDG use/100 g tissue/min. Red color indicates more FDG-use than blue. A) Before treatment, note FDG-uptake reduction in the following brain areas: frontotemporal lobes left>right, basal ganglia, cerebellum. B) After 3 months of NGF-treatment (5 μl NGF per nostril daily), note a significant increase in FDG-uptake (p < 0.05) in the occipital and frontotemporal lobes. C) After 1 year of NGF-treatment (5 μl NGF per nostril daily), the PET scans showed further enhancement of FDG-uptake (p < 0.05) in the same brain areas as in (B) and also in paraolfactory area, posterior cortex of cingulate gyrus, cerebellum. D) After 1 year of stopping NGF-treatment, the brain areas showed a significant reduction in global activities.
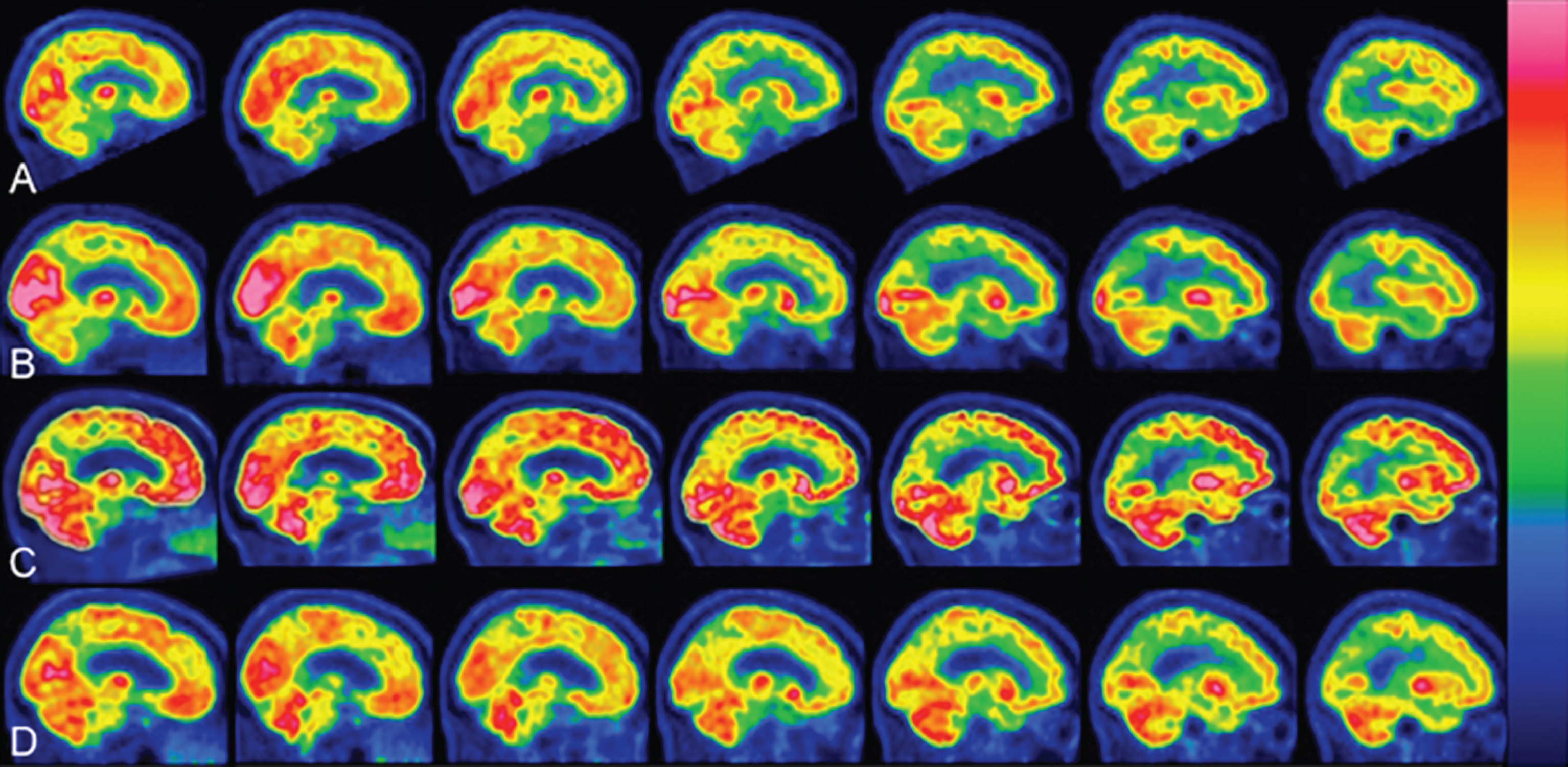
DISCUSSION
As reported above, NGF is an endogenous neurotrophin that exerts trophic and differentiative activity on neurons of the central and peripheral nervous systems with neuroprotective and regenerative effects observed in neurodegenerative diseases or following injury. Intranasal administration of NGF in animal models has shown to protect neurons of the brain and spinal cord from neurodegenerative diseases and/or spinal cord injury [13, 15, 16, 21, 58]. Numerous data show the protective role of NGF in case of neurodegenerative diseases in human models too. Despite that, invasive routes of administration are not optimal for clinical use [24–26, 28, 30], i.e., direct CNS infusion [26, 28], gene therapy approaches [24, 25], the application of an encapsulated cell biodelivery device [30]. In this study we report the case of two patients, both affected by FTD/CBS, who had clinical improvements after intranasal administration of NGF in each nostril for one year (range 12–18 months) and without reporting significant side effects except for rhinitis, rigidity and moderate psychomotor agitation (as reported in Table 1). Any adverse effect was attenuated 3-4 h after the administration and no side effects were observed in a 18–24 months period of follow-up after the last dose of NGF was administered. This study aims at showing how NGF would slow down the common decline of FTD/CBS, such as it was observed in PET-study after 3 months of treatment and confirmed in PET-study and in cognitive, rigidity and speech assessment after 1 year of NGF-treatment (range 12–18 months). These neuroprotective properties of NGF make it a strong candidate for the future treatment of neurodegenerative diseases (FTD/CBS-like). However, the clinical observation presented in this report needs to be confirmed on a large scale clinical trial to better understand the potential neuroprotective role of NGF in neurodegenerative diseases and other pathologies of central nervous system (brain injury, spinal cord injury, ischemic damage) when administered via nasal route. This pilot study confirms the possibility of using NGF via nasal route in order to reach human brain neurons in safe, non-invasive way, supporting the potential clinical use of intranasal NGF therapy for neurodegenerative diseases (as FTD/CBS) which are currently considered untreatable. We are aware that NGF would not be able to cure the FTD/CBS but our observations support the hypothesis that NGF slows down the usual decline of the disease. In the last years some authors have demonstrated the possibility to synthesize in a laboratory a recombinant human NGF for clinical use [64]. Some clinical trials are currently working on it [65]. This innovation could solve the issue of NGF recruitment for clinical use.
Conclusions
A number of previous and recent findings have shown that NGF administration can protect basal forebrain cholinergic neurons and that the olfactory/trigeminal pathway is a promising and non-invasive route for drug delivery to the brain. This means choosing a safe route also for NGF to selected brain areas in case of neurodegenerative disease, with no significant side effects (as reported in Table 1). The present study showed that intranasal administration of NGF could slow down neurodegenerative progression and improve cognitive abilities in two female patients both affected by FTD/CBS at different stage of disease, in comparison with other NGF-untreated patients. For ethical reasons and based on the Declaration of Helsinki, control group patients were not treated with NGF vehicle solution (placebo), but were treated using memantine and idebenone therapy only and did not show improvement comparable to patients who received daily nasal NGF administration for 1 year and no other medication. Nonetheless, we are aware that in order to fully evaluate the potential neuroprotective role of recombinant human NGF, there is so much work to do and we intend to pursue these studies to fully characterize the role of NGF administration via nasal route in patients with neurodegenerative diseases. Meanwhile, we would like to call your attention to the novel findings obtained from our pilot study.
CONFLICT OF INTEREST
The authors have no conflict of interest to report.
ACKNOWLEDGMENTS
We thank the patients and their families who participated in this study. We are grateful to Maria Rosaria Maglione who was involved in this experimental study as a patient.
The study was performed in accordance with Declaration of Helsinki. The patients and their caregivers gave us written informed consent to start the experimental study and the publication of data. This study was performed (between 2008-2014) by the “Maria Rosaria Maglione Foundation onlus” (non-profit organization for Neuroscience) in collaboration with Dr. Lugi Aloe. The contribution of Luigi Aloe was supported by “Fondazione IRET onlus, Ozzano Emilia, Bologna, Italy”.
REFERENCES
[1] | Levi-Montalcini R ((1987) ) The nerve growth factor: Thirty-five years later. Science 237: , 1154–1162. |
[2] | Aloe L , Bracci-Laudiero L , Bonini S , Manni L ((1997) ) The expanding role of nerve growth factor: From neurotrophic activity to immunologic diseases. Allergy 52: , 883–894. |
[3] | Eriksdotter-Nilsson M , Skirboll S , Ebendal T , Hersh L , Grassi J , Massoulié J , Olson L ((1989) ) NGF treatment promotes development of basal forebrain tissue grafts in the anterior chamber of the eye. Exp Brain Res 74: , 89–98. |
[4] | Hefti F , Mash DC ((1989) ) Localization of NGF receptors in the normal human brain and Alzheimer’s disease. Neurobiol Aging 10: , 75–87. |
[5] | Aloe L , Rocco ML , Bianchi P , Manni L ((2012) ) Nerve growth factor: From the early discoveries to the potential clinical use. J Transl Med 10: , 239. |
[6] | Aloe L , Calzá L ((2004) ) NGF and related molecules in health and disease, Vol. 146: . Elsevier Science. |
[7] | Aloe L , Rocco ML , Balzamino BO , Micera A ((2015) ) Nerve growth factor: A focus on neuroscience and therapy. Curr Neuropharmacol 13: , 294–303. |
[8] | Hefti F ((1986) ) Nerve growth factor (NGF) promotes survival of septal cholinergic neurons after fimbrial transection. J Neurosci 6: , 2155–2162. |
[9] | Emerich DF , Winn SR , Harper J , Hammang JP , Baetge EE , Kordower JH ((1994) ) Implants of polymer-encapsulated human NGF-secreting cells in the non-human primate: Rescue and sprouting of degenerating cholinergic basal forebrain neurons. J Comp Neurol 349: , 148–164. |
[10] | Tuszynski MH1 , U HS , Amaral DG , Gage FH ((1990) ) Nerve growth factor infusion in primate brain reduces lesion-induced cholinergic neuronal degeneration. J Neurosci 10: , 3604–3614. |
[11] | Chen XQ , Fawcett JR , Rahman YE , Ala TA , Frey WH II ((1998) ) Delivery of nerve growth factor to the brain via the olfactory pathway. J Alzheimers Dis 1: , 35–44. |
[12] | Cattaneo A , Capsoni S , Paoletti F ((2008) ) Towards non-invasive nerve growth factor therapies for Alzheimer’s disease. J Alzheimers Dis 15: , 255–83. |
[13] | de Bellis A , Rocco ML , Bianchi P , Aloe L ((2012) ) Effect of intranasal nerve growth factor administration in rats with spinal cord injury. Progr Neurosci 1: , 83–90. |
[14] | de Bellis A , Aloe L ((2016) ) Intralnasa administration of nerve growth factor protects injured neurons in spinal cord injury: Pilot study. Proceedings of the 2016 Annual Scientific Meeting American Spinal Injury Association. Philadelphia, USA. Abstract ID 1490. |
[15] | de Bellis A , Aloe L ((2016) ) New non-invasive way to rescue neurons in spinal cord injury via intranasal administration of nerve growth factor: Pilot Study. Am J Neuroprotec Neuroregen 8: , 37–44. |
[16] | De Rosa R , Garcia AA , Braschi C , Capsoni S , Maffei L , Berardi N , Cattaneo A ((2005) ) Intranasal administration of nerve growth factor (NGF) rescues recognition memory deficits in AD11 anti-NGF transgenic mice. Proc Natl Acad Sci U S A 102: , 3811–3816. |
[17] | Frey WH II , Liu J , Thorne RG , Rahman Y-E ((1995) ) Intranasal delivery of 125I-NGF to the brain via the olfactory route. In Research Advances in Alzheimer’s Disease and Related Disorders, Iqbal K, Mortimer J, Winblad B, Wisniewski H, eds. John Wiley & Sons, New York, NY, pp. 329–335. |
[18] | Thorne RG , Emory CR , Ala TA , Frey WH II ((1995) ) Quantitative analysis of the olfactory pathway for drug delivery to the brain. Brain Res 692: , 278–282. |
[19] | Frey WH II , Liu J , Chen X , Thorne RG , Fawcett JR , Ala TA , Rahman Y-E ((1997) ) Delivery of 125I-NGF to the brain via the olfactory route. Drug Delivery 4: , 87–92. |
[20] | Alcala-Barraza SR , Lee MS , Hanson LR , McDonald AA , Frey 2nd WH , McLoon LK ((2010) ) Intranasal delivery of neurotrophic factors BDNF, CNTF, EPO, and NT-4 to the CNS. J Drug Target 18: , 179–190. |
[21] | de Bellis A , Aloe L ((2016) ) New non-invasive way to rescue neurons in spinal cord injury via intranasal administration of nerve growth factor: Pilot study. Proceedings of the Annual Scientific Meeting Society for Neuroscience, Novembe, 12-16; San Diego, USA. Session 499-Neurotrophins. 499.15: , G7. |
[22] | Chiaretti A , Conti G , Falsini B , Buonsenso D , Crasti M , Manni L , Soligo M , Fantacci C , Genovese O , Calcagni ML , Di Giuda D , Mattoli MV , Cocciolillo F , Ferrara P , Ruggiero A , Staccioli S , Colafati GS , Riccardi R ((2017) ) Intranasal nerve growth factor administration improves cerebral functions in a child with severe traumatic brain injury: A case report. Brain Inj 31: , 1538–1547. |
[23] | Matías-Guiu JA1 , Cabrera-Martín MN , Moreno-Ramos T , Valles-Salgado M , Fernandez-Matarrubia M , Carreras JL , Matías-Guiu J ((2015) ) Amyloid and FDG-PET study of logopenic primary progressive aphasia: Evidence for the existence of two subtypes. J Neurol 262: , 1463–1472. |
[24] | Tuszynski MH , Thal L , Pay M , Salmon DP , U HS , Bakay R , Patel P , Blesch A , Vahlsing HL , HO G , Tong G , Potkin SG , Fallon J , Hansen L , Mufson EJ , Kordower JH , Gall C , Conner J ((2005) ) A phase 1 clinical trial of nerve growth factor gene therapy for Alzheimer disease. Nat Med 11: , 551–555. |
[25] | Tuszynski MH , Yang JH , Barba D , U HS , Bakay RA , Pay MM , Masliah E , Conner JM , Kobalka P , Roy S , Nagahara AH ((2015) ) Nerve growth factor gene therapy: Activation of neuronal responses in Alzheimer disease. JAMA Neurol 72: , 1139–1147. |
[26] | Gill SS , Patel NK , Hotton GR , O’Sullivan K , McCarter R , Bunnage M , Brooks DJ , Svendsen CN , Heywood P ((2003) ) Direct brain infusion of glial cell line–derived neurotrophic factor in Parkinson disease. Nat Med 9: , 589–595. |
[27] | Aron L , Klein R ((2011) ) Repairing the parkinsonian brain with neurotrophic factors. Trends Neurosci 34: , 88–100. |
[28] | Eriksdotter-Jönhagen M , Nordberg A , Amberla K , Bäckman L , Ebendal T , Meyerson B , Olson L , Seiger Å , Shigeta M , Theodorsson E , Viitanen M , Winblad B , Wahlund L-O ((1998) ) Intracerebroventricular infusion of nerve growth factor in three patients with Alzheimer’s disease. Dement Geriatr Cogn Disord 9: , 246–257. |
[29] | Lee HJ , Lim IJ , Park SW , Kim YB , Ko Y , Su Kim ((2012) ) Human neural stem cells genetically modified to express human nerve growth factor (NGF) gene restore cognition in the mouse with ibotenic acid-induced cognitive dysfunction. Cell Transplant 21: , 2487–2496. |
[30] | Eyjolfsdottir H , Eriksdotter M , Linderoth B , Lind G , Juliusson B , Kusk P , Almkvist O , Andreasen N , Blennow K , Ferreira D , Westman E , Nennesmo I , Karami A , Darreh-Shori T , Kadir A , Nordberg A , Sundström E , Wahlund LO , Wall A , Wiberg M , Winblad B , Seiger Å , Wahlberg L , Almqvist P ((2016) ) Targeted delivery of nerve growth factor to the cholinergic basal forebrain of Alzheimer’s disease patients: Application of a second generation encapsulated cell biodelivery device. Alzheimers Res Ther 8: , 30. |
[31] | McAllister AK ((2001) ) Neurotrophins and neuronal differentiation in the central nervous system. Cell Mol Life Sci 58: , 1054–1060. |
[32] | Aloe L , Levi-Montalcini R ((1977) ) Mast cells increase in tissues of neonatal rats injected with the nerve growth factor. Brain Res 133: , 358–366. |
[33] | Murphy RA , Saide JD , Blanchard MH , Young M ((1977) ) Nerve growth factor in mouse serum and saliva: Role of the submandibular gland. Proc Natl Acad Sci U S A 74: , 2330–2333. |
[34] | Huang EJ , Reichardt LF ((2003) ) Trk receptors: Roles in neuronal signal transduction. Annu Rev Biochem 72: , 609–642. |
[35] | Micera A , Lambiase A , Stampachiacchiere B , Bonini S , Bonini S , Levi-Schaffer F ((2007) ) Nerve growth factor and tissue repair remodeling: trkA(NGFR) and p75(NTR), two receptors one fate. Cytokine Growth Factor Rev 18: , 245–256. |
[36] | Harrison BJ , Venkat G , Lamb JL , Hutson TH , Drury C , Rau KK , Bunge MB , Mendell LM , Gage FH , Johnson RD , Hill CE , Rouchka EC , Moon LD , Petruska JC ((2016) ) The adaptor protein CD2AP is a coordinator of neurotrophin signaling-mediated arbor plasticity. J Neurosci 36: , 4259–4275. |
[37] | Naj AC , Jun G , Beecham GW , Wang LS , Vardarajan BN , Buros J , Larson EB ((2011) ) Common variants at MS4A4/MS4A6E, CD2AP, CD33 and EPHA1 are associated with late-onset Alzheimer’s disease. Nat Genet 43: , 436–441. |
[38] | Olney NT , Spina S , Miller BL ((2017) ) Frontotemporal dementia. Neurol Clin 35: , 339–374. |
[39] | Bang J , Spina S , Miller BL ((2015) ) Frontotemporal dementia. Lancet 386: , 1672–1682. |
[40] | Ratnavalli E , Brayne C , Dawson K , Hodges JR ((2002) ) The prevalence of frontotemporal dementia. Neurology 58: , 1615–1621. |
[41] | Wittenberg D , Possin KL , Rascovsky K , Rankin KP , Miller BL , Kramer JH ((2008) ) The early neuropsychological and behavioral characteristics of frontotemporal dementia. Neuropsychol Rev 18: , 91–102. |
[42] | Neumann M , Kwong LK , Sampathu DM , Trojanowski JQ , Lee VM ((2007) ) TDP-43 proteinopathy in frontotemporal lobar degeneration and amyotrophic lateral sclerosis: Protein misfolding diseases without amyloidosis. Arch Neurol 64: , 1388–1394. |
[43] | van Swieten John C ((2007) ) Genetic basis of frontotemporal dementia. Lancet Neurol 6: , 840–841. |
[44] | Blauwendraat C , Wilke C , Simón-Sánchez J , Jansen IE , Reifschneider A , Capell A , Haass C , Castillo-Lizardo M , Biskup S , Maetzler W , Rizzu P , Heutink P , Synofzik M ((2017) ) The wide genetic landscape of clinical frontotemporal dementia: Systematic combined sequencing of 121 consecutive subjects. Genet Med 88: , 728–735. |
[45] | Grossman M ((2010) ) Primary progressive aphasia: Clinicopathological correlations. Nat Rev Neurol 6: , 88–97. |
[46] | Gorno-Tempini ML , Hillis AE , Weintraub S , Kertesz A , Mendez M , Cappa SF , Ogar JM , Rohrer JD , Black S , Boeve BF , Manes F , Dronkers NF , Vandenberghe R , Rascovsky K , Patterson K , Miller BL , Knopman DS , Hodges JR , Mesulam MM , Grossman M ((2011) ) Classification of primary progressive aphasia and its variants. Neurology 76: , 1006–1014. |
[47] | Whitwell JL , Duffy JR , Strand EA , Machulda MM , Senjem ML , Schwarz CG , Reid R , Baker MC , Perkerson RB , Lowe VJ , Rademakers R , Jack CR Jr , Josephs KA ((2015) ) Clinical and neuroimaging biomarkers of amyloid-negative logopenic primary progressive aphasia. Brain Lang 142: , 45. |
[48] | Armstrong MJ ((2014) ) Diagnosis and treatment of corticobasal degeneration. Curr Treat Options Neurol 16: , 282. |
[49] | Chahine LM , Rebeiz T , Rebeiz JJ , Grossman M , Gross RG ((2014) ) Corticobasal syndrome: Five new things. Neurol Clin Pract 4: , 304–312. |
[50] | Rebeiz JJ , Kolodny EH , Richardson EP Jr ((1967) ) Corticodentatonigral degeneration with neuronal achromasia: A progressive disorder of late adult life. Trans Am Neurol Assoc 92: , 23–26. |
[51] | Gibb WR , Luthert PJ , Marsden CD ((1989) ) Corticobasal degeneration. Brain 112: , 1171–1192. |
[52] | Boeve BF , Maraganore DM , Parisi JE , Aklskog JE , Graff-Radford N , Caselli RJ , Dickson DW , Kokmen E , Petersen RC ((1999) ) Pathologic heterogeneity in clinically diagnosed corticobasal degeneration. Neurology 53: , 795–800. |
[53] | Litvan I , Agid Y , Goetz C , Jankovic J , Wenning GK , Brandel JP , Lai EC , Verny M , Ray-Chaudhuri K , McKee A , Jellinger K , Pearce RK , Bartko JJ ((1997) ) Accuracy of the clinical diagnosis of corticobasal degeneration: A clinicopathologic study. Neurology 48: , 119–125. |
[54] | Kertesez A , Davidson W , Munoz DG ((1999) ) Clinical and pathological overlap between frontotemporal dementia, primary progressive aphasia and corticobasal degeneration: The Pick complex. Dement Geriatr Cogn Disord 10: (Suppl 1), 46–49. |
[55] | Kertesz A , Martinez-Lage P , Davidson W , Munoz DG ((2000) ) The corticobasal degeneration syndrome overlaps progressive aphasia and frontotemporal dementia. Neurology 55: , 1368–1375. |
[56] | Armstrong MJ , Litvan I , Lang AE , Bak TH , Bhatia KP , Borroni B , Boxer AL , Dickson DW , Grossman M , Hallett M , Josephs KA , Kertesz A , Lee SE , Miller BL , Reich SG , Riley DE , Tolosa E , Tröster AI , Vidailhet M , Weiner WJ ((2013) ) Criteria for the diagnosis of corticobasal degeneration. Neurology 80: , 496–503. |
[57] | Thorne RG , Pronk GJ , Padmanabhan V , Frey WHI ((2004) ) Delivery of insuline-like growth factor-I to the rat brain and spinal cord along olfactory and trigeminal pathways following intranasal administration. Neuroscience 127: , 481–496. |
[58] | Aloe L , Bianchi P , de Bellis A , Soligo M , Rocco ML ((2014) ) Intranasal nerve growth factor bypasses the blood-brain barrier and affects spinal cord neurons in spinal cord injury. Neural Regen Res 9: , 1025–1030. |
[59] | Bocchini V , Angeletti PU ((1969) ) The nerve growth factor: Purification as a 30,000-molecular-weight protein. Proc Natl Acad Sci U S A 64: , 787–794. |
[60] | Lambiase A , Rama P , Bonini S , Caprioglio G , Aloe L ((1998) ) Topical treatment with nerve growth factor for corneal neurotrophic ulcers. N Eng J Med 338: , 1174–1180. |
[61] | De Chiara G , Marcocci ME , Sgarbanti R , Civitelli L , Ripoli C , Piacentini R , Garaci E , Grassi C , Palamara AT ((2012) ) Infectious agents and neurodegeneration. Mol Neurobiol 46: , 614–638. |
[62] | Itzhaki RF ((2014) ) Herpes simplex virus type 1 and Alzheimer’s disease: Increasing evidence for a major role of the virus. Front Aging Neurosci 6: , 202. |
[63] | Itzhaki RF , Lathe R , Balin BJ , Ball MJ , Bearer EL , Braak H , Bullido MJ , Carter C , Clerici M , Cosby SL , Del Tredici K , Field H , Fulop T , Grassi C , Griffin WS , Haas J , Hudson AP , Kamer AR , Kell DB , Licastro F , Letenneur L , Lövheim H , Mancuso R , Miklossy J , Otth C , Palamara AT , Perry G , Preston C , Pretorius E , Strandberg T , Tabet N , Taylor-Robinson SD , Whittum-Hudson JA ((2016) ) Microbes and Alzheimer’s disease. J Alzheimers Dis 51: , 979–984. |
[64] | Aloe L , Bianchi P , Rocco ML , Micera A , Lambiase A , Bonini S ((2013) ) Recombinant human nerve growth factor protects photoreceptor degeneration in a rat model of inherited retinitis pigmentosa. Invest Ophthalmol Vis Sci 54: , 4021. |
[65] | Mantelli F ((2015) ) Evaluation of dfficacy of 20 μg/ml rhNGF new formulation (with anti-oxidant) in patients with stage 2 and 3 neurotrophic keratitis. ClinicalTrials.gov identifier: NCT02227147. |