Mini-review: The market growth of diagnostic and therapeutic monoclonal antibodies – SARS CoV-2 as an example
Abstract
BACKGROUND:
The emergence of novel viruses poses severe challenges to global public health highlighting the crucial necessity for new antivirals.
MAIN BODY:
Monoclonal antibodies (mAbs) are immunoglobulins that bind to a single epitope. Mouse mAbs are generated by classic hybridoma technology and are mainly used for immunodiagnostics. For immunotherapy, it is critical to use monoclonal antibodies in their human form to minimize adverse reactions. They have been successfully used to treat numerous illnesses, accordingly, an increasing number of mAbs, with high potency against emerging viruses is the target of every biopharmaceutical company. The diagnostic and therapeutic mAbs market grows rapidly into a multi-billion-dollar business. Biopharmaceuticals are innovative resolutions which revolutionized the treatment of significant chronic diseases and malignancies. Currently, a variety of therapeutic options that include antiviral medications, monoclonal antibodies, and immunomodulatory agents are available for the management of COVID-19.
SHORT CONCLUSION:
The invasion of mAbs in new medical sectors will increase the market magnitude as it is expected to generate revenue of about 300 billion $ by 2025. In the current mini-review, the applications of monoclonal antibodies in immune-diagnosis and immunotherapy will be demonstrated, particularly for COVID-19 infection and will focus mainly on monoclonal antibodies in the market.
1.Background
1.1Definition of monoclonal antibodies (mAbs) versus polyclonal antibodies
Monoclonal antibodies (mAbs) are immunoglobulins with mono specificity for an epitope. They are exactly driven from cloning of human plasma cell producing antibody. While polyclonal antibodies are antibody preparations from immunized animals with complex mixtures of various antibodies produced by many B cell clones. The first mAbs were made by merging the spleen cells of the immunized animal with malignant self-multiplying cells called myeloma cells to give rise to hybrid cells continuously producing the desired antibody [1]. These early mAbs product were murine antibodies and were of great value for research and diagnostic tests.
Polyclonal antibodies are heterogeneous mixture of antibodies produced by different B cell clones. They can recognize and bind to many different epitopes of a single antigen. Chicken immunoglobulin yolk (IgY), a polyclonal antibody yet will be an additional option for production of therapeutic antibodies as it is fast-acting, easy to generate at low cost [2]. IgY antibodies can readily be generated in large amounts with minimal environmental contamination or infrastructure asset by using egg-laying chickens. In addition, IgY does not cause allergic reaction for human use because it doesn’t cause complement stimulation, so IgY can be used for detection, prevention and treatment of human and animal infection. The in vitro applications of egg yolk-derived IgYs to the field of disease diagnosis in human and veterinary medicine through in vitro immunodetection of target biomolecules is highly promising [3]. Moreover, it is reported that IgY is used as a therapeutic agent for neutralization of viral antigens [4].
1.2Monoclonal antibodies (mAbs) production techniques
1.2.1Mouse monoclonal antibodies
Milstein and Köhler first described the method for stable development of monoclonal antibody production technique in 1975 [5]. The method comprises the creation of hybrid cells called hybridoma that produces a single antibody type against a definite antigen epitope. It was first formed from mouse models and the method consists of separating a pool of activated B cells from an immunized mouse’s spleen and mixing them with immortal myeloma cells to form the hybridomas. Propagation of hybrid clones in culture (Invitro) and in mice (Invivo) techniques were used for mAbs production. For diagnostic purposes, the production of mouse monoclonal antibodies in ascetic fluid in the abdominal cavity of the mice is cost saving method than propagation of hybrid cells in classic cell culture production methods. lately, the use of cell factory system or use of bioreactors or production of recombinant mouse monoclonal antibodies in expression system (s) have been implemented to level the production scale from lab to industry [6]. Major molecular engineering progress has been achieved enabling the usage of monoclonal antibodies as targeted therapies in many neoplastic cases, autoimmune, post-transplant immunosuppression, and infectious diseases [7].
1.2.2Production of human monoclonal antibodies
After few infusions, mouse antibody molecules elicited the human anti-mouse antibody (HAMA) response of the human immune system, non-self-identi- fication by adaptive immune response [8]. To solve this problem, novel techniques were adopted to make mAbs close to human fragments, so the chimeric technology evolved by phage display technique to make less immunogenic antibodies where the variable mouse regions are linked to the human constant region developing novel therapeutic options [9] Afterwards, the necessity for a lower immunogenic substitute enhanced producing humanized antibodies where only the part that interacts with the antigen epitope comes from mice by transgenic mice technique [10]. Even fully human antibodies by single cell technique were made [11]. Thus, there are 4 classes of mAbs: Mouse (-omab): entirely derived from mice origin. They may cause hypersensitive reactions in humans. Chimeric (-ximab): the constant regions are human and the variable regions are of murine origins. They may also cause allergy. Humanized (-zumab): mostly derived from a human origin except for the part that binds to its target. Human (-umab): entirely driven from a human origin. There are a lot of modified recombinant versions of mAbs that may have completely artificial Fc fragments, may be produced as mini/nano-bodies, scFvs, Fab fragments [12]. Recently, another alternative received consideration by using the camelid Variable region of heavy chain only antibodies (VHH) fragments (nanobodies) that can recognize epitopes that are mostly not accessible by conventional antibodies. Accordingly, Xu et al., (2021) isolated anti-RBD nanobodies from alpacas, dromedaries and camels, some of which targeted highly conserved epitopes in COVID-19, hardly recognized by human antibodies, with efficient neutralizing activities especially when expressed as a homotrimer [13].
Figure 1.
The general sequence for production and evaluation of immuno-diagnostic monoclonal antibodies.
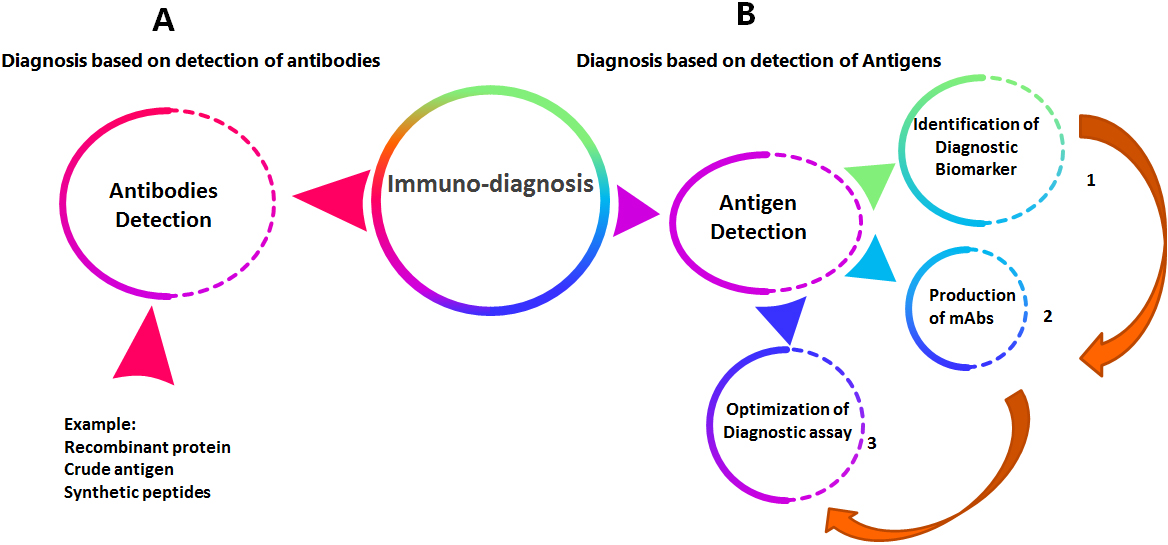
The major disadvantage of diagnostic and therapeutic monoclonal antibodies is the cost. Since more than 30 years ago, it was only imaginable to generate sufficient grams of therapeutic monoclonal antibodies annually but currently, pharmaceuticals can produce more than 10 tons. Scientists and technologists are always planning to improve procedures to help deliver medicines to individuals who want them rapidly, in addition of keeping the maximum standards of excellence and safety. So, there are several trails for improvement of methods of production of diagnostic or therapeutic antibodies. Invivo and invitro methods are generally used but invivo technology is cost-effective compared to invitro [14]. However, as the required quantity of mAb rises, the invitro production technology can be more economic due to spreading the high costs over large amounts making the cost per gm comparable to the invivo production technology, also animal use is reduced and the presence of contaminating foreign antigens is decreased. If mAb production time is critical and small quantities are required, invivo technique is chosen as it only needs few weeks [15, 16]. The benefits adopting invitro methods for production of mAbs, comprising time efficiency, economic viability, and large-scale production, are expected to fuel the segment growth over the forecast period. For therapeutic purposes, the production of human monoclonal antibodies, using chimeric or humanized antibodies in low-cost expression system is one of the best ways [17]. For commercial use, high-density cell culture for mAb production is adopted using the hollow fiber bioreactors or the new disposable bioreactors for economic biopharmaceutical production [18].
2.Main text
2.1Applications of mouse monoclonal antibodies for immunodiagnostics
The general sequence for production and evaluation of diagnostic monoclonal antibodies passes through three major steps starting with the selection of diagnostic biomarker followed by production of mouse monoclonal antibodies and then studying the sensitivity and specificity of the developed diagnostic assay as shown in Fig. 1.
Monoclonal antibodies possess wide range of applications, taking the advantage of the specific binding to the target antigen. The applications are essentially unlimited; in forensics, in biological examining of foods and in examining the presence of pathogenic organisms or toxins in blood especially that the test can be made quantitative for the tested antigen. Assays relying on antibody testing are greatly employed in medical field to assess the level of hormones, growth factors, blood cells, or tumors. MAbs can trace antigen’s place that is why they are broadly used in immunohistochemistry to localize antigens in tissue sections by conjunction with color forming labels and microscopy [19]. Identifying specific types of cells using antibody recognition technique is used to purify these cells from crude mixtures as blood clotting factor 8 from large volumes of blood in milligram quantities as a valuable therapeutic material. Preparative immunoaffinity methods are very powerful in comparison to the traditional chemical purification techniques, though generally combing both techniques yield better purification process [20]. Another method of purification is to attach the antibody to magnetic beads, which can be used physically to isolate cells with required antigen only. A more influential technique utilizes the fluorescence activated cell sorter, a cell may be recognized based on the antibody tagged with fluorescent dye and substantially sorted from others [21].
Monoclonal antibodies enabled comprehensive examination of functional molecules on the surface of the cell. Cells express on their surface the molecules implicated in their function. Whereas some cell surface molecules are expressed by entire cells, while others are expressed in a controlled way that permits them to be taken as ‘markers’ of particular cell types. Accordingly, entirely leucocytes only express CD45 and all T cells only express CD3. Monoclonal antibodies are widely used for analysis of cell functions, tissue pathology, hematology, immunopathology and blood transfusion [22]. It is worth mentioning that mouse mAb were previously used invitro to study viral neutralization. Murine monoclonal antibodies against conserved HCV E1 specific epitope induced viral inhibition in Huh7 cells, making this specific epitope a possible candidate for developing HCV therapeutic antibodies [23].
2.1.1Monoclonal antibodies for detection of severe acute respiratory syndrome corona virus 2 (SARS-CoV-2) antigens as a diagnostic tool for COVID-19 infection
Detecting SARS-CoV-2 RNA from nasopharyngeal samples using real-time reverse transcription-polymerase chain reaction (RT-PCR) is the gold standard technique for viral diagnosis. SARS-CoV-2 antigen detection tests using specific mouse monoclonal antibodies targeting nucleocapsid are fast, less laborious, and cheaper alternatives.
Lately, Favresse et al., (2021) compared the clinical performance of five antigen detection (AD) tests, including four rapid AD (RAD) tests (biotical, Panbio, Healgen, and Roche) and one automated AD test (VITROS). The RAD tests showed lower sensitivity allowing the identification of RT-PCR positive patients with higher viral loads while, the VITROS assay showed 100% sensitivity and had a specificity of 100% concluding that, the VITROS assay allows faster, easier and cheaper SARS-CoV-2 [24].
A new assay based on mAbs against four spike S1 regions of COVID-19 by Barlev-Gross et al., (2021) who evaluated its performance for virus detection from nasopharyngeal swabs, versus an in-house assay based on nucleocapsid protein, suggested that using antigens combination might increase the results validity. Such assays are fast and less expensive prescreening tools for antigen detection but sensitivity vs. specificity should be considered [25].
SARS-CoV-2 mutants can affect the performance of current laboratory diagnostics. An in-house antigen detection assay combining four S1mAbs was carried out on pool of samples extracted from nasopharyngeal swabs that tested negative via qRTPCR. Sensitive viral detection of both the wild type and the variants took place, in spite of the various mutations in the variants’ S1 region, the results proposed that this mAb mixture, against specific regions, allows both specificity and the universality [26].
2.2Applications of human monoclonal antibodies in Immunotherapy
The general sequence for production and evaluation of therapeutic monoclonal antibodies requires defining the therapeutic target, production of human monoclonal antibodies, studying the efficacy of the generated monoclonal antibodies by in vitro experiments and preclinical studies then clinical studies and finally distribution to the market (Fig. 2). Worldwide, at least five hundred and seventy, monoclonal antibodies for therapy have been investigated in clinical trials by Medical corporations [27], and seventy nine monoclonal antibodies for therapy gained approval by the US FDA (United States Food and Drug Administration) and are today in the market, involving thirty Monoclonal antibodies for cancer treatment [28]. MAb for therapy could be divided in two major groups, one includes the usage of naked antibodies to treat cancer and induce apoptosis using various modes such as antibody dependent cell mediated cytotoxicity (ADCC), pursuing the tumor microenvironment, or immune checkpoints. While the other therapeutic mAb group, involves added Ab engineering as conjugating an immunocytokine, radionuclide, immunoliposome or bispecific Ab to improve the therapeutic activity [28, 29, 30, 31, 32, 33].
Figure 2.
The general sequence for production and evaluation of therapeutic monoclonal antibodies.
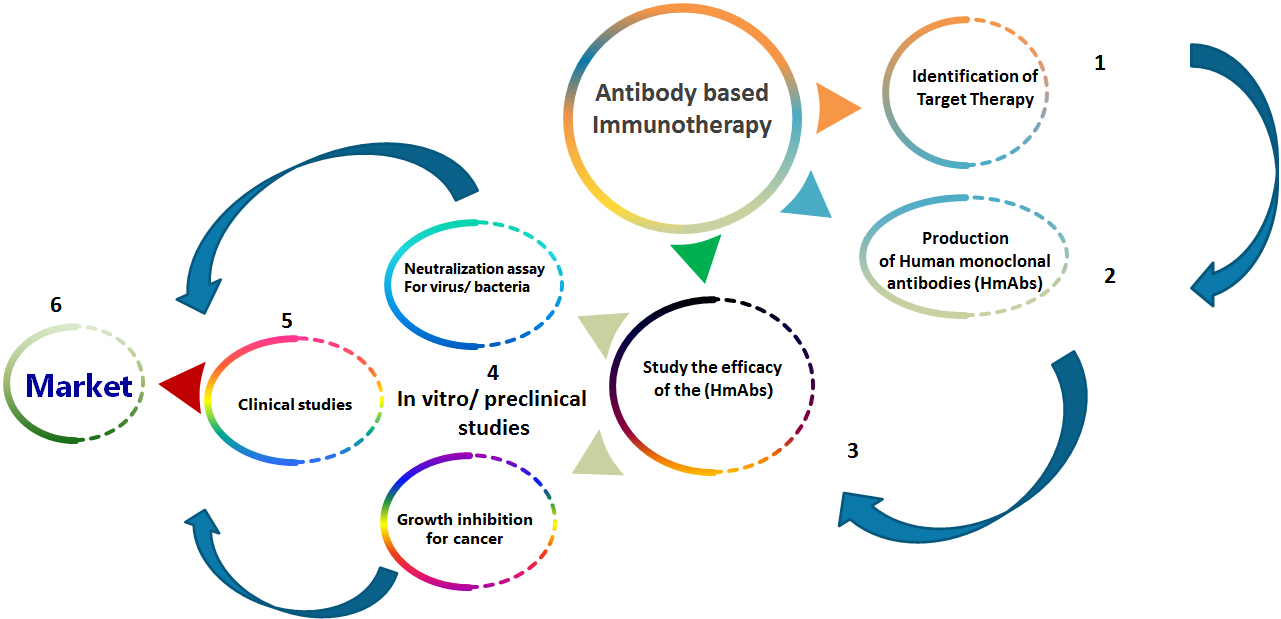
2.2.1Monoclonal antibodies and immunotherapy of COVID-19
The recently worldwide emergent pandemic COVID-19 has mortality rate up to 7% till now. The virus is transmitted via direct contact or droplets causing lung inflammation and acute respiratory distress syndrome (ARDS). COVID-19 infection is escorted with severe inflammatory response caused by the release of excessive amounts of cytokines such as TNFa, interleukins IL6 and IL1 leading to what is known by cytokine storm (CS). Thus, a vigorous host immune reaction recruiting macrophages, lymphocytes, neutrophils and T cells against virus infected cells led to this super inflammatory state causing several organs failures and lung injury [34]. Therapeutic tactics for managing the COVID-19 CS could give a hope for decreasing the virus associated mortality and morbidity [35]. The early usage of antivirals and antibody mediated therapy during early COVID-19 infection showed great improvement, also it is recommended to use immunomodulators together with antivirals and mAbs in late stages to overcome the cytokine-mediated hyperinflammatory state causing severe attacks [36]. MAbs against COVID-19 possess the potential for both prophylaxis and infection therapy. Positive results have been shown in experimental animals for both SARS-CoV-2 and Middle East respiratory syndrome coronavirus (MERS-CoV) monoclonal antibodies [37]. Recovered patients generated humoral and cellular immune responses against the virus. Knowing the absences of efficient therapy for COVID-19 infected cases, convalescent plasma was collected and the titer of neutralizing SARS-CoV-2 antibodies was measured. To date in the biggest study, two units of the retrieved plasma were given to five thousand patients having life-threatening SARS-CoV-2 causing more than 50% reduction in mortality rates supporting the effectiveness of human convalescent plasma as a therapeutic agent in hospitalized COVID-19 cases [38, 39]. Other studies have concluded that there isn’t positive effect of convalescent plasma in COVID-19 patients with severe disease [40, 41]. The divergent outcomes could be attributed to many factors, as the class and the quality of the neutralizing Anti SARS-CoV-2 antibodies, the time of therapy, the amount of anti-IFN antibodies and the volume of plasma used, which may cause conflicting therapeutic results. Contrarily, increasing evidence supports using plasma therapy in immunocompromised individuals, especially those receiving B cells depleting drugs such as Rituximab [42, 43]. Thus, it is hard to standardize convalescent plasma therapy and its role may be limited to early epidemic phase with limited therapeutic options or for certain patient groups. It must be recognized that many therapeutic monoclonal antibodies have been developed to target molecules involved in the regulation of the immune response or proinflammatory cytokines contributing to disease progression [44].
It is also noteworthy to consider the progress for production of monoclonal egg yolk IgYs and IgY-like immunoglobulin using the phage display technology or in transgenic chickens. In the current COVID-19 pandemic, passive vaccination could be applied for quick defense against modified agents. Several papers showed the efficacy of IgY in preventing viral binding and replication of SARS-CoV-2 [45, 46]. More studies about production and characterization of IgY and showing its ability to inhibit Middle East Respiratory Syndrome Coronavirus Infection in animal model was carried out [47, 48].
Tocilizumab is a humanized bispecific monoclonal antibody, designed to bind both membrane bound and soluble receptors for IL-6 and blocks JAK-STAT signaling pathway to stop the vigorous host immune reactions as in rheumatoid arthritis and respiratory syndromes as COVID 19 [49, 50]. Eculizumab is a monoclonal anticomplement antibody; the anti-C5 antibody is an alternative for the treatment of critical COVID-19 patients by reducing the severe clinical manifestations such as respiratory distress and acute kidney failure [51].
2.2.2COVID-19 Update: FDA authorizes monoclonal antibodies for treatment of COVID-19
Monoclonal antibodies mimic the ability of the immune system to fight against harmful pathogens. In 2019, the US-Food and Drug Administration FDA approved six mAbs that are realized to the market, namely: caplacizumab (from Ablynx/Sanofi) for thrombotic thrombocytopenic purpura, romosozumab (Amgen/UCB) for osteoporosis in females during the postmenopause period with higher fracture risks, risankizumab (Boehringer Ingelheim/AbbVie) used for treating plaque psoriasis, polatuzumab (Roche, F. Hoffmann-La Roche, Ltd.) for diffusing large B cell lymphoma, and brolucizumab and crizanlizumab, both from Novartis, for macular degeneration and sickle cell disease, respectively [28]. Later the U.S. FDA issued an emergency use authorization (EUA) for casirivimab and imdevimab, two mAbs that specifically target the SARS-CoV-2 spike protein, in order to halt the viral binding and entrance used with equal amounts to decrease the risk for development of escape mutants (Regeneron Pharmaceuticals Inc, https://www.regeneron.com/casirivimab-imdevimab) to be taken together for the treatment of mild to moderate COVID-19 in grown-ups and children patients (
2.3Monoclonal antibodies in the market
More than Five thousand companies use different technologies for cancer immunotherapy adopting 3 major classes which are cytokines and mAbs and cell therapy. Innovators for immunotherapy used interleukins and interferons to stimulate immune reaction to cancer cells. Using cytokines led to nonspecific reactions that commonly cause severe adverse reactions. Later on, novel, advanced stimulation and modulation immunity pathways have been discovered, as a trial for decreasing such adverse reactions and rise efficiency [55]. Immunotherapy using mAbs marketed drugs persevered the stronghold in that field. Monoclonal antibodies act either by carrying chemotherapeutic agents to specific sites to induce immune stimulation to cancer cells, or by inhibiting important biotic pathways in cancerous cells. A lot of pharmaceutical corporations are safeguarding a tactical place in this area [56]. A big attention was given by medicinal companies to cell therapy where, genetic engineering reprogramming of the patient’s isolated immune cells is carried out allowing them to identify and shoot down cancer cells when reintroduced again to the patient [57]. Immunodiagnostic and immunotherapeutic mAbs agents owned marvelous market elevation in the past five years. The market progress for immunotherapy first started at 1980, the initial FDA approval was earned by 1986 for the inhibition of rejection of kidney transplantation. The monoclonal antibody market trends reformed quickly the next period becoming the main therapeutic tools for various illnesses. Reports about returns of global sales of these products in 2018 were
The international monoclonal antibody-based therapy market is anticipated to rise to
Due to the major advancements in biological medicines, monoclonal antibodies possessed a major sector in immunotherapy for dealing with various illnesses, mainly tumors, immunologic, transmittable, metabolic and neuronal diseases. The growth in the sales and the governing agreement for monoclonal antibody drugs were sluggish till the end of 1990 when first chimeric mAbs were approved making a $0.3 billion annual sales by 1997. Following the successive approval of humanized then later the full human mAbs, the approval rates and sales for monoclonal antibody drugs have augmented fast, making $114 billion revenue from global sales by 2019 as shown in Fig. 3 [28]. The continuous raise in the production of mAbs drugs in the near-term years is anticipated making mAbs the main sales driver for the global biopharmaceutical products.
Figure 3.
The growth of monoclonal antibodies market. The mAb market is enjoying a healthy pipeline and is expected to grow at a faster pace, reaching a valuation of US $300 billion by 2025.
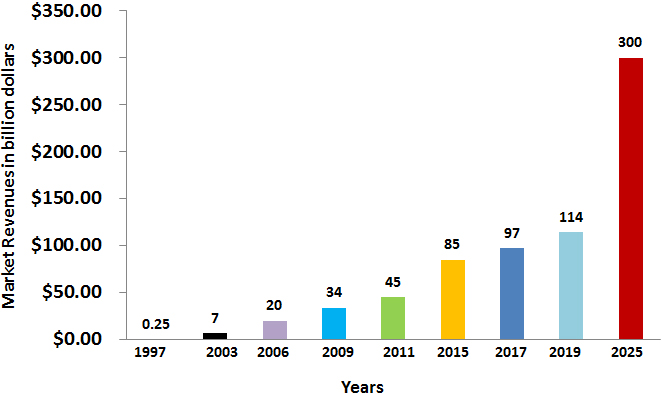
Evolving economies are anticipated to progressively contribute to the market of monoclonal antibodies. Different corporations are not likely to occupy the market that is presently controlled by 7 companies [60]. An economic study revealed that twenty-two firms performed well in the market of mAbs, to reach $98 billion sales and 18.3% growth in December 2017. In 2013, the growth in market of mAbs was ranging from 7.2% and 18.3% annually. Presently, it is expected that the quicker product approval rates will positively contribute to the market growth raise up. On the other hand, bio-similar and further drug formulae may negatively affect mAbs market growth. Taking in account this ambiguity and studying current market situation, forecasts for upcoming market trends were made presuming annual growth rates of 6%, 10% or 15% resulting in a forecasted value of $137–200 billion by 2022 reaching to $300 billion by 2025 as shown in Fig. 3 [28].
Only seven prevalent companies out of the twenty-two working mAbs firms have high market performance acquiring market shares of 87%, controlled by Genentech previously Roche company with sales 31% and 11 approved products [60]. Most monoclonal antibody companies are located either in the United States of America or in the European Union regions, keeping only a 7% of the other manufacturing identities in Asia [60]. Seven out of the ten chart-topping new drugs in 2019 were monoclonal antibodies for autoimmune diseases and cancer [61]. Monoclonal antibodies are amongst the maximum priced pharmaceutical products in the world. The utmost costly mAbs are those for oncology and hematology, with yearly therapy costs
Previous market analysis during 2012 till 2017 revealed two-fold market size increase. This increasing trend in mAbs sales is predicted to last till 2022 reaching to $130–200 billion motivated by a healthy pipeline and collective positions of bio-similar and developing economies. Bio-similars are biopharmaceuticals made and vended on the market after the expiry of the patents for these innovative biological products, the originating company loses production exclusivity and the technology comes into the public domain. The market is presently subjugated by 7 industrial identities holding 87% of the mAbs market share, a situation that is expected to continue for a while [63]. Monoclonal antibodies-based treatments are progressively given in sequence or in combination, resulting in elevated therapy expenses, and the major limitation in mAb production. Intermarket race with Bio-similar is anticipated to increase forcing the big bio therapeutic companies to counter act this effect via trying to detect novel disease targets for the present monoclonal antibodies besides trying to augment the continuous bioprocessing and develop new know-hows that ease quicker drug discovery and rapider time to clinic procedures. Monoclonal antibodies started to enter all medical fields besides the well-studied ones among which are infectious, autoimmune, metabolic, and neural and cancer diseases. The new mAbs targets under advancement include diabetes, Alzheimer’s disease, and obesity. The invasion of mAbs in new medical sectors will with no doubt increase the market magnitude and enlarge it the coming years [64].
2.4Conclusion
Finally, more hybridoma centers are required worldwide as in big universities whether for research or clinical services, as providing affordable and sustainable monoclonal antibody-based products is a global health priority. Establishing such Centres will make it possible to work efficiently in vaccines development using the therapeutic monoclonal antibodies for saving and improving millions of lives plus exploiting the involvement to the technology and development sectors, augmenting technology management and lessening the gap between research centres, universities and industrial medical companies, collaborating for probable partnerships in novel technologies.
Ethics approval and consent to participate
Not applicable.
Consent for publication
Not applicable.
Availability of data and materials
All data analyzed during this study are included in this published article in reference section and websites listed in the manuscript which are https://www.regener on.com/casirivimab-imdevimabhttps://www.fda.gov/news-events/press-announcements/coronavirus-covid-19-update-fda-authorizes-monoclonal-antibodies-treatment-covid-19. https://www.antibodysociety.org/covid-19.
Funding
Not applicable.
Author’s contributions
YE performed overall writing of the all-review sections and made the market research.
AT conceived the idea, drew the figures and formulated the conclusion.
RS performed analysis of data research work and edited the main body of the review.
MS updated the market research and edited the review paper.
All authors read and approved the final manuscript.
Acknowledgments
This work was supported through National Research Centre, Internal project ID 12010130 to Dr. Yasmine El Abd.
Conflict of interest
The authors declare that they have no competing interests.
References
[1] | G. Köhler and C. Milstein, Continuous cultures of fused cells secreting antibody of predefined specificity, Nature 256: ((1975) ), 495–497. |
[2] | L. Lee, K. Samardzic, M. Wallach, L.R. Frumkin and D. Mochly-Rosen, Immunoglobulin Y for potential diagnostic and therapeutic applications in infectious diseases, Front Immunol 12: ((2021) ), 696003. |
[3] | C.E. Karachaliou, V. Vassilakopoulou and E. Livaniou, IgY technology: Methods for developing and evaluating avian immunoglobulins for the in vitro detection of biomolecules, World J Methodol 11: ((2021) ), 243–262. |
[4] | M.T.L. da Silva, R.M. Deodato and L.M. Villar, Exploring the potential usefulness of IgY for antiviral therapy: A current review, Int J Biol Macromol 189: ((2021) ), 785–791. |
[5] | G. Köhler and C. Milstein, Continuous cultures of fused cells secreting antibody of predefined specificity. 1975, J Immunol 174: ((2005) ), 2453–2455. |
[6] | B. Kelley, Industrialization of mAb production technology: The bioprocessing industry at a crossroads, MAbs 1: ((2009) ), 443–452. |
[7] | T.T. Hansel, H. Kropshofer, T. Singer, J.A. Mitchell and A.J. George, The safety and side effects of monoclonal antibodies, Nat Rev Drug Discov 9: ((2010) ), 325–338. |
[8] | J.K. Liu, The history of monoclonal antibody development – progress, remaining challenges and future innovations, Ann Med Surg (Lond) 3: ((2014) ), 113–116. |
[9] | G. Winter, A.D. Griffiths, R.E. Hawkins and H.R. Hoogenboom, Making antibodies by phage display technology, Annu Rev Immunol 12: ((1994) ), 433–455. |
[10] | N. Tsurushita, P.R. Hinton and S. Kumar, Design of humanized antibodies: From anti-Tac to Zenapax, Methods 36: ((2005) ), 69–83. |
[11] | P. Chames, M. Van Regenmortel, E. Weiss and D. Baty, Therapeutic antibodies: Successes, limitations and hopes for the future, Br J Pharmacol 157: ((2009) ), 220–233. |
[12] | P. Bannas, J. Hambach and F. Koch-Nolte, Nanobodies and nanobody-based human heavy chain antibodies as antitumor therapeutics, Front Immunol 8: ((2017) ). |
[13] | J. Xu, K. Xu, S. Jung, A. Conte, J. Lieberman, F. Muecksch, J.C.C. Lorenzi, S. Park, F. Schmidt, Z. Wang, Y. Huang, Y. Luo, M.S. Nair, P. Wang, J.E. Schulz, L. Tessarollo, T. Bylund, G.Y. Chuang, A.S. Olia, T. Stephens, I.T. Teng, Y. Tsybovsky, T. Zhou, V. Munster, D.D. Ho, T. Hatziioannou, P.D. Bieniasz, M.C. Nussenzweig, P.D. Kwong and R. Casellas, Nanobodies from camelid mice and llamas neutralize SARS-CoV-2 variants, Nature 595: ((2021) ), 278–282. |
[14] | D.C. Andersen and D.E. Reilly, Production technologies for monoclonal antibodies and their fragments, Curr Opin Biotechnol, 15: ((2004) ), 456–462. |
[15] | N.C. Peterson and J.E. Peavey, Comparison of in vitro monoclonal antibody production methods with an in vivo ascites production technique, Contemp Top Lab Anim Sci 37: ((1998) ), 61–66. |
[16] | J.R. Birch and A.J. Racher, Antibody production, Adv Drug Deliv Rev 58: ((2006) ), 671–685. |
[17] | W. Ansar and S. Ghosh, Monoclonal antibodies: A tool in clinical research, Indian Journal of Clinical Medicine 4: ((2013) ), IJCM.S11968. |
[18] | I. Jyothilekshmi and N.S. Jayaprakash, Trends in monoclonal antibody production using various bioreactor syst, J Microbiol Biotechnol 31: ((2021) ), 349–357. |
[19] | H. Zola and P. roberts-thomson, Monoclonal Antibodies: Diagnostic Uses, (2001) . |
[20] | K. Nogami, T. Soeda, T. Matsumoto, Y. Kawabe, T. Kitazawa and M. Shima, Routine measurements of factor VIII activity and inhibitor titer in the presence of emicizumab utilizing anti-idiotype monoclonal antibodies, J Thromb Haemost 16: ((2018) ), 1383–1390. |
[21] | H. Jang, E.Y. Hwang, Y. Kim, J. Choo, J. Jeong and D.W. Lim, Surface-enhanced raman scattering and fluorescence-based dual nanoprobes for multiplexed detection of bacterial pathogens, J Biomed Nanotechnol 12: ((2016) ), 1938–1951. |
[22] | K.R. Rodgers and R.C. Chou, Therapeutic monoclonal antibodies and derivatives: Historical perspectives and future directions, Biotechnol Adv 34: ((2016) ), 1149–1158. |
[23] | A.A. Tabll, R.I. Moustafa, Y.S. El Abd, N.G. Bader El Din, R. El-Shenawy, H. Yousef, M. Hussein, R.M. Dawood, M.H. Omran and M.K. El-Awady, Mouse monoclonal antibody towards e1 specific epitope blocks viral entry and intracellular viral replication in vitro, J Immunoassay Immunochem 35: ((2014) ), 60–73. |
[24] | J. Favresse, C. Gillot, M. Oliveira, J. Cadrobbi, M. Eslen, C. Eucher, K. Laffineur, C. Rosseels, S. Van Eeckhoudt, J.-B. Nicolas, L. Morimont, J.-M. Dogne and J. Douxfils, Head-to-Head Comparison of Rapid and Automated Antigen Detection Tests for the Diagnosis of SARS-CoV-2 Infection, journal of Clinical Medicine 10: ((2021) ), 265. |
[25] | M. Barlev-Gross, S. Weiss, A. Ben-Shmuel, A. Sittner, K. Eden, N. Mazuz, I. Glinert, E. Bar-David, R. Puni, S. Amit, O. Kriger, O. Schuster, R. Alcalay, E. Makdasi, E. Epstein, T. Noy-Porat, R. Rosenfeld, H. Achdout, O. Mazor, T. Israely, H. Levy and A. Mechaly, Spike vs nucleocapsid SARS-CoV-2 antigen detection: Application in nasopharyngeal swab specimens, Anal Bioanal Chem 413: ((2021) ), 3501–3510. |
[26] | M. Barlev-Gross, S. Weiss, N. Paran, Y. Yahalom-Ronen, O. Israeli, I. Nemet, L. Kliker, N. Zuckerman, I. Glinert, T. Noy-Porat, R. Alcalay, R. Rosenfeld, H. Levy, O. Mazor, M. Mandelboim, E. Mendelson, A. Beth-Din, T. Israely and A. Mechaly, Sensitive immunodetection of severe acute respiratory syndrome coronavirus 2 variants of concern 501Y.V2 and 501Y.V1, J Infect Dis 224: ((2021) ), 616–619. |
[27] | H. Kaplon, M. Muralidharan, Z. Schneider and J.M. Reichert, Antibodies to watch in 2020, MAbs 12: ((2019) ), 1703531. |
[28] | R.M. Lu, Y.C. Hwang, I.J. Liu, C.C. Lee, H.Z. Tsai, H.J. Li and H.C. Wu, Development of therapeutic antibodies for the treatment of diseases, J Biomed Sci 27: ((2020) ), 1. |
[29] | K.O. Saunders, Conceptual approaches to modulating antibody effector functions and circulation half-life, Front Immunol 10: ((2019) ), 1296. |
[30] | D. Neri, Antibody-cytokine fusions: Versatile products for the modulation of anticancer immunity, Cancer Immunol Res 7: ((2019) ), 348–354. |
[31] | A. Beck, L. Goetsch, C. Dumontet and N. Corvaïa, Strategies and challenges for the next generation of antibody-drug conjugates, Nat Rev Drug Discov 16: ((2017) ), 315–337. |
[32] | A.F. Labrijn, M.L. Janmaat, J.M. Reichert and P. Parren, Bispecific antibodies: A mechanistic review of the pipeline, Nat Rev Drug Discov 18: ((2019) ), 585–608. |
[33] | A. Ohradanova-Repic, E. Nogueira, I. Hartl, A.C. Gomes, A. Preto, E. Steinhuber, V. Mühlgrabner, M. Repic, M. Kuttke, A. Zwirzitz, M. Prouza, M. Suchanek, G. Wozniak-Knopp, V. Horejsi, G. Schabbauer, A. Cavaco-Paulo and H. Stockinger, Fab antibody fragment-functionalized liposomes for specific targeting of antigen-positive cells, Nanomedicine 14: ((2018) ), 123–130. |
[34] | D. Ragab, H. Salah Eldin, M. Taeimah, R. Khattab and R. Salem, The COVID-19 cytokine storm; what we know so far, Front Immunol 11: ((2020) ), 1446. |
[35] | L.A. Henderson, S.W. Canna, G.S. Schulert, S. Volpi, P.Y. Lee, K.F. Kernan, R. Caricchio, S. Mahmud, M.M. Hazen, O. Halyabar, K.J. Hoyt, J. Han, A.A. Grom, M. Gattorno, A. Ravelli, F. De Benedetti, E.M. Behrens, R.Q. Cron and P.A. Nigrovic, On the alert for cytokine storm: Immunopathology in COVID-19, Arthritis Rheumatol 72: ((2020) ), 1059–1063. |
[36] | R.T. Gandhi, J.B. Lynch and C. Del Rio, Mild or moderate COVID-19, N Engl J Med 383: ((2020) ), 1757–1766. |
[37] | Y. Kim, H. Lee, K. Park, S. Park, J.H. Lim, M.K. So, H.M. Woo, H. Ko, J.M. Lee, S.H. Lim, B.J. Ko, Y.S. Park, S.Y. Choi, D.H. Song, J.Y. Lee, S.S. Kim and D.Y. Kim, Selection and characterization of monoclonal antibodies targeting middle east respiratory syndrome coronavirus through a human synthetic fab phage display library panning, Antibodies (Basel) 8: ((2019) ). |
[38] | M.J. Joyner, S.A. Klassen, J. Senefeld, P.W. Johnson, R.E. Carter, C.C. Wiggins, S. Shoham, B.J. Grossman, J.P. Henderson, J.M. Musser, E. Salazar, W.R. Hartman, N.M. Bouvier, S.T.H. Liu, L.-a. Pirofski, S.E. Baker, N. Van Helmond, R.S. Wright, D. Fairweather, K.A. Bruno, N.S. Paneth and A. Casadevall, Evidence favouring the efficacy of convalescent plasma for COVID-19 therapy, medRxiv, (2020) , 2020.2007.2029.20162917. |
[39] | C. Del Fante, M. Franchini, F. Baldanti, E. Percivalle, C. Glingani, G. Marano, C. Mengoli, C. Mortellaro, G. Viarengo, C. Perotti and G.M. Liumbruno, A retrospective study assessing the characteristics of COVID-19 convalescent plasma donors and donations, Transfusion 61: ((2021) ), 830–838. |
[40] | P. Janiaud, C. Axfors, A.M. Schmitt, V. Gloy, F. Ebrahimi, M. Hepprich, E.R. Smith, N.A. Haber, N. Khanna, D. Moher, S.N. Goodman, J.P.A. Ioannidis and L.G. Hemkens, Association of convalescent plasma treatment with clinical outcomes in patients with COVID-19: A systematic review and meta-analysis, Jama 325: ((2021) ), 1185–1195. |
[41] | S.T.H. Liu and J.A. Aberg, Convalescent plasma in patients hospitalised with COVID-19, Lancet 397: ((2021) ), 2024–2025. |
[42] | S. Ferrari, C. Caprioli, A. Weber, A. Rambaldi and F. Lussana, Convalescent hyperimmune plasma for chemo-immunotherapy induced immunodeficiency in COVID-19 patients with hematological malignancies, Leuk Lymphoma 62: ((2021) ), 1490–1496. |
[43] | E. McKemey, A.M. Shields, S.E. Faustini, H.J. Hill, A. Baranskaya, Z. Stamataki, S. Gompertz, A.G. Richter, D. Dosanjh and S. Madathil, Correction to: Resolution of persistent COVID-19 after convalescent plasma in a patient with B cell aplasia, J Clin Immunol 41: ((2021) ), 930. |
[44] | L. Hammarström, H. Marcotte, A. Piralla, F. Baldanti and Q. Pan-Hammarström, Antibody therapy for COVID-19, Curr Opin Allergy Clin Immunol ((2021) ). |
[45] | C. Artman, K.D. Brumfield, S. Khanna and J. Goepp, Avian antibodies (IgY) targeting spike glycoprotein of severe acute respiratory syndrome coronavirus 2 (SARS-CoV-2) inhibit receptor binding and viral replication, PLoS One 16: ((2021) ), e0252399. |
[46] | S. Wei, S. Duan, X. Liu, H. Wang, S. Ding, Y. Chen, J. Xie, J. Tian, N. Yu, P. Ge, X. Zhang, X. Chen, Y. Li and Q. Meng, Chicken egg yolk antibodies (IgYs) block the binding of multiple SARS-CoV-2 spike protein variants to human ACE2, Int Immunopharmacol, 90: ((2021) ), 107172. |
[47] | A.T. Abbas, S.A. El-Kafrawy, S.S. Sohrab, A.A. Tabll, A.M. Hassan, N. Iwata-Yoshikawa, N. Nagata and E.I. Azhar, Anti-S1 MERS-COV IgY specific antibodies decreases lung inflammation and viral antigen positive cells in the human transgenic mouse model, Vaccines (Basel) 8: ((2020) ), 634. |
[48] | S.A. El-Kafrawy, A.T. Abbas, S.S. Sohrab, A.A. Tabll, A.M. Hassan, N. Iwata-Yoshikawa, N. Nagata and E.I. Azhar, Immunotherapeutic efficacy of IgY antibodies targeting the full-length spike protein in an animal model of middle east respiratory syndrome coronavirus infection, Pharmaceuticals (Basel) 14: ((2021) ), 511. |
[49] | A. Saha, A.R. Sharma, M. Bhattacharya, G. Sharma, S.S. Lee and C. Chakraborty, Tocilizumab: A therapeutic option for the treatment of cytokine storm syndrome in COVID-19, Arch Med Res 51: ((2020) ), 595–597. |
[50] | Y. Mo, O. Adarkwah, J. Zeibeq, E. Pinelis, J. Orsini and J. Gasperino, Treatment with tocilizumab for patients with COVID-19 infections: A case-series study, J Clin Pharmacol 61: ((2021) ), 406–411. |
[51] | J. Laurence, J.J. Mulvey, M. Seshadri, A. Racanelli, J. Harp, E.J. Schenck, D. Zappetti, E.M. Horn and C.M. Magro, Anti-complement C5 therapy with eculizumab in three cases of critical COVID-19, Clin Immunol 219: ((2020) ), 108555. |
[52] | A. Baum, D. Ajithdoss, R. Copin, A. Zhou, K. Lanza, N. Negron, M. Ni, Y. Wei, K. Mohammadi, B. Musser, G.S. Atwal, A. Oyejide, Y. Goez-Gazi, J. Dutton, E. Clemmons, H.M. Staples, C. Bartley, B. Klaffke, K. Alfson, M. Gazi, O. Gonzalez, E. Dick, Jr., R. Carrion, Jr., L. Pessaint, M. Porto, A. Cook, R. Brown, V. Ali, J. Greenhouse, T. Taylor, H. Andersen, M.G. Lewis, N. Stahl, A.J. Murphy, G.D. Yancopoulos and C.A. Kyratsous, REGN-COV2 antibodies prevent and treat SARS-CoV-2 infection in rhesus macaques and hamsters, Science 370: ((2020) ), 1110–1115. |
[53] | D.M. Weinreich, S. Sivapalasingam, T. Norton, S. Ali, H. Gao, R. Bhore, B.J. Musser, Y. Soo, D. Rofail, J. Im, C. Perry, C. Pan, R. Hosain, A. Mahmood, J.D. Davis, K.C. Turner, A.T. Hooper, J.D. Hamilton, A. Baum, C.A. Kyratsous, Y. Kim, A. Cook, W. Kampman, A. Kohli, Y. Sachdeva, X. Graber, B. Kowal, T. DiCioccio, N. Stahl, L. Lipsich, N. Braunstein, G. Herman and G.D. Yancopoulos, REGN-COV2, a neutralizing antibody cocktail, in outpatients with Covid-19, N Engl J Med 384: ((2021) ), 238–251. |
[54] | P.C. Taylor, A.C. Adams, M.M. Hufford, I. de la Torre, K. Winthrop and R.L. Gottlieb, Neutralizing monoclonal antibodies for treatment of COVID-19, Nat Rev Immunol 21: ((2021) ), 382–393. |
[55] | T. Wieder, E. Brenner, H. Braumüller, O. Bischof and M. Röcken, Cytokine-induced senescence for cancer surveillance, Cancer Metastasis Rev 36: ((2017) ), 357–365. |
[56] | T. Kieber-Emmons, The future of monoclonal antibodies in immunodiagnosis and immunotherapy, Monoclon Antib Immunodiagn Immunother 39: ((2020) ), 105–106. |
[57] | C.H. June, R.S. O’Connor, O.U. Kawalekar, S. Ghassemi and M.C. Milone, CAR T cell immunotherapy for human cancer, Science 359: ((2018) ), 1361–1365. |
[58] | R.-M. Lu, Y.-C. Hwang, I.J. Liu, C.-C. Lee, H.-Z. Tsai, H.-J. Li and H.-C. Wu, Development of therapeutic antibodies for the treatment of diseases, Journal of Biomedical Science 27: ((2020) ), 1. |
[59] | H.A. Parray, S. Shukla, S. Samal, T. Shrivastava, S. Ahmed, C. Sharma and R. Kumar, Hybridoma technology a versatile method for isolation of monoclonal antibodies, its applicability across species, limitations, advancement and future perspectives, Int Immunopharmacol 85: ((2020) ), 106639. |
[60] | A.L. Grilo and A. Mantalaris, The increasingly human and profitable monoclonal antibody market, Trends Biotechnol 37: ((2019) ), 9–16. |
[61] | L. Urquhart, Top companies and drugs by sales in 2019, Nat Rev Drug Discov 19: ((2020) ), 228. |
[62] | I. Hernandez, S.W. Bott, A.S. Patel, C.G. Wolf, A.R. Hospodar, S. Sampathkumar and W.H. Shrank, Pricing of monoclonal antibody therapies: Higher if used for cancer? Am J Manag Care 24: ((2018) ), 109–112. |
[63] | J.F. Santos-Neto, F.O. Oliveira, K.V.S. Hodel, L.M.S. Fonseca, R. Badaró and B.A.S. Machado, Technological Advancements in Monoclonal Antibodies, TheScientificWorldJournal, 2021. 2021, 6663708. doi: 10.1155/2021/6663708. |
[64] | B. Kelley, Developing therapeutic monoclonal antibodies at pandemic pace, Nat Biotechnol 38: ((2020) ), 540–545. |