Application of extracorporeal carbon dioxide removal combined with continuous blood purification therapy in ARDS with hypercapnia in patients with critical COVID-19
Abstract
INTRODUCTION:
Coronavirus disease-19 (COVID-19) is a new type of epidemic pneumonia caused by severe acute respiratory syndrome coronavirus 2 (SARS-CoV-2). The population is generally susceptible to COVID-19, which mainly causes lung injury. Some cases may develop severe acute respiratory distress syndrome (ARDS). Currently, ARDS treatment is mainly mechanical ventilation, but mechanical ventilation often causes ventilator-induced lung injury (VILI) accompanied by hypercapnia in 14% of patients. Extracorporeal carbon dioxide removal (ECCO2R) can remove carbon dioxide from the blood of patients with ARDS, correct the respiratory acidosis, reduce the tidal volume and airway pressure, and reduce the incidence of VILI.
CASE REPORT:
Two patients with critical COVID-19 combined with multiple organ failure undertook mechanical ventilation and suffered from hypercapnia. ECCO2R, combined with continuous renal replacement therapy (CRRT), was conducted concomitantly. In both cases (No. 1 and 2), the tidal volume and positive end-expiratory pressure (PEEP) were down-regulated before the treatment and at 1.5 hours, one day, three days, five days, eight days, and ten days after the treatment, together with a noticeable decrease in PCO2 and clear increase in PO2, while FiO2 decreased to approximately 40%. In case No 2, compared with the condition before treatment, the PCO2 decreased significantly with down-regulation in the tidal volume and PEEP and improvement in the pulmonary edema and ARDS after the treatment.
CONCLUSION:
ECCO2R combined with continuous blood purification therapy in patients with COVID-19 who are criti-cally ill and have ARDS and hypercapnia might gain both time and opportunity in the treatment, down-regulate the ventilator parameters, reduce the incidence of VILI and achieve favorable therapeutic outcomes.
1Introduction
Coronavirus disease-19 (COVID-19) is caused by the severe acute respiratory syndrome coronavirus 2 (SARS-CoV-2). It has the characteristics of acute onset, fever, fatigue, and dry cough. The population is generally susceptible to COVID-19, which will mainly cause lung injury. Some cases may develop severe acute respiratory distress syndrome (ARDS) [1, 2]. Currently, the treatment of ARDS is mainly mechanical ventilation, but this often causes ventilator-induced lung injury (VILI). Research has found that hypercapnia can develop in 14% of patients with ARDS who are ventilated with pulmonary protective ventilation [3]. Extracorporeal carbon dioxide removal (ECCO2R) is an extracorporeal gas exchange technique. The ECCO2R devices consist of an output tube, a pump, the membrane lung, and a return tube to remove carbon dioxide and improve hypercapnia and the decompensated respiratory acidosis [4]. In the present study, two patients who were critically ill with COVID-19 and who had multiple organ failure were admitted to Shenzhen Third People’s Hospital on February 3, 2020. These patients were treated with ventilator-assisted breathing and suffered complications from hypercapnia. After consultation with the expert team, ECCO2R, combined with continuous renal replacement therapy (CRRT), was conducted to gain time and opportunity for treatment. The details were reported as follows.
1.1Clinical data
Case No 1: A 69-year-old male patient complaining of “fever for four days and dry cough for two days” was admitted on February 3, 2020. The patient had a fever without apparent cause on January 29, with a temperature of 37.6. There was no fear of cold or chills, no nasal congestion, or runny nose. He developed a dry cough on January 31, accompanied by body aches, chest tightness, and shortness of breath. The chest CT in the hospital showed ground-glass changes in both lungs, and the nasal swab tested positive for the nucleic acid of the novel coronavirus. Thus, the patient was transferred to the Infection Department of our hospital for further diagnosis and treatment. The patient had a history of hypertension for ten years with the long-term administration of Irbesartan and relatively good cont-rol of blood pressure. The patient also had a 5-year history of “mitral regurgitation” without treatment. The details of the epidemic history were as follows: Two weeks before admission, the patient flew with his wife from Shenzhen to Malaysia and Thailand. There were tourists with “colds” on the same flight. His wife was admitted to our hospital for treatment on February 3 due to “COVID-19.” The diagnosis at admission: 1. COVID-19; 2. Hypertension, grade 2 (High risk); 3. Mitral disease (mit-ral regurgitation).
Anti-viral medications, nebulization, oxygen therapy, immune regulation, anti-infection, and other active treatments were performed after hospitalization. On February 12, the patient developed shortness of breath with a respiratory rate of 29–36 times/minute. There was detected arterial blood gas oxygen partial pressure (PO2) of 56.4 mmHg, carbon dioxide partial pressure (PCO2) of 33 mmHg, and oxygenation index (OI) of 112.8 mmHg. The patient’s condition worsened, and ARDS occurred. The patient was transferred to ICU for tracheal intubation and ventilator-assisted ventilation. On February 13, the patient developed a hepatic injury, acute renal injury, and myocardial injury with a total detected bilirubin of 107.5 umol/L, an activity of prothrombin time of 65%, N-terminal brain natriuretic peptide of 5110 pg/mL, an isoenzyme of creatine kinase of 12.3 ng/mL, and blood creatinine of 177 umol/L. Plasma exchange and CRRT were conducted. On February 14, the detected arterial blood gas PO2 was 65.5 mmHg, PCO2 was 40.7 mmHg, and OI was 82 mmHg. As the patient had severe ARDS, and conventional treatment was invalid, the Vein-Vein extracorporeal membrane oxygenation (VV-ECMO) was performed. On the 5th day of ECMO support on February 18, with arterial blood gas PO2 of 115 mmHg, PCO2 of 40 mmHg, and OI of 288 mmHg, and with spontaneous breathing experiments, ECMO was withdrawn, and the CRRT continued. On February 21, the arterial blood gas PO2 was 132 mmHg, PCO2 was 39 mmHg, and OI was 357 mmHg. Due to the improved oxygenation, the tracheal intubation was removed and replaced with noninvasive ventilator-assisted ventilation.
On March 2, the patient developed shortness of breath again, with a breathing rate of 30–35 times/min. The detected arterial blood gas PO2 was 55 mmHg, PCO2 was 32 mmHg, and OI was 110 mmHg. Assisted ventilation with a tracheal intubation ventilator was conducted.
On March 5, the patient had an arterial blood gas PO2 of 71 mmHg, PCO2 of 91 mmHg, OI of 89 mmHg, and had developed carbon dioxide retention. With the consultation of the expert team, ECCO2R, combined with life-supporting CRRT therapy, was conducted.
The course of therapy: The EQUA Smart blood purification system produced by the Italian Belk Company was adopted. The hollow fiber membrane oxygenator was CX*RW05RW produced by Terumo with a membrane area of 0.5 m2, blood flow velocity of 250–300 mL/min, and oxygen flow velocity of 4–10 L/min. The sequence of the oxygenator connection was as follows: the blood flows at the venous end of the temporary dialysis catheter in the right femoral vein ⟶ membrane oxygenator ⟶ dialyzer ⟶ blood return at the venous end of the catheter. Due to the low platelet count, argatroban was administered for anticoagulation at the dosage of 1–2 ug/(Kg•min) to maintain activated partial thromboplastin time (APTT) or INR at the venous return end at 1.5–2.5 times the basic value. The above treatments were continued, and the oxygenation and carbon dioxide retention improved after 90 minutes of the combination therapy of CRRT and ECCO2R. The ventilator parameters were down-regulated to avoid rapid deterioration of the disease, which gained therapeutic time and opportunity for the patient. The changes in blood gas and ventilator parameters during the treatment are shown in Tables 1 and 2.
Table 1
The changes in blood gas before and after V-VECCO2R
The date | PH | PO2 | PCO2 | Fi02 | HCO3- | HCO3std | BE | OI |
(mmHg) | (mmHg) | (%) | (mmol/L) | (mmol/L) | (mmol/L) | (mmHg) | ||
March 5th# | 7.1 | 71 | 91 | 80 | 28.3 | 21.9 | –3.6 | 89 |
March 5th* | 7.34 | 107 | 57 | 70 | 30.8 | 28.3 | 4.3 | 153 |
March 6th | 7.33 | 91 | 59 | 70 | 28.4 | 25.9 | 1.2 | 130 |
March 8th | 7.32 | 137 | 52 | 70 | 26.8 | 25.1 | 0.2 | 196 |
March 10th | 7.39 | 132 | 48 | 60 | 29.1 | 27.5 | 3.2 | 220 |
March 13th | 7.36 | 121 | 59 | 40 | 33.3 | 29.7 | 6.0 | 302 |
March 15th | 7.36 | 132 | 54 | 40 | 30.50 | 27.9 | 3.7 | 330 |
Note: PO2: Oxygen partial pressure; PCO2: partial pressure of carbon dioxide; Fi02: fractional concentration of inspired oxygen; HCO3-: Actual Bicarbonate Radical; HCO3std: Standard Bicarbonate Radical; BE: base excess; OI: oxygenation index; #Blood gas before ECCO2R treatment; *Blood gas after ECCO2R treatment for 1.5 hours.
Table 2
The changes in ventilator parameters before and after V-VECCO2R
The date | Mode | Fi02(%) | VTe(ml) | PEEP(cmH2O) | RR(bpm) |
March 5th | SIMV+PCV | 80 | 645 | 12 | 22 |
March 8th | SIMV+PCV | 60 | 580 | 8 | 18 |
March 8th | SIMV+PCV | 70 | 429 | 8 | 25 |
March 11th | SIMV+PCV | 50 | 450 | 8 | 18 |
March 15th | SIMV+VCV | 40 | 460 | 7 | 25 |
Note: Mode: ventilatory mode; VTe: tidal volume; PEEP: positive end expiratory pressure; RR: respiratory rate.
Case No 2: A 73-year-old male patient with “fever for two days” was admitted on January 22, 2020. The diagnosis at admission: 1. COVID-19, critical type; 2. Pneumonia, critical type; 3. Multiple organ dysfunction syndrome (MODS) (Septic shock, severe ARDS, acute renal insufficiency); 4. Chronic obstructive pulmonary disease (COPD); 5. Hypertension, grade 2 (severely high risk); 6. Sclerosis of the coronary artery and aorta; 7. Severe acquired myasthenia; 8. Venous thrombosis in bilateral lower limbs and the right upper limb. The duration of the disease was 56 days. The vital signs on the 53rd day of admission, the 42nd day of mechanical ventilation, and the 24th day of tracheotomy were as follows: T 36.2°C, P 85 times/min, R20 times/min, BP 159/83 mmHg. Due to the aggravation of the infection, the disease’s condition changed, with carbon dioxide retention and hypercapnia. With the addition of a device to remove carbon dioxide based on CRRT, the PCO2 stopped rising and gradually decreased. At the same time, the pulmonary lesions were absorbed after the enhancement of blood ultra-filtration by CRRT, as shown in Table 3 and Table 4. The comparison of the chest radiographs before and after treatment indicated that the multiple patchy opacities in the bilateral lungs decreased. The lesions in the bilateral lungs were absorbed, as shown in Fig. 1.
Table 3
The changes in blood gas before and after V-VECCO2R
The date | PH | PO2 | PCO2 | Fi02 | HCO3- | HCO3std | BE | OI |
(mmHg) | (mmHg) | (%) | (mmol/L) | (mmol/L) | (mmol/L) | (mmHg) | ||
March 14th* | 7.21 | 66 | 113 | 100 | 45.2 | 34.2 | 12.2 | 66 |
March 14th# | 7.23 | 74 | 88 | 100 | 36.9 | 30.2 | 6.9 | 74 |
March 16th | 7.34 | 103 | 68 | 70 | 36.7 | 31.8 | 8.8 | 147 |
March 18th | 7.36 | 133 | 65 | 90 | 36.7 | 32.2 | 9.3 | 148 |
March 23th | 7.35 | 78 | 50 | 40 | 27.5 | 25.6 | 1.0 | 195 |
Note: #Blood gas before ECCO2R treatment; *Blood gas after ECCO2R treatment for 2 hours.
Table 4
The changes in ventilator parameters before and after V-VECCO2R
The date | Mode | Fi02(%) | VTe(ml) | PEEP(cmH2O) | RR(bpm) |
March 14th | A/C+VCA | 100 | 425 | 10 | 30 |
March 15th | A/C+VCA | 85 | 385 | 9 | 25 |
March 17th | A/C+VCA | 70 | 356 | 9 | 24 |
March 20th | A/C+VCA | 90 | 327 | 7 | 25 |
March 22th | A/C+VCA | 90 | 279 | 7 | 30 |
Note: Mode: ventilatory mode; VTe: tidal volume; PEEP: positive end expiratory pressure; RR: respiratory rate.
Fig. 1
Chest radiograph comparison before and after treatment in case 2.
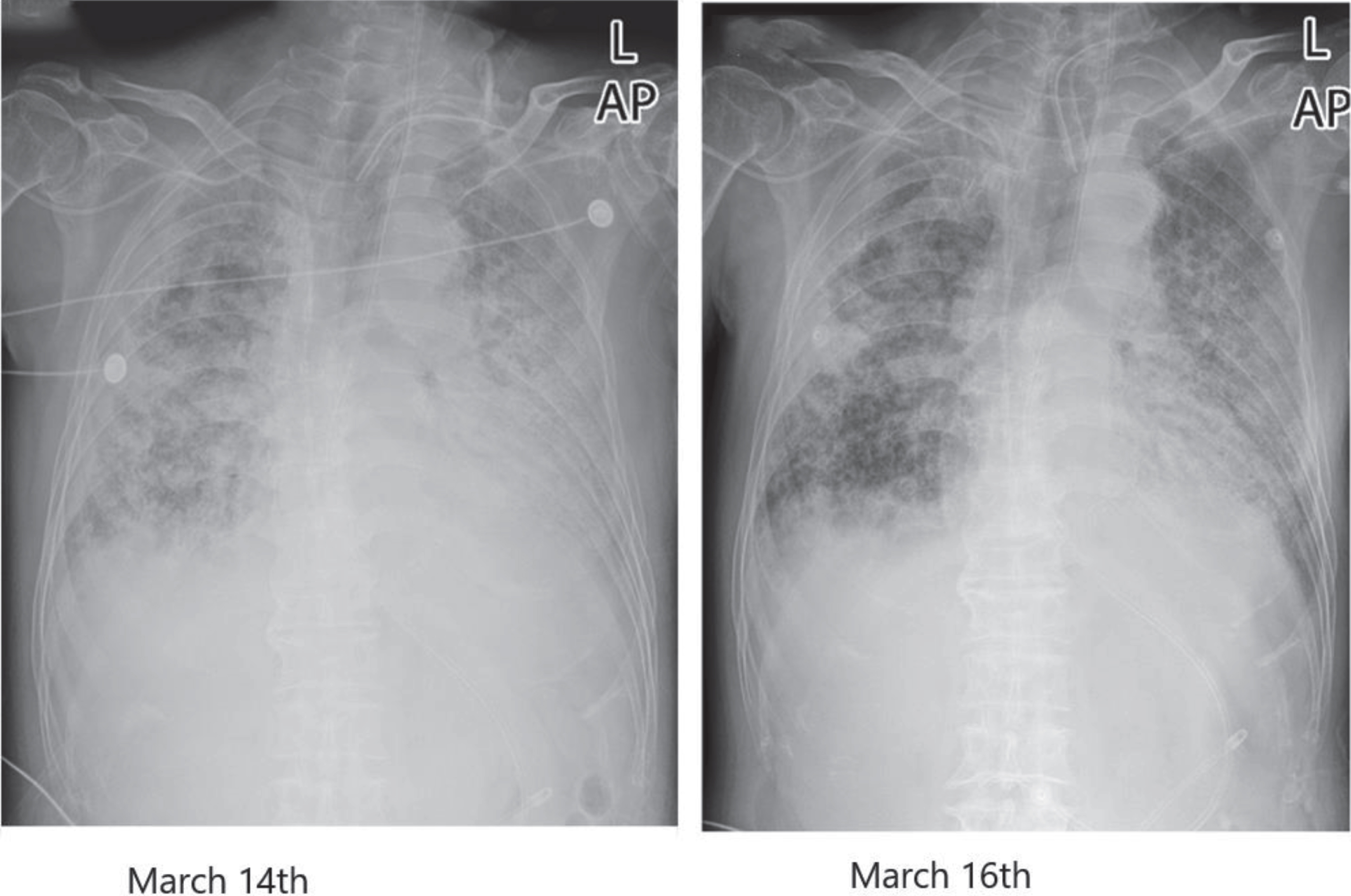
2Discussion
COVID-19 is the seventh coronavirus that can infect humans. It belongs to the β-coronavirus with a diameter of 60–140 nm. The genetic characteristics are significantly different from that of the SARS-CoV and MERS-CoV. A review discuss evidence that SARS-CoV evolved towards greater ‘fitness’ in the human host during the course of the outbreak,The major routes of transmission of SARS are droplet infection, aerosolization and fomites [29]. COVID-19 can infect cells through the binding of S protein to the angiotensin-converting enzyme 2 (ACE2) receptor on the surface of human cells. ACE2 is expressed in the lungs and kidneys; thus, it is highly infectious to humans [5]. ACE2 has been identified as the functional receptor for SARS-CoV, Hamming et al. investigated the localization of ACE2 protein in various human organs (oral and nasal mucosa, nasopharynx, lung, stomach, small intestine, colon, skin, lymph nodes, thymus, bone marrow, spleen, liver, kidney, and brain),The most remarkable finding was the surface expression of ACE2 protein on lung alveolar epithelial cells and enterocytes of the small intestine. which might provide possible routes of entry for the SARS-CoV [27]. A study used QRT-PCR to quantitatively map the transcriptional expression profile of ACE2 in 72 human tissues, the study confirmed that ACE 2 expression is high in renal and cardiovascular tissues, the novel observation has been made that ACE 2 shows comparably high levels of expression in the gastrointestinal system, in particular in ileum, duodenum, jejunum, caecum and colon [28]. Tian et al. found that in two patients with COVID-19 and lung cancer, the histopathology of lungs at the early infection mainly showed pulmonary edema, with protein exudating in the alveolar cavity. The hyaline membrane was not obvious [6]. A recent review of the scientific literature suggests that acute respiratory distress syndrome (ARDS) is associated with the damage of the pulmonary endothelium [24]. Recent studies showed that COVID-19 not only affects the lungs but beyond that the endothelial system, COVID-19 lead to microcirculatory impairments and in consequence to functional disorders of all inner organs,The combination of endothelial dysfunction with a generalized inflammatory state and complement elements may together contribute to the overall pro-coagulative state described in COVID-19 patients leading to venular as well as to arteriolar occlusions [25].
Wang et al. performed an autopsy on a 50-year-old male patient with COVID-19. The pulmonary histology revealed diffused alveolar injury with cellular fibrous mucus-like exudates in the bilateral lungs and obvious pulmonary cell desquamation and hyaline membrane formation in the right lung, indicating the occurrence of ARDS. The pulmonary edema and hyaline membrane formation in the left lung suggested the pathological features of early ARDS [7]. A pathological biopsy was conducted on a 66-year-old patient who died from COVID-19 in the Shenzhen Third People’s Hospital (our hospital). The main histopathology showed extensive pulmonary interstitial fibrosis with partial hyalinosis. The inflammatory cells, such as the monocytes, lymphocytes, and plasma cells, mainly infiltrated the pulmonary interstitium with several multinucleated giant cells and intracytoplasmic virus inclusion bodies. The fibrous exudation, exfoliated epithelial cells, and inflammatory cells were visible in the lumen of necrotizing bronchiolitis [8].
Wang et al. investigated the clinical data of 138 patients hospitalized with COVID-19 in which ARDS accounted for 19.6% [2]. A study followed up with 201 patients diagnosed with COVID-19 in Wuhan Jinyintan Hospital. The results found that 84 patients (41.8%) developed ARDS, and 44 of the 84 patients (52.4%) died [9].
Jung EM et al. implemented use of contrast enhanced ultrasound (CEUS) in severe cases of COVID-19 infection to assess pulmonary changes near the pleura, the results found that in all 11 cases, using CEUS low perfused areas of the pleura with adjacent hyperemia could be detected, while, with CT segmental contrast medium, gaps with subpleural compressions were found [23].
The main therapy for ARDS is mechanical ventilation, and mechanical ventilation often causes VILI. At present, lung-protective ventilation strategies are mostly used to prevent VILI. VILI should also be avoided as much as possible when implementing mechanical ventilation, in addition to ensuring the basic oxygenation and ventilation requirements. The PEEP should be regulated to maintain the alveolar in an open state. The tidal volume should be restricted to avoid a too-high end-inhalation lung volume. The occurrence and severity of VILI are jointly determined by PEEP and the tidal volume [10]. With the restriction of the tidal volume and PEEP, the alveolar ventilation will decrease, and PaCO2 will increase, resulting in hypercapnia. Research has shown that hypercapnia could develop in 14% of the patients with ARDS who are treated with pulmonary protective ventilation [3]. A study concerning the lung protection ventilation on 32 patients found that when adjusting the tidal volume, the average airway pressure was less than 28 cmH20, and hypercapnia occurred in all patients [11].
Hypercapnia can cause injury to the lungs by destroying the alveolar epithelium, cell proliferation, neutrophils, and innate immune functions [12]. Severe hypercapnia alone might increase mortality. A follow-up of 25281 patients with severe hypercapnia showed that the fatality of patients with hypercapnia assisted by mechanical ventilation was significantly higher than those with hypocapnia and normal PH [13].
One single centre cross-section study indicates that severity of lung opacities in COVID-19 patients and correlates with CO2 retention in patients with ARDS [26].
ECCO2R is an external gas exchange technique that can remove carbon dioxide. Most of the carbon dioxide in the human body is dissolved in the blood in the form of bicarbonate and has linear dynamics without saturation. There is more soluble carbon dioxide in the blood than oxygen; 250 mL of carbon dioxide can be removed from less than 1 L of blood [14]. ECMO’s main function is oxygenation, and it can also remove carbon dioxide, but the risk is high with high blood flow requirements. The technical operation is difficult, with many complications. ECCO2R can remove carbon dioxide from the blood of patients with ARDS, correct the respiratory acidosis, reduce the tidal volume and airway pressure, reduce the incidence of VILI, and achieve lung-protective ventilation. In 2016, the British Thoracic Association and the British Intensive Care Association released ventilation management guidelines for adults with acute respiratory failure. The management guidelines for hypercapnic respiratory failure states that if lung-protective ventilation strategies have been adopted but there is an uncorrectable and severe case of hypercapnic acidosis (PH < 7.15), ECCO2R can be used to correct hypercapnia [15]. The National Institute of Health and Clinical Optimization issued interventional treatment guidelines, IPG564, in 2016. These stated that the indications for the use of ECCO2R to treat respiratory failure are those with extremely abnormal hypoxemia or extremely abnormal hypercapnia, or severe ARDS in adults with acute respiratory failure caused by sepsis, pneumonia, or thoracic trauma [16]. One study used ECCO2R in 33 patients with hypercapnia who underwent ventilator-assisted ventilation in ICU. Of these patients, 22 were eventually transferred from ICU [17]. A 2-year multicenter, prospective cohort study observed the effect of ECCO2R. It found that in patients with ARDS who had hypercapnia and who were treated using ECCO2R, the median tidal volume of the ventilator could be reduced from 5.9 to 4.1 mL/kg (P < 0.001) [18]. In the present study, compared with the results before treatment, the therapeutic effects were significant. The tidal volume and PEEP were down-regulated, together with an increase of PO2 and the decrease of FiO2 to approximately 40% after the treatment.
Li et al. retrospectively analyzed the renal function of 59 patients with COVID-19. Among them, 32 developed proteinuria, and 11 had elevated plasma creatinine, indicating that renal injury was common in patients with COVID-19 [19]. The proportion of patients with COVID-19 who developed acute kidney injury was 3% –7%, the proportion who received CRRT was 7% –9%, and the proportion of patients who received CRRT in the ICU was as high as 23% [20, 21]. The advantages of CRRT in the treatment of COVID-19 were as follows [22]: (1) Correct and maintain the water, electrolyte, and acid-base balance, maintain the stability of the internal environment, and provide life support; (2) Remove toxic substances such as metabolites; (3) Exert effective treatment for volume overload; (4) Effective control of high fever; (5) Improve the inflammation, endothelial function, and immune status. Therefore, the rational application of CRRT might be beneficial to the treatment of critically ill patients and reduce mortality.
ECCO2R, combined with CRRT, was used in the treatment of the two cases in the present study. The ECCO2R system was used under lung-protective ventilation to correct hypercapnia and respiratory acidosis, reduce the tidal volume, PEEP, and other mechanical ventilation parameters, and reduce the severity of VILI to assist the recovery of pulmonary function. The present treatment could be used to treat critically ill patients with COVID-19 who had respiratory failure and renal failure with carbon dioxide retention. At the same time, CRRT was conducted with the regulation from breathing to the internal environment, removal of inflammatory factors, volume management, and anticoagulation to achieve coordination and improvement between lung ⟶ kidney ⟶ infection ⟶ sepsis ⟶ coagulation disorder. As a result, the patient’s general condition could improve to a relatively stable state and gain treatment opportunities. The present therapy had provided patients with more suitable treatment modes to achieve good therapeutic effects and create more treatment opportunities, and the clinical application prospects were worth exploring.
Funding
Novel Coronavirus Infection prevention and control project of Guangdong Province, Project No. (2020B1111300004).
Conflict of interest
The authors declare that they have no competing interests.
Acknowledgments
We would like to acknowledge the hard and dedicated work of all the staff that implemented the intervention and evaluation components of the study.
References
[1] | COVID-19 Diagnosis and Treatment Protocol (trial 7th Edition) National Health Commission. journal of practical traditional chinese internal medicine, (2020) ,34: (03):3. http://wwwnhcgovcn/yzygj/s7653p/202003/46c9294a7dfe4cef80dc7f5912eb1989shtml. |
[2] | Wang D , Hu B , Hu C , Zhu F , Liu X , Zhang J , et al. Clinical characteristics of 138 hospitalized patients with 2019 novel coronavirus–infected pneumonia in Wuhan Jama. 2020. |
[3] | Kregenow DA , Rubenfeld GD , Hudson LD , Swenson ER . Hypercapnic acidosis and mortality in acute lung injury. Critical Care Medicine. (2006) ;34: :1–7. |
[4] | Morimont P , Batchinsky A , Lambermont B . Update on the role of extracorporeal CO2 removal as an adjunct to mechanical ventilation in ARDS. Critical Care. 19: :117. |
[5] | Xu X , Chen P , Wang J , Feng J , Zhou H , Li X , et al. Evolution of the novel coronavirus from the ongoing Wuhan outbreak and modeling of its spike protein for risk of human transmission. Science China-life Sciences. 2020. |
[6] | Tian S , Hu W , Niu L , Liu H , Xu H , Xiao S-Y . Pulmonary pathology of early phase 2019 novel coronavirus (COVID-19) pneumonia in two patients with lung cancer. Journal of Thoracic Oncology. 2020. |
[7] | Xu Z , Shi L , Wang Y , Zhang J , Huang L , Zhang C , et al. Pathological findings of COVID-19 associated with acute respiratory distress syndrome. The Lancet Respiratory Medicine. 2020. |
[8] | Luo W , Yu H , Gou J , Li X , Sun Y , Li J , et al. Clinical Pathology of Critical Patient with Novel Coronavirus Pneumonia (COVID-19). 2020. |
[9] | Wu C , Chen X , Cai Y , Zhou X , Xu S , Huang H , et al. Risk Factors Associated With Acute Respiratory Distress Syndrome and Death in Patients With Coronavirus Disease 2019 Pneumonia in Wuhan, China. JAMA Internal Medicine. 2020. |
[10] | Pinhu L , Whitehead T , Evans T , Griffiths M . Ventilator-associated lung injury. The Lancet. (2003) ;361: :332–40. |
[11] | Terragni PP , Del Sorbo L , Mascia L , Urbino R , Martin EL , Birocco A , et al. Tidal volume lower than 6 ml/kg enhances lung protectionrole of extracorporeal carbon dioxide removal. Anesthesiology: The Journal of the American Society of Anesthesiologists. (2009) ;111: :826–35. |
[12] | Doerr CH , Gajic O , Berrios JC , Caples S , Abdel M , Lymp JF , et al. Hypercapnic acidosis impairs plasma membrane wound resealing in ventilator-injured lungs. American Journal of Respiratory and Critical Care Medicine. (2005) ;171: :1371–7. |
[13] | Herridge MS , Tansey CM , Matté A , Tomlinson G , Diaz-Granados N , Cooper A , et al. Functional Disability 5 Years after Acute Respiratory Distress Syndrome. New England Journal of Medicine. 364: :1293–304. |
[14] | MacLaren G , Combes A , Bartlett RH . Contemporary extracorporeal membrane oxygenation for adult respiratory failure: life support in the new era. Intensive Care Medicine. (2012) ;38: :210–20. |
[15] | Davidson AC , Banham S , Elliott M , Kennedy D , Gelder C , Glossop A , et al. BTS/ICS guideline for the ventilatory management of acute hypercapnic respiratory failure in adults. Thorax. (2016) ;71: :ii1–ii35. |
[16] | [IPG564] Ipg. Extracorporeal carbon dioxide removal for acute respiratory failure. https://wwwniceorguk/guidance/ipg564. 2016. |
[17] | Winiszewski H , Aptel F , Belon F , Belin N , Chaignat C , Patry C , et al. Daily use of extracorporeal CO 2 removal in a critical care unit: indications and results. Journal of Intensive Care. (2018) ;6: :36. |
[18] | Augy J , Aissaoui N , Richard C , Maury E , Fartoukh M , Mekontso-Dessap A , et al. A 2-year multicenter, observational, prospective, cohort study on extracorporeal CO 2 removal in a large metropolis area. Journal of Intensive Care. (2019) ;7: :45. |
[19] | Li Z , Wu M , Guo J , Yao J , Liao X , Song S , et al. Caution on kidney dysfunctions of 2019-nCoV patients. MedRxiv. 2020. |
[20] | Huang C , Wang Y , Li X , Ren L , Zhao J , Hu Y , et al. Clinical features of patients infected with 2019 novel coronavirus in Wuhan, China. The Lancet. (2020) ;395: :497–506. |
[21] | Chen N , Zhou M , Dong X , Qu J , Gong F , Han Y , et al. Epidemiological and clinical characteristics of 99 cases of 2019 novel coronavirus pneumonia in Wuhan, China: a descriptive study. The Lancet. (2020) ;395: :507–13. |
[22] | NationalMedical Quality Control Center for Kidney Diseases, Blood Purification and Engineering Technology Branch of CPAM, Academic Committee of Blood Purification of PLA. Expert Opinion on CRRT Application in Treatment of Novel Coronavirus. 2020. |
[23] | Jung EM , Stroszczynski C , Jung F . Contrast enhanced ultrasound (CEUS) to assess pleural pulmonal changes in severe COVID-19 infection: First results. Clinical Hemorheology and Microcirculation. (2020) ;75: :1–8. |
[24] | Millar FR , Summers C , Griffiths MJ , et al. The pulmonary endothelium in acute respiratory distress syndrome: insights and therapeutic opportunities. Thorax. (2016) ;71: :462–73. |
[25] | Jung F , Krüger-Genge A , Franke RP , Hufert F , Küpper J-H . COVID-19 and the endothelium. Clin Hemorheol Microcirc. (2020) ;75: (1):7–11. |
[26] | Peschel G , Jung EM , Fisser C , Putz FJ , Wertheimer T , Sinner B , Lunz D , Jung F , Müller M . Clin Hemorheol Microcirc. Interstitial lung opacities in patients with severe COVID-19 pneumonia by bedside high-resolution ultrasound in association to CO2 retention. Peschel G, Jung EM, Fisser C, Putz FJ, Wertheimer T, Sinner B, Lunz D, Jung F, Müller M. Clin Hemorheol Microcirc. 2020 Nov 28. doi: 10.3233/CH-200925 |
[27] | Hamming I , Timens W , Bulthuis MLC , Lely AT , Goor HV . Tissue distribution of ACE2 protein, the functional receptor for SARS coronavirus. A first step in understanding SARS pathogenesis. The Journal of Pathology. (2004) ;203: :631–7. |
[28] | Dan H , Gilbert M , Borman R , Clark KL . Quantitative mRNA expression profiling of ACE 2, a novel homologue of angiotensin converting enzyme. Febs Letters. (2002) ;532: :107–10. |
[29] | Peiris JS , Guan Y , Yuen KY . Severe acute respiratory syndrome. Nat Med. (2004) ;10: :S88–97. |