Development of biliary stent applying the antibacterial activity of silver: A literature review
Abstract
BACKGROUND:
Endoscopic transpapillary stenting is commonly performed in patients with obstructive jaundice caused by a biliary stricture. Although the plastic stent (PS) is widely used for biliary drainage because of the low-cost and easy procedure, patency is short after placement in the bile duct because of the small diameter. Dysfunction of PS is primarily caused by biliary sludge that forms as a result of bacterial adhesion and subsequent biofilm formation on the inner surface of the stent. It is well known that silver ions have excellent antibacterial activity against a wide range of microorganisms.
OBJECTIVE:
This review provides an overview and perspective of the significance of silver-coated biliary stents.
METHODS:
We collected literature regarding silver-coated biliary stents, reviewed the current research/development status and discussed their possible usefulness.
RESULTS:
To date, several in vivo/vitro studies evaluated the patency of silver-blended or silver-coated biliary stents. These studies suggested that the silver coating on a PS was likely to prolong the patency period.
CONCLUSION:
The development of biliary stents using silver is expected to prolong stent patency and prevent frequent stent replacement.
1.Introduction
Biliary tract obstruction caused by biliary tract cancer, pancreatic head cancer, and bile duct stones causes jaundice and with bacterial infection can cause acute cholangitis, which can result in serious outcomes depending on the circumstances (Fig. 1). Therefore, patients with obstructive jaundice (irrespective of the presence or absence of infection) are generally treated with endoscopic biliary stenting with a stent placed endoscopically via the duodenal papilla. If the patient’s condition is resectable, the stent can be temporarily placed until the day of surgery; however, in the event of obstructive jaundice caused by unresectable neoplastic lesions, a permanent stent is placed. In such instances, a plastic stent (PS, Fig. 2) or self-expandable metal stent (SEMS, Fig. 3) is used. Although PSs are inexpensive and technically easy to use, they become easily obstructed as they have a narrow lumen. In contrast, SEMSs have a wide lumen and are less likely to become obstructed; however, they are expensive and pose a problem in terms of medical economics. The cause of stent obstruction is biofilm formation as a result of infection within the stent, i.e. if biofilm formation can be inhibited through some type of antibacterial action (such as silver), long-term patency could be achieved even with PSs that have a narrow diameter. In this paper, we reviewed literature on the significance and efficacy of silver-coated biliary stents.
Fig. 1.
Diagram of the hepatobiliary system. Each disease causes the obstruction of the bile duct.
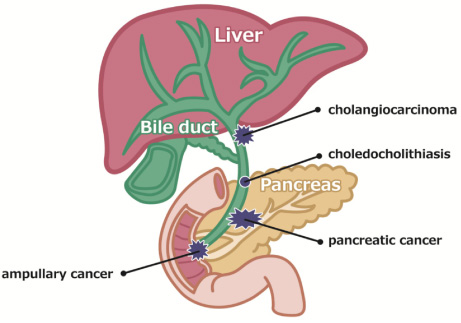
Fig. 2.
Biliary plastic stent (PS), straight type with double flaps (Advanix J®, Boston Scientific Japan, Tokyo). PS is easy to place, but the patency period is short because of the small diameter and biofilm formation.
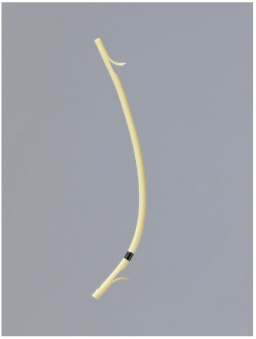
Fig. 3.
Biliary self-expandable metal stent (SEMS) with silicon cover (HANAROSTENT®, Boston Scientific Japan, Tokyo). SEMS has large diameter and long patency period, but the cost is high.
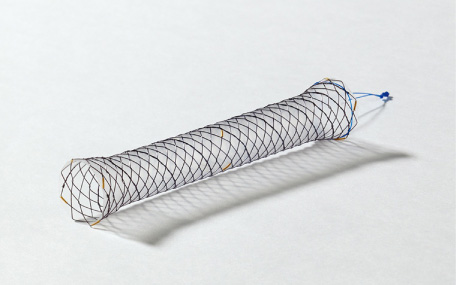
2.Biliary stent occlusion in patients with obstructive jaundice
The major limitation of endoscopic stent placement is stent occlusion. It has been reported that the median patency period of PS ranges from 80 days to 126 days [1,2] and stent exchanges are often needed at intervals of approximately 3 months to prevent stent occlusion even if the PS remains functional [3]. Compared with PSs, SEMSs have the benefits of prolongation of the stent patency period. Therefore, SEMSs are more often used than PSs for patients who are expected to survive for more than 3 months [4,5]. However, in benign biliary strictures, the average unobstructed period is approximately 6–9 months, whereas the average period for malignant biliary obstructions is 4–8 months [3].
According to previous studies, stent occlusion is caused by the deposition of biliary sludge, which is composed of cholesterol crystals, calcium bilirubinate and palmitate, bacteria and/or fungi, microbial byproducts, proteins, dietary fibers, and glycoproteins [6–8]. In vitro studies suggest that bacterial adhesion is the driving force of sludge development [9,10]. In particular, bacterial infection and biofilm formation on the surface of biliary stents are the main factors in stent occlusion. When a stent is placed in the bile duct, bacteria attach to the stent surface by means of their pili and/or glycocalyx to form a biofilm [11]. Biofilms formed inside the biliary stents consist of a mixed spectrum of bacterial communities [12]. Most of these bacteria, generally originating from the enterocolon, are uncultivable by standard culture methods. Schneider et al. [13] analyzed the spectrum and number of biliary microorganisms on the surface of stents in relation to sludge. In their study, the most prevalent microorganisms were Enterococcus species (spp.) (22%), followed by Klebsiella spp. (10%) and Candida spp. (8%). Colonization of the stents mainly began with aerobic gram-positive bacteria (88%) and Candida spp. (51%), whereas stents with an indwelling period of 60 days showed an almost equal colonization rate of aerobic gram-negative (96%) and aerobic gram-positive bacteria (99%) and a high proportion of anaerobes (69%).
β-glucuronidase and lecithinase (or phospholipase C) enzymatic activities of colonizing microorganisms lead to the precipitation of calcium bilirubinate and palmitate, thereby contributing to sludge accumulation within the biliary system followed by stent occlusion [9,10]. Thus, prevention of bacterial infection before the formation of bacterial biofilms on the surface of the stent is critical to achieve long-term stent patency.
3.Biofilm as the cause of stent occlusion
In nature, unicellular bacteria prefer to accumulate to form a surface-attached multicellular consortium called a biofilm rather than live as isolated planktonic cells [14]. Biofilm refers to the complex, sessile communities of microbes found either attached to a surface or buried firmly in an extracellular matrix as aggregates (Fig. 4).
Fig. 4.
Biofilm on the surface of a plastic stent observed with the scanning electron microscope (×3000). The meshwork-like structures around the bacteria, characteristic of biofilm-forming bacteria.
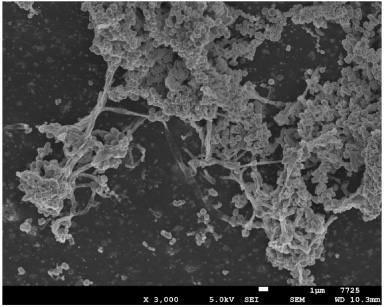
In the natural setting, bacteria composed of a single species are seldom found in biofilms, and most biofilms are multispecies consortia with a synergistic effect on biofilm formation [15]. Biofilm formation is a common phenomenon in which virtually every material that comes into contact with naturally occurring fluids becomes rapidly covered with bacteria [16,17]. Biofilms are composed of bacterial cells attached to a biotic or abiotic surface and extracellular polymeric substances excreted by the participant cells. Cells within biofilms are distinct from planktonic cells both physiologically and genetically. The gene expression profile of biofilm-associated cells is significantly different from that of planktonic cells [18–21]. According to the previous literature, in a gene expression profile study of Escherichia coli, the transcription of 38% of the genes was affected within biofilms [20]. Biofilms provide bacteria with various survival and metabolic advantages over the planktonic form. By forming biofilms, bacteria can avail themselves of various survival and metabolic advantages, including survival in a nutrient-deprived environment, resistance to environmental stresses, resistance to biocides and antibiotics, and better abilities for the acquisition of foreign genes [22–24]. Regarding biofilm formation, Lübbert et al. [25] reported that enterococci play a significant role in microbial colonization of biliary stents. Additionally, Yu et al. [26] reported the attachment of fibronectin to the inner surface of the stents within 24 h of exposure to bile. Another contributing factor is the bile immunoglobulin–bacteria complex, which further promotes the binding of bacteria to the inner surface of the stents [27]. In patients with cholangitis, in which these factors are expected to be high, there are higher risks of biofilm formation and stent occlusion.
4.Function of silver ions in antimicrobial activity
One area of rapidly growing interest in the development of new antimicrobials is the use of nanoparticles coupled with metals. Several methods have been proposed to prevent biofilm formation, but none have yielded promising results except silver, which has a durable antibacterial effect. Silver has shown antimicrobial activity against a wide range of microorganisms. Therefore, silver-based nanomaterials are being used for their bactericidal activity. Compared to antibiotics, silver acts more broadly against a wide range of bacteria, and microbes without intrinsic resistance cannot gain resistance [28,29]. Silver acts by binding to membranes, enzymes, and nucleic acids. Consequently, the respiratory chain is inhibited; therefore, the aerobic metabolism of microorganisms is disturbed. Antibacterial effects are directly proportional to silver concentrations and therefore directly depend on silver release into the surrounding environment [30,31]. Several studies have confirmed higher bactericidal potency of silver against gram-negative bacteria compared with that against gram-positive bacteria [32,33]. Additionally, in 2003, Burrell reported that if a silver ion solution of 60 ppm is used, most bacteria and fungi can be controlled. Furthermore, the same study revealed that silver preparations are extremely effective against Pseudomonas aeruginosa, a pathogenic bacteria of nosocomial and opportunistic infections, and silver was considered a therapeutic agent that was as highly effective as antibiotics [34].
5.Medical devices utilizing silver ions
As mentioned above, the antimicrobial action of silver ions is promising, and they have been used to date in various pharmaceuticals for medical treatment. Recently, several new antibacterial materials and catheters have been studied and developed to counteract biofilm. Because the silver ion causes a dramatic decrease in biofilm bioactivity measured by an ATP bioluminescence assay, the silver ions are more effective against biofilm-forming bacteria at an extremely low concentration compared with other antibacterial agents, and most types of antibacterial catheters developed and sold in the market apply the antibacterial action of silver ions [35].
With regard to biliary stents, in 1992, Leung et al. [36] compared the number of bacteria on the surface of PS with silver coating against that on the surface of PS without silver coating and found that in vitro, bacterial adhesion on silver-coated PS was broadly reduced by 10–100-fold in a dose-dependent manner, which suggested that the silver coating was effective against stent obstruction. Although no extensive studies were subsequently conducted for some time, in 2016, Wen et al. [37] established a model of bacterial cholangitis in pigs and, upon placing a silver-coated stent, confirmed its effectiveness. In this study, a significantly lower inflammatory response was observed in the group with silver-coated stent than in the group without silver-coated stent, and electron microscopy clearly revealed that fewer bacteria adhered to the stent surface. We have also reported the effectiveness of silver ion-coated PS in a theoretical study [38], and similar to the study by Wen et al., we found minimal bacterial adhesion on the surface of silver ion-coated stents, with only several cells found and no meshwork or biofilm formation observed. Wen et al. conducted an examination in a short period of 1 or 7 days after stent placement; however, we performed our culture after the stent was soaked in infected bile over a long period, i.e., 4 weeks, and even after such a long period, no bacterial adhesion as noted on the surface of the silver-coated stent, which suggested that stent patency could be achieved over a long period. Furthermore, Yang et al. [39] developed a novel biliary stent coated with silver nanoparticles (AgNPs) and investigated its function using pigs in vitro and in vivo. In vitro antibacterial experiments indicated that the AgNP-coated biliary stent exhibited highly efficient antibacterial activity and strong, broad-spectrum antibacterial function in both the short and long term. Importantly, the use of the AgNP-coated biliary stent significantly prolonged the unobstructed period of the biliary system and improved survival in preclinical studies as a result of its antimicrobial activity and decreased granular tissue formation on the surface of the anastomotic biliary structure, providing a novel and effective treatment strategy for symptomatic biliary strictures. The effectiveness of the silver coating on SEMS has also been examined. Lee et al. [40] reported that silver particle (Ag-P)-integrated silicone polymer-covered SEMS reduced biofilm or sludge formation and stent-related pathologic changes in the bile duct and had a long-term antibacterial effect. Smaller-diameter stents have a higher risk of occlusion due to biofilm formation. A larger diameter entails greater bile flow velocity and subsequently less predisposition to bile salt precipitation, protein accumulation, and biofilm formation. Thus, large-diameter stents have always been better than smaller-diameter stents in terms of durability [41], and one of the major advantages of metallic stents is in fact its large diameter [42].
A concern with the use of silver-coated PS in the human biliary system is the possible gut absorption of the silver released in bile. Ag-Ps are toxic to mammalian cells, as reported in various in vitro studies [43–45]. In 1995, Suzuki [46] examined the antibacterial effect and safety of stents containing silver. The study revealed that the antibacterial effect of stents with a silver content of 0.04 wt% was ineffective in many patients, whereas a silver content of 1.5 wt% was effective in several patients, and that the antibacterial activity increased with an increase in silver content. Furthermore, the total amount of silver elution soaked up by bile collected with stents containing silver has been examined in a theoretical and experimental study. The study revealed that when trial stents containing 1.5 wt% of silver were soaked in bile for 5 months, the total amount of silver elusion was 0.0026 ppm, whereas when a trial stent containing 0.04 wt% of silver was soaked in bile for 6 months, trace amounts of 0.0012 ppm were found. Furthermore, Liu et al. [47] evaluated AgNPs for antibacterial activities and cytotoxicity. They reported that the antibacterial activity of AgNPs against Escherichia coli started at the effective concentration of 0.1–1 ppm, while that against Staphylococcus aureus started at higher concentrations of 1–10 ppm. AgNPs of smaller sizes had better antibacterial properties but higher cytotoxicity. In contrast, they also reported that silver ions were strongly bactericidal at a concentration of 0.1 ppm, and significant cytotoxicity was detected at higher concentrations (10 ppm) of small-sized AgNPs, but not at 1 ppm. To investigate the effect duration of the AgNP biliary stent, Yang et al. [39] conducted a Ag+ release experiment through measurements of Ag+ concentration using inductively-coupled plasma mass spectrometry. They found that the concentration of Ag+ release of biliary stent coated with AgNPs achieved the maximum (8.52 ± 0.81 μg/L) on the first day, was significantly decreased during the initial 10 days (2.86 ± 0.58 μg/L), and then gradually reduced at 20, 30, 45, and 60 days, corresponding to 2.53 ± 0.46, 2.39 ± 0.29, 2.07 ± 0.23, and 1.81 ± 0.17 μg/L, respectively. These data indicated that the Ag+ release in the AgNP-coated biliary stent was an effective and slow process. Based on these results, it has been found in vitro that, in stents containing silver at a concentration with antibacterial action and silver-coated stents, silver elution is at a level that does not cause cellular damage. In vivo, silver ions that leak from biliary stents pose a problem when absorbed from the intestinal tract; however, according to Leung et al. [48], there is little absorption via the intestinal tract, and even if absorbed for a long period, the only problem is argyria. This is unlikely to be a serious problem for patients with malignant disease and limited prognosis. While there are no reports on the toxicity of silver absorbed via the intestinal tract in humans, Lee et al. investigated the efficacy and safety of SEMSs covered with a silicone membrane containing integrated 250-nm Ag-Ps using pigs. They found that no Ag-Ps indicative of systemic absorption were isolated from the serum in vitro or in vivo [49].
As mentioned above, the silver-based bile duct stents have been studied. However, they have not yet been put into practical use. Although the silver coating on a plastic stent is likely to prolong the patency period, the period may be limited because of the small diameter. The past controlled studies showed SEMS had remained patent up to a median of 9 months [2,4,50,51]. PS is extremely inexpensive compared to SEMS, and reintervention is easily performed in the event of PS occlusion. As such, if the patency period of PS using silver is at least equivalent to SEMS, PS using silver would be recommended as the first choice in the treatment of biliary obstruction. Therefore, we must carry out research and development so that even PS with a small diameter can have a patency period of at least nine months. Although the silver-coated SEMS seems ideal, high prices are likely to be a significant obstacle to practical use. In the future, it may be ideal to use silver-coated PS and silver-coated SEMS properly according to the prognosis of the patient.
6.Conclusions
This review article summarizes previous studies of the biliary stent applying the antibacterial activity of silver. The development of biliary stents using silver is expected to prolong stent patency and prevent frequent stent replacement. At present, silver-based bile duct stents have not yet been put into practical use, however we believe that this research and development will greatly contribute to improving the patient’s quality of life and cost-effectiveness of the treatment as the medical economy.
Conflict of interest
There is no conflict of interest related to this manuscript.
References
[1] | A.M. Van Berkel, C. Boland, W.K. Redekop, J.J.G.H.M. Bergman, A.K. Groen, G.N.J. Tytgat , A prospective randomized trial of Teflon versus polyethylene stents for distal malignant biliary obstruction, Endoscopy 30: (8) ((1998) ), 681–686. doi:10.1055/s-2007-1001388. |
[2] | P.H. Davids, A.K. Groen, E.A.J. Rauws, G.N. Tytgat and K. Huibregtse, Randomized trial of self-expanding metal stents versus polyethylene stents for distal malignant biliary obstruction, Lancet 340: ((1992) ), 1488–1492. doi:10.1016/0140-6736(92)92752-2. |
[3] | J.M. Dumonceau, A. Tringali, D. Blero, J. Devière, R. Laugiers, D. Heresbach , Biliary stenting: Indications, choice of stents and results: European Society of Gastrointestinal Endoscopy (ESGE) clinical guideline, Endoscopy 44: (3) ((2012) ), 277–298. doi:10.1055/s-0031-1291633. |
[4] | F. Prat, O. Chapat, B. Ducot, T. Ponchon, G. Pelletier, J. Fritsch , A randomized trial of endoscopic drainage methods for inoperable malignant strictures of the common bile duct, Gastrointest Endosc 47: (1) ((1998) ), 1–7. doi:10.1016/s0016-5107(98)70291-3. |
[5] | D. Walter, P.G. van Boeckel, M.J. Groenen, B.L. Weusten, B.J. Witteman, G. Tan , Cost efficacy of metal stents for palliation of extrahepatic bile duct obstruction in a randomized controlled trial, Gastroenterology 149: (1) ((2015) ), 130–138. doi:10.1053/j.gastro.2015.03.012. |
[6] | N. Dowidar, H.J. Kolmos, H. Lyon and P. Matzen, Clogging of biliary endoprostheses. A morphologic and bacteriologic study, Scand J Gastroenterol 26: (11) ((1991) ), 1137–1144. doi:10.3109/00365529108998605. |
[7] | E.W. McAllister, L.C. Carey, P.G. Brady, R. Heller and S.G. Kovacs, The role of polymeric surface smoothness of biliary stents in bacterial adherence, biofilm deposition, and stent occlusion, Gastrointest Endosc 39: (3) ((1993) ), 422–425. doi:10.1016/s0016-5107(93)70120-0. |
[8] | U. Weickert, T. Venzke, J. König, J. Janssen, K. Remberger and L. Greiner, Why do bilioduodenal plastic stents become occluded? A clinical and pathological investigation on 100 consecutive patients, Endoscopy 33: (9) ((2001) ), 786–790. doi:10.1055/s-2001-16519. |
[9] | J.W.C. Leung, T.K.W. Ling, J.L.S. Kung and J. Vallance-Owen, The role of bacteria in the blockage of biliary stents, Gastrointest Endosc 34: (1) ((1988) ), 19–22. doi:10.1016/s0016-5107(88)71223-7. |
[10] | N. Dowidar, H.J. Kolmos and P. Matzen, Experimental clogging of biliary endoprostheses. Role of bacteria, endoprosthesis material, and design, Scand J Gastroenterol 27: (1) ((1992) ), 77–80. doi:10.3109/00365529209011171. |
[11] | J.W. Costerton, G.G. Gessey and K.J. Cheng, How bacteria stick, Sci Am 238: (1) ((1978) ), 86–95. doi:10.1038/scientificamerican0178-86. |
[12] | G. Donelli, E. Guaglianone, R. Di Rosa, F. Fiocca and A. Basoli, Plastic biliary stent occlusion: Factors involved and possible preventive approaches, Clin Med Res 5: (1) ((2007) ), 53–60. doi:10.3121/cmr.2007.683. |
[13] | J. Schneider, A. Hapfelmeier, J. Fremd and P. Schenk, Biliary endoprosthesis: A prospective analysis of bacterial colonization and risk factors for sludge formation, PLoS One 9: (10) ((2014) ), e110112. doi:10.1371/journal.pone.0110112. |
[14] | S.A.I. Ahmad, S.N. Bari and M. Mohiuddin, Biofilm: Multicellular living of the unicellular bacteria, Int J Biosci 2: (6) ((2012) ), 59–71. |
[15] | J.W. Leung, Y.L. Liu, T. Desta, E. Libby, J.F. Inciardi and K. Lam, Is there a synergistic effect between mixed bacterial infection in biofilm formation on biliary stents? Gastrointest Endosc 48(3) (1998), 250–257. doi:10.1016/s0016-5107(98)70186-5. |
[16] | J.W. Costerton, K.-J. Cheng, G.G. Geesey, T.I. Ladd, J.C. Nickel, M. Dasgupta , Bacterial biofilms in nature and disease, Annu Rev Microbiol 41: ((1987) ), 435–464. doi:10.1146/annurev.mi.41.100187.002251. |
[17] | J.W. Costerton, Z. Lewandowski, D. DeBeer, D. Caldwell, D. Korber and G. James, Biofilms, the customized microniche, J Bacteriol 176: (8) ((1994) ), 2137–2142. doi:10.1128/jb.176.8.2137-2142.1994. |
[18] | L.A. Pratt and R. Kolter, Genetic analysis of Eschericia coli biofilm formation: Roles of flagella, motility, chemotaxis and type I pili, Mol Microbial 30: (2) ((1998) ), 285–293. doi:10.1046/j.1365-2958.1998.01061.x. |
[19] | G.A. O’Toole and R. Kolter, Flagellar and twitching motility are necessary for Pseudomonas aeruginosa biofilm development, Mol Microbial 30: (2) ((1998) ), 295–304. doi:10.1046/j.1365-2958.1998.01062.x. |
[20] | C. Prigent-Combaret, O. Vidal, C. Dorel and P. Lejeune, Abiotic surface sensing and biofilm-dependent regulation of gene expression in Eschericia coli, J Bacteriol 181: (19) ((1999) ), 5993–6002. doi:10.1128/JB.181.19.5993-6002.1999. |
[21] | P. Genevaux, S. Muller and P. Bauda, A rapid screening procedure to identify mini-Tn10 insertion mutants of Eschericia coli K-12 with altered adhesion properties, FEMS Microbiol Lett 142: (1) ((1996) ), 27–30. doi:10.1111/j.1574-6968.1996.tb08402.x. |
[22] | M.R.W. Brown and P. Gilbert, Sensitivity of biofilms to antimicrobial agents, J Appl Bacterial 74: ((1993) ), 87S–97S. doi:10.1111/j.1365-2672.1993.tb04345.x. |
[23] | M.E. Davey and G.A. O’Toole, Microbial biofilms: From ecology to molecular genetics, Microbiol Mol Biol Rev 64: (4) ((2000) ), 847–867. doi:10.1128/mmbr.64.4.847-867.2000. |
[24] | P. Watnick and R. Kolter, Biofilm, city of microbes, J Bacteriol 182: (10) ((2000) ), 2675–2679. doi:10.1128/jb.182.10.2675-2679.2000. |
[25] | C. Lübbert, K. Wendt, J. Feisthammel, A. Moter, N. Lippmann, T. Busch , Epidemiology and resistance patterns of bacterial and fungal colonization of biliary plastic stents: A prospective cohort study, PLoS One 11: (5) ((2016) ), e0155479. doi:10.1371/journal.pone.0155479. |
[26] | J.L. Yu, R. Andersson and A. Ljungh, Protein adsorption and bacterial adhesion to biliary stent materials, J Surg Res 62: (1) ((1996) ), 69–73. doi:10.1006/jsre.1996.0175. |
[27] | F.K. Chan, M. Suen, J.Y. Li and J.J. Sung, Bile immunoglobulins and blockage of biliary endoprosthesis: An immunohistochemical study, Biomed Pharmacother 52: (9) ((1998) ), 403–407. doi:10.1016/s0753-3322(99)80009-2. |
[28] | R. Kumar and H. Munstedt, Silver ion release from antimicrobial polyamide/ silver composites, Biomaterials 26: (14) ((2005) ), 2081–2088. doi:10.1016/j.biomaterials.2004.05.030. |
[29] | D. Lee, R.E. Cohen and M.F. Rubner, Antibacterial properties of Ag nanoparticle loaded multilayers and formation of magnetically directed antibacterial microparticles, Langmuir 21: (21) ((2005) ), 9651–9659. doi:10.1021/la0513306. |
[30] | J.M. Schierholz, L.J. Lucas, A. Rump and G. Pulverer, Efficacy of silver-coated medical devices, J Hosp Infect 40: (4) ((1998) ), 257–262. doi:10.1016/s0195-6701(98)90301-2. |
[31] | J.R. Morones, J.L. Elechiguerra, A. Camacho, K. Holt, J.B. Kouri, J.T. Ramirez , The bactericidal effect of silver nanoparticles, Nanotechnology 16: (10) ((2005) ), 2346–2353. doi:10.1088/0957-4484/16/10/059. |
[32] | C.Y. Flores, A.G. Minan, C.A. Grillo, R.C. Salvarezza, C. Vericat and P.L. Schilardi, Citrate-capped silver nanoparticles showing good bactericidal effect against both planktonic and sessile bacteria and a low cytotoxicity to osteoblastic cells, ACS Appl Mater Interfaces 5: (8) ((2013) ), 3149–3159. doi:10.1021/am400044e. |
[33] | J.S. Kim, E. Kuk, K.N. Yu, J.H. Kim, S.J. Park, H.J. Lee , Antimicrobial effects of silver nanoparticles, Nanomedicine 3: (1) ((2007) ), 95–101. doi:10.1016/j.nano.2006.12.001. |
[34] | R.E. Burrell, A scientific perspective on the use of topical silver preparations, Ostomy Wound Manage 49: (5A Suppl) ((2003) ), 19–24. |
[35] | H. Kumon, Pathogenesis and management of bacterial biofilms in the urinary tract, J Infect Chemother 2: ((1996) ), 18–28. doi:10.1007/BF02355193. |
[36] | J.W.C. Leung, G.T.C. Lau, J.J.Y. Sung and J.W. Costerton, Bacterial adherence to silver-coated stent material: An in vitro study, Gastrointest Endosc 38: (3) ((1992) ), 338–340. doi:10.1016/s0016-5107(92)70428-3. |
[37] | W. Wen, L.M. Ma, W. He, X.W. Tang, Y. Zhang, X. Wang , Silver-nanoparticle-coated biliary stent inhibits bacterial adhesion in bacterial cholangitis in swine, Hepatobiliary Pancreat Dis Int 15: (1) ((2016) ), 87–92. doi:10.1016/s1499-3872(15)60410-6. |
[38] | A. Yamabe, A. Irisawa, I. Wada, G. Shibukawa, M. Fujisawa, A. Sato , Application of a silver coating on plastic biliary stents to prevent biofilm formation: An experimental study using electron microscopy, Endosc Int Open 4: (10) ((2016) ), E1090–E1095. doi:10.1055/s-0042-115173. |
[39] | F. Yang, Z. Ren, Q. Chai, G. Cui, L. Jiang, H. Chen , A novel biliary stent coated with silver nanoparticles prolongs the unobstructed period and survival via anti-bacterial activity, Sci Rep 6: ((2016) ), 21714. doi:10.1038/srep21714. |
[40] | T.H. Lee, M.K. Jung, T.K. Kim, C.G. Pack, Y.K. Park, S.O. Kim , Safety and efficacy of a metal stent covered with a silicone membrane containing integrated silver particles in preventing biofilm and sludge formation in endoscopic drainage of malignant biliary obstruction: A phase 2 pilot study, Gastrointest Endosc 90: (4) ((2019) ), 663–672.e2. doi:10.1016/j.gie.2019.06.007. |
[41] | A.G. Speer, P.B. Cotton and K.D. MacRae, Endoscopic management of malignant biliary obstruction: Stents of 10 French gauge are preferable to stents of 8 French gauge, Gastrointest Endosc 34: (5) ((1988) ), 412–417. doi:10.1016/s0016-5107(88)71407-8. |
[42] | C. Soderlund and S. Linder, Covered metal versus plastic stents for malignant common bile duct stenosis: A prospective, randomized, controlled trial, Gastrointest Endosc 63: (7) ((2006) ), 986–995. doi:10.1016/j.gie.2005.11.052. |
[43] | M.A. Radzig, V.A. Nadtochenko, O.A. Koksharova, J. Kiwi, V.A. Lipasova and I.A. Khmel, Antibacterial effects of silver nanoparticles on gram-negative bacteria: Influence on the growth and biofilms formation, mechanisms of action, Colloids Surf B Biointerfaces 102: ((2013) ), 300–306. doi:10.1016/j.colsurfb.2012.07.039. |
[44] | Q.L. Feng, J. Wu, G.Q. Chen, F.Z. Cui, T.N. Kim and J.O. Kim, A mechanistic study of the antibacterial effect of silver ions on Escherichia coli and Staphylococcus aureus, J Biomed Mater Res 52: (4) ((2000) ), 662–668. doi:10.1002/1097-4636(20001215)52:4<662::aid-jbm10>3.0.co;2-3. |
[45] | J.K. Schluesener and H.J. Schluesener, Nanosilver: Application and novel aspects of toxicology, Arch Toxicol 87: (4) ((2013) ), 569–576. doi:10.1007/s00204-012-1007-z. |
[46] | A. Suzuki, An in vitro study on prophylaxis of biliary stent occlusion by silver containing stent, Gastroenterol Endosc 37: (7) ((1995) ), 1383–1393 (in Japanese, English abstract), doi:10.11280/gee1973b.37.1383. |
[47] | H.L. Liu, S.A. Dai, K.Y. Fu and S.H. Hsu, Antibacterial properties of silver nanoparticles in three different sizes and their nanocomposites with a new waterborne polyurethane, Int J Nanomedicine 5: ((2010) ), 1017–1028. doi:10.2147/IJN.S14572. |
[48] | J.W.C. Leung, G.T.C. Lau, J.J.Y. Sung and J.W. Costerton, Decreased bacterial adherence to silver-coated stent material: An in vitro study, Gastrointest Endosc 38: (3) ((1992) ), 338–340. doi:10.1016/s0016-5107(92)70428-3. |
[49] | T.H. Lee, B.S. Jang, M.K. Jung, C.G. Pack, J.H. Choi and D.H. Park, Fabrication of a silver particle-integrated silicone polymer-covered metal stent against sludge and biofilm formation and stent-induced tissue inflammation, Sci Rep 6: ((2016) ), 35446. doi:10.1038/srep35446. |
[50] | K. Knyrim, H.J. Wagner, J. Pausch , A prospective, randomised, controlled trial of metal stents for malignant obstruction of the common bile duct, Endoscopy 25: ((1993) ), 207–212. |
[51] | M. Kaassis, J. Boyer, R. Dumas , Plastic or metal stents for malignant stricture of the common bile duct? Results of a randomized prospective study, Gastrointest Endosc 57: ((2003) ), 178–182. |